- 1Department of Intensive Care Unit, The First Affiliated Hospital of Wenzhou Medical University, Wenzhou, China
- 2Key Laboratory of Intelligent Treatment and Life Support for Critical Diseases of Zhejiang Province, Wenzhou, China
- 3Wenzhou Key Laboratory of Critical Care and Artificial Intelligence, Wenzhou, China
- 4Zhejiang Engineering Research Center for Hospital Emergency and Process Digitization, Wenzhou, China
Background: Sepsis is a severe condition that often leads to complications such as acute kidney injury, which significantly increases morbidity and mortality rates. Septic AKI (S-AKI) is common in ICU patients and is associated with poor outcomes. However, there is no consensus on the optimal transfusion threshold for achieving the best clinical results. This retrospective study aims to investigate the relationship between different transfusion thresholds during hospitalization and the prognosis of septic AKI.
Methods: Data from patients with S-AKI was extracted from MIMIC-IV. Based on the lowest hemoglobin level 24 h before transfusion, patients were divided into high-threshold (≥7 g/L) and low-threshold (<7 g/L) groups. We compared the outcomes between these two groups, including hospital and ICU mortality rates as primary outcomes, and 30 days, 60 days, and 90 days mortality rates, as well as duration of stay in ICU and hospital as secondary outcomes.
Results: A total of 5,654 patients were included in our study. Baseline characteristics differed significantly between the two groups, with patients in the low-threshold group generally being younger and having higher SOFA scores. After performing propensity score matching, no significant differences in survival rates were found between the groups. However, patients in the low-threshold group had a longer overall hospital stay.
Conclusion: A lower transfusion threshold does not impact the mortality rate in S-AKI patients, but it may lead to a longer hospital stay.
Introduction
Sepsis is a life-threatening condition characterized by a dysregulated immune response to infection (1). Acute kidney injury (AKI) is a severe complication of sepsis, significantly contributes to morbidity and mortality. AKI occurs in approximately 30% to 50% of ICU patients with sepsis (2, 3). AKI presence in sepsis patients can increase in-hospital mortality risk by 6 to 8 times and extend hospital stays (3–5).
Sepsis-associated AKI, or septic AKI (S-AKI), represents a significant global public health challenge (2, 4). Characterized by rapid renal function decline, it leads to metabolic waste accumulation and electrolyte imbalances (4). The pathophysiology of S-AKI is complex and multifactorial, involving inflammation, microvascular dysfunction, and direct tubular injury (2–8). Particularly, hypoxia plays a crucial role in septic AKI’s pathogenesis (9).
Managing S-AKI requires a multidisciplinary approach, including prompt identification and treatment of the underlying infection, hemodynamic optimization, fluid resuscitation, and supportive care (2, 3, 6). du Cheyron et al. (10) reported an association between low initial hemoglobin levels and increased mortality risk in ARF patients. Experimental studies in animals demonstrate that fluid resuscitation (FR) improves hemodynamics but does not ameliorate renal microcirculation dysfunction (11). Blood transfusion, however, can restore renal microcirculation oxygenation in endotoxemia rat models, suggesting its potential benefits in renal function restoration (12).
The influence of transfusion thresholds on S-AKI prognosis remains under-explored. Presently, conclusive evidence is lacking on the optimal transfusion threshold for these patients. Studies indicate similar mortality rates and ischemic event incidences in septic patients with high and low transfusion thresholds (13–18). Consequently, the 2016 and 2021 Surviving Sepsis Campaign guidelines have eliminated higher hemoglobin targets for sepsis resuscitation, recommending a transfusion threshold below 7.0 g/dL (1, 19). There is no universally agreed-upon transfusion threshold specifically tailored for AKI patients (6). Transfusion decisions in AKI patients are multifactorial, depending on the patient’s hemodynamic stability, AKI cause, concurrent conditions, and overall clinical profile. Numerous guidelines support a restrictive transfusion strategy for critically ill patients, AKI patients included (15, 16, 20, 21). Research on the optimal red blood cell transfusion threshold in cardiac surgery-acute kidney injury (CS-AKI) patients suggests that a restrictive strategy (Hb <7.5 g/dL) is comparable to a liberal approach (22).
In S-AKI patients, blood transfusion is commonly used to treat anemia, aiming to enhance tissue oxygenation and offset anemia. However, the optimal transfusion threshold for these patients is still undefined. Although various studies suggest different transfusion thresholds, a consensus on the optimal level for the best clinical outcomes is yet to be established.
Consequently, this retrospective study aims to evaluate the relationship between transfusion thresholds (≥7 g/dL and <7 g/dL) and S-AKI prognosis. Our goal, through analyzing a large cohort of S-AKI patients, is to ascertain the link between higher or lower transfusion thresholds and improved clinical outcomes. This study aims to bridge the existing knowledge gap and offer significant insights into S-AKI management.
Methods
Database
This investigation adheres to the guidelines outlined in the strengthening the reporting of observational studies in epidemiology (STROBE) statement (23). Data was extracted from the publicly available Medical Information Mart for Intensive Care (MIMIC)-IV database, which contains comprehensive hospitalization records. This relational database includes genuine hospital stays for patients admitted to a tertiary academic medical center (Beth Israel Deaconess Medical Center) in the United States. It covers 76,540 ICU admissions related to 53,150 patients spanning the period from 2008 to 2019.
Ethical considerations
This research adhered to the ethical principles outlined in the Declaration of Helsinki and received approval from the Institutional Review Boards of both MIT and Beth Israel. The author XR completed the necessary training for using this database and obtained certification (Record ID 42912881).
Study cohort
We included all patients with suspected sepsis who met the diagnostic criteria for infection, as indicated by a sequential organ failure assessment (SOFA) score ≥2, based on the third international consensus definition of sepsis and septic shock (Sepsis-3.0) (24). The diagnosis of AKI was based on elevated serum creatinine levels and/or reduced urine output, following the KDIGO (kidney disease improving global outcomes) guidelines. AKI staging was determined using the KDIGO serum creatinine criteria (25). We included data from adult patients (aged ≥18 years) who were admitted to the ICU for at least 1 day during their initial hospitalization. Patients who did not receive transfusions were excluded. Subsequently, patients were divided into two groups based on their lowest hemoglobin value 24 h before transfusion: the higher threshold group (hemoglobin level ≥7 g/dL) and the lower threshold group (hemoglobin level <7 g/dL).
Covariates
Data was extracted from the database using Structured Query Language (SQL). We collected the following demographic parameters: gender, age, race (White, Black, other), SOFA, Charlson comorbidity index myocardial infarct, congestive heart failure, cerebrovascular disease, chronic pulmonary disease, diabetes mellitus, liver disease, renal disease, malignant cancer, vital signs (heart rate, mean arterial pressure, respiratory rate, SpO2) and laboratory values (Hb, PLT, WBC, INR, PT, APTT, creatinine, potassium, sodium, bicarbonate, lactate, PO2, PCO2, pH).
Outcomes
The primary outcomes of the study were hospital mortality and ICU mortality. The secondary outcomes included 30 days, 60 days, and 90 days mortality, as well as the duration of stay in the ICU and the hospital.
Statistical methods
Baseline characteristics and measurements of interest that are normally distributed are presented as mean ± standard deviation. Conversely, attributes not adhering to a normal distribution are represented by the median (M) and interquartile range (IQR). For comparative purposes, chi-square tests, one-way ANOVA, and Kruskal–Wallis tests were utilized to compare categorical, normally distributed, and non-normally distributed continuous variables, respectively. A multivariate Cox regression was employed to discern the correlation between two patient cohorts and the primary outcome.
To mitigate potential bias arising from missing data, the main outcome analysis was carried out after imputation of data using the Random Forest methodology. Propensity score matching (PSM), known for its ability to adjust for intergroup variations and eliminate bias in non-randomized comparative studies (26), was extensively implemented. In this study, a 1:1 matching was achieved through the optimal matching algorithm. Both Cox regression and PSM were utilized to adjust covariates, based on a nearest neighbour algorithm and a caliper width of 0.2 (27); all variables were adjusted via PSM. The standardized mean difference (SMD) was computed to assess the effectiveness of the PSM, with a threshold less than 0.1 considered acceptable. Within the matched cohorts, both the Kaplan–Meier method and the Cox proportional hazards regression were employed to compare hospital mortality, ICU mortality, and 30 days, 60 days, and 90 days mortality. The Wilcoxon rank-sum test was used to determine whether there was a significant difference in the median duration of stay in the ICU and the hospital between the two groups. Statistical significance was set at a two-sided p-value <0.05.
To mitigate the possibility of bias from the designated treatment and confounding conditions, all statistical analyses in our research was undertaken rendering to the provisions of R software, version 4.2.2.
Results
Basic information
After applying the exclusion criteria, a total of 5,654 patients were included in the original cohort, with 3,873 in the high threshold group and 1,781 in the lower threshold group. After performing PSM, successful matches were obtained for both the higher and lower threshold groups, resulting in 3,406 patients included in the current investigation. An flowchart depicting the screening and matching process is presented in the designated Figure 1. Demographic characteristics (such as gender, age, and ethnicity), SOFA scores, vital signs, concurrent medical conditions, and laboratory test results are compared before and after matching, as shown in Table 1. In the original cohort, patients in the low threshold group were relatively younger and had higher SOFA scores, as well as a higher incidence of comorbidities, compared to the high threshold group. A significant difference in certain baseline features was observed between the two groups prior to PSM. Using PSM in a 1:1 ratio, 1,703 patients in the low threshold group were matched with an equal number of patients in the high threshold group (Table 1). A graphical representation demonstrating the comparison curves of weighted data pre and post-matching is illustrated in Supplementary Figure S1.
Hemoglobin concentrations and transfusion
The distribution of hospital mortality among patients, stratified by transfusion thresholds, is depicted in Supplementary Figure S2. The hemoglobin threshold for the high-threshold group was 7.9 g/dL [interquartile range (IQR), 7.4–8.7], while the low-threshold group had a threshold of 6.6 g/dL (IQR, 6.2–6.8) (Figure 2B). We also compared the hemoglobin concentrations of patients within the first 24 h after ICU admission. The average hemoglobin concentration upon ICU admission differed significantly between the high-threshold and low-threshold groups [9.8 g/dL (IQR, 8.6–10.5) vs. 8.3 g/dL (IQR, 7.5–9.4), p < 0.001] (Figure 2A). The comparison of hemoglobin concentration trends from ICU admission to 14 days post-transfusion is shown in Figure 3. Compared to baseline levels, the hemoglobin concentration significantly increased on the first day post-transfusion (p < 0.001), and gradually stabilized in the following days.
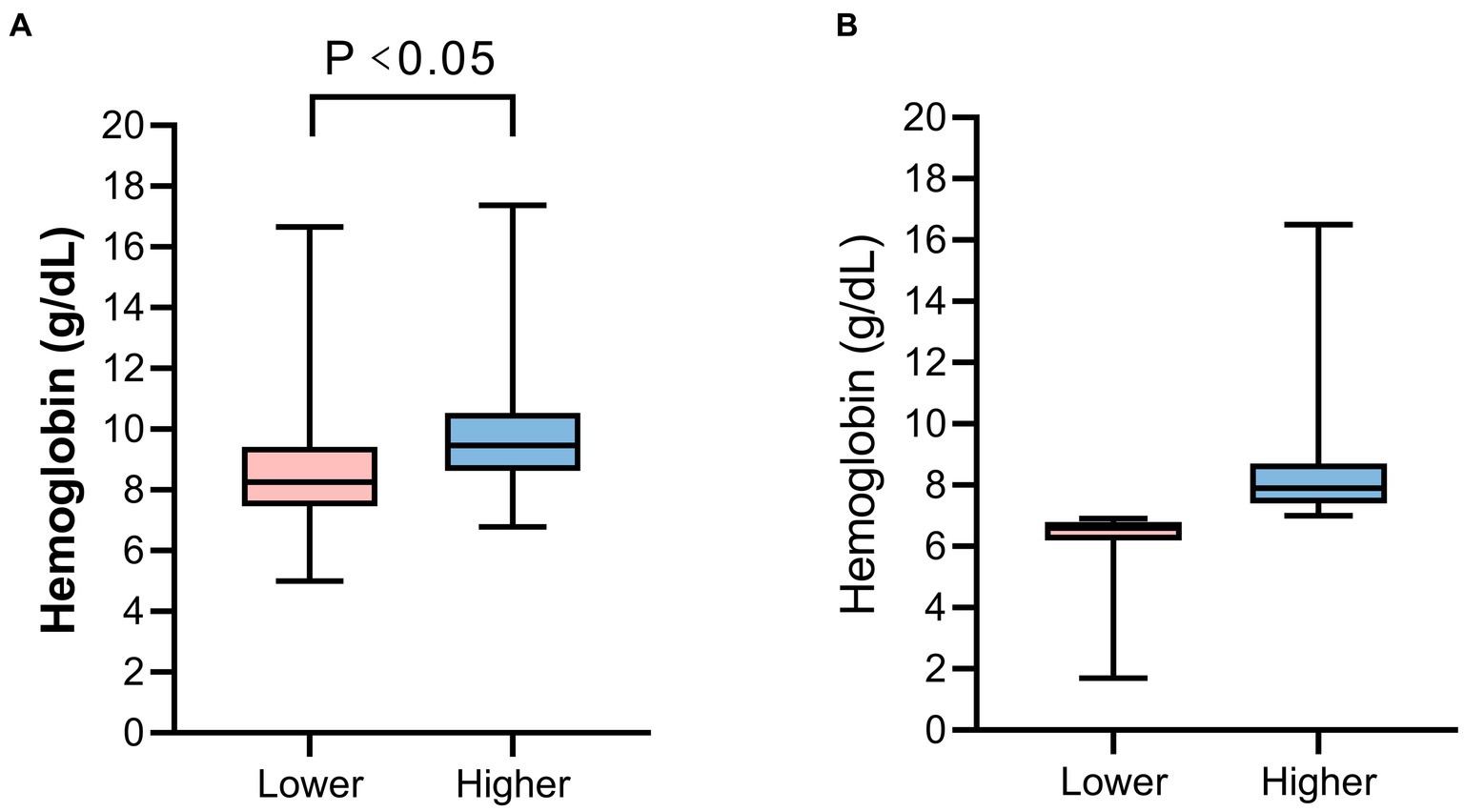
Figure 2. The hemoglobin concentration of patients. (A) The hemoglobin concentration upon ICU admission. (B) The hemoglobin threshold before blood transfusion between the two groups.
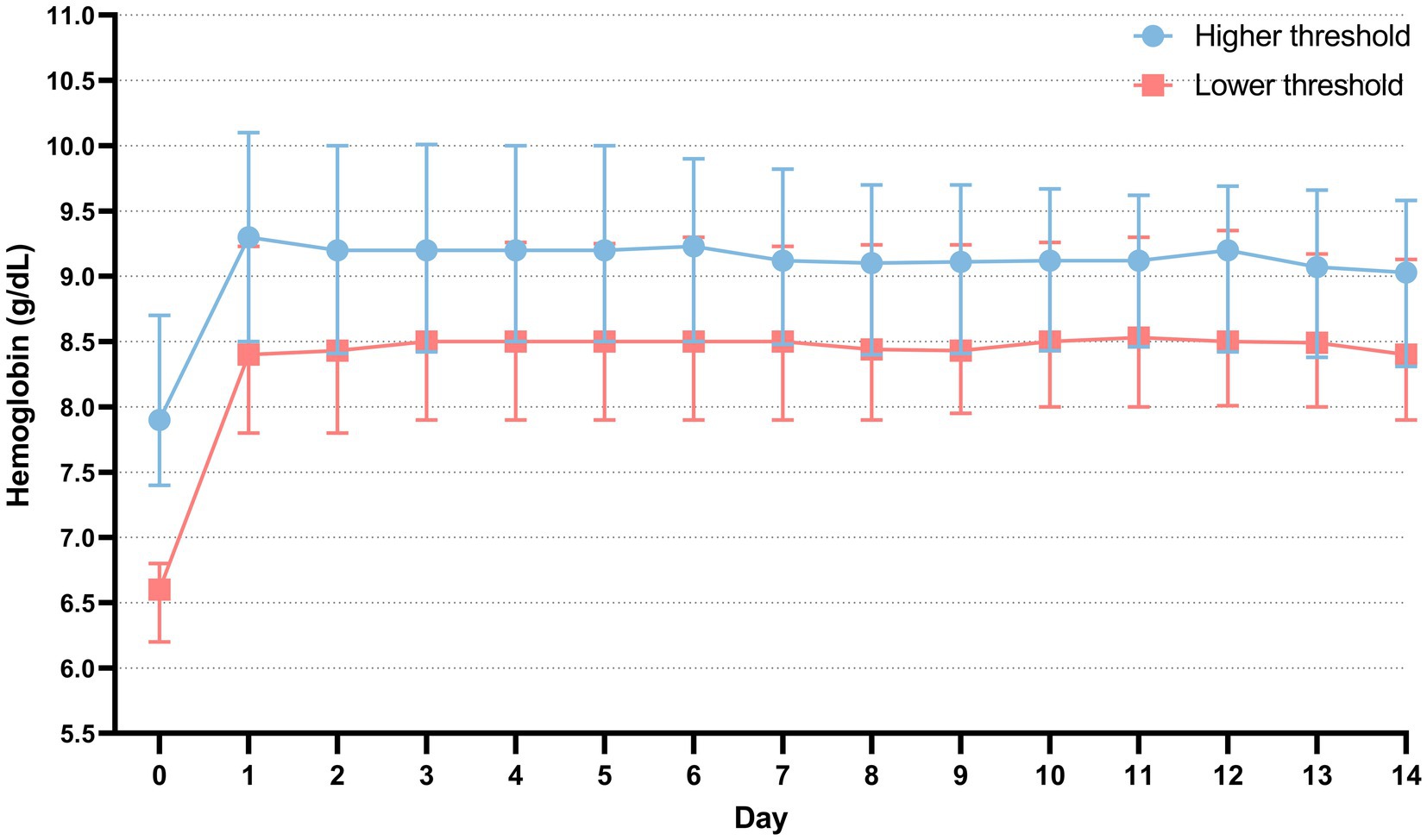
Figure 3. The comparison of hemoglobin concentration trends within 14 days post-transfusion and at ICU admission. Day 0 is the day of ICU admission. Day 1 is defined as the first day after blood transfusion. The data is presented as the median, 25th percentile, and 75th percentile.
Primary outcome
After performing PSM, the hospital mortality rates were determined to be 26.1% (445/1,703) in the higher threshold cohort and 27.1% (461/1,703) in the lower threshold cohort. The corresponding ICU mortality rates were determined to be 21.1% (358/1,703) in the higher threshold cohort and 19.3% (328/1,703) in the lower threshold cohort (Table 2). The Kaplan–Meier survival analysis shows no significant differences in survival probabilities between the high-threshold group and the low-threshold group in terms of both hospital mortality and ICU mortality, as illustrated in Figure 4.
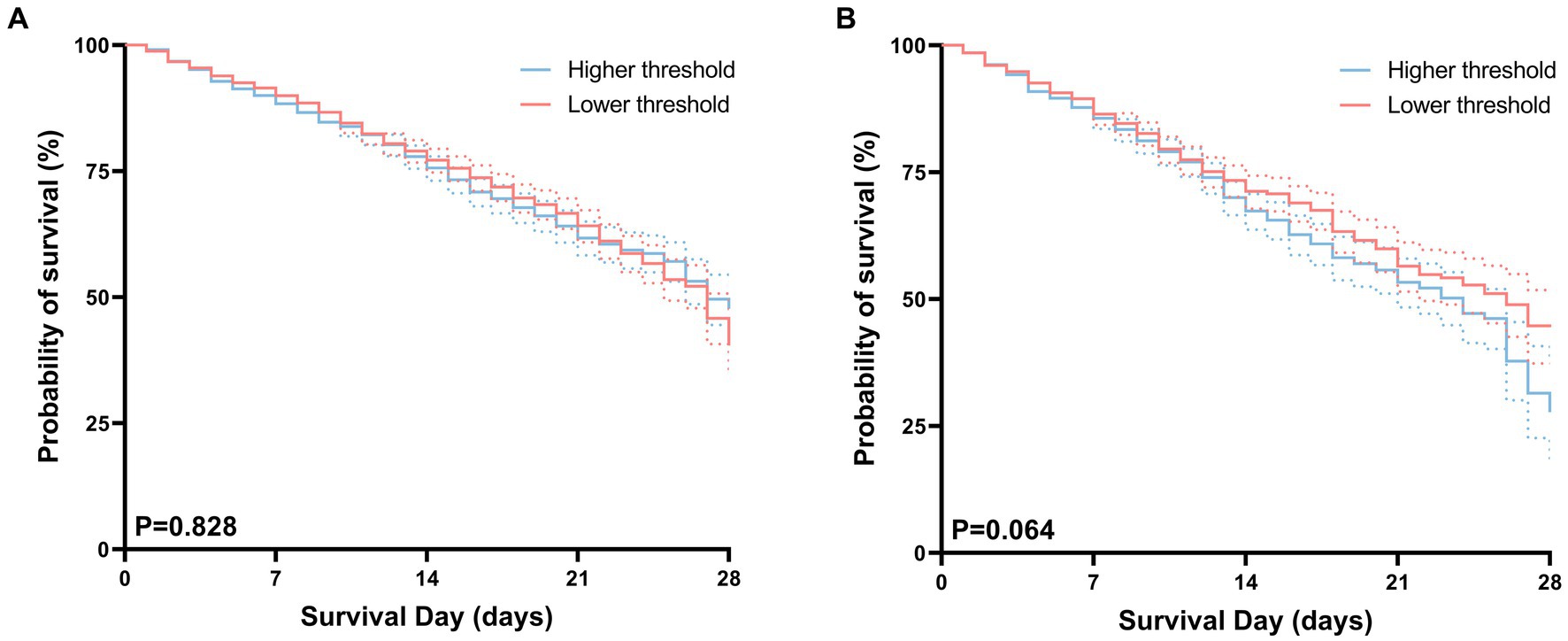
Figure 4. Kaplan–Meier curves for hospital (A), ICU (B) survival probability comparing patients with permissive lower threshold and higher threshold. For each curve, 95% confidence intervals (dotted lines) are shown.
Secondary outcomes
There were no substantial differences in 30 days, 60 days, and 90 days mortality between the high-threshold group and the low-threshold group (Figure 5). In this study, we compared the duration of hospital and intensive care unit (ICU) stays between two groups of patients. The results indicate that although there was no significant difference in the duration of ICU stays between the two groups (6.5 vs. 6.3, p = 0.347), but patients in the low-threshold group had a significantly longer overall hospital stay compared to their high-threshold group counterparts (15.7 vs. 13.8, p < 0.001) (Table 2).
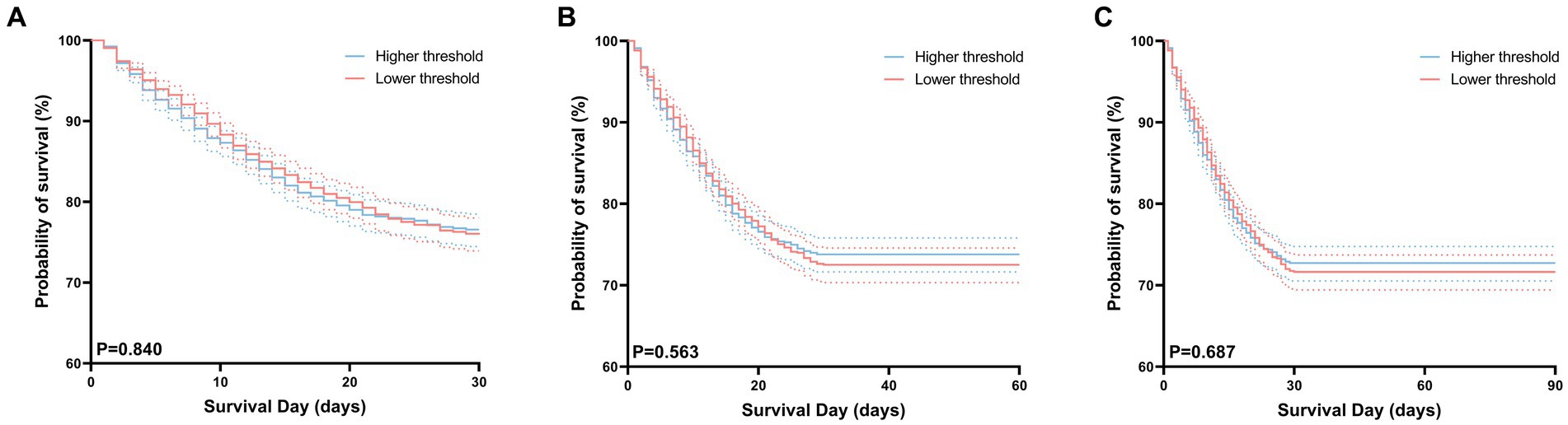
Figure 5. Kaplan–Meier curves for 30 days (A), 60 days (B), 90 days (C) survival probability comparing patients with permissive lower threshold and higher threshold. For each curve, 95% confidence intervals (dotted lines) are shown.
Subgroup analysis
Figure 6 illustrates the results of the subgroup analysis. Observations within each subgroup, categorized by gender, ethnicity, myocardial infarction, congestive heart failure, cerebrovascular disease, chronic pulmonary disease, liver disease, diabetes mellitus, renal disease, and malignant cancer, led to similar conclusions, indicating no difference in hospital mortality between the two groups.
Discussion
Our retrospective cohort study investigated the association between various transfusion thresholds and S-AKI prognosis. The analysis showed minimal differences in mortality and ICU stay duration between high and low transfusion threshold groups. Interestingly, despite no significant difference in ICU stays, patients in the lower transfusion threshold group experienced longer hospitalization periods. These findings shed light on the complexity of deciding on transfusion strategies for S-AKI patients, underscoring the need for individualized and context-specific approaches.
Theoretically, sepsis patients exhibit increased basal metabolic rates and oxygen requirements (28). Red blood cell transfusions can potentially correct anemia, enhance renal oxygen delivery, and improve microcirculation, indicating possible benefits (29). However, current research does not entirely corroborate the anticipated outcomes of red blood cell transfusion. Plataki’s et al. (30) study, examining AKI incidence post-transfusion in ICU septic patients, highlighted transfusion’s harmful effects. Repeated transfusions may cause complications such as febrile reactions, acute lung injury, electrolyte imbalances (hyperkalemia and hypocalcemia), and iron overload. Some studies indicate that transfusions, through immune mechanisms and overload, may cause “acute kidney injury” akin to lung injury, potentially leading to or worsening kidney damage (30–32).
Recently, many studies have compared restrictive versus liberal transfusion thresholds (33). The combined data from these studies indicate that, in terms of mortality and adverse reactions, restrictive transfusion thresholds are not inferior to liberal thresholds (34, 35).
Understanding the complex physiological interplay in S-AKI and the subtle effects of different transfusion thresholds may lead to more precise and effective management. Lower transfusion thresholds, not impacting ICU and hospital mortality rates, support the trend towards conservative transfusion in critically ill patients, aligning with guidelines like the “surviving sepsis campaign” (1). However, lower transfusion thresholds could result in prolonged hospital stays, increasing medical costs and complication risks. This may be attributed to the longer recovery time needed for anemic conditions in the low threshold group, or insufficient hemoglobin levels failing to meet clinical needs, causing more complications and extended recovery. Additionally, prolonged hospitalization raises the risk of hospital-acquired infections and other complications. The primary challenge of conservative transfusion strategies (low thresholds) lies in balancing transfusion needs against potential risks. Reducing transfusion frequency may lower complication risks but could delay anemia correction in some patients. This strategy requires finding a balance between reducing unnecessary transfusions and ensuring sufficient blood supply to meet the physiological needs of patients. Future guidelines are expected to provide detailed guidance on transfusion timing and methods, particularly for sepsis with AKI, emphasizing individualized patient assessments to tailor treatment plans.
Naturally, our study has some limitations. Firstly, it is inherently retrospective, which means it may be vulnerable to biases from unmeasured and residual confounding factors, despite our efforts to make comprehensive adjustments for the compared groups. Secondly, the single-center data collection may limit the generalizability of our findings to broader patient populations.
In conclusion, our findings suggest that lower transfusion thresholds may not adversely affect survival in critically ill S-AKI patients, aligning with current clinical thresholds, but could lead to longer hospital stays. Future multicenter, randomized trials are needed to further validate these findings.
Going forward, the paradigm of transfusion will not be merely dichotomized into restrictive or “liberal” strategies. Rather, it will pivot towards a more nuanced approach, tailoring “individualized” transfusion strategies based on the specific disease type, patient characteristics, and prevailing clinical conditions. This shift aims to maximize patients’ long-term prognosis.
Conclusion
In S-AKI patients, a conservative transfusion strategy with a threshold of <7 g/dL does not adversely affect survival rates compared to a more aggressive approach. However, this approach is linked to longer hospital stays.
Data availability statement
The raw data supporting the conclusions of this article will be made available by the authors, without undue reservation.
Ethics statement
The studies involving humans were approved by Boards of the Beth Israel Deaconess Medical Center and Massachusetts Institute of Technology. The studies were conducted in accordance with the local legislation and institutional requirements. Written informed consent for participation was not required from the participants or the participants’ legal guardians/next of kin because written informed consent for participation was not required for this study in accordance with the national legislation and the institutional requirements.
Author contributions
XR: Writing – original draft, Writing – review & editing. BW: Writing – original draft, Writing – review & editing. YG: Writing – original draft, Writing – review & editing. JW: Writing – original draft, Writing – review & editing. XY: Writing – original draft, Writing – review & editing. CL: Writing – original draft, Writing – review & editing. JP: Writing – original draft, Writing – review & editing.
Funding
The author(s) declare financial support was received for the research, authorship, and/or publication of this article. This research was supported by National Natural Science Foundation of China (Grant No. 82272204). The 5G Network-Based Platform for Precision Emergency Medical Care in Regional Hospital Clusters MIIT PRC (2020 No. 78). Key Clinical Specialty of Zhejiang Province (Critical Care Medicine, Y2022). “Pioneer” and “Leading Goose” R&D Program of Zhejiang (2023C03084).
Acknowledgments
The authors would like to thank the participants, developers and investigators associated with the Medical Information Mart for Intensive Care (MIMIC)-IV database.
Conflict of interest
The authors declare that the research was conducted in the absence of any commercial or financial relationships that could be construed as a potential conflict of interest.
Publisher’s note
All claims expressed in this article are solely those of the authors and do not necessarily represent those of their affiliated organizations, or those of the publisher, the editors and the reviewers. Any product that may be evaluated in this article, or claim that may be made by its manufacturer, is not guaranteed or endorsed by the publisher.
Supplementary material
The Supplementary material for this article can be found online at: https://www.frontiersin.org/articles/10.3389/fmed.2023.1308275/full#supplementary-material
References
1. Evans, L, Rhodes, A, Alhazzani, W, Antonelli, M, Coopersmith, CM, French, C, et al. Surviving sepsis campaign: international guidelines for management of sepsis and septic shock 2021. Intensive Care Med. (2021) 47:1181–247. doi: 10.1007/s00134-021-06506-y
2. Schrier, RW, and Wang, W. Acute renal failure and sepsis. N Engl J Med. (2004) 351:159–69. doi: 10.1056/NEJMra032401
3. Peerapornratana, S, Manrique-Caballero, CL, Gómez, H, and Kellum, JA. Acute kidney injury from sepsis: current concepts, epidemiology, pathophysiology, prevention and treatment. Kidney Int. (2019) 96:1083–99. doi: 10.1016/j.kint.2019.05.026
4. Patel, S, Puri, N, and Dellinger, RP. Sepsis management for the nephrologist. Clin J Am Soc Nephrol. (2022) 17:880–9. doi: 10.2215/CJN.14381121
5. Gómez, H, and Kellum, JA. Sepsis-induced acute kidney injury. Curr Opin Crit Care. (2016) 22:546–53. doi: 10.1097/MCC.0000000000000356
6. Khwaja, A. KDIGO clinical practice guidelines for acute kidney injury. Nephron Clin Pract. (2012) 120:c179–84. doi: 10.1159/000339789
7. Gomez, H, Ince, C, De Backer, D, Pickkers, P, Payen, D, Hotchkiss, J, et al. A unified theory of sepsis-induced acute kidney injury: inflammation, microcirculatory dysfunction, bioenergetics, and the tubular cell adaptation to injury. Shock. (2014) 41:3–11. doi: 10.1097/SHK.0000000000000052
8. Chen, S, Hao, X, Chen, G, Liu, G, Yuan, X, Shen, P, et al. Effects of mesencephalic astrocyte-derived neurotrophic factor on sepsis-associated acute kidney injury. World J Emerg Med. (2023) 14:386–92. doi: 10.5847/wjem.j.1920-8642.2023.077
9. Johannes, T, Mik, EG, and Ince, C. Nonresuscitated endotoxemia induces microcirculatory hypoxic areas in the renal cortex in the rat. Shock. (2009) 31:97–103. doi: 10.1097/SHK.0b013e31817c02a5
10. du Cheyron, D, Parienti, JJ, Fekih-Hassen, M, Daubin, C, and Charbonneau, P. Impact of anemia on outcome in critically ill patients with severe acute renal failure. Intensive Care Med. (2005) 31:1529–36. doi: 10.1007/s00134-005-2739-5
11. Legrand, M, Mik, EG, Balestra, GM, Lutter, R, Pirracchio, R, Payen, D, et al. Fluid resuscitation does not improve renal oxygenation during hemorrhagic shock in rats. Anesthesiology. (2010) 112:119–27. doi: 10.1097/ALN.0b013e3181c4a5e2
12. Zafrani, L, Ergin, B, Kapucu, A, and Ince, C. Blood transfusion improves renal oxygenation and renal function in sepsis-induced acute kidney injury in rats. Crit Care. (2016) 20:406. doi: 10.1186/s13054-016-1581-1
13. Rygård, SL, Holst, LB, Wetterslev, J, Winkel, P, Johansson, PI, Wernerman, J, et al. Long-term outcomes in patients with septic shock transfused at a lower versus a higher haemoglobin threshold: the TRISS randomised, multicentre clinical trial. Intensive Care Med. (2016) 42:1685–94. doi: 10.1007/s00134-016-4437-x
14. Carson, JL, Sieber, F, Cook, DR, Hoover, DR, Noveck, H, Chaitman, BR, et al. Liberal versus restrictive blood transfusion strategy: 3 years survival and cause of death results from the FOCUS randomised controlled trial. Lancet. (2015) 385:1183–9. doi: 10.1016/S0140-6736(14)62286-8
15. Holst, LB, Petersen, MW, Haase, N, Perner, A, and Wetterslev, J. Restrictive versus liberal transfusion strategy for red blood cell transfusion: systematic review of randomised trials with meta-analysis and trial sequential analysis. BMJ. (2015) 350:h1354. doi: 10.1136/bmj.h1354
16. Fominskiy, E, Putzu, A, Monaco, F, Scandroglio, AM, Karaskov, A, Galas, FR, et al. Liberal transfusion strategy improves survival in perioperative but not in critically ill patients. A meta-analysis of randomised trials. Br J Anaesth. (2015) 115:511–9. doi: 10.1093/bja/aev317
17. Holst, LB, Haase, N, Wetterslev, J, Wernerman, J, Guttormsen, AB, Karlsson, S, et al. Lower versus higher hemoglobin threshold for transfusion in septic shock. N Engl J Med. (2014) 371:1381–91. doi: 10.1056/NEJMoa1406617
18. Walsh, TS, Boyd, JA, Watson, D, Hope, D, Lewis, S, Krishan, A, et al. Restrictive versus liberal transfusion strategies for older mechanically ventilated critically ill patients: a randomized pilot trial. Crit Care Med. (2013) 41:2354–63. doi: 10.1097/CCM.0b013e318291cce4
19. Rhodes, A, Evans, LE, Alhazzani, W, Levy, MM, Antonelli, M, Ferrer, R, et al. Surviving sepsis campaign: international guidelines for management of sepsis and septic shock: 2016. Intensive Care Med. (2017) 43:304–77. doi: 10.1007/s00134-017-4683-6
20. Hébert, PC, Wells, G, Blajchman, MA, Marshall, J, Martin, C, Pagliarello, G, et al. A multicenter, randomized, controlled clinical trial of transfusion requirements in critical care. Transfusion requirements in critical care investigators, Canadian critical care trials group. N Engl J Med. (1999) 340:409–17. doi: 10.1056/NEJM199902113400601
21. Cable, CA, Razavi, SA, Roback, JD, and Murphy, DJ. RBC transfusion strategies in the ICU: a concise review. Crit Care Med. (2019) 47:1637–44. doi: 10.1097/CCM.0000000000003985
22. Peng, K, McIlroy, DR, Bollen, BA, Billings, FT, Zarbock, A, Popescu, WM, et al. Society of cardiovascular anesthesiologists clinical practice update for management of acute kidney injury associated with cardiac surgery. Anesth Analg. (2022) 135:744–56. doi: 10.1213/ANE.0000000000006068
23. von Elm, E, Altman, DG, Egger, M, Pocock, SJ, Gøtzsche, PC, and Vandenbroucke, JP. The strengthening the reporting of observational studies in epidemiology (STROBE) statement: guidelines for reporting observational studies. Lancet. (2007) 370:1453–7. doi: 10.1016/S0140-6736(07)61602-X
24. Singer, M, Deutschman, CS, Seymour, CW, Shankar-Hari, M, Annane, D, Bauer, M, et al. The third international consensus definitions for sepsis and septic shock (Sepsis-3). JAMA. (2016) 315:801–10. doi: 10.1001/jama.2016.0287
25. Ostermann, M, Bellomo, R, Burdmann, EA, Doi, K, Endre, ZH, Goldstein, SL, et al. Controversies in acute kidney injury: conclusions from a kidney disease: improving global outcomes (KDIGO) conference. Kidney Int. (2020) 98:294–309. doi: 10.1016/j.kint.2020.04.020
26. Zhang, Z. Propensity score method: a non-parametric technique to reduce model dependence. Ann Transl Med. (2017) 5:7. doi: 10.21037/atm.2016.08.57
27. Austin, PC. A comparison of 12 algorithms for matching on the propensity score. Stat Med. (2014) 33:1057–69. doi: 10.1002/sim.6004
28. Gilbert, EM, Haupt, MT, Mandanas, RY, Huaringa, AJ, and Carlson, RW. The effect of fluid loading, blood transfusion, and catecholamine infusion on oxygen delivery and consumption in patients with sepsis. Am Rev Respir Dis. (1986) 134:873–8. doi: 10.1164/arrd.1986.134.5.873
29. den Uil, CA, Lagrand, WK, Spronk, PE, and Simoons, ML. Does red blood cell transfusion result in a variate microvascular response in sepsis? Crit Care Med. (2007) 35:2464–5. doi: 10.1097/01.CCM.0000284756.78940.C1
30. Plataki, M, Kashani, K, Cabello-Garza, J, Maldonado, F, Kashyap, R, Kor, DJ, et al. Predictors of acute kidney injury in septic shock patients: an observational cohort study. Clin J Am Soc Nephrol. (2011) 6:1744–51. doi: 10.2215/CJN.05480610
31. Engoren, M. Does erythrocyte blood transfusion prevent acute kidney injury? Propensity-matched case control analysis. Anesthesiology. (2010) 113:1126–33. doi: 10.1097/ALN.0b013e181f70f56
32. Legrand, M, Dupuis, C, Simon, C, Gayat, E, Mateo, J, Lukaszewicz, AC, et al. Association between systemic hemodynamics and septic acute kidney injury in critically ill patients: a retrospective observational study. Crit Care. (2013) 17:R278. doi: 10.1186/cc13133
33. Carson, JL, Guyatt, G, Heddle, NM, Grossman, BJ, Cohn, CS, Fung, MK, et al. Clinical practice guidelines from the AABB: red blood cell transfusion thresholds and storage. JAMA. (2016) 316:2025–35. doi: 10.1001/jama.2016.9185
34. Vlaar, AP, Oczkowski, S, de Bruin, S, Wijnberge, M, Antonelli, M, Aubron, C, et al. Transfusion strategies in non-bleeding critically ill adults: a clinical practice guideline from the European Society of Intensive Care Medicine. Intensive Care Med. (2020) 46:673–96. doi: 10.1007/s00134-019-05884-8
Keywords: transfusion threshold, septic acute kidney injury, hemoglobin concentration, S-AKI, sepsis transfusion threshold, sepsis
Citation: Ruan X, Wang B, Gao Y, Wu J, Yu X, Liang C and Pan J (2023) Assessing the impact of transfusion thresholds in patients with septic acute kidney injury: a retrospective study. Front. Med. 10:1308275. doi: 10.3389/fmed.2023.1308275
Edited by:
Penglin Ma, Guiqian International General Hospital, ChinaReviewed by:
Hong Jun Kang, First Affiliated Hospital of Chinese PLA General Hospital, ChinaMeili Duan, Capital Medical University, China
Copyright © 2023 Ruan, Wang, Gao, Wu, Yu, Liang and Pan. This is an open-access article distributed under the terms of the Creative Commons Attribution License (CC BY). The use, distribution or reproduction in other forums is permitted, provided the original author(s) and the copyright owner(s) are credited and that the original publication in this journal is cited, in accordance with accepted academic practice. No use, distribution or reproduction is permitted which does not comply with these terms.
*Correspondence: Jingye Pan, panjingye@wmu.edu.cn