- 1Lee Kong Chian School of Medicine, Nanyang Technological University, Singapore, Singapore
- 2National Skin Centre, Singapore, Singapore
1. Introduction
Immunotherapy for skin conditions has a long and successful history. While the main strategy for treating inflammatory conditions is local or systemic immunosuppression, immunotherapy aims to stimulate parts or all of the immune system to bring about a therapeutic response. The immune system contains multiple, redundant avenues of checks and balances, and some immune cells have immunomodulatory or regulatory roles. These cells are targeted by immunotherapy in inflammatory skin conditions. Immunotherapy is effective in treating some hair loss disorders, supporting recent findings that the hair cycle is influenced by immune cells (1, 2).
The normal hair cycle consists of sequential growth (anagen), regression (catagen) and rest (telogen) phases. In humans, the anagen phase (9–10 years) reflects the growing hair shaft, while the end of telogen (2–3 months) is marked by hair shedding. When the hair cycle is disrupted, premature anagen-to-catagen transition results in majority of hair follicles (HFs) entering telogen and shedding concurrently, causing significant, noticeable hair loss.
The immune system is closely associated with the HF, with macrophages and mast cells described in the perifollicular dermis (3, 4). Skin-resident macrophages clear apoptotic cell fragments following catagen (5) and may also interact with hair follicle stem cells (HFSC) to maintain quiescence during telogen (1). The dynamic nature of the hair cycle results in a constant flux of antigens, both self and non-self, from the manufacture of the hair shaft and the infundibular connection to the outside world, respectively. To protect the hair bulb from inappropriate immune attack, the HF has evolved a status of relative “immune privilege” (IP). This was demonstrated elegantly by Billingham et al. decades ago showing that the presence of HFs in allografts was sufficient to prevent immune rejection of melanocytes in transplantation experiments on guinea pigs (6). Various mechanisms have since been proposed for the IP of the HF, with the most prominent one being a downregulation of MHC Class I expression (7). Hair loss disorders are associated with disruptions and changes in the immune milieu of the HF (8, 9), and conversely, immunotherapy has been utilized to promote HF regrowth and regeneration.
2. The immune system interaction with the hair follicle niche
With evidence of immune cells being associated with the HF, it is reasonable to hypothesize that changes in immune cell composition and activity around HFs can affect HFSC and the hair cycle. However, the direct influence of immune cells in promoting hair regrowth and regeneration in humans is still unknown. In mice, regulatory T-cells (T-regs) promote anagen re-entry via Notch signaling (10), and macrophages may release Wnt factors to stimulate anagen under certain conditions (11). Wound-induced HF neogenesis (WIHN) is another phenomenon in rodents whereby brand-new HFs arise from scar tissue of a wound. Cotsarelis and Ito showed that relatively large wounds in the back skin of mice initially heal without HFs, but these scars are soon populated by de novo HFs via WIHN (12). Small cutaneous wounds in mice upregulate Wnt and Shh pathways (13), while larger wounds recruit dermal γδ-T-cells (14) and M2 macrophages (15) to promote WIHN. While the mouse's fur coat and human scalp hair differ in their stage of hair cycle, fundamental processes and pathways are likely conserved, and human HFs may respond in a similar manner to microtrauma.
In humans, crude immune stimulation has been shown to induce hair growth. Friction and irritation are known causes of hypertrichosis, and excessive hair growth occurs after limb fixation with plaster cast application (16, 17), and on burned skin borders (18). Allergic contact dermatitis to wig adhesives is reported to have a therapeutic effect in alopecia areata (AA) (19), forming the basis of topical immunotherapy for AA. Contact dermatitis introduces “antigenic competition” which recruits suppressor T-cells and macrophages, producing an immunomodulatory environment and dampening the autoimmune attack on HFs (20). These reports point to a role for microtrauma to elicit a permissive environment for hair regrowth and regeneration.
Newer treatment modalities for AGA harnessing the effects of microtrauma include platelet-rich plasma (PRP), microneedling and ablative and non-ablative lasers, which aim to reproduce the effects of wounding in areas of alopecia. In PRP, intra-dermal injection of activated platelets release growth factors near HFs (21), including transforming growth factor-β (TGF-β), epidermal growth factor, basic fibroblast growth factor (FGF), vascular endothelial growth factor (VEGF), platelet-derived growth factor (PDGF), and insulin-like growth factor-1, which support the anagen phase of the hair cycle. The efficacy of PRP appears operator- and protocol-dependent, but has been shown in systematic reviews to promote hair regrowth modestly (22). In randomized, split-scalp studies of PRP vs. sham injections, hair density also increased among the controls, suggesting microtrauma alone may stimulate hair regrowth (23). Indeed, several groups have demonstrated that microneedling may be effective in treating AGA (24) and AA (25). Both ablative and non-ablative settings of Er:YAG lasers have been used in AGA and AA, with promising results (26–28).
The efficacy of these treatments supports the hypothesis that the microenvironment of HFSC can be manipulated to promote hair regrowth. Immune cells are likely involved in the process of microtrauma. The treatments above, however, are non-specific. They induce an irritant, allergic or wounding response that varies between patients, resulting in inconsistent effectiveness. We are still in the process of understanding this process, and fine tuning the procedures and patient selection to obtain the best possible clinical outcomes.
3. Targeted immunotherapy for hair regrowth
Targeted immunotherapy in promoting hair regeneration is expected to grow with understanding of the detailed control of the human hair cycle. The first mode of targeted immunotherapy has been applied for AA, as we know most about its immunopathology.
3.1. Immunosuppressive therapies with stimulatory action in other parts of the immune system or HFSC
3.1.1. JAK inhibitors
Janus kinase (JAK) inhibitors have revolutionized therapeutics in dermatology, successfully utilized in many inflammatory skin conditions including atopic dermatitis. The JAK-STAT pathway is an integral component of AA pathophysiology, downstream of interferon-gamma (IFN-γ) and interleukin (IL)-15 signaling.
Baricitinib is the first FDA-approved drug for AA in 2022. In two concurrent phase III randomized controlled trials (BRAVE-AA1 and BRAVE-AA2), baricitinib achieved SALT scores <20 at 52 weeks with a good safety profile (29). Baricitinib, which inhibits JAK1 and JAK2 signaling is effective in long-standing AA resistant to traditional therapies (30), besides being safe and effective in pediatric AA (31). Increasingly specific JAK inhibitors (JAK1 ivarmacitinib, JAK3 ritlecitinib, and JAK1/Tyk2 beprocitinib) (29, 32) are investigated for use in AA, making treatment more targeted.
Topical JAK inhibition not only reverses hair loss in AA mice, but also induces the telogen-to-anagen transition in disease-free C56Bl/6 mice (33). This suggests that the JAK-STAT pathway is also involved in normal hair cycles (34), leading to the discovery of a distinct subset of TREM2+ macrophages that maintain HFSC quiescence by secreting oncostatin M (1). Pharmacological, immunological and genetic inhibition of these macrophages sufficiently induced anagen in mice. Whether a similar mechanism is present in the human HF niche is unknown, but STAT3 is upregulated in AGA scalps (35).
The Wnt/β-catenin signaling pathway is the major pathway in activating DPCs, which are crucial in the hair bulb and bulge interaction for anagen initiation. Treatment of HFs with ruxolitinib, a JAK1/2 inhibitor, stimulates the expression of β-catenin mRNA, upregulating Wnt/β-catenin signaling. Further, proinflammatory cytokines of AA, namely IFN-γ-induced caspase-1, IL-1β, IL-15 and IL-18 are also suppressed by JAK1/2 inhibition (36). JAK inhibition in AA thus may have more than an immunosuppressive role, and may have immunotherapeutic roles in stimulation of DP and/or HFSC.
In IFN-treated dermal papilla (DP) culture, ruxolitinib was also shown to downregulate MHC class I expression, contributing to partial IP restoration. In addition, ruxolitinib stimulated several growth factors, including FGF7, that supported DP cell survival which translates to a hair growth-permissive microenviroment independent of its immunosuppressive properties (36).
The in vivo effects of JAK inhibition have yet to be evaluated, partly due to the challenges in analyzing and quantifying the hair cycle, as follicular units are asynchronous and hair cycle phases last months to years. Existing JAK inhibitors also penetrate skin insufficiently (37).
Other challenges to JAK inhibition include disease recurrence after discontinuation and balancing long-term usage against side effects of infections, marrow suppression, transaminitis, and lipid abnormalities (38).
3.1.2. Statins
Statins have been proposed for treating various dermatologic conditions characterized by ingress of activated leukocytes into the skin, including AA (39). In a pilot study, a combination of simvastatin and ezetimibe reduced hair loss and resulted in stable remission in AA mice model, with an increase in FOXP3+ T-regs (40). Simvastatin may improve AA through multidirectional pro- and anti-inflammatory activities. These include increasing Th2 cytokine secretion, upregulating T-reg cells in mice (39), downregulating Th1 cytokine secretion via JAK-STAT pathway modulation (41), inhibiting leukocyte activation, adhesion and migration (42), activating Wnt/β-catenin signaling pathway (43), and downregulating reactive oxygen species production (44). However, larger placebo-controlled trials are still required, as these findings were not always reproducible by other authors (45).
3.2. Biologic therapies
Monoclonal antibody therapy is not as well established for AA as for dermatological conditions like psoriasis and eczema. Most TNF inhibitors are ineffective for AA, while dupilumab was found to be modestly effective in a Phase IIa trial (46). Although AA is associated with atopic dermatitis and psoriasis, treatment for the latter does not always improve AA (47), and may sometimes worsen hair loss (48).
The contrasting effects of biological therapies and JAK inhibitors in AA suggest that current targets of monoclonal antibodies (TNF-α, IL-17, IL-23 and IL-4 signaling) may support the HF immune privileged microenvironment, and an unbalanced blockade of these pathways leads to cytokine imbalance and AA (49). JAK-inhibition is more focused on immune mechanisms that share JAK-STAT pathways, which are more frequently associated with “active” T-cell directives on the HF.
Nivolumab is an anti-PD1 (programmed cell death-1) monoclonal antibody effective in many cancers, including melanoma. It releases the inhibition of autoreactive T-cells, allowing immune system clearance of tumor cells. This same mechanism has been reported to cause AA in these patients (50). The PD-1/PD-L1 pathway has also been implicated in T-cell exhaustion accompanying response to AA treatment with JAK-inhibitors (51). Exploring this pathway in AA may lead to new targets for biological therapy.
3.3. IL-2 complex treatment
A pilot study using low dose IL-2 to expand T-reg populations in severe AA showed initial promise (52). T-regs suppress autoreactive NKG2D+ T-cells that attack HFs and promote hair regrowth by inducing anagen. In mice models, intradermal injection of IL-2/anti-IL-2 antibody complex (IL-2c) efficiently stimulates T-reg proliferation by 8- to 10-fold in the skin. T-regs have also been shown to promote anagen via Jagged-1 expression (10).
A prospective randomized control study with low dose IL-2 was conducted which showed limited efficacy of this treatment (53). In murine models, while the fold ratio of CD8 T-cells over T-regs was also markedly reduced post-IL-2c treatment, CD8 T-cells remained around HFs, including NKG2D+ T-cells, in established AA mouse models (54). Despite no significant reduction in IL-10 or TGF-β secretion, the expanded T-regs were not sufficient to inhibit CD8 T-cell proliferation in established AA, resulting in neither anagen induction nor AA reversal. Further studies are warranted to investigate the role of IL-2/IL-2c in the treatment of AA. Early IL-2c therapy has been hypothesized to be effective in acute AA, which may slow disease progression but may require adjunctive treatments for more chronic, established cases.
3.4. Prevention or restoration of IP collapse
AA develops when the IP of the HF collapses, due to ectopic MHC class-1 expression induced by IFN-γ (55). Ex vivo- application of TGF-β1, α-MSH and the drug FK506 (Tacrolimus) have been found to suppress MHC Class I expression in cultured HF organ cultures, likely through suppressing mRNA transcription (56). α-MSH, which also has immunosuppressive properties, is also increased in AA lesional scalp post-UVA phototherapy (57). While systemic calcineurin inhibitors like ciclosporin have been effective in treating severe recalcitrant AA, topical tacrolimus/FK506 has proven to be less reliable (58), and exploring this method of restoring HF IP may further expand our immunotherapy repertoire.
3.5. Harnessing microtrauma
For AGA, there is currently a wide variety of modalities for inducing microtrauma to promote hair regrowth, including fractional lasers and microneedling. While these may be non-targeted, they enable more targeted drug delivery when combined with topical treatments like minoxidil or PRP. Release of growth factors with microtrauma [such as PDGF, VEGF, β-catenin, Wnt3a and Wnt10b (59)] has been postulated to lead to angiogenesis, dermal thickening, adipogenesis and HF stem cell activation to promote anagen.
Identifying the key factors and signaling pathways that promote microtrauma-induced hair regrowth will pave the way to the next phase of targeted immunotherapy to treat hair loss. Inclusion of these growth factors, or PRP, into customized microneedles is currently explored as a delivery method for treating AGA (60). These factors, as well as other signaling molecules like nucleic acids, membrane receptors or co-factors, can be packaged in exosomes to deliver a targeted signal of hair regeneration (61, 62).
4. Conclusion
Targeted immunotherapy (Figure 1) is a promising form of therapy for hair regrowth and regeneration, targeting immune cells that support and influence the hair cycle. Refining our current methods of immunotherapy will make these treatments more accessible to a wider population of patients suffering hair loss, with potentially fewer side effects. Further studies and controlled trials are required before they can be incorporated into clinical practice. If successful, targeted immunotherapy will provide hope for patients struggling with or have failed traditional treatments.
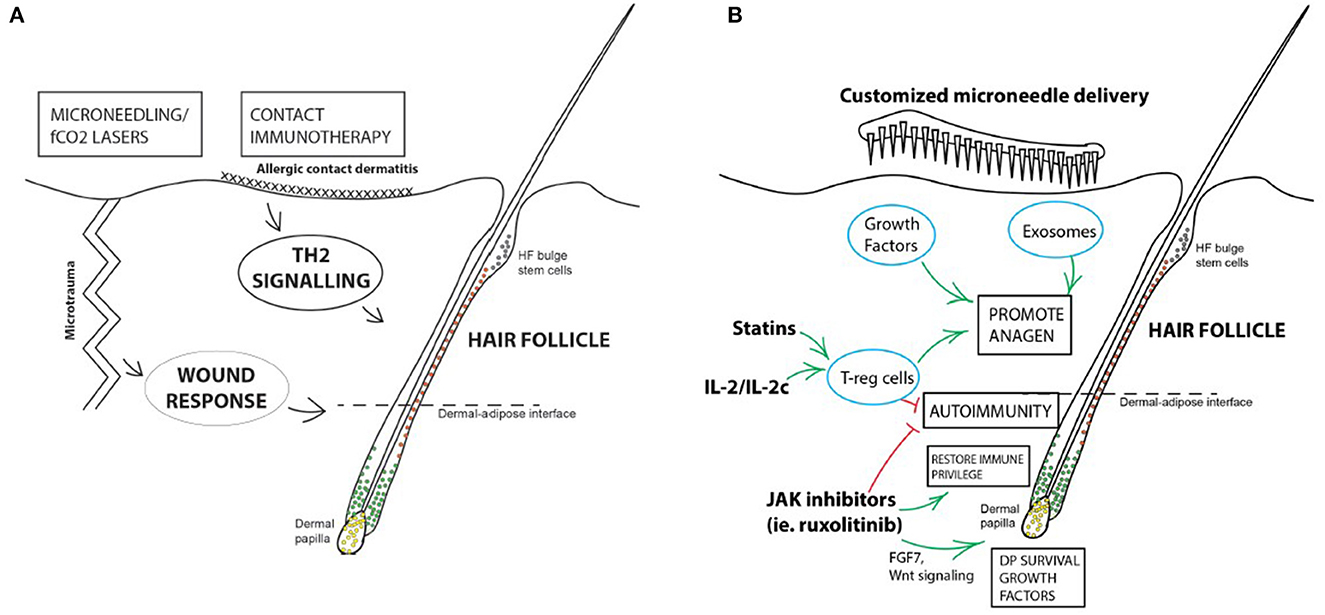
Figure 1. Summary of immunotherapy for hair loss disorders. (A) Current forms of immunotherapy are crude and non-targeted. Microtrauma produced by microneedling or PRP treatments are effective in promoting HF regeneration in AGA, and topical immunotherapy causing allergic contact dermatitis is an established treatment for AA. (B) Potential avenues for developing targeted immunotherapy for hair loss disorders. Some immunosuppressive treatments may have mechanisms of action independent of their suppressive effects, or direct stimulatory roles on the HF itself, like JAK inhibitors. JAK inhibition may have immunotherapeutic roles on the HF via upregulating the Wnt- β-catenin signaling pathway in DPCs, increasing FGF7 release which supports DP survival, and downregulation of MHC Class I expression in the HF, partially restoring IP. Stimulation of T-regs in the vicinity of the HF has both an immunomodulatory, as well as a direct pro-anagen effect on the HF. Targeted factors and signaling molecules can be packaged and delivered with bespoke microneedle patches or systems to mimic the effects of microtrauma to stimulate HF regrowth and regeneration.
Author contributions
ET: Writing—original draft, Writing—review and editing. EW: Conceptualization, Supervision, Writing—original draft, Writing—review and editing.
Funding
The author(s) declare that no financial support was received for the research, authorship, and/or publication of this article.
Conflict of interest
The authors declare that the research was conducted in the absence of any commercial or financial relationships that could be construed as a potential conflict of interest.
Publisher's note
All claims expressed in this article are solely those of the authors and do not necessarily represent those of their affiliated organizations, or those of the publisher, the editors and the reviewers. Any product that may be evaluated in this article, or claim that may be made by its manufacturer, is not guaranteed or endorsed by the publisher.
References
1. Wang EC, Dai Z, Ferrante AW, Drake CG, Christiano AM. A subset of TREM2+ dermal macrophages secretes oncostatin M to maintain hair follicle stem cell quiescence and inhibit hair growth. Cell Stem Cell. (2019) 24:654–69. doi: 10.1016/j.stem.2019.01.011
2. Wang EC, Higgins CA. Immune cell regulation of the hair cycle. Exp Dermatol. (2020) 29:322–33. doi: 10.1111/exd.14070
3. Eichmüller S, Van Der Veen C, Moll I, Hermes B, Hofmann U, Müller-Röver S, et al. Clusters of perifollicular macrophages in normal murine skin: physiological degeneration of selected hair follicles by programmed organ deletion. J Histochem Cytochem. (1998) 46:361–70. doi: 10.1177/002215549804600310
4. Botchkarev VA, Paus R, Czarnetzki BM, Kupriyanov VS, Gordon DS, Johansson O. Hair cycle-dependent changes in mast cell histochemistry in murine skin. Arch Dermatol Res. (1995) 287:683–6. doi: 10.1007/BF00371743
5. Paus R, Van Der Veen C, Eichmüller S, Kopp T, Hagen E, Müller-Röver S, et al. Generation and cyclic remodeling of the hair follicle immune system in mice. J Invest Dermatol. (1998) 111:7–18. doi: 10.1046/j.1523-1747.1998.00243.x
6. Billingham RE. Transplantation immunity evoked by skin homografts and expressed in intact skin. Adv Biol Skin. (1971) 11:183–98.
7. Park JM, Jun MS, Kim JA, Mali NM, Hsi TC, Cho A, et al. Restoration of immune privilege in human dermal papillae controlling epithelial-mesenchymal interactions in hair formation. Tissue Eng Reg Med. (2022) 12:1–12. doi: 10.1007/s13770-021-00392-7
8. Sawaya ME, Bakshandeh H, Hordinsky MK, Penneys NS. CD44 expression in alopecia areata and androgenetic alopecia. J Cutan Pathol. (1994) 21:229–32. doi: 10.1111/j.1600-0560.1994.tb00265.x
9. Michel L, Reygagne P, Benech P, Jean-Louis F, Scalvino S, Ly Ka So S, et al. Study of gene expression alteration in male androgenetic alopecia: evidence of predominant molecular signalling pathways. Br J Dermatol. (2017) 177:1322–36. doi: 10.1111/bjd.15577
10. Ali N, Zirak B, Rodriguez RS, Pauli ML, Truong HA, Lai K, et al. Regulatory T cells in skin facilitate epithelial stem cell differentiation. Cell. (2017) 169:1119–29. doi: 10.1016/j.cell.2017.05.002
11. Hardman JA, Muneeb F, Pople J, Bhogal R, Shahmalak A, Paus R. Human perifollicular macrophages undergo apoptosis, express Wnt ligands, and switch their polarization during catagen. J Invest Dermatol. (2019) 139:2543–6. doi: 10.1016/j.jid.2019.04.026
12. Ito M, Yang Z, Andl T, Cui C, Kim N, Millar SE, et al. Wnt-dependent de novo hair follicle regeneration in adult mouse skin after wounding. Nature. (2007) 447:316–20. doi: 10.1038/nature05766
13. Jiang S, Zhao L, Teklemariam T, Hantash BM. Small cutaneous wounds induce telogen to anagen transition of murine hair follicle stem cells. J Dermatol Sci. (2010) 60:143–50. doi: 10.1016/j.jdermsci.2010.10.008
14. Gay D, Kwon O, Zhang Z, Spata M, Plikus MV, Holler PD, et al. Fgf9 from dermal γδ T cells induces hair follicle neogenesis after wounding. Nat Med. (2013) 19:916–23. doi: 10.1038/nm.3181
15. Kasuya A, Ito T, Tokura Y. M2 macrophages promote wound-induced hair neogenesis. J Dermatol Sci. (2018) 91:250–5. doi: 10.1016/j.jdermsci.2018.05.004
16. Ma HJ, Yang Y, Ma HY, Jia CY, Li TH. Acquired localized hypertrichosis induced by internal fixation and plaster cast application. Ann Dermatol. (2013) 25:365–7. doi: 10.5021/ad.2013.25.3.365
17. Leung AK, Kiefer GN. Localized acquired hypertrichosis associated with fracture and cast application. J Natl Med Assoc. (1989) 81:65.
18. Shafir R, Tsur H. Local hirsutism at the periphery of burned skin. Br J Plast Surg. (1979) 32:93. doi: 10.1016/0007-1226(79)90004-3
19. Torchia D, Giorgini S, Gola M, Francalanci S. Allergic contact dermatitis from 2-ethylhexyl acrylate contained in a wig-fixing adhesive tape and its' incidental'therapeutic effect on alopecia areata. Contact Dermatitis. (2008) 58:170–1. doi: 10.1111/j.1600-0536.2007.01212.x
20. Happle R. Antigenic competition as a therapeutic concept for alopecia areata. Arch Dermatol Res. (1980) 267:109–14. doi: 10.1007/BF00416931
21. Gupta A, Bamimore M. Platelet-rich plasma monotherapies for androgenetic alopecia: a network meta-analysis and meta-regression study. J Drug Dermatol. (2022) 21:943–52. doi: 10.36849/JDD.6948
22. Zhang X, Ji Y, Zhou M, Zhou X, Xie Y, Zeng X, et al. Platelet-rich plasma for androgenetic alopecia: a systematic review and meta-analysis of randomized controlled trials. J Cutan Med Surg. (2023) 2023:12034754231191461. doi: 10.1177/12034754231191461
23. Chuah SY, Tan CH, Wang ECE, Tan KT, Chan RKW, Zhao X, et al. Efficacy of platelet-rich plasma in Asians with androgenetic alopecia: a randomized controlled trial. Indian J Dermatol Venereol Leprol. (2023) 89:135–8. doi: 10.25259/IJDVL_512_2021
24. English RS, Ruiz S, Do Amaral P. Microneedling and its use in hair loss disorders: a systematic review. Dermatol Ther. (2022) 12:1–20. doi: 10.1007/s13555-021-00653-2
25. Strazzulla LC, Avila L, Sicco KL, Shapiro J. An overview of the biology of platelet-rich plasma and microneedling as potential treatments for alopecia areata. J Inves Dermatol. (2017) 19:S21–4. doi: 10.1016/j.jisp.2017.10.002
26. McCarthy M, Day D, Talaber I. Hair regrowth and maintenance in alopecia universalis patient treated with nonablative Er: YAG laser. Clin Case Rep. (2021) 9:e04948. doi: 10.1002/ccr3.4948
27. Su YP, Wu XJ. Ablative 2940 nm Er: YAG fractional laser for male androgenetic alopecia. Dermatol Ther. (2022) 35:e15801. doi: 10.1111/dth.15801
28. Shokeir HA, Yousry A, Ibrahim SMA. Comparative study between topical steroid alone versus combined fractional Erbium: YAG laser with topical steroid in treatment of alopecia areata. Arch Dermatol Res. (2023) 315:241–7. doi: 10.1007/s00403-022-02417-0
29. Kwon O, Senna MM, Sinclair R, Ito T, Dutronc Y, Lin CY, et al. Efficacy and safety of baricitinib in patients with severe alopecia areata over 52 weeks of continuous therapy in two phase III trials (BRAVE-AA1 and BRAVE-AA2). Am J Clin Dermatol. (2023) 24:443–51. doi: 10.1007/s40257-023-00764-w
30. Wang Y, Liu T, Li S, Tang S, Lin P, Ding Y, et al. Efficacy and safety of baricitinib in patients with refractory alopecia areata. Dermatol Ther. (2022) 35:e15845. doi: 10.1111/dth.15845
31. Asfour L, Bokhari L, Bhoyrul B, Eisman S, Moussa A, Rees H. Treatment of moderate-to-severe alopecia areata in pre-adolescent children with baricitinib. Br J Dermatol. (2023) 12:ljad118. doi: 10.1093/bjd/ljad118
32. Zhou C, Yang X, Yang B, Yan G, Dong X, Ding Y, et al. A randomized, double-blind, placebo-controlled phase II study to evaluate the efficacy and safety of ivarmacitinib (SHR0302) in adult patients with moderate to severe alopecia areata. J Am Acad Dermatol. (2023) 1. doi: 10.1016/j.jaad.2023.02.063
33. Harel S, Higgins CA, Cerise JE, Dai Z, Chen JC, Clynes R, et al. Pharmacologic inhibition of JAK-STAT signaling promotes hair growth. Sci Adv. (2015) 1:e1500973. doi: 10.1126/sciadv.1500973
34. Wang E, Harel S, Christiano AM. JAK-STAT signaling jump starts the hair cycle. J Invest Dermatol. (2016) 136:2131–2. doi: 10.1016/j.jid.2016.08.029
35. Hassan AS, Al-Dhoun MQ, Shaker OG, AlOrbani AM. Expression of signal transducer and activator of transcription-3 in androgenetic alopecia: a case-control study. Skin Pharmacol Physiol. (2022) 35:278–81. doi: 10.1159/000525532
36. Kim JE, Lee YJ, Park HR, Lee DG, Jeong KH, Kang H. The effect of JAK inhibitor on the survival, anagen re-entry, and hair follicle immune privilege restoration in human dermal papilla cells. Int J Mol Sci. (2020) 21:5137. doi: 10.3390/ijms21145137
37. Kashetsky N, Turchin I. Utilization of topical ruxolitinib in dermatology: a review. Skin Therapy Lett. (2023) 28:8–13.
38. Yan D, Fan H, Chen M, Xia L, Wang S, Dong W, et al. The efficacy and safety of JAK inhibitors for alopecia areata: a systematic review and meta-analysis of prospective studies. Front Pharmacol. (2022) 13:950450. doi: 10.3389/fphar.2022.950450
39. Cervantes J, Jimenez JJ, DelCanto GM, Tosti A. Treatment of alopecia areata with simvastatin/ezetimibe. Jo Invest Dermatol Symp Proc. (2018) 19:S25–31. doi: 10.1016/j.jisp.2017.10.013
40. Kim YC, Kim KK, Shevach EM. Simvastatin induces Foxp3+ T regulatory cells by modulation of transforming growth factor-β signal transduction. Immunology. (2010) 130:484–93. doi: 10.1111/j.1365-2567.2010.03269.x
41. Youssef S, Stüve O, Patarroyo JC, Ruiz PJ, Radosevich JL, Hur EM, et al. The HMG-CoA reductase inhibitor, atorvastatin, promotes a Th2 bias and reverses paralysis in central nervous system autoimmune disease. Nature. (2002) 420:78–84. doi: 10.1038/nature01158
42. Pruefer D, Scalia R, Lefer AM. Simvastatin inhibits leukocyte–endothelial cell interactions and protects against inflammatory processes in normocholesterolemic rats. Arterioscler Thromb Vasc Biol. (1999) 19:2894–900. doi: 10.1161/01.ATV.19.12.2894
43. Palmer MA, Blakeborough L, Harries M, Haslam IS. Cholesterol homeostasis: Links to hair follicle biology and hair disorders. Exp Dermatol. (2020) 29:299–311. doi: 10.1111/exd.13993
44. Guasti L, Marino F, Cosentino M, Maio RC, Rasini E, Ferrari M, et al. Prolonged statin-associated reduction in neutrophil reactive oxygen species and angiotensin II type 1 receptor expression: 1-year follow-up. Eur Heart J. (2008) 29:1118–26. doi: 10.1093/eurheartj/ehn138
45. Loi C, Starace M, Piraccini BM. Alopecia areata (AA) and treatment with simvastatin/ezetimibe: experience of 20 patients. J Am Acad Dermatol. (2016) 74:e99–100. doi: 10.1016/j.jaad.2015.09.071
46. Guttman-Yassky E, Renert-Yuval Y, Bares J, Chima M, Hawkes JE, Gilleaudeau P, et al. Phase 2a randomized clinical trial of dupilumab (anti-IL-4Rα) for alopecia areata patients. Allergy. (2022) 77:897–906. doi: 10.1111/all.15071
47. Pagnanelli G, Cavani A, Canzona F, Mazzanti C. Mild therapeutic response of alopecia areata during treatment of psoriasis with secukinumab. Eur J Dermatol. (2020) 30:602–3. doi: 10.1684/ejd.2020.3866
48. Gallo R, Trave I, Parodi A. Massive acute alopecia of the scalp in a patient treated with dupilumab. Acta Derm Venereol. (2020) 100:1–2. doi: 10.2340/00015555-3549
49. Toussirot É, Aubin F. Paradoxical reactions under TNF-α blocking agents and other biological agents given for chronic immune-mediated diseases: an analytical and comprehensive overview. RMD open. (2016) 2:e000239. doi: 10.1136/rmdopen-2015-000239
50. Kim KH, Sim WY, Lew BL. Nivolumab-induced alopecia areata: a case report and literature review. Ann Dermatol. (2021) 33:284. doi: 10.5021/ad.2021.33.3.284
51. Dai Z, Sezin T, Chang Y, Lee EY, Wang EHC, Christiano AM. Induction of T cell exhaustion by JAK1/3 inhibition in the treatment of alopecia areata. Front Immunol. (2022) 13:955038. doi: 10.3389/fimmu.2022.955038
52. Castela E, Le Duff F, Butori C, Ticchioni M, Hofman P, Bahadoran P, et al. Effects of low-dose recombinant interleukin 2 to promote T-regulatory cells in alopecia areata. JAMA Dermatol. (2014) 150:748–51. doi: 10.1001/jamadermatol.2014.504
53. Le Duff F, Bouaziz JD, Fontas E, Ticchioni M, Viguier M, Dereure O, et al. Low-dose IL-2 for treating moderate to severe alopecia areata: a 52-week multicenter prospective placebo-controlled study assessing its impact on T regulatory cell and NK cell populations. J Invest Dermatol. (2021) 141:933–6. doi: 10.1016/j.jid.2020.08.015
54. Lee E, Kim M, Lee YJ. Selective expansion of tregs using the IL-2 cytokine antibody complex does not reverse established alopecia areata in C3H/HeJ mice. Front Immunol. (2022) 13:874778. doi: 10.3389/fimmu.2022.874778
55. Rückert R, Hofmann U, van der Veen C, Paus R, Bulfone-Paus S. MHC class I expression in murine skin: developmentally controlled and strikingly restricted intraepithelial expression during hair follicle morphogenesis and cycling, and response to cytokine treatment in vivo. J Invest Dermatol. (1998) 111:25–30. doi: 10.1046/j.1523-1747.1998.00228.x
56. Ito T, Ito N, Bettermann A, Tokura Y, Takigawa M, Paus R. Collapse and restoration of MHC class-I-dependent immune privilege: exploiting the human hair follicle as a model. Am J Pathol. (2004) 164:623–34. doi: 10.1016/S0002-9440(10)63151-3
57. Cuellar-Barboza A, Cardenas-de la Garza JA, Cruz-Gómez LG, Barboza-Quintana O, Flores-Gutiérrez JP, Gómez-Flores M. Local secretion of stress hormones increases in alopecia areata lesions after treatment with UVA-1 phototherapy. Exp Dermatol. (2020) 29:259–64. doi: 10.1111/exd.14077
58. Rallis E, Korfitis C, Gregoriou S, Rigopoulos D. Assigning new roles to topical tacrolimus. Expert Opin Investig Drugs. (2007) 16:1267–76. doi: 10.1517/13543784.16.8.1267
59. Kim YS, Jeong KH, Kim JE, Woo YJ, Kim BJ, Kang H. Repeated microneedle stimulation induces enhanced hair growth in a murine model. Ann Dermatol. (2016) 28:586–92. doi: 10.5021/ad.2016.28.5.586
60. Sun Y, Yang L, Du L, Zhou Y, Xu K, Chen J, et al. Duo-role Platelet-rich Plasma: temperature-induced fibrin gel and growth factors' reservoir for microneedles to promote hair regrowth. J Adv Res. (2023). doi: 10.1016/j.jare.2023.02.014
61. Wu J, Yang Q, Wu S, Yuan R, Zhao X, Li Y, et al. Adipose-derived stem cell exosomes promoted hair regeneration. Tissue Eng Regen Med. (2021) 18:685–91. doi: 10.1007/s13770-021-00347-y
Keywords: targeted, immunotherapy, hair, alopecia, regrowth, regeneration
Citation: Toh EQ and Wang ECE (2023) Targeted immunotherapy for hair regrowth and regeneration. Front. Med. 10:1285452. doi: 10.3389/fmed.2023.1285452
Received: 30 August 2023; Accepted: 26 September 2023;
Published: 10 October 2023.
Edited by:
Satoshi Nakamizo, Agency for Science, Technology and Research (A*STAR), SingaporeReviewed by:
Toshiaki Kogame, Kyoto University, JapanCopyright © 2023 Toh and Wang. This is an open-access article distributed under the terms of the Creative Commons Attribution License (CC BY). The use, distribution or reproduction in other forums is permitted, provided the original author(s) and the copyright owner(s) are credited and that the original publication in this journal is cited, in accordance with accepted academic practice. No use, distribution or reproduction is permitted which does not comply with these terms.
*Correspondence: Etienne C. E. Wang, ZXRpZW5uZUBuc2MuY29tLnNn