- 1General, Visceral and Transplant Surgery, Department of Surgery, Medical University of Graz, Graz, Austria
- 2Department of Anesthesiology, LMU Hospital, Munich, Germany
- 3CytoSorbents Europe GmbH, Berlin, Germany
Introduction: Hemoadsorption shows promising signals in organ preservation and post lung transplantation. However, its potential impact on the pharmacokinetics of immunosuppressant drugs (ID) is still unknown.
Methods: In this interventional study, CytoSorb® hemoperfusion was tested in healthy sheep (n = 5) against a sham extracorporeal circuit (n = 3). Seven different ID (tacrolimus (TAC), cyclosporin A (CYA), mycophenolate mofetil (MMF), everolimus (EVER), basiliximab (BAS), methylprednisolone (MP) and prednisolone (PRED)) were administered in clinically relevant doses and combinations. Their levels were measured repeatedly in blood samples from the extracorporeal circulation over 6 h following administration. Population pharmacokinetic modeling analysis (NONMEM® 7.5) was performed.
Results: Negligible clearance was observed for PRED and BAS. For all other substances, a saturable adsorption sub-model with linear decrease of the adsorption effect over the adsorbed amount best described the measured concentrations. The maximum absolute adsorbed amounts (95% CI) for TAC, CYA, MMF, EVER, and MP were 0.040 (0.028–0.053), 1.15 (0.39–1.91), 4.17 (2.00–6.35), 0.0163 (0.007–0.026), and 53.4 mg (20.9–85.9), respectively, indicating an adsorption of less than 5% of the daily administered dosages for all investigated substances.
Discussion: In this large animal model, CytoSorb® hemoperfusion appears to have a limited effect on the clearance of tested ID.
Introduction
Immunosuppressant drugs (ID) are used for various indications. Beyond their long-term use in an increasing number of patients for a range of conditions (1), ID are of vital importance for over 120,000 patients who annually undergo solid organ transplantations worldwide (2). However, scarcity of organs presents the most challenging barrier with long waiting lists and many patients failing to receive a transplant in time (3).
In this context, ex-vivo lung perfusion (EVLP) has recently gained a role in donor lung assessment, but also donor lung preservation, with the target to increase the donor pool and improve organ quality. However, cytokine accumulation during EVLP has been shown to correlate with worse outcomes after lung transplantation (4). Consequently, cytokine adsorption has recently been evaluated in this setting (5).
The CytoSorb® hemoadsorption device (CytoSorbents Corporation, NJ, USA), has been marketed and licensed for extracorporeal cytokine removal since 2011 within the European Union (6). It can be integrated into continuous renal replacement therapy (CRRT) or hemoperfusion circuits as well as into bypass circuits within extracorporeal membrane oxygenation (ECMO) machines or cardiopulmonary bypass (CPB). The cartridges contain highly biocompatible polystyrene divinylbenzene copolymer beads, coated with polyvinylpyrrolidone capable of removing small to midsize hydrophobic molecules up to a molecular weight of approximately 60 kDa (7) in a concentration-dependent manner. Various terms are used to describe the use of hemoadsorption techniques. In the following article, we will speak of “hemoperfusion” if the adsorption device is used in a stand-alone set-up and of “hemoadsorption” if use is in conjunction with other procedures like concomitant renal replacement therapy or when referring to literature.
Along with its main purpose of reducing excessive levels of cytokines, bilirubin (8), and myoglobin (9), hemoadsorption has also been shown to adsorb certain drugs from the blood (10). Although this can be of therapeutic advantage in the case of intoxication (11) or the prevention of bleeding by removal of antithrombotic agents (12), it may also imply a significant risk for the patient when unwanted removal of potentially life-saving medication occurs. Regarding anti-infectives, a standardized animal model in pigs showed an existing but overall limited effect on the pharmacokinetics of the majority of examined drugs (13).
Knowledge about the effect of hemoadsorption on the pharmacokinetics of immunosuppressants, however, remains limited. So far, post market surveillance has not suggested major adverse events with the use of CytoSorb® in patients on immunosuppression. To our knowledge, immunosuppressant drug levels have never been systematically investigated in blood taken directly from the inlet and outlet of the cartridge in-vivo. Published literature consists mainly of single cases with a focus on clinical outcome and if any, only on performing measurements of systemic drug levels (14–17). In summary, these reports do not represent sufficient safety information and lack consistency and reproducibility.
To address the urgent need for more reliable data we designed an experimental animal study to investigate the potential impact of hemoperfusion on the pharmacokinetics of immunosuppressants to generate standardized data contributing to the safety portfolio of the device.
Materials and methods
Animals
Fifteen 5-year-old ewes with a body weight of approximately 85 kg, purchased from a legal breeder (Christoph Priedl, Austria; LFBIS-/AMA-KI.Nr: 3335976), were housed at the Institute for Biomedical Research (Medical University of Graz, Graz, Austria) and were taken to the pasture daily. The study protocol was approved by the Austrian Committee for Animal Trials (Approval No: 2020-0.437.202). Animals had free access to fresh hay or grass and water as required. The present report was prepared following the ARRIVE 2.0 guidelines by the NC3Rs (National Centre for the Replacement Refinement and Reduction of Animals in Research).
Catheter implantation and extracorporeal circulation
The dialysis catheter was implanted according to standard procedures. After appropriate shaving and disinfection, the right jugular vein was punctured under general anesthesia and a 60 cm central venous catheter (Palindrom; Medtronic) was placed according to the Seldinger technique. Blood aspiration and flushing of the catheter with heparinized saline were performed to verify the correct position. The jugular catheter was then sutured to the skin, the site covered with a disinfectant swab, and the catheter secured to the neck with a bandage.
The central venous access was secured to the animal in such a way that the sheep could not remove it during normal movement. Daily catheter care consisted of inspection and cleaning of the entry site (bandage change if necessary), flushing of the catheter with heparinized saline solution, and blocking of the catheter lumen with Taurolock® Hep500. If the central venous access was no longer useable, a new catheter was placed in the jugular vein on the contralateral side in the same way as described above.
A schematic representation of the extracorporeal circuit (ECC) is shown in Figure 1. It was established in all animals with a dedicated device [BM11a, Baxter, Deerfield, IL, USA) and corresponding circuit (BM11-Lines-BA-HP tube system set, Baxter, Deerfield, IL, USA]. For animals allocated to the intervention group, a CytoSorb® cartridge was inserted in the ECC. For those allocated to the control group, no cartridge was integrated (sham hemoperfusion). The blood flow rate was kept at around 120 mL/min throughout the experiment. Immediately prior to the start of hemoperfusion or sham procedure animals were anticoagulated by intravenous application of 10,000 IU of Heparin. To maintain anti-coagulation 5,000 IU heparin was administered hourly to the inlet port.
Drug administration
All animals were granted a 14-day acclimatization period prior to experiment onset. After insertion of a central venous catheter as described below, the desired medication was applied, with subsequent random assignment to the intervention or control group. Since most immunosuppressants are absorbed enterally, the absorption behavior of Cyclosporin A (CYA) Tacrolimus (TAC), Mycophenolat Mofetil (MMF), and Everolimus (EVER), was tested in 4 pilot animals and data included for analysis. Since EVER is only available as an oral preparation and TAC could not be administered continuously via syringe pump over 24 h due to the trial set-up and limited staffing, all tested ID except MP and BAS were administered orally. Drugs were administered to the animals twice daily, as described elsewhere (18, 19). For TAC, EVER, and CYA, blood levels were determined three times weekly and if necessary, the doses were adapted accordingly. Upon achieving target blood levels as defined by current recommendations (20–23) an extracorporeal circuit with (intervention group) or without (control group) hemoadsorber was applied for a total duration of 6 h. On the morning of the first post-intervention day, blood levels of the respective immunosuppressive drugs were determined, and the doses were adjusted to keep up the required target levels. PRED was applied for all combination therapies in the morning directly prior to the onset of the extracorporeal intervention. See Table 1 for dosages and group distribution. For each immunosuppressant combination, five animals were allocated to the intervention group and compared to 3 control animals. BAS (20 mg) was applied in 3 animals directly before the onset of the extracorporeal intervention. For BAS no control animals were examined as due to its large molecular size of around 144 kDa no elimination by the device was to be suspected (24) (see Figure 2). For Methylprednisolone (MP) two sheep served as control and received 1 g MP without integration of the adsorber. In the study group, two scenarios simulating intraoperative application of the drug during running CPB (mimicking a heart transplant scenario) plus hemoadsorption were tested. We investigated either an increased starting dose (1.5 g MP) or the application of an additional dose of 1 g after 1.5 h (see Figure 3).
Blood samples and laboratory analysis
Baseline blood samples were collected before administration of the immunosuppressants. For combinations of TAC, CYA, MMF, EVER, and PRED blood samples were collected immediately prior to and at 30, 90, 250, and 330 min after the adsorber/sham procedure initiation (see Figure 2). For MP measurement samples were taken at 10, 30, 60, 90, 120, and 180 min after administration of the first MP dosage (see Figure 3). For animals allocated to the intervention group, two samples per time point, before (inlet) and after (outlet) the adsorber, were collected. For those allocated to the control group, we also collected samples from the extracorporeal circuit. Two ethylenediaminetetraacetic acid (EDTA) tubes and one serum tube were collected for inlet and outlet samples, respectively. Serum was separated from cells by centrifugation at 1,900× g for 10 min. The serum was split into two 2 mL Eppendorf tubes (Eppendorf AG, Hamburg, Germany) and frozen at −80°C until determination of PRED levels by a commercially available ELISA kit (MyBiosource, San Diego, USA).
In the case of TAC, EVER, and CYA whole blood levels and MMF EDTA plasma levels were measured at the laboratory of University Hospital Graz. TAC, CYA, and EVER levels were determined by LC–MS/MS (QTRAP 4500, Sciex) combined with a Shimadzu HPLC System whereas for detection of MMF levels, an Agilent HPLC System (G1310A) in combination with a multiple wavelength detector (G1365B) was used. For BAS the final absorbance was read at 490 and 630 nm (for correction) on an ELx808 ELISA plate reader (BioTek, VT, USA) at Centre Hospitalier Regional et Universitaire de Tours, France. Methylprednisolone was quantified using a sensitive ultra-performance liquid chromatography–tandem mass spectrometry (UPLC–MS/MS; ThermoFisher Scientific, San Jose, CA, USA) at University Hospital Reims, France.
Determination of clearance and elimination by the adsorber
In the first step, the course of the drug levels measured pre- and post-adsorber were compared to controls without adsorber (see Supplementary Figures 1–4). For BAS only pre- and post-adsorber levels were compared regarding each time point.
In the second step, clearances were calculated according to the following formula:
where CL = clearance (in mL/min), Ci = inlet drug concentration, Co = outlet drug concentration, and FL = plasma/serum flow through the adsorber (in mL/min), calculated as blood flow×(1-hematocrit). Hematocrit was calculated as 33% according to the standard value in sheep (25). The drug elimination rate (E) was calculated as E = Cl × Ci.
Pharmacokinetic parameters calculations
In the third step, non-linear mixed effect modeling was performed for all substances with measurable clearance in pre- versus post-adsorber samples using the software NONMEM 7.5 (NONMEM, Version 7.5; Icon Development Solutions, Ellicot City, MD, USA) to investigate adsorption characteristics and provide drug clearance over time. Graphical and statistical analysis was performed using R (Version 4.0.2).1
To determine the pharmacokinetics of the administered drugs without adsorption-related effects in sheep, hemoperfusion as a covariate was initially ignored. Based on an initial graphical evaluation of the concentration-time-profile, for orally administered drugs (CYA, MMF, TAC, EVER) only a one-compartment model was investigated, while for MP a one- and a two-compartment model was examined. For the orally administered drugs, volume of distribution (V) and clearance (CL) were estimated in relation to bioavailability (V/F and CL/F). To account for a possible delay to the onset of adsorption, a lag time was evaluated for orally administered drugs (ALAG). Models were selected based on the plausibility and identifiability of the parameters and statistical criteria (∆ Objective function value (OFV) > 3.84 for one additional parameter, Akaike information criterion). Structural parameters were assumed to be log-normally distributed and interindividual variability was evaluated for all parameters. In addition to numeric criteria, the prediction quality of the models for the measured concentrations was evaluated employing goodness of fit plots.
Second, the adsorption characteristics of hemoperfusion were investigated. Therefore, all parameters of step 1 except clearance were fixed and an additional clearance-pathway from the central compartment was added for the cartridge evaluating saturable and non-saturable CL by CytoSorb®, see Eqs 1 and 2. For the saturable CL via a linear decrease model (Eq. 2) the adsorption rate was linked to the maximum CL ( ), the drug amount already adsorbed at the cartridge ( ) and the maximum drug amount that can be adsorbed ( ):
Formula for constant adsorption (kconstant = elimination rate constant):
Formula for saturable adsorption:
Selection of an appropriate model was based on the precision and plausibility of parameter estimates and statistical criteria (see above).
Results
First, drug levels measured pre- and post-adsorber were compared to controls without adsorber (see Supplementary Figures 1–4). If direct adsorption (difference pre- and post-measurements) took place it occurred mostly within the first 1–2 h of device exposure. For BAS only pre- and post-adsorber measurements were compared and systemic blood levels showed only a slow decrease over time (see Supplementary Figure 5).
In the second step of analysis, cross-adsorber clearance was calculated from pre- versus post-adsorber measurements and flow rate. Clearance was shown to be negligible both for PRED and BAS (see Supplementary Figures 6–8).
Finally, for all substances that showed relevant clearance rates (TAC, MMF, EVER, CYA, and MP), pharmacokinetic modeling was performed. A one-compartment model with linear elimination and proportional residual variability adequately described the pharmacokinetics of orally administered drugs, while a two-compartment model with linear elimination and a combined proportional and additive residual variability model best-described data of MP. Interindividual Variabilities (IIVs) were included for CL or CL/F, respectively, for all drugs and additionally for V/F for TAC. A lag time improved the model for TAC (15.3 h), MMF (4.7 h), and EVER (4.1 h) and was consequently incorporated into the models. Eta shrinkage was low (<16%) indicating that all individuals contributed to the estimated IIVs. While most parameters could be estimated with high precision (Relative Standard Error (RSE) <30%), few parameters could be identified with only moderate precision (RSE 30–50%, e.g., V/F and first order oral absorption rate (KA) for EVER and CYA), presumably due to the limited number of individuals and the rather sparse sampling schedule during the initial period after oral administration. Residual variabilities were in an acceptable range (16.4–34.5% Coefficient of variation (CV)) for all drugs (see Supplementary Table 1).
In the second step of modeling, adsorption by the device was investigated. Evidence of adsorption was noted for all drugs except BAS and PRED, and was therefore included in the models. The (sub-)model representing a linear decrease (Eq. 2) of adsorption rate corresponding to saturable kinetics and characterizing Amax and CLmax was better (∆OFV: CYA, MMF, TAC, EVER, MP: −13.0, −19.8, −34.4, −16.6, −39.0, p < 0.001) than constant adsorption without saturation for all drugs. Estimated maximum adsorbed amounts and CLmax of CYA, MMF, TAC, EVER, and MP were 1.15 mg/2.80 L/h, 4.17 mg /3.71 L/h, 0.04 mg /4.02 L/h, 0.0163 mg /3.23 L/h and 53.4 mg /8.21 L/h (see Figures 4, 5). Of note, the estimated CLmax of MP was higher than the blood flow, but the confidence interval included the value of 7.2 L/h (95% CI: 6.16–10.25 L/h). For further information see Supplementary Table 2.
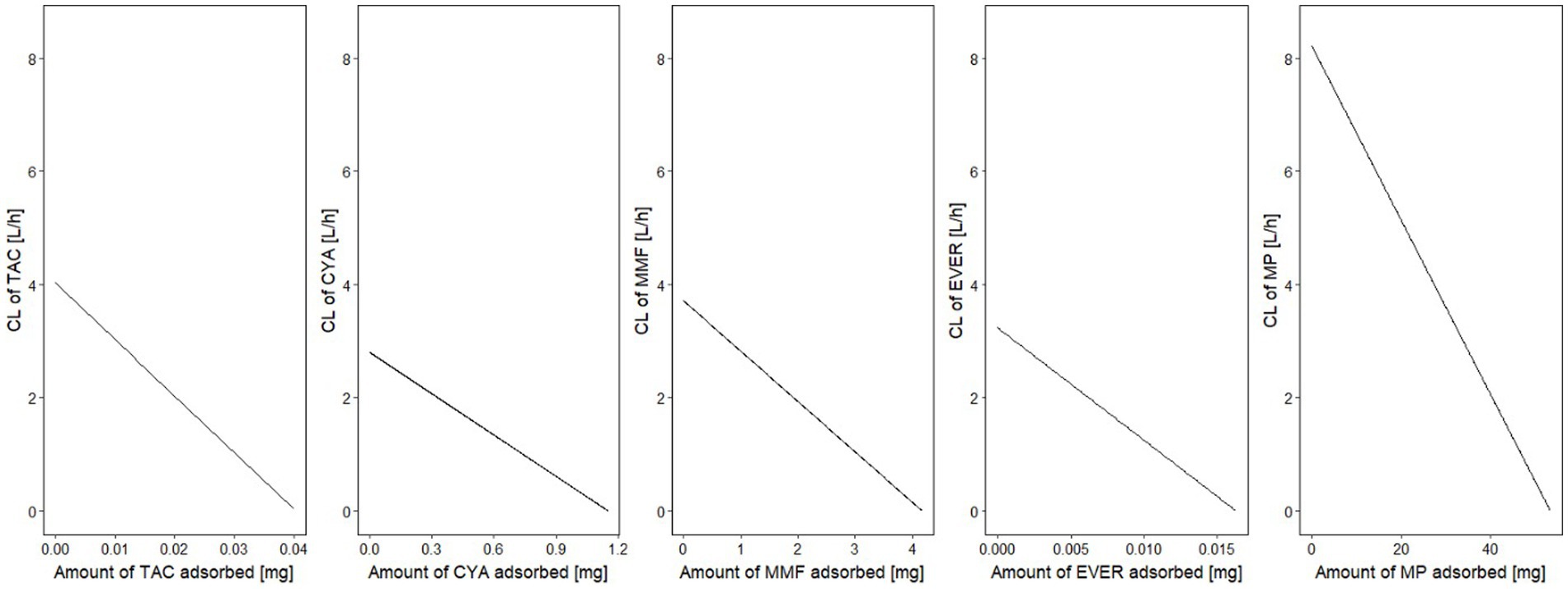
Figure 4. Clearance of the investigated drugs by the hemoadsorber. For all drugs, a linear decrease of clearance indicated saturation of the adsorption kinetics depending on the amount already adsorbed. CL, clearance; TAC, tacrolimus; MMF, mycophenolate mofetil; EVER, everolimus; MP, methylprednisolone.
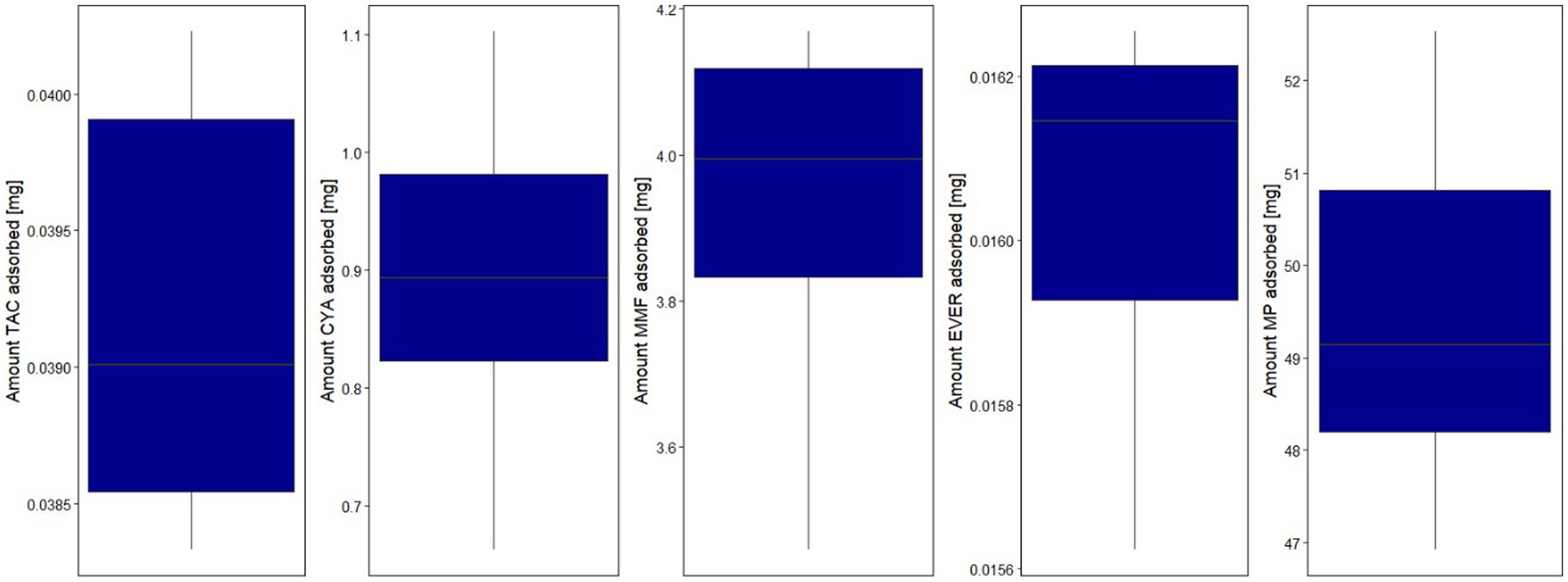
Figure 5. Boxplot of the total estimated adsorbed amount for the investigated drugs by hemoperfusion. Lower and upper box boundaries: 25th and 75th percentiles, respectively; line inside box: median; lower and upper error lines: 10th and 90th percentiles, respectively; TAC, tacrolimus; MMF, mycophenolate mofetil; EVER, everolimus; MP, methylprednisolone.
A separate residual variability for samples before (=plasma) and after the cartridge was estimated and residual variabilities post adsorber was <30% CV for all drugs (combined residual variability model for MP) indicating adequate representation of the data. Adequate representation of the data was confirmed by graphical evaluation methods stratified by pre- and post-adsorber (see Supplementary Figure 9). After adding the hemoperfusion elimination pathway systemic clearance decreased by less than 10% for all drugs indicating that hemoperfusion elimination accounted for only a small proportion of the total clearance. Overall, the maximum adsorbed amounts indicate an adsorption of less than 5% of the daily administered dosages for all tested substances.
Discussion
Key findings
Negligible clearance was observed in the measurements before and after the adsorber for PRED and BAS. For all other substances, a saturable adsorption sub-model with linear decrease of adsorption efficiency over the adsorbed amount best described the results. The maximum absolute adsorption amounts implied an adsorption rate of less than 5% of the daily administered doses for all tested substances.
Mechanistic considerations for drug removal
The possibility of inadvertent removal of medications in critically ill patients is an important issue that must be considered with the use of all extracorporeal therapies (10, 26). To evaluate the clinical significance of potential drug removal, several factors should be taken into account. These include the patient’s unique medical condition, any other extracorporeal therapies being used concurrently, the length of time the device will be in use, and whether the drug has just been administered or has reached steady-state levels in the patient’s system. Data on the influence of other extracorporeal therapies such as CPB or renal replacement therapy on ID are very scarce and recommendations on necessary dosing adaptations are lacking (27–29). Since hemoadsorption mainly targets hydrophobic substances which are usually protein-bound, other influencing factors such as the distribution volume must be considered when examining and interpreting the impact of such devices on drug levels (Figure 6). Therefore, drug removal data from in-vitro experiments are informative but not necessarily directly transferable to more complex in-vivo conditions (10).
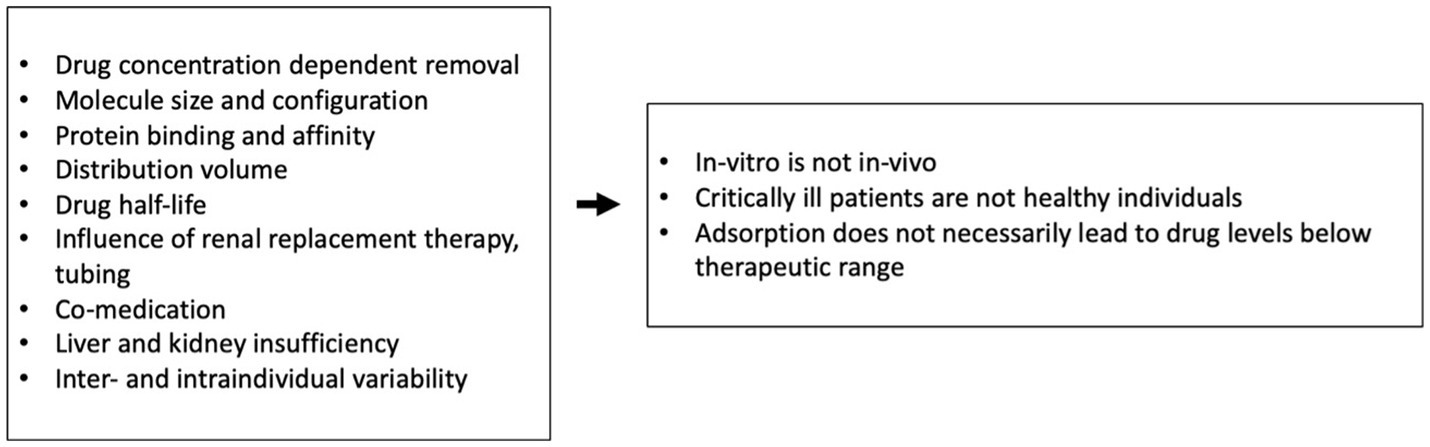
Figure 6. Influencing factors and their consequences on examination of hydrophobic protein-bound substances (8, 11).
Comparison with previous studies
Preexisting data on hemoadsorption in the context of immunosuppressive agents are scarce and partly contradictory. An early in-vitro study using uremic blood suggested moderate removal of TAC and CYA (30). Another more recent in-vitro study with whole blood as the carrier solution showed, in contrast, insignificant removal of tacrolimus (Hartmann J, 2017, Report P-2017-09 - In vitro Adsorption von Immunsuppressiva durch Cytosorb. University of Krems, Austria, data on file). The same investigation found moderate removal of CYA and high removal of mycophenolate and MP. However, as mentioned above such in-vitro have several limitations, and their results are not necessarily transferrable to in-vivo settings (Figure 6).
Regarding corticosteroids, ex-vivo elimination has been shown for methylprednisolone in a lung perfusion model (31) and for cortisol in brain-dead humans (32). In contrast, in an interim analysis in patients with myocardial revascularization divided into three different groups (one of them with a hemoadsorber integrated into the CPB) all hormone levels including cortisol remained comparable between groups suggesting no clinically relevant removal by the device (15).
In general, the use of hemoperfusion in the setting of transplantation is currently a topic of intense investigation which shows the importance of the systematic safety evaluation provided by this study but also the potential impact in clinical outcomes. A recent porcine acute respiratory distress syndrome (ARDS) lung transplantation model showed that the use of the extracorporeal blood purification device CytoSorb® during EVLP and for 12 h post-transplantation was able to restore lung function and reduce primary graft dysfunction (5). The authors suggest that this approach could increase not only the availability of donor organs but also increase tolerability by recipients. In another large animal model, the use of CytoSorb® during EVLP improved airway pressure and dynamic compliance, and reduced electrolyte imbalance and pulmonary edema (4). When lungs were treated with hemoadsorption during EVLP and subsequently transplanted, graft function was better when compared to untreated control lungs (31). In contrast, another recent porcine study investigating the use of hemoadsorption during reperfusion for 6 h in a lung transplant model failed to show similar effects (33).
Two single cases report on good organ function after 1 year post liver transplantation with the perioperative use of MP, BAS, and MMF (34), and 6 months after heart transplantation with the use of TAC, MMF, and PRED (16). Lastly, in an open randomized trial in 30 patients with CytoSorb® versus 30 without, safety interim analysis regarding rejection/mortality showed no harm or disadvantage for any group (Nemeth E, 2019, Reduction of Inflammation in Heart Transplantation, presented at 33rd European Association for Cardiothoracic Surgery – EACTS congress, Lisbon, Portugal). Of note, our data show that most of any adsorption occurs within the first 1–2 h of device exposure which is in line with previous studies (13).
Implications for clinicians and policymakers
There are various clinical settings in which the use of ID plays a major role, not only during and after transplantation of solid organs, stem cells, or bone marrow, but also in long term treatment of autoimmune diseases. According to our data, we can suggest some initial recommendations when using hemoperfusion in this patient cohort. However, it remains important to conduct human studies before implementing these recommendations into clinical practice.
In this large animal model, hemoperfusion had a very limited effect on the clearance of ID. These findings provide reassuring information for the safe use of the device in patients receiving ID. However, the need for regular blood level checks of immunosuppressants in clinical routine remains unchanged, and therapeutic drug monitoring during hemoadsorption therapy is recommended as with any other extracorporeal circuit. For BAS we were able to exclude any removal in this study which will reassure the usage of the device in patients on other antibody treatments with a similarly high molecular weight.
Strengths and limitations
This study provides the first standardized data on the possible influence of the hemoadsorption device and also examines an extracorporeal circuit on ID. To bridge the gap between benchtop results and the clinic, in-vivo preclinical trials are indispensable given the importance of immunosuppressants and the specific characteristics of the hemoadsorption device. Immunosuppressants have been tested in sheep before (35) and hemoperfusion has been used before in an animal septic shock sheep model (36). In our study sheep were chosen rather than pigs because they could be kept awake without the need for anesthesia for the whole duration of this study. In general, of course, any animal model comes with several limitations that must be considered when transferring the results of experiments to humans (37). Furthermore, the relatively small number of animals may compromise the firmness of conclusions drawn from the study. Also, we tested the substances in healthy sheep without allowing for influences on pharmacokinetics and dynamics resulting from pathophysiological changes seen in the critically ill. All tested ID except MP were administered orally which will presumably show different bioavailability compared to humans. To counteract these limitations, we measured blood drug levels directly within the extracorporeal circuit to assess direct removal from the blood. To gain detailed insights into elimination processes after oral administration, pharmacokinetic compartmental models were developed representing non-linear processes like adsorption more accurately compared to non-compartmental methods. Lastly, substances were administered in combinations which might have a reciprocal influence on adsorption. However, these groups were designed in line with clinical practice and therefore reflect the interactions expected in daily routine (38, 39).
Conclusion
Herein we report the first standardized examination of the possible influence on ID blood levels for a hemoadsorption device, as well as the examination of the impact of any extracorporeal circulation on ID. We were able to show a lack of removal for basiliximab and prednisolone and very limited influence on the blood levels of tacrolimus, cyclosporin A, mofetil mycophenolate, everolimus, and methylprednisolone. PK modeling revealed negligible clearance attributable to hemoadsorption for the latter substances. Despite the described limitations of the animal model, this represents important safety information for the usage of the CytoSorb® device in patients on immunosuppressants, both in the context of organ transplantation and long-term treatment for other reasons. Clinical decision-making should always be supported by therapeutic drug monitoring whenever available. Ideally, clinical studies in patients should be conducted to confirm our findings.
Data availability statement
The raw data supporting the conclusions of this article will be made available by the authors, without undue reservation.
Ethics statement
The animal study was approved by Austrian Committee for Animal Trials, BMBWF – V/3b, Minoritenplatz 5, 1010 Wien, Austria. The study was conducted in accordance with the local legislation and institutional requirements.
Author contributions
BL: Investigation, Writing – review & editing, Conceptualization, Data curation, Formal analysis, Methodology, Visualization, Writing – original draft. UL: Methodology, Visualization, Writing – original draft, Software, Writing – review & editing, Formal analysis. LR: Investigation, Writing – review & editing. JW: Investigation, Writing – review & editing. TK: Conceptualization, Project administration, Writing – original draft, Writing – review & editing. JS: Conceptualization, Methodology, Supervision, Writing – review & editing. RS: Investigation, Writing – review & editing, Supervision. PS: Conceptualization, Investigation, Methodology, Writing – review & editing, Supervision.
Funding
The research was supported by a financial grant by CytoSorbents Europe GmbH.
Acknowledgments
The authors want to thank Rudolf Brunnhofer for his on-site support and Harriet Adamson for language editing and literature research.
Conflict of interest
TK and JS are full-time employees of CytoSorbents Europe GmbH. UL has received fees for consulting activities from CytoSorbents Europe GmbH. BL and PS have received research grants from CytoSorbents Europe GmbH.
The remaining authors declare that the research was conducted in the absence of any commercial or financial relationships that could be construed as a potential conflict of interest.
Publisher’s note
All claims expressed in this article are solely those of the authors and do not necessarily represent those of their affiliated organizations, or those of the publisher, the editors and the reviewers. Any product that may be evaluated in this article, or claim that may be made by its manufacturer, is not guaranteed or endorsed by the publisher.
Supplementary material
The Supplementary material for this article can be found online at: https://www.frontiersin.org/articles/10.3389/fmed.2023.1258661/full#supplementary-material
Footnotes
References
1. Hsu, D, and Katelaris, CH. Long-term management of patients taking immunosuppressive drugs. Aust Prescr. (2009) 32:68–71. doi: 10.18773/austprescr.2009.035
2. Pilch, NA, Bowman, LJ, and Taber, DJ. Immunosuppression trends in solid organ transplantation: the future of individualization, monitoring, and management. Pharmacotherapy. (2021) 41:119–31. doi: 10.1002/phar.2481
3. Lewis, A, Koukoura, A, Tsianos, GI, Gargavanis, AA, Nielsen, AA, and Vassiliadis, E. Organ donation in the US and Europe: the supply vs demand imbalance. Transplant Rev (Orlando). (2021) 35:100585. doi: 10.1016/j.trre.2020.100585
4. Iskender, I, Cosgun, T, Arni, S, Trinkwitz, M, Fehlings, S, Yamada, Y, et al. Cytokine filtration modulates pulmonary metabolism and edema formation during ex vivo lung perfusion. J Heart Lung Transplant. (2017) 37:283–91. doi: 10.1016/j.healun.2017.05.021
5. Ghaidan, H, Stenlo, M, Niroomand, A, Mittendorfer, M, Hirdman, G, Gvazava, N, et al. Reduction of primary graft dysfunction using cytokine adsorption during organ preservation and after lung transplantation. Nat Commun. (2022) 13:4173. doi: 10.1038/s41467-022-31811-5
6. Poli, EC, Rimmele, T, and Schneider, AG. Hemoadsorption with CytoSorb((R)). Intensive Care Med. (2019) 45:236–9. doi: 10.1007/s00134-018-5464-6
7. Malard, B, Lambert, C, and Kellum, JA. In vitro comparison of the adsorption of inflammatory mediators by blood purification devices. Intensive Care Med Exp. (2018) 6:12. doi: 10.1186/s40635-018-0177-2
8. Scharf, C, Liebchen, U, Paal, M, Becker-Pennrich, A, Irlbeck, M, Zoller, M, et al. Successful elimination of bilirubin in critically ill patients with acute liver dysfunction using a cytokine adsorber and albumin dialysis: a pilot study. Sci Rep. (2021) 11:10190. doi: 10.1038/s41598-021-89712-4
9. Scharf, C, Liebchen, U, Paal, M, Irlbeck, M, Zoller, M, and Schroeder, I. Blood purification with a cytokine adsorber for the elimination of myoglobin in critically ill patients with severe rhabdomyolysis. Crit Care. (2021) 25:41. doi: 10.1186/s13054-021-03468-x
10. Scheier, J, Nelson, PJ, Schneider, A, Colombier, S, Kindgen-Milles, D, Deliargyris, EN, et al. Mechanistic considerations and pharmacokinetic implications on concomitant drug administration during CytoSorb therapy. Crit Care Explor. (2022) 4:e0688. doi: 10.1097/CCE.0000000000000688
11. Schroeder, I, Zoller, M, Angstwurm, M, Kur, F, and Frey, L. Venlafaxine intoxication with development of takotsubo cardiomyopathy: successful use of extracorporeal life support, intravenous lipid emulsion and CytoSorb. Int J Artif Organs. (2017) 40:358–60. doi: 10.5301/ijao.5000595
12. Hassan, K, Brüning, T, Caspary, M, Wohlmuth, P, Pioch, H, Schmoeckel, M, et al. Hemoadsorption of rivaroxaban and ticagrelor during acute type a aortic dissection operations. Ann Thorac Cardiovasc Surg. (2022) 28:186–92. doi: 10.5761/atcs.oa.21-00154
13. Schneider, AG, André, P, Scheier, J, Schmidt, M, Ziervogel, H, Buclin, T, et al. Pharmacokinetics of anti-infective agents during CytoSorb hemoadsorption. Sci Rep. (2021) 11:10493. doi: 10.1038/s41598-021-89965-z
14. Hawchar, F, Rao, C, Akil, A, Mehta, Y, Rugg, C, Scheier, J, et al. The potential role of extracorporeal cytokine removal in hemodynamic stabilization in hyperinflammatory shock. Biomedicine. (2021) 9:768. doi: 10.3390/biomedicines9070768
15. Deppe, AC, Weber, C, Choi, YH, and Wahlers, T. Einsatz eines Zytokinfilters in die Herz-Lungen-Maschine. Z Herz- Thorax- Gefäßchir. (2016) 30:254–9. doi: 10.1007/s00398-016-0075-4
16. Immohr, MB, Lichtenberg, A, Boeken, U, and Akhyari, P. Succesful treatment of a severe case of rhabdomyolysis following heart transplantation by hemoadsorption. J Card Surg. (2020) 35:940–1. doi: 10.1111/jocs.14481
17. Nemeth, E, Kovacs, E, Racz, K, Soltesz, A, Szigeti, S, Kiss, N, et al. Impact of intraoperative cytokine adsorption on outcome of patients undergoing orthotopic heart transplantation-an observational study. Clin Transpl. (2018) 32:e13211. doi: 10.1111/ctr.13211
18. Wei, L, Xue, T, Yang, H, Zhao, GY, Zhang, G, Lu, ZH, et al. Modified uterine allotransplantation and immunosuppression procedure in the sheep model. PLoS One. (2013) 8:e81300. doi: 10.1371/journal.pone.0081300
19. Gauthier, T, Bertin, F, Fourcade, L, Maubon, A, Saint Marcoux, F, Piver, P, et al. Uterine allotransplantation in ewes using an aortocava patch. Hum Reprod. (2011) 26:3028–36. doi: 10.1093/humrep/der288
20. Shihab, F, Qazi, Y, Mulgaonkar, S, McCague, K, Patel, D, Peddi, VR, et al. Association of clinical events with everolimus exposure in kidney transplant patients receiving low doses of tacrolimus. Am J Transplant. (2017) 17:2363–71. doi: 10.1111/ajt.14215
21. Vitko, S, Tedesco, H, Eris, J, Pascual, J, Whelchel, J, Magee, JC, et al. Everolimus with optimized cyclosporine dosing in renal transplant recipients: 6-month safety and efficacy results of two randomized studies. Am J Transplant. (2004) 4:626–35. doi: 10.1111/j.1600-6143.2004.00389.x
22. Franco, A, Más-Serrano, P, Balibrea, N, Rodriguez, D, Javaloyes, A, Díaz, M, et al. Envarsus, a novelty for transplant nephrologists: observational retrospective study. Nefrología (English Edition). (2019) 39:506–12. doi: 10.1016/j.nefroe.2018.11.020
23. Suwelack, B. Konsensus der Arbeitsgemeinschaft der Nierentransplantationszentren Nordrhein-Westfalens, Germany. Manual zur Vereinheitlichung der Evaluation vor Nierentransplantation und Nierenlebendspende, der Wartelistenführung vor Nierentransplantation und zur Nachsorge nach Nierentransplantation und Nierenlebendspende Consensus of the Working Group of Kidney Transplant Centres of North Rhine- Westphalia, Germany. Manual for standardization of evaluation before kidney transplantation and living kidney donation, waiting list management before kidney transplantation and follow-up after kidney transplantation and living kidney donation. (2018). Available at: https://www.d-t-g-online.de/images/Downloads/Manual_Nierentransplantation_10-2018.pdf (Accessed Jun 21, 2023).
24. Basiliximab. Drugs and lactation database (LactMed®). National Institute of Child Health and Human Development, Bethesda (MD). (2006). Available at: https://www.ncbi.nlm.nih.gov/books/NBK500584/ (Accessed June 21, 2023).
25. Veterinärmedizinische Faultät Labor des Großtierklinikums (Veterinary Faculty, Laboratory for Large Animal Hospital. Referenzwerte: Schaf (Reference values Sheep). Leipzig, Germany. Available at: https://www.vetmed.uni-leipzig.de/fileadmin/Fakult%C3%A4t_VMF/Klinik_Klauentiere/Dokumente/Labor/ReferenzWerte-Schaf.pdf
26. Monaghan, KN, and Acierno, MJ. Extracorporeal removal of drugs and toxins. Vet Clin North Am Small Anim Pract. (2011) 41:227–38. doi: 10.1016/j.cvsm.2010.09.005
27. Pea, F, Pavan, F, and Furlanut, M. Clinical relevance of pharmacokinetics and pharmacodynamics in cardiac critical care patients. Clin Pharmacokinet. (2008) 47:449–62. doi: 10.2165/00003088-200847070-00002
28. Faelens, R, Luyckx, N, Kuypers, D, Bouillon, T, and Annaert, P. Predicting model-informed precision dosing: a test-case in tacrolimus dose adaptation for kidney transplant recipients. CPT Pharmacometrics Syst Pharmacol. (2022) 11:348–61. doi: 10.1002/psp4.12758
29. Cheng, V, Abdul-Aziz, MH, Roberts, JA, and Shekar, K. Optimising drug dosing in patients receiving extracorporeal membrane oxygenation. J Thorac Dis. (2018) 10:S629–41. doi: 10.21037/jtd.2017.09.154
30. Reiter, K, Bordoni, V, Dall’Olio, G, Ricatti, MG, Soli, M, Ruperti, S, et al. In vitro removal of therapeutic drugs with a novel adsorbent system. Blood Purif. (2002) 20:380–8. doi: 10.1159/000063108
31. Iskender, I, Arni, S, Maeyashiki, T, Citak, N, Sauer, M, Rodriguez, JM, et al. Perfusate-adsorption during ex vivo lung perfusion improves early post-transplant lung function. J Thorac Cardiovasc Surg. (2021) 161:e109–21. doi: 10.1016/j.jtcvs.2019.12.128
32. Kellum, JA, Venkataraman, R, Powner, D, Elder, M, Hergenroeder, G, and Carter, M. Feasibility study of cytokine removal by hemoadsorption in brain-dead humans. Crit Care Med. (2008) 36:268–72. doi: 10.1097/01.CCM.0000291646.34815.BB
33. Frick, AE, Orlitová, M, Bleeser, T, Vanstapel, A, Claes, S, Schols, D, et al. Can we attenuate ischaemia-reperfusion injury of allografts in a porcine left lung transplant models by adsorption of cytokines? Eur J Cardiothorac Surg. (2022) 63:ezac483. doi: 10.1093/ejcts/ezac483
34. Tomescu, D. First use of CytoSorb during living related liver transplantation in a patient with acute iver failure due to Wilson's disease In: Proceedings of the 2018 joint international congress of ILTS. Lisbon, Portugal: ELITA and LICAGE (2018)
35. Diehl, R, Ferrara, F, Müller, C, Dreyer, AY, McLeod, DD, Fricke, S, et al. Immunosuppression for in vivo research: state-of-the-art protocols and experimental approaches. Cell Mol Immunol. (2017) 14:146–79. doi: 10.1038/cmi.2016.39
36. Hessler, M, Schmidt, C, Kaiser, LE, Zurek-Leffera, F, Kampmeier, TG, Ertmer, C, et al. Early cytokine adsorption in septic shock reduces volume responsiveness-guided fluid requirement after initial hemodynamic stabilization in sheep In: Proceedings of the ESICM Lives 2022 Paris, France Intensive Care Management (2022). 197–8. doi: 10.1186/s40635-022-00469-0
37. Bracken, MB. Why animal studies are often poor predictors of human reactions to exposure. J R Soc Med. (2009) 102:120–2. doi: 10.1258/jrsm.2008.08k033
38. Viklicky, O, Slatinska, J, Novotny, M, and Hruba, P. Developments in immunosuppression. Curr Opin Organ Transplant. (2021) 26:91–6. doi: 10.1097/MOT.0000000000000844
39. Fernandez-Castillo, JC, and Cypel, M. Immunosuppressive therapy in lung transplantation. Curr Pharm Des. (2020) 26:3385–8. doi: 10.2174/1381612826666200628023150
Glossary
Keywords: CytoSorb, hemoadsorption, hemoperfusion, immunosuppressants, immunosuppressant drugs, adsorption, blood purification
Citation: Leber B, Liebchen U, Rohrhofer L, Weber J, Klaus T, Scheier J, Sucher R and Stiegler P (2023) Pharmacokinetics of immunosuppressive agents during hemoperfusion in a sheep model. Front. Med. 10:1258661. doi: 10.3389/fmed.2023.1258661
Edited by:
Rami Namas, University of Pittsburgh, United StatesReviewed by:
Sridhar R. Allam, Texas Christian University, United StatesTobias Kammerer, Cologne University Hospital, Germany
Copyright © 2023 Leber, Liebchen, Rohrhofer, Weber, Klaus, Scheier, Sucher and Stiegler. This is an open-access article distributed under the terms of the Creative Commons Attribution License (CC BY). The use, distribution or reproduction in other forums is permitted, provided the original author(s) and the copyright owner(s) are credited and that the original publication in this journal is cited, in accordance with accepted academic practice. No use, distribution or reproduction is permitted which does not comply with these terms.
*Correspondence: Bettina Leber, bettina.leber@medunigraz.at