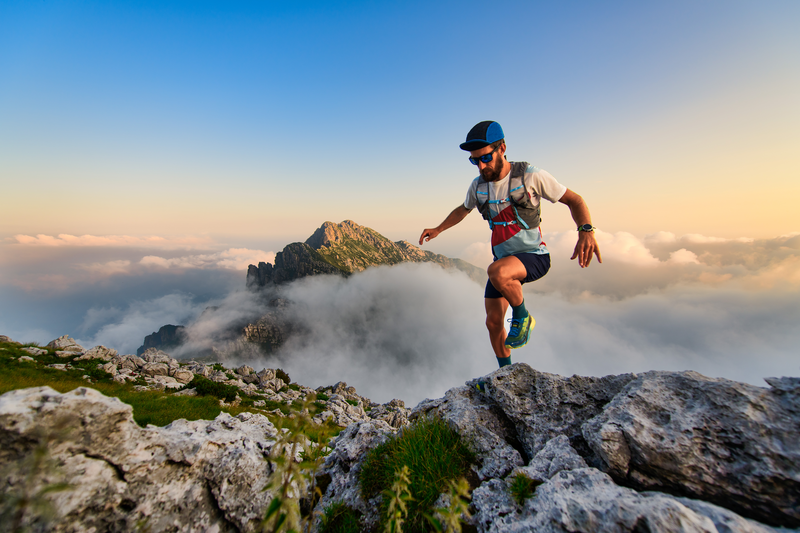
95% of researchers rate our articles as excellent or good
Learn more about the work of our research integrity team to safeguard the quality of each article we publish.
Find out more
SYSTEMATIC REVIEW article
Front. Med. , 04 September 2023
Sec. Infectious Diseases: Pathogenesis and Therapy
Volume 10 - 2023 | https://doi.org/10.3389/fmed.2023.1249030
This article is part of the Research Topic Community Series: Spotlight on the Relationship between Sepsis and Infection: from Mechanisms to Therapy - Volume II View all 8 articles
Introduction: Carbapenem-resistant Enterobacterales (CRE) and multidrug-resistant Pseudomonas aeruginosa (MDR-PA) infections are associated with a high risk of morbidity, mortality, and treatment costs. We aimed to evaluate in vitro, in vivo and clinical studies comparing the efficacy of ceftazidime-avibactam (CZA) combination regimens with CZA alone against CRE and/or MDR-PA isolates or infections.
Methods: We systematically reviewed the relevant literature in CINAHL/MEDLINE, Pubmed, Cochrane, Web of Science, Embase, and Scopus until December 1, 2022. Review articles, grey literature, abstracts, comments, editorials, non-peer reviewed articles, non-English articles, and in vitro synergy studies conducted on single isolates were excluded.
Results: 22 in vitro, 7 in vivo and 20 clinical studies were evaluated. In vitro studies showed reliable synergy between CZA and aztreonam against metallo-β-lactamase (MBL)-producing isolates. Some studies indicated good in vitro synergy between CZA and amikacin, meropenem, fosfomycin and polymyxins against CRE isolates. For MDR-PA isolates, there are comparatively fewer in vitro or in vivo studies. In observational clinical studies, mortality, clinical cure, adverse events, and development of CZA resistance after exposure were generally similar in monotherapy and combination therapy groups. However, antibiotic-related nephrotoxicity and infection relapses were higher in patients receiving CZA combination therapies.
Discussion: The benefit, if any, of CZA combination regimens in MDR-PA infections is elusive, as very few clinical studies have included these infections. There is no currently documented clinical benefit for the use of CZA combination regimens rather than CZA monotherapy. CZA combined with aztreonam for serious infections due to MBL producers should be evaluated by randomized controlled trials.
Systematic review registration: https://www.crd.york.ac.uk/prospero/display_record.php?RecordID=278552, CRD42021278552.
Antimicrobial resistance (AMR) is a global public health threat from which no country or region is spared (1–3). In a recent report, deaths attributable to bacterial AMR were estimated to be 1.27 million (95% UI 0.91–1.71) worldwide in 2019 (4). Each of the 6 bacteria mainly causing AMR-related mortality was responsible for more than 250,000 deaths: Escherichia coli, Staphylococcus aureus, Klebsiella pneumoniae, Streptococcus pneumoniae, Acinetobacter baumannii, and Pseudomonas aeruginosa by order of death count (4).
According to the Centre for Disease Control and Prevention (CDC) definition, Enterobacterales that are either resistant to any carbapenem or express a carbapenemase enzyme are defined as CRE (5). CRE are made up of a heterogeneous group of bacteria with various carbapenem resistance mechanisms, broadly classified as carbapenemase producers and non-carbapenemase producers. When treating infections caused by CRE, knowing whether the causative microorganism produces a carbapenemase enzyme and, if so, the type of carbapenemase expressed is very important to inform optimal treatment decisions. MDR-PA is defined as P. aeruginosa not susceptible to at least 1 of 3 antibiotic classes for which anti-pseudomonal activity is typically anticipated (6). MDR-PA generally arises as a consequence of a coaction of multiple resistance mechanisms (7, 8). Although carbapenemase expression is not a common resistance mechanism for P. aeruginosa, it can be detected frequently in some parts of the world (9, 10). Recently, a new phenomenon, difficult-to-treat resistant (DTR) P. aeruginosa was defined as P. aeruginosa isolates displaying non-susceptibility to all of the following antibiotics: piperacillin-tazobactam, aztreonam, cephalosporins, carbapenems, ciprofloxacin, and levofloxacin (11).
Ceftazidime-avibactam (CZA) is the first new generation β-lactam/β-lactamase inhibitor combination to come to market and consists of a third-generation cephalosporin and a new generation non-β-lactam/β-lactamase inhibitor (12). CZA has potent activity against KPC and OXA-48-like carbapenemases, extended-spectrum β-lactamases (ESBL) and AmpC β-lactamases, as well as against non-carbapenemase-producing CRE (13). CZA also has enhanced activity against MDR-PA strains with susceptibility rates ranging from 67% to 88% (13). Conversely, the combination of ceftazidime with avibactam does not confer antibacterial activity against carbapenem-resistant A. baumannii strains and metallo-β-lactamase-producing gram-negative bacteria (14). In observational studies, CZA-containing regimens have been shown to be associated with favorable clinical outcomes and a lower risk of nephrotoxicity compared to colistin-based regimens (15, 16). However, little is known on the benefits and harms of CZA combination therapies over CZA monotherapy.
The role of combination regimens in the treatment of infections with CRE and MDR-PA is a matter of long-standing debate (17, 18). The main expectation of clinicians from CZA combination regimens is to increase the clinical efficacy of CZA through synergistic interaction and to prevent the development of resistance to CZA (13). The latter is particularly critical, because several studies have demonstrated the emergence of resistance during or following therapy in 3.7%–8.1% of CZA receiving patients infected by CRE (19, 20). However, combination therapies may increase the likelihood of side effects and treatment costs.
In this scoping review, we aim to evaluate in vitro, in vivo and clinical studies comparing CZA monotherapy and CZA combination therapies. The insights generated by this scoping review can contribute to identifying the benefits and harms of CZA combination therapies over CZA monotherapy for infections caused by CRE and MDR-PA, and lead to hypotheses that can be tested in randomized clinical trials (RCTs).
This scoping review was performed in accordance with the Preferred Reporting Items for Systematic reviews and Meta-Analyses extension for Scoping Reviews (PRISMA-ScR) (21), Prospero ID: CRD42021278552.
We searched relevant literature in PubMed, MEDLINE, CINAHL, Cochrane, Embase, Scopus, and Web of Science in December 2022. The search terms, MeSH terms and publication filters used are presented in the Supplementary material. The references were imported into an EndNote (Clarivate Analytics, Philadelphia, Pennsylvania, United States) database, and duplicated and non-English studies were not included. References within the recruited articles were searched manually to capture additional studies.
The titles and abstracts of all records were screened by 2 reviewers (AA and YE) independently to evaluate full-text eligibility. Any disagreements were resolved by discussion. Articles were included after full-text review if they met the following inclusion criteria: (1) original research, (2) in vitro, in vivo or clinical studies comparing CZA alone vs. CZA-containing combinations against CRE and/or MDR-PA isolates (regardless of their resistance mechanisms) or infections, and (3) published up to December 1, 2022. Otherwise, articles were excluded for the following reasons: (1) review articles; (2) the full text was not available; (3) grey literature; (4) abstracts, comments, letters, and editorials; (5) non-peer reviewed articles; (6) studies published in a language other than English; (7) in vitro synergy studies conducted on only one isolate.
Data were extracted into predesigned spreadsheets in duplicate by two independent reviewers. In in vitro studies, data pertaining with authors, publication year, country, AMR phenotype, predominant AMR mechanisms, CZA susceptibility rate, type of bacterial strain, number of isolates tested, method(s) used for antimicrobial susceptibility tests and criteria used for assessment of their results, type of in vitro synergy test applied, companion antibiotic(s) used, definition of synergism, additive effect, and antagonism, concentrations of CZA and companion antibiotics used in experiments, and number of repeats in experiments were extracted. In in vivo studies, authors, publication year, country, type of bacterial strain, AMR phenotype, main molecular determinants of AMR, type of in vivo infection model, number of isolates tested, method(s) used for antimicrobial susceptibility tests and criteria used for interpretation of their results, doses of CZA and companion antibiotics adjusted in experiments, and killing activity of tested antibiotics were extracted. In clinical studies, we extracted authors, publication year, country, the study design, statistical method, and relevant data, including the number of patients in comparison groups, type of companion antibiotics, in vitro activity of concomitant antibiotics, median time from onset of infection to active treatment, demographic information of patients, the infection type, number of bacteremic patients, carbapenem non-susceptibility rate of the causative pathogens, main AMR mechanism, presence of septic shock, and outcomes parameters of individual studies (i.e., all-cause mortality, clinical cure, microbiological cure, adverse events, acute kidney injury, emergence of CZA resistance and infection relapse). Because of the substantial heterogeneity of study designs, treatment regimens, definitions of study outcomes and combination regimens (i.e., in vitro inactive antibiotics being considered as co-antibiotics in some studies), we did not perform quantitative synthesis of the data using a meta-analysis and a narrative synthesis was undertaken to evaluate the efficacy and safety of CZA monotherapy and CZA combination therapies.
In our database search, 6,958 studies were identified and 5,068 were excluded based on duplications. After reviewing titles and abstracts, 1,440 were not included and 401 articles were excluded for the reasons depicted in Figure 1. Overall, 49 studies comprising 22 in vitro, 7 in vivo and 20 clinical studies were included in the final data synthesis.
Some antibiotics can be combined with CZA to expand or enhance its antibacterial capacity. For example, avibactam protects aztreonam from the hydrolytic activity of ESBLs and AmpC β-lactamases, which are frequently co-produced by metallo-β-lactamase-producing gram-negative bacteria (22). As aztreonam is not hydrolyzed by metallo-β-lactamases, it can show significant antibacterial activity in the presence of CZA against metallo-β-lactamase-producing gram-negative bacteria (22). Furthermore, CZA plus aztreonam may substantially influence the “divisome” of these bacteria by acting on different types of penicillin-binding proteins (22). Another potential way of enhancing CZA’s in vitro activity is to take advantage of enhanced susceptibility to antibiotics brought about by mutations that compromise CZA’s activity. A unique mutation (D179Y) within or proximal to KPC-3-Ω-loop is responsible for CZA resistance in KPC-producing CRE isolates (19). Intriguingly, the same mutation may restore meropenem susceptibility in some strains. However, the susceptibility of these mutant strains to meropenem is generally unsustainable and, as a result, the clinical relevance of this finding is unclear (23). Nevertheless, the combination of CZA plus meropenem may open a new avenue for the treatment of KPC-producing CRE infections. Other combination regimens containing antibiotics with mechanisms of action different from CZA may increase cumulative antibacterial activity through synergistic interactions by less clearly explained mechanisms.
Among in vitro studies, 22 were included in final data synthesis (Figure 1). The main features of all included studies are shown in Table 1. Among 22 studies, 5 reported data on Enterobacterales, 10 K. pneumoniae, 3 P. aeruginosa, 2 both K. pneumoniae and P. aeruginosa, and 1 both Enterobacterales and P. aeruginosa and resistance mechanisms of tested pathogens were quite heterogeneous (Table 2). In vitro synergy was examined by time-kill (n = 13), gradient diffusion (n = 3), E-test MIC:MIC (n = 4), checkerboard (n = 4), and pharmacokinetic/pharmacodynamic (n = 2) studies. In four of these studies, two different methods were used to analyze the in vitro interactions of antibiotic combinations (29, 31, 32, 35). In studies utilizing E-test MIC:MIC, gradient diffusion and checkerboard, the fractional inhibitory concentration index (FICI) was calculated to define synergy. Synergy was defined as FICI ≤ 0.50, additivity >0.50 to ≤1.00, indifference >1.00 to ≤4.00, and antagonism >4.00. In time-kill and pharmacokinetic/pharmacodynamic studies, reduction in bacterial load ≥2log10 colony forming unit (CFU) as compared to most active single antibiotic was defined as synergy, while ≥2-log10 increase in bacterial burden was defined as antagonism. In approximately half of the studies (n = 10), the number of repetitions of the experiments was not provided (26, 29–32, 36, 40, 43–45). The remainder repeated the experiments either 2 (24, 25, 33, 35, 37–39, 41) or ≥ 3 (27, 28, 34, 42) times. All in vitro synergy studies were between CZA and commercially available antibiotics, except for one study in which Idowu et al. analyzed the synergy between CZA and tobramycin-cyclam conjugate, which abrogates the antimicrobial effects of tobramycin but potentiates the full antimicrobial activity of the concomitant antibiotic (32).
Table 1. Main characteristics of in vitro studies comparing ceftazidime-avibactam alone vs. ceftazidime-avibactam containing combinations.
Table 2. In vitro synergy and antagonism of antibiotic combinations against carbapenem-resistant Enterobacterales and multidrug-resistant Pseudomonas aeruginosa.
Table 2 presents the in vitro synergy and antagonism rates of the combination regimens tested for CRE and MDR-PA. The synergy rate between CZA and aztreonam was >80% in all studies using metallo-β-lactamases-producing CRE and MDR-PA (25, 27, 34, 37, 43). Even though the results were quite heterogeneous as shown in Table 2, CZA plus meropenem or imipenem (30, 38, 40, 44) and CZA plus amikacin can show synergistic activity, particularly against CRE isolates (24, 29, 31, 38, 39). Similarly, synergy rates for the combination of CZA plus fosfomycin were highly variable against CRE isolates (26, 38, 41, 44) but very low against MDR-PA in one study (38). In other combinations, the synergy rates were generally low against CRE and MDR-PA isolates (Table 2). For CZA-resistant MDR-PA isolates, Montero et al. found high rate of synergy between colistin and CZA (39). Conversely, Shields et al. demonstrated significant antagonism between colistin and CZA against KPC-expressing CZA-susceptible Enterobacterales isolates (45).
After assessment of relevant articles, seven were included in narrative data synthesis. All studies except one from Canada were reported from the United States. Five studies included carbapenem-resistant Klebsiella pneumoniae (CRKP) isolates (3 KPC, 1 NDM and 1 NDM plus OXA-48 producers) and two assessed MDR-PA. The in vivo infection models used are as follows: Galleria mellonella survival model (n = 3), neutropenic mouse thigh infection model (n = 2), and neutropenic mouse pneumonia model (n = 2).
In a Galleria mellonella survival model, CZA (1.56/1.56 mg/kg) ensured 70% survival of MDR-PA-challenged larvae after 24 h, while CZA (1.56/1.56 mg/kg) plus tobramycin-cyclam (3.12 mg/kg) provided 100% survival after 24 h (32). In another Galleria mellonella survival model, human simulated regimens of CZA had better antibacterial activity when combined with either meropenem or amikacin against KPC-producing K. pneumoniae isolates (n = 2) with CZA MIC level of 8/4 mg/L (40). In contrast, these combination regimens did not have significant killing effect compared to single CZA regimen against K. pneumoniae isolates (n = 2) with lower (1/4 mg/L) MIC of CZA in virtue of excellent in vivo activity of CZA monotherapy (40). In the last Galleria mellonella survival model, CZA and polymyxin B were tested with concentrations of being four times MIC alone and in combination against KPC-producing K. pneumoniae isolates (n = 3). The CZA plus polymyxin B combination did not significantly improve larval survival in comparison with CZA alone against any strain (28). Likewise, mean survival times were similar in both groups.
In two neutropenic mouse thigh infection models, the authors demonstrated significant synergy between CZA plus aztreonam and CZA plus fosfomycin, respectively. Marshall et al. showed that as compared to CZA (32 mg/kg q8h) and aztreonam (32 mg/kg q8h) alone, CZA (32/8 mg/kg q8h) plus aztreonam (32 mg/kg q8h) combination reduces bacterial titers of an NDM-producing K. pneumoniae by 3.79 log10 CFU and 2.08 log10 CFU per thigh, respectively (22). Papp-Wallace et al. revealed that the human simulated doses of CZA and fosfomycin combination provides significant reduction in CFUs of CZA-susceptible MDR-PA isolates (n = 2) by approximately 5 logs, compared with the vehicle-treated control (46). Separate administration of CZA and fosfomycin reduced bacterial loads by approximately 1 log and 2 logs, respectively, compared with those of the vehicle-treated control (46).
Huang et al. performed a neutropenic mouse pneumonia model with inoculation of a KPC-producing K. pneumoniae isolate (31). At 24 h, mean bacterial lung concentrations (± the standard deviation) for the untreated control group, gentamicin, CZA, and gentamicin plus CZA combination were 8.97 ± 0.09, 4.64 ± 0.34, 5.95 ± 0.25, and 2.95 ± 1.07 log10 CFU/lung, respectively. In a neutropenic murine lung infection model utilizing NDM, OXA-48 and CTX-M expressing K. pneumoniae strain (n = 1), the humanized dose of CZA monotherapy (2.5 g q8h) led to substantial in vivo killing activity (2.43 log10 CFU reduction at 24 h), and consequently, no significant benefit was found compared to CZA monotherapy with CZA plus aztreonam (2 g every 6 h) or CZA plus tigecycline (100 mg q12h) (2.54 log10 CFU reduction with CZA plus aztreonam and 2.15 log10 CFU reduction with CZA plus tigecycline at 24 h) (47). Even with CZA plus aztreonam plus tigecycline combination, bacterial load can be reduced by 2.18 log10 CFU at 24 h, which did not provide significant advantage over CZA monotherapy (47).
In summary, CZA plus fosfomycin and CZA plus aztreonam were shown to have more robust antibacterial activity than CZA alone in neutropenic mouse thigh infection models utilizing MDR-PA and NDM-producing K. pneumoniae, respectively. Intriguingly, in a neutropenic murine lung infection model, CZA alone showed excellent killing activity against NDM, OXA-48 and CTX-M-expressing K. pneumoniae, raising the clinical impact of NDM production on these infections into question (47). In another study, more effective antibacterial activity was shown with CZA plus meropenem or amikacin against KPC-producing K. pneumoniae strains if CZA MIC level is near to susceptibility breakpoint. For other combinations, no benefit of the combination regimens was demonstrated over CZA alone.
Of the 20 studies evaluated, 9 were retrospective multi-center cohort (48–56), 8 retrospective single-center cohort (15, 57–63), 1 prospective multi-center cohort (64), 1 prospective single-center cohort (65) and 1 multi-center case–control study (66). There were no RCTs which address combination therapy and monotherapy with CZA. The number of patients included ranged from 13 to 577. Only four studies assessed bloodstream infections alone and the rest included patients with different types of infections. These studies were conducted in various countries as follows: Spain (6), United States (4), Italy (4), Greece (1), Saudi Arabia (1), Brazil (1), India (1), China (1), Europe, Israel and Australia (1). As shown in Table 3, in vitro susceptibility of carbapenems was not tested in two studies (55, 60) and carbapenem non-susceptibility rate was <100% among isolates collected in four studies (49, 57, 64, 65). Similarly, CZA susceptibility rate was not documented in three studies (49, 50, 55), as well as it was found as 89.5% and 79% in two studies, respectively (57, 61). More than half of the studies had CRE infections, while six included CRKP infections (15, 52–54, 56, 60). MDR/XDR-PA infections were assessed only in one study (57) and two studies included mixed types of infections caused by CRE or carbapenem-resistant P. aeruginosa (CRPA) and CRE or MDR-PA, respectively (49, 51). The causative pathogens were susceptible to concomitantly administered antibiotic(s) in the vast majority of the studies, five studies did not document in vitro antimicrobial activity of partner antibiotics (48, 56, 58, 61, 66) and 70% of concomitant antibiotics had in vitro antimicrobial activity against the causative pathogens in one study (62). Intriguingly, none of the co-administered antibiotics were in vitro active against the identified pathogens in one study (53). Although median time between the onset of infection and administration at least one in vitro active antibiotic was not reported in 12 studies, it was generally quite long in other studies as shown in Table 3. Except four studies included only bloodstream infections, secondary bacteremia was frequent in other studies (Table 3). The definitions of investigated outcomes were highly heterogeneous and are portrayed in Table 4. In three studies, 30-day mortality rate was explored along with mortality rates at other time points (56, 63, 65). Since the majority of the studies included in this scoping review analyzed 30-day mortality, we preferred to report the 30-day mortality rates in these studies. All-cause mortality was investigated in all but one and ranged from 6.3% to 47.6% in patients treated with CZA monotherapy and from 0% to 44.0% in those receiving CZA combination regimens (Table 5). Only four studies performed a multivariable analysis while comparing monotherapy and combination therapies. In the study conducted by Zheng et al., CZA as a component of combination regimens was found to be associated with lower risk of mortality in comparison with CZA monotherapy (24.4% vs. 47.6%; HR, 0.167; 95 CI, 0.06–0.46) (53). In contrast, CZA combination regimens were associated with higher mortality in another study (27.8% vs. 11.8%; HR, 2.49; 95% CI, 1.10–5.63) (64). Alqahtani et al. reported no significant difference in mortality between CZA combination therapies and CZA monotherapy (27% vs. 16%; OR, 1.5; 95% CI, 0.61–3.64) (63). Lastly, in an Italian retrospective matched cohort study, there was no difference between CZA plus fosfomycin and CZA ± other antibiotic groups in terms of 30-day mortality (14.8% vs. 18.0%; HR, 0.72; 95% CI, 0.28–1.85) (56).
Table 3. Main characteristics of clinical studies comparing ceftazidime-avibactam vs. ceftazidime-avibactam-containing combination regimens.
Table 4. Definitions and time points used in clinical studies to define mortality, clinical cure, microbiological cure, and infection relapse.
Table 5. Studies assessing clinical outcomes of ceftazidime-avibactam monotherapy vs. ceftazidime-avibactam-containing combination regimens.
Among all studies, 14 assessed clinical cure or improvement of recruited patients by applying definitions shown in Table 4. The clinical cure rate ranged from 55.9% to 86.6% in the CZA monotherapy group and 44.8% to 100% in the CZA combination therapy group. Only the study showing lowest rate of clinical cure with CZA combination regimens executed multivariable analysis and reported that CZA combination therapy is significantly associated with lower clinical cure rate as compared to CZA monotherapy (44.8% vs. 62.5%; OR, 0.02; 95% CI, 0.01–0.38) (57). Only eight studies documented microbiological eradication rates in the CZA monotherapy and combination therapy groups (Table 5). While they were between 35.2 and 67.9% in the monotherapy groups, they ranged from 38.2% to 66.6% in the CZA combination therapy groups. In the study conducted by Oliva et al., blood culture negativity 72 h after treatment initiation for KPC-producing CRKP was higher in CZA plus fosfomycin arm than in CZA ± another antibiotic arm. However, there was no difference between the microbiological eradication rates of the two groups on the 7th and 14th days (56). Adverse event rates of monotherapy and combination therapies were reported in four studies and they seem to be quite similar between the two treatment groups in all of these studies (Table 5). Additionally, six studies explored the acute kidney injury (AKI) rates of CZA monotherapy and CZA combination regimens (Table 5). The AKI rates were numerically higher in CZA combination regimens in all but one study in which no AKI episode was detected in both groups (50).
One of the biggest issues of CZA treatment is the development of resistance during or following treatment. Except one study conducted by Ackley et al. (48), none of nine studies demonstrated a substantial difference between monotherapy and combination therapies in terms of development of CZA resistance during or following therapy (Table 5). In the study of Ackley et al., resistance development was not observed in any patients treated with CZA combination regimens, while 7.3% of monotherapy group developed resistance against CZA (48). Similarly, except the studies conducted by Ackley et al. and Oliva et al. (48, 56), five studies indicated numerically higher infection relapse rates in CZA combination therapy groups and no infection relapse was observed in one study (Table 5).
This scoping review systematically documents the existing data on in vitro, in vivo and clinical studies comparing CZA monotherapy and CZA combination therapies. In vitro studies elucidated reliable synergy between CZA and aztreonam against metallo-β-lactamase-producing CRE and MDR-PA isolates. Consistently, in a recent prospective cohort study including 102 patients with bloodstream infection caused by metallo-β-lactamase-producing CRE being treated with either CZA plus aztreonam (n = 52) or colistin and tigecycline-based regimens (n = 50), propensity-score-adjusted multivariable analysis showed a significant association between CZA plus aztreonam and lower 30-day mortality (HR 0.37, 95% CI 0.13–0.74) (67). It should be underlined that absence of data from RCTs, absence of standardized antimicrobial susceptibility testing method and clinical interpretative breakpoints approved for this combination and lack of availability of aztreonam in some countries are important barriers to routine use of this combination regimen in daily practice. Furthermore, aztreonam (8 g daily) can lead to elevations in hepatic transaminases (68).
In general, in vitro studies showed reliable synergy between CZA and meropenem against KPC-producing CRE isolates. Considering the restoration of meropenem susceptibility in some CRE isolates carrying KPC-Ω-loop mutations and results of in vitro synergy studies, CZA plus meropenem can be considered to test in clinical studies as an alternative combination regimen for treatment of KPC-producing CRE infections.
CZA plus amikacin is one of the most common combination regimens investigated in in vitro synergy studies. The great majority of these studies found good synergy between amikacin and CZA against CRE isolates as well as a low number of studies showed good synergy between the two antibiotics against MDR-PA strains (24, 29, 31, 38, 39). However, two studies demonstrated low rate of in vitro synergy between the two antibiotics (36, 40). These conflicting findings are likely stemmed from differences in methods used to test in vitro synergy, doses of antibiotics adjusted, molecular backgrounds of tested microorganisms, and MIC levels of CZA and amikacin against pathogens used in these experiments.
The synergy between CZA and polymyxins was frequently explored in in vitro studies. While some studies reported high rate of synergy between these two antibiotics (33, 35, 39), others showed very low synergy between polymyxins and CZA (28, 36, 40, 45). Two studies also underlined the high risk of antagonism between polymyxins and CZA against KPC-producing K. pneumoniae isolates (28, 45). For other combinations, existing studies generally demonstrated low synergy between CZA and these antibiotics.
As CZA generally has an excellent in vitro antibacterial activity against CRE and MDR-PA isolates, synergistic interactions would be more useful for isolates with CZA MICs very close to the susceptibility breakpoints or non-metallo-β-lactamase-producing CZA-resistant isolates (69–72). Only two studies investigated in vitro synergy between CZA and partner antibiotics against non-metallo-β-lactamase-producing MDR-PA and/or CRE isolates with <50% CZA susceptibility rate and showed favorable results between CZA and amikacin (29, 37). Likewise, Nath et al. showed that there is no need to combine CZA with meropenem for highly CZA-susceptible KPC-producing K. pneumoniae isolates. In contrast, CZA plus meropenem yielded positive results for these isolates with a higher CZA MIC level (8/4 mg/L) (40). Although one study demonstrated enhanced synergy between CZA and colistin in CZA-resistant MDR-PA isolates, more studies underpinning this finding are needed (39).
There are very small numbers of in vivo studies comparing antibacterial activities of CZA combination regimens vs. CZA monotherapy. These studies were typically conducted on very few pathogens using various types of in vivo infection models. Therefore, it is not possible to confirm the activity of a specific combination regimen with at least 2 different studies performed using the same in vivo infection model testing the same bacterial species with a similar CZA MIC level and molecular resistance profile. As a result, it is highly unlikely to draw a firm conclusion from these in vivo studies’ results.
Even though in vitro and in vivo studies indicated that some combination regimens have better antimicrobial activity than CZA alone against CRE and MDR-PA, clinical studies comparing the clinical efficacy and safety of these combination regimens compared to CZA monotherapy play a key role in determining optimal therapeutic regimens for patients infected with these pathogens (73, 74). Evidence on CZA monotherapy and combination therapies comes from observational and case–control studies involving patients with mixed-type infections of varying clinical severity and these studies have significant limitations that should be highlighted. First, CZA combination regimens are basically composed of two or more antibiotics with variable in vitro antimicrobial activity. Although great majority of studies defined combination therapy as CZA plus at least one in vitro active antibiotic, in two studies, overall in vitro susceptibility rates of partner antibiotics used were 0% and 70%, respectively (53, 62). More importantly, individual data for specific combination regimens (e.g., CZA plus amikacin) were not presented in the vast majority of studies. Second, these studies typically include infections due to pathogens with various patterns of antimicrobial resistance, ranging from strains susceptible to carbapenems to those resistant to all available antimicrobials. Of these studies, little explored clinical outcomes of non-carbapenemase producers, CRE other than K. pneumoniae and MDR-PA. Furthermore, the definitions of combination therapy, clinical cure/improvement and infections relapses as well as mortality time points (e.g., 30-day, 90-day, in-hospital) were highly variable among the studies. Finally, multivariable analyses were seldom performed in these studies mainly due to the small number of patients included. Overall, in clinical studies published up to now, mortality, clinical cure, adverse events, and development of CZA resistance after exposure were generally similar in CZA monotherapy and combination therapy groups. However, antibiotic-related acute kidney injury and infection relapses were numerically higher in patients receiving CZA combination therapies. As only one study compared CZA monotherapy vs. CZA combination therapies against MDR/XDR-PA infections, more data are urgently needed to appreciate the differences between the two therapies against MDR-PA infections (57).
In tandem with our findings, recent European Society of Clinical Microbiology and Infectious Diseases (ESCMID) guidelines and Infectious Diseases Society of America (IDSA) guidance document recommend not using CZA as a component of combination regimens for CRE infections except for those caused by metallo-β-lactamase producers where CZA can be combined with aztreonam (75, 76). ESCMID guidelines do not also make any firm suggestion on the use of CZA for CRPA infections even as a monotherapy due to shortage of adequate evidence. In contrast, the IDSA guidance document does recommend CZA monotherapy for severe DTR-PA infections, but does not recommend prescribing CZA combination regimens for these infections (76). Considering the paucity of clinical data to make a recommendation on treatment decisions of CRE and MDR-PA infections, RCTs comparing CZA monotherapy vs. combination therapies for infections caused by these resistant pathogens (i.e., pathogen-directed RCTs) are needed. Given the paucity of clinical and preclinical data that consistently shows that a particular CZA-containing combination regimen(s) may be beneficial against CRE and/or MDR-PA infections, it is extremely difficult to prioritize any combination regimen in a pathogen-directed RCT.
Currently, treatment approaches for CRE and MDR-PA infections are constantly evolving, and precision medicine has recently gained significant popularity. In this innovative multistep medicinal approach, patient management is individualized based on patient and pathogen characteristics (13). Similarly, aminoglycoside-containing regimens should be individualized based on aminoglycosides modifying enzymes produced by the causative microorganisms. Huang et al. showed that gentamicin plus CZA typically had better in vitro activity against KPC-producing K. pneumoniae isolates with aac (6′)-Ib, while amikacin in combination with CZA exhibited more favorable activity against the isolates with aac (6′)-Ib’ (31).
Given the limited evidence on CZA monotherapy vs. CZA-containing combination therapies, we definitely need further studies to address some important questions. First, pathogen-directed RCTs are urgently needed to fill this evidence gap considering the high-certainty evidence provided by RCTs. Second, large-scale observational studies should be conducted to understand efficacy and safety of CZA monotherapy and combination therapies in real life conditions and for some patients who are not eligible to be included in a RCT. In these observational studies, biases (e.g., selection bias, immortal time bias) and effects of relevant confounding factors should be mitigated using appropriate statistical methods. For this purpose, depending on the investigated outcomes, inverse probability treatment weighting using the propensity score, propensity score matching, multivariable logistic regression or Cox regression analyses can be performed (77). If there is a risk of immortal time bias in the survival analysis, time dependent Cox regression can be chosen to reduce this bias (78, 79). Third, genomic characterization of the causative bacteria should be undertaken to direct a precision medicine approach. Fourth, optimal dosing and infusion regimens of CZA and companion antibiotics and their treatment durations should be explored in future studies. Fifth, the impact of high-inoculum infections on the efficacy of CZA treatment should be investigated. Although in vitro studies demonstrated the resilience of CZA against high inoculum effect, the clinical activity of CZA monotherapy vs. combination therapies are not yet compared in high-inoculum infections such as osteomyelitis and endocarditis (80). Sixth, in vitro activity of CZA diminishes against MDR-PA isolates identified in sputum samples of cystic fibrosis patients compared to those isolated from other clinical samples (81). P. aeruginosa can form a biofilm and grow anaerobically in the cystic fibrosis patients’ lower respiratory tract (82). The biofilm structure enables P. aeruginosa strains to resist the actions of both antibiotics and the immune response (83). Considering the difficulties of treatment of these infections, the combination regimens including CZA and an in vitro active aminoglycoside or polymyxins may be more reasonable option than CZA monotherapy for the treatment of these patients. Future studies addressing this question are required to optimize treatment recommendations in this group. Lastly, clinical evidence is lacking on the efficacy and safety of CZA monotherapy vs. combination therapies for infections caused by pathogens with CZA MIC value very close to the susceptibility breakpoints.
Although in vitro studies have shown positive results with some CZA combination regimens, clinical studies have generally not found any clinical benefit with CZA combination therapies. Despite these findings, the potential benefit of CZA-containing combination therapies remains controversial for some specific patient groups. In addition, there is an unmet need for RCTs comparing CZA monotherapy and CZA-containing combination therapies.
The original contributions presented in the study are included in the article/Supplementary material, further inquiries can be directed to the corresponding author.
AA: conceptualization, methodology, literature search, and drafting of the manuscript. YE: conceptualization, literature search, and editing and approval of manuscript. JH: literature search and editing and approval of manuscript. PH and DP: conceptualization, supervision, and editing and approval of manuscript. All authors contributed to the article and approved the submitted version.
PH has received research grants from Merck Sharpe & Dohme (MSD), Sandoz and Shionogi outside of the submitted work; speakers fees from Pfizer, Sandoz, OpGen and Sumitomo and has served on advisory boards for Sandoz, OpGen and MSD. JH has received speaker fees from Pfizer, MSD, Angelini and Zambon, and has served on advisory boards for Menarini, Pfizer and TFF Pharmaceuticals. DP has received research grants from Shionogi, Pfizer, bioMerieux and Merck, and has served on advisory boards for Pfizer, Merck, Entasis, VenatoRx, Qpex and the AMR Action Fund.
The remaining authors declare that the research was conducted in the absence of any commercial or financial relationships that could be construed as a potential conflict of interest.
All claims expressed in this article are solely those of the authors and do not necessarily represent those of their affiliated organizations, or those of the publisher, the editors and the reviewers. Any product that may be evaluated in this article, or claim that may be made by its manufacturer, is not guaranteed or endorsed by the publisher.
The Supplementary material for this article can be found online at: https://www.frontiersin.org/articles/10.3389/fmed.2023.1249030/full#supplementary-material
1. World Health Organization. Global antimicrobial resistance surveillance system (GLASS) report: early implementation 2017–2018 (2019). Available at: https://www.who.int/publications/i/item/9789241515061 (Accessed May 31, 2023).
2. Centers for Disease Control and Prevention. Antibiotic resistance threats in the United States (2019). Available at: https://www.cdc.gov/drugresistance/biggest-threats.html (Accessed May 31, 2023).
3. GBD. Antimicrobial resistance collaborators. Global mortality associated with 33 bacterial pathogens in 2019: a systematic analysis for the global burden of disease study 2019. Lancet. (2019) 400:2221–48. doi: 10.1016/S0140-6736(22)02185-7
4. Murray, CJ, Ikuta, KS, Sharara, F, Swetschinski, L, Aguilar, GR, Gray, A, et al. Global burden of bacterial antimicrobial resistance in 2019: a systematic analysis. Lancet. (2022) 399:629–55. doi: 10.1016/S0140-6736(21)02724-0
5. Centers for Disease Control and Prevention. Facility guidance for control of carbapenem-resistant Enterobacteriaceae (CRE): November 2015 update – CRE toolkit (2015). Available at: https://www.cdc.gov/hai/pdfs/cre/cre-guidance-508.pdf (Accessed 31 May 2023).
6. Magiorakos, AP, Srinivasan, A, Carey, RB, Carmeli, Y, Falagas, ME, Giske, CG, et al. Multidrug-resistant, extensively drug-resistant and pandrug-resistant bacteria: an international expert proposal for interim standard definitions for acquired resistance. Clin Microbiol Infect. (2012) 18:268–81. doi: 10.1111/j.1469-0691.2011.03570.x
7. Lister, PD, Wolter, DJ, and Hanson, ND. Antibacterial-resistant Pseudomonas aeruginosa: clinical impact and complex regulation of chromosomally encoded resistance mechanisms. Clin Microbiol Rev. (2009) 22:582–610. doi: 10.1128/CMR.00040-09
8. Wolter, DJ, and Lister, PD. Mechanisms of beta-lactam resistance among Pseudomonas aeruginosa. Curr Pharm Des. (2013) 19:209–22. doi: 10.2174/138161213804070311
9. Karlowsky, JA, Kazmierczak, KM, de Jonge, BLM, Hackel, MA, Sahm, DF, and Bradford, PA. In vitro activity of aztreonam-avibactam against Enterobacteriaceae and Pseudomonas aeruginosa isolated by clinical laboratories in 40 countries from 2012 to 2015. Antimicrob Agents Chemother. (2017) 61:e00472–17. doi: 10.1128/AAC.00472-17
10. Reyes, J, Komarow, L, Chen, L, Ge, L, Hanson, BM, Cober, E, et al. Antibacterial resistance leadership group and multi-drug resistant organism network investigators. Global epidemiology and clinical outcomes of carbapenem-resistant Pseudomonas aeruginosa and associated carbapenemases (POP): a prospective cohort study. Lancet Microbe. (2023) 4:e159–70. doi: 10.1016/S2666-5247(22)00329-9
11. Kadri, SS, Adjemian, J, Lai, YL, Spaulding, AB, Ricotta, E, Prevots, DR, et al. Difficult-to-treat resistance in gram-negative bacteremia at 173 US hospitals: retrospective cohort analysis of prevalence, predictors, and outcome of resistance to all first-line agents. Clin Infect Dis. (2018) 67:1803–14. doi: 10.1093/cid/ciy378
12. Livermore, DM, Meunier, D, Hopkins, KL, Doumith, M, Hill, R, Pike, R, et al. Activity of ceftazidime/avibactam against problem Enterobacteriaceae and Pseudomonas aeruginosa in the UK, 2015–2016. J Antimicrob Chemother. (2018) 73:648–57. doi: 10.1093/jac/dkx438
13. Aslan, AT, and Akova, M. The role of colistin in the era of new β-lactam/β-lactamase inhibitor combinations. Antibiotics. (2022) 11:277. doi: 10.3390/antibiotics11020277
14. Monogue, ML, Sakoulas, G, Nizet, V, and Nicolau, DP. Humanized exposures of a β-lactam-β-lactamase inhibitor, Tazobactam, versus non-β-lactam-β-lactamase inhibitor, avibactam, with or without colistin, against Acinetobacter baumannii in murine thigh and lung infection models. Pharmacology. (2018) 101:255–61. doi: 10.1159/000486445
15. Shields, RK, Nguyen, MH, Chen, L, Press, EG, Potoski, BA, Marini, RV, et al. Ceftazidime-avibactam is superior to other treatment regimens against carbapenem-resistant Klebsiella pneumoniae bacteremia. Antimicrob Agents Chemother. (2017) 61:e00883e917. doi: 10.1128/AAC.00883-17
16. van Duin, D, Lok, JJ, Earley, M, Cober, E, Richter, SS, Perez, F, et al. Colistin versus ceftazidime-avibactam in the treatment of infections due to carbapenem resistant Enterobacteriaceae. Clin Infect Dis. (2018) 66:163–71. doi: 10.1093/cid/cix783
17. Paul, M, Carmeli, Y, Durante-Mangoni, E, Mouton, JW, Tacconelli, E, Theuretzbacher, U, et al. Combination therapy for carbapenem-resistant gram-negative bacteria. J Antimicrob Chemother. (2014) 69:2305–9. doi: 10.1093/jac/dku168
18. Vardakas, KZ, Mavroudis, AD, Georgiou, M, and Falagas, ME. Intravenous colistin combination antimicrobial treatment vs. monotherapy: a systematic review and meta-analysis. Int J Antimicrob Agents. (2018) 51:535–47. doi: 10.1016/j.ijantimicag.2017.12.020
19. Shields, RK, Potoski, BA, Haidar, G, Hao, B, Doi, Y, Chen, L, et al. Clinical outcomes, drug toxicity, and emergence of ceftazidime-avibactam resistance among patients treated for carbapenem-resistant Enterobacteriaceae infections. Clin Infect Dis. (2016) 63:1615–8. doi: 10.1093/cid/ciw636
20. Tumbarello, M, Raffaelli, F, Giannella, M, Mantengoli, E, Mularoni, A, Venditti, M, et al. Ceftazidime-avibactam use for Klebsiella pneumoniae carbapenemases-producing K. pneumoniae infections: a retrospective observational multicenter study. Clin Infect Dis. (2021) 73:1664–76. doi: 10.1093/cid/ciab176
21. Tricco, AC, Lillie, E, Zarin, W, O’Brien, KK, Colquhoun, H, Levac, D, et al. PRISMA extension for scoping reviews (PRISMA-ScR): checklist and explanation. Ann Intern Med. (2018) 169:467–73. doi: 10.7326/m18-0850
22. Marshall, S, Hujer, AM, Rojas, LJ, Papp-Wallace, KM, Humphries, RM, Spellberg, B, et al. Can ceftazidime-avibactam and aztreonam overcome β-lactam resistance conferred by metallo-β-lactamases in Enterobacteriaceae? Antimicrob Agents Chemother. (2017) 61:e02243–16. doi: 10.1128/AAC.02243-16
23. Giddins, MJ, Macesic, N, Annavajhala, MK, Stump, S, Khan, S, McConville, TH, et al. Successive emergence of ceftazidime-avibactam resistance through distinct genomic adaptations in blaKPC-2- harboring Klebsiella pneumoniae sequence type 307 isolates. Antimicrob Agents Chemother. (2018) 62:e02101–17. doi: 10.1128/AAC.02101-17
24. Almarzoky Abuhussain, SS, Kuti, JL, and Nicolau, DP. Antibacterial activity of human simulated epithelial lining fluid concentrations of ceftazidime-avibactam alone or in combination with amikacin inhale (BAY41-6551) against carbapenem-resistant Pseudomonas aeruginosa and Klebsiella pneumoniae. Antimicrob Agents Chemother. (2018) 62:e00113–8. doi: 10.1128/AAC.00113-18
25. Avery, LM, and Nicolau, DP. Assessing the in vitro activity of ceftazidime/avibactam and aztreonam among carbapenemase-producing Enterobacteriaceae: defining the zone of hope. Int J Antimicrob Agents. (2018) 52:688–91. doi: 10.1016/j.ijantimicag.2018.07.011
26. Avery, LM, Sutherland, CA, and Nicolau, DP. In vitro investigation of synergy among fosfomycin and parenteral antimicrobials against carbapenemase-producing Enterobacteriaceae. Diagn Microbiol Infect Dis. (2019) 95:216–20. doi: 10.1016/j.diagmicrobio.2019.05.014
27. Biagi, M, Wu, T, Lee, M, Patel, S, Butler, D, and Wenzler, E. Searching for the optimal treatment for metallo- and serine-β-lactamase producing Enterobacteriaceae: aztreonam in combination with ceftazidime-avibactam or meropenem-vaborbactam. Antimicrob Agents Chemother. (2019) 63:e01426–19. doi: 10.1128/AAC.01426-19
28. Borjan, J, Meyer, KA, Shields, RK, and Wenzler, E. Activity of ceftazidime-avibactam alone and in combination with polymyxin B against carbapenem-resistant Klebsiella pneumoniae in a tandem in vitro time-kill/in vivo galleria mellonella survival model analysis. Int J Antimicrob Agents. (2020) 55:105852. doi: 10.1016/j.ijantimicag.2019.11.009
29. Chen, T, Xu, W, Yu, K, Zeng, W, Xu, C, Cao, J, et al. In vitro activity of ceftazidime-avibactam alone and in combination with amikacin against colistin-resistant gram-negative pathogens. Microb Drug Resist. (2021) 27:401–9. doi: 10.1089/mdr.2019.0463
30. Gaibani, P, Lewis, RE, Volpe, SL, Giannella, M, Campoli, C, Landini, MP, et al. In vitro interaction of ceftazidime-avibactam in combination with different antimicrobials against KPC-producing Klebsiella pneumoniae clinical isolates. Int J Infect Dis. (2017) 65:1–3. doi: 10.1016/j.ijid.2017.09.017
31. Huang, Y, Sokolowski, K, Rana, A, Singh, N, Wang, J, Chen, K, et al. Generating genotype-specific aminoglycoside combinations with ceftazidime/avibactam for KPC-producing Klebsiella pneumoniae. Antimicrob Agents Chemother. (2021) 65:e0069221. doi: 10.1128/AAC.00692-21
32. Idowu, T, Ammeter, D, Arthur, G, Zhanel, GG, and Schweizer, F. Potentiation of β-lactam antibiotics and β-lactam/β-lactamase inhibitor combinations against MDR and XDR Pseudomonas aeruginosa using non-ribosomal tobramycin-cyclam conjugates. J Antimicrob Chemother. (2019) 74:2640–8. doi: 10.1093/jac/dkz228
33. Mataraci Kara, E, Yilmaz, M, Istanbullu Tosun, A, and Özbek, ÇB. Evaluation of the synergy of ceftazidime/avibactam in combination with colistin, doripenem, levofloxacin, tigecycline, and tobramycin against OXA-48 producing Enterobacterales. J Chemother. (2020) 32:171–8. doi: 10.1080/1120009X.2020.1761172
34. Lee, M, Abbey, T, Biagi, M, and Wenzler, E. Activity of aztreonam in combination with ceftazidime–avibactam against serine- and metallo-β-lactamase–producing Pseudomonas aeruginosa. Diagn Microbiol Infect Dis. (2021) 99:115227. doi: 10.1016/j.diagmicrobio.2020.115227
35. Ma, X, He, Y, Yu, X, Cai, Y, Zeng, J, Cai, R, et al. Ceftazidime/avibactam improves the antibacterial efficacy of polymyxin B against polymyxin B Heteroresistant KPC-2-producing Klebsiella pneumoniae and hinders emergence of resistant subpopulation in vitro. Front Microbiol. (2019) 10:2029. doi: 10.3389/fmicb.2019.02029
36. Manning, N, Balabanian, G, Rose, M, Landman, D, and Quale, J. Activity of ceftazidime-avibactam against clinical isolates of Klebsiella pneumoniae, including KPC-carrying isolates, endemic to New York City. Microb Drug Resist. (2018) 24:35–9. doi: 10.1089/mdr.2016.0293
37. Maraki, S, Mavromanolaki, VE, Moraitis, P, Stafylaki, D, Kasimati, A, Magkafouraki, E, et al. Ceftazidime-avibactam, meropenen-vaborbactam, and imipenem-relebactam in combination with aztreonam against multidrug-resistant, metallo-β-lactamase-producing Klebsiella pneumoniae. Eur J Clin Microbiol Infect Dis. (2021) 40:1755–9. doi: 10.1007/s10096-021-04197-3
38. Mikhail, S, Singh, NB, Kebriaei, R, Rice, SA, Stamper, KC, Castanheira, M, et al. Evaluation of the synergy of ceftazidime-avibactam in combination with meropenem, amikacin, aztreonam, colistin, or fosfomycin against well-characterized multidrug-resistant Klebsiella pneumoniae and Pseudomonas aeruginosa. Antimicrob Agents Chemother. (2019) 63:e00779–19. doi: 10.1128/AAC.00779-19
39. Montero, MM, Domene Ochoa, S, López-Causapé, C, Luque, S, Sorlí, L, Campillo, N, et al. Time-kill evaluation of antibiotic combinations containing ceftazidime-avibactam against extensively drug-resistant Pseudomonas aeruginosa and their potential role against ceftazidime-avibactam-resistant isolates. Microbiol Spectr. (2021) 9:e0058521. doi: 10.1128/Spectrum.00585-21
40. Nath, S, Moussavi, F, Abraham, D, Landman, D, and Quale, J. In vitro and in vivo activity of single and dual antimicrobial agents against KPC-producing Klebsiella pneumoniae. J Antimicrob Chemother. (2018) 73:431–6. doi: 10.1093/jac/dkx419
41. Ojdana, D, Gutowska, A, Sacha, P, Majewski, P, Wieczorek, P, and Tryniszewska, E. Activity of ceftazidime-avibactam alone and in combination with ertapenem, fosfomycin, and tigecycline against carbapenemase-producing Klebsiella pneumoniae. Microb Drug Resist. (2019) 25:1357–64. doi: 10.1089/mdr.2018.0234
42. Okoliegbe, IN, Hijazi, K, Cooper, K, Ironside, C, and Gould, IM. Trends of antimicrobial resistance and combination susceptibility testing of multidrug-resistant Pseudomonas aeruginosa isolates from cystic fibrosis patients: a 10-year update. Antimicrob Agents Chemother. (2021) 65:e02483–20. doi: 10.1128/AAC.02483-20
43. Pragasam, AK, Veeraraghavan, B, Shankar, BA, Bakthavatchalam, YD, Mathuram, A, George, B, et al. Will ceftazidime/avibactam plus aztreonam be effective for NDM and OXA-48-like producing organisms: lessons learnt from in vitro study. Indian J Med Microbiol. (2019) 37:34–41. doi: 10.4103/ijmm.IJMM_19_189
44. Romanelli, F, De Robertis, A, Carone, G, Dalfino, L, Stufano, M, Del Prete, R, et al. In vitro activity of ceftazidime/avibactam alone and in combination with fosfomycin and carbapenems against KPC-producing Klebsiella Pneumoniae. New Microbiol. (2020) 43:136–8.
45. Shields, RK, Nguyen, MH, Hao, B, Kline, EG, and Clancy, CJ. Colistin does not potentiate ceftazidime-avibactam killing of carbapenem-resistant Enterobacteriaceae in vitro or suppress emergence of ceftazidime-avibactam resistance. Antimicrob Agents Chemother. (2018) 62:e01018–8. doi: 10.1128/AAC.01018-18
46. Papp-Wallace, KM, Zeiser, ET, Becka, SA, Park, S, Wilson, BM, Winkler, ML, et al. Ceftazidime-avibactam in combination with fosfomycin: a novel therapeutic strategy against multidrug-resistant Pseudomonas aeruginosa. J Infect Dis. (2019) 220:666–76. doi: 10.1093/infdis/jiz149
47. Monogue, ML, Abbo, LM, Rosa, R, Camargo, JF, Martinez, O, Bonomo, RA, et al. In vitro discordance with in vivo activity: humanized exposures of ceftazidime-avibactam, aztreonam, and tigecycline alone and in combination against New Delhi metallo-β-lactamase-producing Klebsiella pneumoniae in a murine lung infection model. Antimicrob Agents Chemother. (2017) 61:e00486–17. doi: 10.1128/AAC.00486-17
48. Ackley, R, Roshdy, D, Meredith, J, Minor, S, Anderson, WE, Capraro, GA, et al. Meropenem-vaborbactam versus ceftazidime-avibactam for treatment of carbapenem-resistant Enterobacteriaceae infections. Antimicrob Agents Chemother. (2020) 64:e02313–9. doi: 10.1128/AAC.02313-19
49. Balandín, B, Ballesteros, D, Pintado, V, Soriano-Cuesta, C, Cid-Tovar, I, Sancho-González, M, et al. Multicentre study of ceftazidime/avibactam for gram-negative bacteria infections in critically ill patients. Int J Antimicrob Agents. (2022) 59:106536. doi: 10.1016/j.ijantimicag.2022
50. King, M, Heil, E, Kuriakose, S, Bias, T, Huang, V, El-Beyrouty, C, et al. Multicenter study of outcomes with ceftazidime-avibactam in patients with carbapenem-resistant Enterobacteriaceae infections. Antimicrob Agents Chemother. (2017) 61:e00449–17. doi: 10.1128/AAC.00449-17
51. Temkin, E, Torre-Cisneros, J, Beovic, B, Benito, N, Giannella, M, Gilarranz, R, et al. Ceftazidime-avibactam as salvage therapy for infections caused by carbapenem-resistant organisms. Antimicrob Agents Chemother. (2017) 61:e01964–16. doi: 10.1128/AAC.01964-16
52. Tumbarello, M, Trecarichi, EM, Corona, A, De Rosa, FG, Bassetti, M, Mussini, C, et al. Efficacy of ceftazidime-avibactam salvage therapy in patients with infections caused by Klebsiella pneumoniae carbapenemase–producing K. pneumoniae. Clin Infect Dis. (2019) 68:355–64. doi: 10.1093/cid/ciy492
53. Zheng, G, Zhang, J, Wang, B, Cai, J, Wang, L, Hou, K, et al. Ceftazidime-avibactam in combination with in vitro non-susceptible antimicrobials versus ceftazidime-avibactam in monotherapy in critically ill patients with carbapenem-resistant Klebsiella Pneumoniae infection: a retrospective cohort study. Infect Dis Ther. (2021) 10:1699–713. doi: 10.1007/s40121-021-00479-7
54. Tumbarello, M, Raffaelli, F, Giannella, M, Mantengoli, E, Mularoni, A, Venditti, M, et al. Ceftazidime-avibactam use for Klebsiella pneumoniae carbapenemase–producing K. pneumoniae infections: a retrospective observational multicenter study. Clin Infect Dis. (2021) 73:1664–76. doi: 10.1093/cid/ciab176
55. Castón, JJ, Cano, A, Pérez-Camacho, I, Aguado, JM, Carratalá, J, Ramasco, F, et al. Impact of ceftazidime/avibactam versus best available therapy on mortality from infections caused by carbapenemase-producing Enterobacterales (CAVICOR study). J Antimicrob Chemother. (2022) 77:1452–60. doi: 10.1093/jac/dkac049
56. Oliva, A, Volpicelli, L, Di Bari, S, Curtolo, A, Borrazzo, C, Cogliati Dezza, F, et al. Effect of ceftazidime/avibactam plus fosfomycin combination on 30-day mortality in patients with bloodstream infections caused by KPC-producing Klebsiella pneumoniae: results from a multicentre retrospective study. JAC Antimicrob Resist. (2022) 4:dlac121. doi: 10.1093/jacamr/dlac121
57. Corbella, L, Boán, J, San-Juan, R, Fernández-Ruiz, M, Carretero, O, Lora, D, et al. Effectiveness of ceftazidime-avibactam for the treatment of infections due to Pseudomonas aeruginosa. Int J Antimicrob Agents. (2022) 59:106517. doi: 10.1016/j.ijantimicag.2021.106517
58. De la Calle, C, Rodríguez, O, Morata, L, Marco, F, Cardozo, C, García-Vidal, C, et al. Clinical characteristics and prognosis of infections caused by OXA-48 carbapenemase-producing Enterobacteriaceae in patients treated with ceftazidime-avibactam. Int J Antimicrob Agents. (2019) 53:520–4. doi: 10.1016/j.ijantimicag.2018.11.015
59. Shields, RK, Nguyen, MH, Chen, L, Press, EG, Kreiswirth, BN, and Clancy, CJ. Pneumonia and renal replacement therapy are risk factors for ceftazidime-avibactam treatment failures and resistance among patients with carbapenem-resistant Enterobacteriaceae infections. Antimicrob Agents Chemother. (2018) 62:e02497–17. doi: 10.1128/AAC.02497-17
60. Castón, JJ, Gallo, M, García, M, Cano, A, Escribano, A, Machuca, I, et al. Ceftazidime-avibactam in the treatment of infections caused by KPC-producing Klebsiella pneumoniae: factors associated with clinical efficacy in a single-center cohort. Int J Antimicrob Agents. (2020) 56:106075. doi: 10.1016/j.ijantimicag.2020.106075
61. Rathish, B, Wilson, A, Warrier, A, Prakash, S, Babu, R, and Joy, S. Clinical outcomes in carbapenem-resistant Enterobacteriaceae infections treated with ceftazidime-avibactam: a single-center observational study. Cureus. (2021) 13:e13081. doi: 10.7759/cureus.13081
62. Iannaccone, M, Boattini, M, Bianco, G, Corcione, S, Cavallo, R, and Costa, C. Ceftazidime-avibactam susceptible to resistant KPC-producing Enterobacterales bloodstream infections: an observational study. J Chemother. (2020) 32:160–2. doi: 10.1080/1120009X.2019.1709363
63. Alqahtani, H, Alghamdi, A, Alobaidallah, N, Alfayez, A, Almousa, R, Albagli, R, et al. Evaluation of ceftazidime/avibactam for treatment of carbapenemase-producing carbapenem-resistant Enterobacterales with OXA-48 and/or NDM genes with or without combination therapy. JAC Antimicrob Resist. (2022) 4:dlac104. doi: 10.1093/jacamr/dlac104
64. Karaiskos, I, Daikos, GL, Gkoufa, A, Adamis, G, Stefos, A, Symbardi, S, et al. Ceftazidime/avibactam in the era of carbapenemase-producing Klebsiella pneumoniae: experience from a national registry study. J Antimicrob Chemother. (2021) 76:775–83. doi: 10.1093/jac/dkaa503
65. Sousa, A, Pérez-Rodríguez, MT, Soto, A, Rodríguez, L, Pérez-Landeiro, A, Martínez-Lamas, L, et al. Effectiveness of ceftazidime/avibactam as salvage therapy for treatment of infections due to OXA-48 carbapenemase-producing Enterobacteriaceae. J Antimicrob Chemother. (2018) 73:3170–5. doi: 10.1093/jac/dky295
66. Guimarães, T, Nouér, SA, Martins, RCR, Perdigão Neto, LV, Martins, WMBS, Narciso Barbosa, AC, et al. Ceftazidime-avibactam as salvage therapy for infections caused by Enterobacteriales coresistant to carbapenems and polymyxins. Antimicrob Agents Chemother. (2019) 63:e00528–19. doi: 10.1128/AAC.00528-19
67. Falcone, M, Daikos, GL, Tiseo, G, Bassoulis, D, Giordano, C, Galfo, V, et al. Efficacy of ceftazidime-avibactam plus aztreonam in patients with bloodstream infections caused by metallo-beta-lactamase-producing Enterobacterales. Clin Infect Dis. (2021) 72:1871–8. doi: 10.1093/cid/ciaa586
68. Lodise, TP, O’Donnell, JN, Raja, S, Guptill, JT, Zaharoff, S, Schwager, N, et al. Safety of ceftazidime-avibactam in combination with aztreonam (COMBINE) in a phase I, open-label study in healthy adult volunteers. Antimicrob Agents Chemother. (2022) 66:e0093522. doi: 10.1128/aac.00935-22
69. Castanheira, M, Doyle, TB, Deshpande, LM, Mendes, RE, and Sader, HS. Activity of ceftazidime/avibactam, meropenem/vaborbactam and imipenem/relebactam against carbapenemase-negative carbapenem-resistant Enterobacterales isolates from US hospitals. Int J Antimicrob Agents. (2021) 58:106439. doi: 10.1016/j.ijantimicag.2021.106439
70. Sader, HS, Castanheira, M, Farrell, DJ, Flamm, RK, and Jones, RN. Ceftazidime-avibactam activity when tested against ceftazidime-nonsusceptible Citrobacter spp., Enterobacter spp., Serratia marcescens, and Pseudomonas aeruginosa from unites states medical centers (2011–2014). Diagn Microbiol Infect Dis. (2015) 83:389–94. doi: 10.1016/j.diagmicrobio.2015.06.008
71. Aslan, AT, Kırbaş, E, Sancak, B, Tanrıverdi, ES, Otlu, B, Gürsoy, NC, et al. A retrospective observational cohort study of the clinical epidemiology of bloodstream infections due to carbapenem-resistant Klebsiella pneumoniae in an OXA-48 endemic setting. Int J Antimicrob Agents. (2022) 59:106554. doi: 10.1016/j.ijantimicag.2022.106554
72. Isler, B, Özer, B, Çınar, G, Aslan, AT, Vatansever, C, Falconer, C, et al. Characteristics and outcomes of carbapenemase harbouring carbapenem-resistant Klebsiella spp. bloodstream infections: a multicentre prospective cohort study in an OXA-48 endemic setting. Eur J Clin Microbiol Infect Dis. (2022) 41:841–7. doi: 10.1007/s10096-022-04425-4
73. Scudeller, L, Righi, E, Chiamenti, M, Bragantini, D, Menchinelli, G, Cattaneo, P, et al. Systematic review and meta-analysis of in vitro efficacy of antibiotic combination therapy against carbapenem-resistant gram-negative bacilli. Int J Antimicrob Agents. (2021) 57:106344. doi: 10.1016/j.ijantimicag.2021.106344
74. Isler, B, Aslan, AT, Akova, M, Harris, P, and Paterson, DL. Treatment strategies for OXA-48-like and NDM producing Klebsiella pneumoniae infections. Expert Rev Anti-Infect Ther. (2022) 20:1389–400. doi: 10.1080/14787210.2022.2128764
75. Paul, M, Carrara, E, Retamar, P, Tängdén, T, Bitterman, R, Bonomo, RA, et al. European Society of Clinical Microbiology and Infectious Diseases (ESCMID) guidelines for the treatment of infections caused by multidrug-resistant gram-negative bacilli (endorsed by European society of intensive care medicine). Clin Microbiol Infect. (2022) 28:521–47. doi: 10.1016/j.cmi.2021.11.025
76. Tamma, PD, Aitken, SL, Bonomo, RA, Mathers, AJ, van Duin, D, and Clancy, CJ. Infectious Diseases Society of America 2022 guidance on the treatment of extended-Spectrum β-lactamase producing Enterobacterales (ESBL-E), carbapenem-resistant Enterobacterales (CRE), and Pseudomonas aeruginosa with difficult-to-treat resistance (DTR-P. aeruginosa). Clin Infect Dis. (2022) 75:187–212. doi: 10.1093/cid/ciac268
77. Paul, M, Huttner, A, Bielicki, JA, Rodríguez-Baño, J, Kalil, AC, Leeflang, MMG, et al. Reporting methods of observational cohort studies in CMI. Clin Microbiol Infect. (2020) 26:395–8. doi: 10.1016/j.cmi.2020.01.024
78. Jones, M, and Fowler, R. Immortal time bias in observational studies of time-to-event outcomes. J Crit Care. (2016) 36:195–9. doi: 10.1016/j.jcrc.2016.07.017
79. Tabah, A, Buetti, N, Staiquly, Q, Ruckly, S, Akova, M, Aslan, AT, et al. Epidemiology and outcomes of hospital-acquired bloodstream infections in intensive care unit patients: the EUROBACT-2 international cohort study. Intensive Care Med. (2023) 49:178–90. doi: 10.1007/s00134-022-06944-2
80. Danjean, M, Hobson, CA, Gits-Muselli, M, Courroux, C, Monjault, A, Bonacorsi, S, et al. Evaluation of the inoculum effect of new antibiotics against carbapenem-resistant Enterobacterales. Clin Microbiol Infect. (2022) 28:1503.e1–3. doi: 10.1016/j.cmi.2022.06.018
81. Chalhoub, H, Tunney, M, Elborn, JS, Vergison, A, Denis, O, Plésiat, P, et al. Avibactam confers susceptibility to a large proportion of ceftazidime-resistant Pseudomonas aeruginosa isolates recovered from cystic fibrosis patients. J Antimicrob Chemother. (2015) 70:1596–8. doi: 10.1093/jac/dku551
82. Hassett, DJ, Sutton, MD, Schurr, MJ, Herr, AB, Caldwell, CC, and Matu, JO. Pseudomonas aeruginosa hypoxic or anaerobic biofilm infections within cystic fibrosis airways. Trends Microbiol. (2009) 17:130–8. doi: 10.1016/j.tim.2008.12.003
83. Rowe, SM, Miller, S, and Sorscher, EJ. Cystic fibrosis. N Engl J Med. (2005) 352:1992–2001. doi: 10.1056/NEJMra043184
Keywords: antimicrobial resistance, carbapenem resistance, synergy, combination, monotherapy
Citation: Aslan AT, Ezure Y, Horcajada JP, Harris PNA and Paterson DL (2023) In vitro, in vivo and clinical studies comparing the efficacy of ceftazidime-avibactam monotherapy with ceftazidime-avibactam-containing combination regimens against carbapenem-resistant Enterobacterales and multidrug-resistant Pseudomonas aeruginosa isolates or infections: a scoping review. Front. Med. 10:1249030. doi: 10.3389/fmed.2023.1249030
Received: 28 June 2023; Accepted: 23 August 2023;
Published: 04 September 2023.
Edited by:
Milena Dropa, University of São Paulo, BrazilReviewed by:
Biagio Pinchera, University of Naples Federico II, ItalyCopyright © 2023 Aslan, Ezure, Horcajada, Harris and Paterson. This is an open-access article distributed under the terms of the Creative Commons Attribution License (CC BY). The use, distribution or reproduction in other forums is permitted, provided the original author(s) and the copyright owner(s) are credited and that the original publication in this journal is cited, in accordance with accepted academic practice. No use, distribution or reproduction is permitted which does not comply with these terms.
*Correspondence: Abdullah Tarık Aslan, YS5hc2xhbkB1cS5lZHUuYXU=
Disclaimer: All claims expressed in this article are solely those of the authors and do not necessarily represent those of their affiliated organizations, or those of the publisher, the editors and the reviewers. Any product that may be evaluated in this article or claim that may be made by its manufacturer is not guaranteed or endorsed by the publisher.
Research integrity at Frontiers
Learn more about the work of our research integrity team to safeguard the quality of each article we publish.