- 1Division of Academic Neurosurgery, Department of Clinical Neurosciences, University of Cambridge, Cambridge, United Kingdom
- 2Medical Library, University of Cambridge, Cambridge, United Kingdom
Study design: Systematic review.
Objective: The objective of this study was to evaluate the impact of phosphodiesterase (PDE) inhibitors on neurobehavioral outcomes in preclinical models of traumatic and non-traumatic spinal cord injury (SCI).
Methods: A systematic review was conducted following the Preferred Reporting Items for Systematic Reviews and Meta-Analysis (PRISMA) guidelines and was registered with PROSPERO (CRD42019150639). Searches were performed in MEDLINE and Embase. Studies were included if they evaluated the impact of PDE inhibitors on neurobehavioral outcomes in preclinical models of traumatic or non-traumatic SCI. Data were extracted from relevant studies, including sample characteristics, injury model, and neurobehavioral assessment and outcomes. Risk of bias was assessed using the SYRCLE checklist.
Results: The search yielded a total of 1,679 studies, of which 22 met inclusion criteria. Sample sizes ranged from 11 to 144 animals. PDE inhibitors used include rolipram (n = 16), cilostazol (n = 4), roflumilast (n = 1), and PDE4-I (n = 1). The injury models used were traumatic SCI (n = 18), spinal cord ischemia (n = 3), and degenerative cervical myelopathy (n = 1). The most commonly assessed outcome measures were Basso, Beattie, Bresnahan (BBB) locomotor score (n = 13), and grid walking (n = 7). Of the 22 papers that met the final inclusion criteria, 12 showed a significant improvement in neurobehavioral outcomes following the use of PDE inhibitors, four papers had mixed findings and six found PDE inhibitors to be ineffective in improving neurobehavioral recovery following an SCI. Notably, these findings were broadly consistent across different PDE inhibitors and spinal cord injury models.
Conclusion: In preclinical models of traumatic and non-traumatic SCI, the administration of PDE inhibitors appeared to be associated with statistically significant improvements in neurobehavioral outcomes in a majority of included studies. However, the evidence was inconsistent with a high risk of bias. This review provides a foundation to aid the interpretation of subsequent clinical trials of PDE inhibitors in spinal cord injury.
Systematic review registration: https://www.crd.york.ac.uk/prospero/display_record.php?RecordID=150639, identifier: CRD42019150639.
Introduction
Spinal cord injury (SCI) has a prevalence that ranges from 250 cases per million in the Rhone-Alpes region of France to 906 cases per million in the United States of America (1). It encompasses sensory, motor, and autonomic impairments with severe consequences for physical, psychological, and social health (2).
The initial phase of SCI arises directly from mechanical trauma on the spinal cord. This triggers a secondary phase of damage from mechanisms including excitotoxicity, ischemia, and inflammation (3, 4). Neutrophils and macrophages release cytokines, proteolytic enzymes, and reactive oxygen species, resulting in damage to neurons, glia, and vascular structures (5). Vascular impairment may catalyze increased activation of voltage-gated sodium channels, leading to sodium influx and cell swelling (6, 7). Disrupted calcium homeostasis triggers glutamate release, leading to neuronal excitotoxicity and cell death (8, 9). Drugs with anti-inflammatory effects, such as phosphodiesterase (PDE) inhibitors, may therefore have efficacy in reducing the irreversible spinal cord damage that ensues from these secondary mechanisms of injury (10).
PDEs are enzymes that have proinflammatory effects, in part through degrading cyclic adenosine monophosphate (cAMP), which regulates microglia homeostasis and inflammatory cytokine expression (11). By elevating cAMP levels, PDE inhibitors have been shown to reduce inflammatory cytokine production (9, 12, 13) and promote central nervous system (CNS) regeneration (14). PDE4 is the most frequently expressed cAMP-specific PDE in neurological tissue (15) and monocytes (16) and is a therapeutic target in inflammatory disease (17).
Given the pathophysiology of SCI, adjuvant therapy with PDE inhibitors may prove beneficial through mechanisms including remyelination (18). For example, rolipram is a non-specific PDE4 inhibitor that has diverse anti-inflammatory properties (19–22) and inhibitory effects on glutamate toxicity, caspases (23, 24), and neurite outgrowth inhibition (25). Rolipram has been used both in experimental models and as a clinical therapy for asthma (26), arthritis (27), Huntington's disease (28), multiple sclerosis (21), Alzheimer's disease (29), human immunodeficiency virus (HIV) (30), and traumatic brain injury (31, 32). In addition, cilostazol, a selective PDE3 inhibitor, has been shown to have neuroprotective effects in ischemic cerebral injury (33–35) and diabetic retinal dysfunction (36). PDE3 inhibitors have also been found to have antiplatelet (36), antithrombotic (37), and vasodilatory effects (38). The role of PDE inhibition is currently being evaluated in RECEDE Myelopathy, a phase III randomized trial of the PDE4 inhibitor ibudilast in patients with degenerative cervical myelopathy (39).
The aim of this systematic review was to evaluate the impact of PDE inhibitors on neurobehavioral outcomes in preclinical models of traumatic and non-traumatic SCI.
Methods
This systematic review was conducted following the Preferred Reporting Items for Systematic Reviews and Meta-Analysis (PRISMA) guidelines (40).
Protocol and registration
The protocol was published on PROSPERO (CRD42019150639).
Eligibility criteria
The inclusion and exclusion criteria used in this review are presented in Table 1.
Population and injury model
The focus was animal models, including those using rats, mice, or rabbits. Studies involving humans were excluded. SCI models such as traumatic injury, degenerative cervical myelopathy (DCM), or spinal cord ischemia were included; injury models such as peripheral nerve injury or traumatic brain injury were excluded.
Intervention and comparison
Studies were included if they utilized PDE inhibitors, such as rolipram and cilostazol, administered intravenously, intraperitoneally, intrathecally, or via implanted drug-eluting materials. To be included, studies required a control treatment group and at least one PDE inhibitor treatment group. Studies were not excluded based on drug administration parameters such as size, frequency, or duration of dosing.
Outcomes
Neurobehavioral outcomes were the focus of this review. Studies that involved any neurobehavioral outcome, such as Basso, Beattie, and Bresnahan locomotor score, grid walking assessment, and mechanical or thermal allodynia were included. Studies that only assessed parameters such as histological or autonomic outcomes were excluded.
Information sources
A systematic search was performed of MEDLINE and Embase databases from inception until 10 January 2023.
Search
The search strategy was developed with the assistance of a medical librarian (IK) at the University of Cambridge Medical Library. The terms used to search MEDLINE and Embase are provided in Supplementary material 1. No additional search limits were applied.
Study selection
Duplicates were excluded in EndNote (Clarivate, London, UK). The abstracts were then screened independently by 19 authors using Rayyan software. Following an initial pilot of 100 articles, reviewers met to resolve disagreements and ensure consistency in the interpretation of inclusion criteria. Abstracts were then divided into seven groups. Each group was screened in duplicate by a pair of reviewers. Disagreements were resolved through discussion between the reviewers.
Data extraction
The data extracted were author, year of publication, country of experiments, study characteristics (e.g., number of experimental groups and level of evidence), sample characteristics (e.g., size, number of groups, animal species, age, sex, weight, and comorbidities), intervention (including injury model and the type, dose, frequency, and route of drug), the methods and results of any neurobehavioral assessment, and the nature of any relevant statistical analysis performed. Data were extracted by one reviewer (MB).
Data synthesis
Due to heterogeneity in injury models, interventions, and outcome reporting, a narrative synthesis was conducted using the Synthesis Without Meta-analysis (SWiM) guideline (41).
Risk of bias in individual studies
The SYRCLE (Systematic Review Center for Laboratory Animal Experimentation) tool was used to evaluate the risk of bias in included studies. The checklist is a modification of the Cochrane Collaboration risk-of-bias tool (42) using only the components that are directly applicable to animal selection (Table 2) (43). This checklist includes 10 domains relating to 6 forms of bias: selection, performance, detection, attrition, reporting, and other biases.
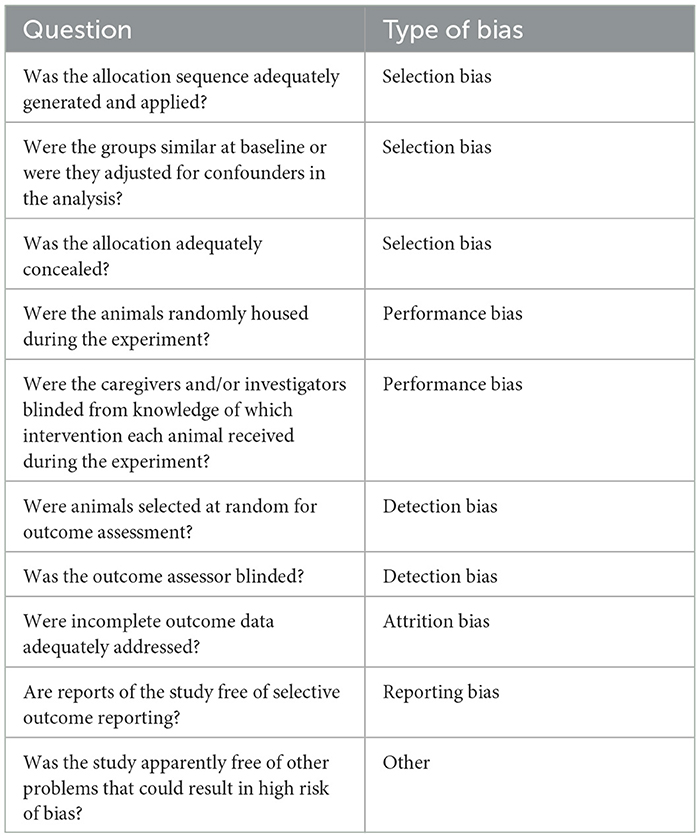
Table 2. Systematic review center for laboratory animal experimentation (SYRCLE) tool (43).
Results
Study selection
The search generated 1,679 results. A total of 223 duplicates were removed using EndNote, resulting in 1,456 unique studies, of which 23 were found to satisfy inclusion criteria following title and abstract screening. During full-text screening, eight studies were excluded for the reasons outlined in Supplementary material 2. In total, seven additional relevant studies were found on reviewing the reference lists of included studies. In total, 22 studies were therefore included in the review (Figure 1).
Study characteristics
Studies utilized either rat, mouse, or rabbit models of spinal cord injury. Models included acute SCI via spinal cord impaction devices (44–49), rod dropping (31, 50–52), microscissors (53, 54), microvascular clips (55, 56), scalpel blade incision (57), crushing with forceps (15); spinal cord ischemia via aortic clamping (58–60); and DCM via an expanding polymer insert (61) (Figure 2). Acute SCI models were either at the thoracic (n = 12) (31, 45–49, 51–53, 55, 56, 62) or cervical (n = 5) (44, 54, 57, 63, 64) level. The PDE inhibitors used were rolipram (n = 16) (31, 44–54, 57, 62–64), cilostazol (n = 4) (58–61), roflumilast (n = 1) (56), and PDE4-I (n = 1) (55) (Figure 3; Table 3). The most commonly assessed outcome measures (Table 4) were BBB (Basso, Beattie, and Bresnahan) locomotor score (n = 13) (31, 44–52, 55, 56, 62) and grid walking (n = 7) (44, 46, 50, 52, 53, 62, 63). Table 5 summarizes the sample features, injury models, interventions, outcomes, and assessments of the included studies.
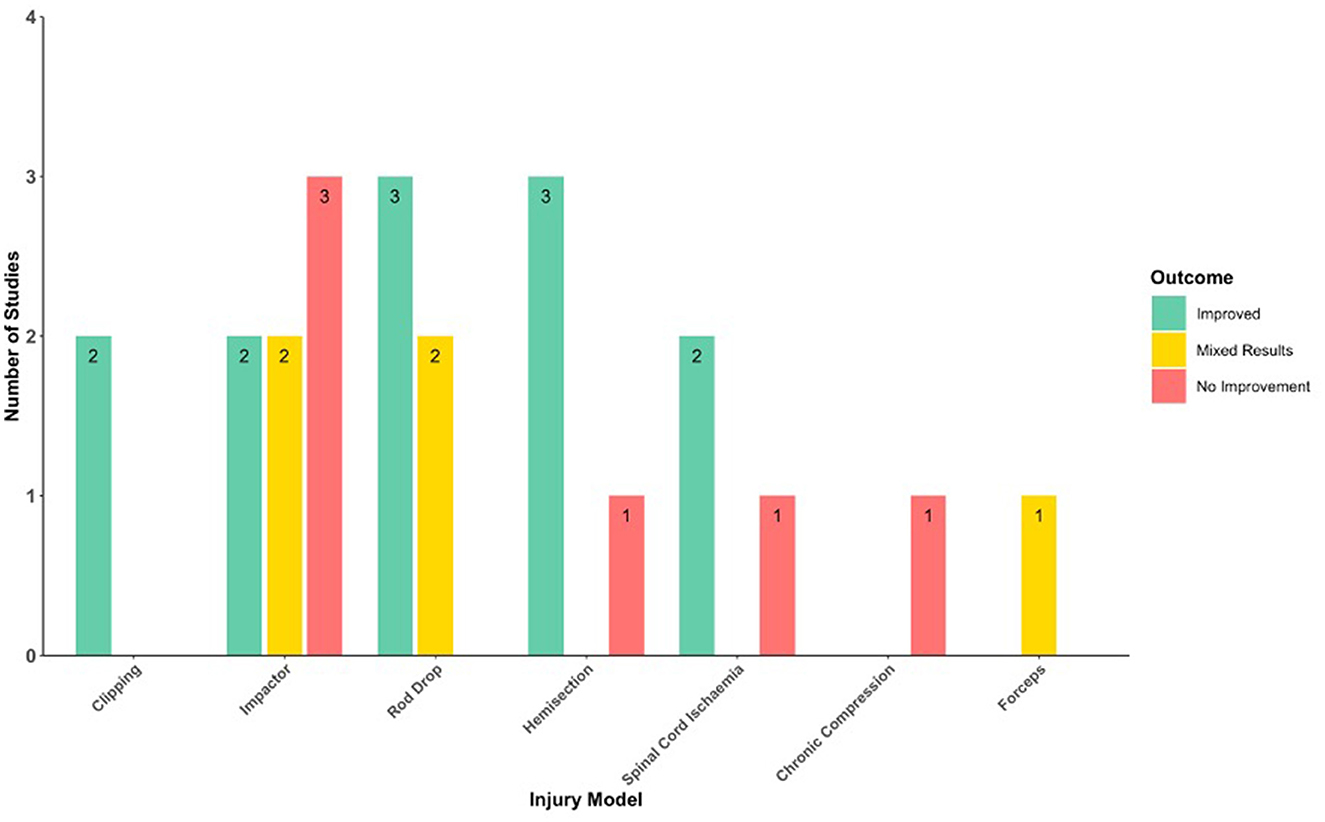
Figure 2. Histogram of the neurobehavioral outcomes following PDE inhibition in different injury models.
Risk of bias
The allocation sequence was only adequately generated and applied in 10 of 22 studies. The remaining studies may have been randomized but did not describe their allocation sequence. One study described random housing of animals (63). In total, nine studies stated that group neurobehavioral characteristics were similar to baseline (31, 44, 48, 49, 52, 60–63). No studies stated whether animals were randomly selected for assessment or whether group allocation was adequately concealed. The outcome assessor was blinded to treatment groups in 16 studies (31, 44, 45, 47, 48, 50, 51, 53–55, 57–59, 62–64). Comprehensive risk-of-bias assessment scores are provided in Supplementary material 3.
What is the impact of PDE inhibition on neurobehavioral outcomes?
The findings of each included study are summarized in Table 6.
Basso, Beattie, and Bresnahan (BBB) locomotor score
Of the 13 studies that assessed BBB scores, 11 involved rolipram-treated animals (31, 44–52, 55, 56, 62), one involved PDE4 inhibitor-treated animals (55), and one involved roflumilast-treated animals (56).
Of nine studies involving animals treated exclusively with rolipram, three found that rolipram-treated animals had significantly higher BBB scores than vehicle-treated animals (31, 45, 46). This was observed from 7 days post-SCI by Costa et al. (45), from 4 weeks post-SCI by Schaal et al. (31), and at 4 and 5 weeks post-SCI (but not at 6–8 weeks) by Pearse et al. (46). In total, six studies found that the BBB scores of animals treated with rolipram alone were not significantly different to vehicle treatment groups (44, 47, 48, 51, 52, 62); two studies assessing BBB score did not include a group treated solely with rolipram (49, 50).
In total, four studies found BBB scores were significantly higher than vehicle-treated animals when rolipram was combined with stem cells with cAMP (from 3 weeks post-SCI with rolipram delivered at the time of injury) (46); Nogo-66 receptor protein (at 49 days post-SCI) (47); methylprednisolone (from 3 weeks post-SCI) (52); thalidomide (at 7 and 42 days post-SCI) (48); and stem cells with a green fluorescent protein (from 2 weeks post-SCI) (50). In contrast, four studies found BBB scores were not significantly different to vehicle-treated animals when rolipram treatment combined with clodronate (51), Schwann cells (with or without cAMP) (62), and cAMP (with or without glial restricted precursor cells) (49).
Using PDE4-I, a selective PDE4 inhibitor, Bao et al. found treatment with 0.5 mg/kg improved BBB scores significantly from 4 to 8 weeks post-SCI (55). Moradi et al. found that treatment with 0.25, 0.5, and 1 mg/kg of roflumilast all improved the BBB score significantly compared with the vehicle 28 days post-SCI (12).
Basso, Beattie, and Bresnahan (BBB) locomotor subscore
In total, six studies assessed BBB subscore, all of which involved rolipram-treated rats; five studies involved animals treated exclusively with rolipram, two of which found animals treated with rolipram alone had significantly higher BBB scores than vehicle-treated animals. Significant benefit was observed from 1 week post-SCI by Iannoti et al. (51) and from 4 weeks post-SCI by Pearse et al. (with acute, not delayed rolipram administration) (46); In total, three studies found that the BBB subscores of animals treated exclusively with rolipram were not significantly different from vehicle treatment groups (31, 48, 62). One study assessing BBB subscore did not include a group treated solely with rolipram (50).
In total, four studies found BBB subscores were significantly higher than vehicle-treated animals when combining rolipram with clondronate (from 1 week post-SCI) (51), stem cells and cAMP (from 2 weeks post-SCI) (46), thalidomide (at 14, 21, and 42 days post-SCI) (48), and stem cells and green fluorescent protein (at 4 and 8–12 weeks post-SCI, or with GFP-D15A at 3–5 and 7–12 weeks post-SCI) (50). One study found BBB subscores were not significantly different than vehicle-treated animals when rolipram was combined with Schwann cells (with or without cAMP) (62).
Modified Tarlov score
Three studies used the modified Tarlov score in their assessments (58–60), each using a spinal cord ischemia injury model and cilostazol treatment. Nazli et al. studied rabbits and found that median Tarlov scores were significantly higher in the cilostazol group than in the ischemia–reperfusion-only group at all post-ischemia intervals (1–3 days) (58). In contrast, Sahin et al. reported that mean Tarlov scores in the cilostazol group were similar to the ischemia group (assessed at 2 days only, p = 0.08) in rats (59). Kurtoglu et al. studied rats and found that there was no significant difference in Tarlov scores between injured groups at any timepoint (60). In their study, sham group rats were subjected to laparotomy without aortic occlusion. Control group rats were pre-treated with intraperitoneal dimethyl sulfoxide while the cilostazol group rats received intraperitoneal cilostazol (20 mg/kg/day) for 3 days before the induction of ischemia. Ischemia was induced by clamping of the infrarenal aorta.
Grid walking
In total, seven studies assessed grid walk performance, all involving rolipram-treated rats (44, 46, 50, 52, 53, 61, 62). A regular grid was used in three studies (44, 53, 63), and an irregular grid was used in four studies (46, 50, 52, 62). Two studies found that rats treated exclusively with rolipram had significantly fewer footfall errors than vehicle-treated rats (44, 46). Beaumont et al. reported that rolipram-treated rats had a higher percentage of hindlimb steps without footfall errors, although no significant difference was found in the total number of steps or percentage of forelimb steps without footfall errors (44). Three studies found that in grid walk testing, there was no significant difference in the rates of footfall errors between rolipram- and vehicle-treated groups (52, 62, 63).
In total, five studies assessed grid walking after rolipram combined with other treatments (46, 50, 52, 53, 62); two of these studies did not include a group treated exclusively with rolipram (50, 53). These studies found that, when compared to vehicle-treated rats, grid walk footfall errors occurred at significantly lower rates when rolipram was combined with methylprednisolone (52); stem cells (with acute, not delayed rolipram administration, and with or without dc-cAMP) (46); D15A stem cells (50); and clodronate (with or without chondroitinase) (53). Sharp et al. found rats that received a combination of rolipram, Schwann cells, and db-cAMP had significantly fewer errors than the rolipram-treated group, but did not differ from the vehicle-treated group (62).
Vertical exploration/rearing
Three studies assessed vertical exploration behaviors (54, 63, 64). Dai et al. found that when rearing, there was no significant difference in right forelimb wall contacts between rolipram and vehicle-treated animals (63). Bretzner et al. found that there was no significant difference in forelimb usage between rolipram and vehicle-animal-treated animals in vertical exploration, though animals treated with a combination of rolipram and olfactory ensheathing cells demonstrated significantly greater usage of the injured forelimb (64). Nikulina et al. found that animals treated with embryonic spinal cord tissue and rolipram had significantly fewer incorrect (dorsal) forelimb contacts and raised the injured limb more frequently above the horizontal plane than animals receiving transplant alone (54).
Footprint and gait analysis
The footprint assessment method was variable, involving video-based kinematic analysis (62, 63), paint (62), or ink (50), or an unspecified technique (46). Multiple studies found no significant difference between animals treated exclusively with rolipram- and vehicle-treated animals when measuring foot exrotation (46, 62), base of support (46, 62, 63), or stride length (46, 62, 63). When rolipram was combined with stem cells and db-cAMP significantly less foot exrotation was observed (46), and when combined with D15A stem cells significantly improved base of support and stride length were observed (50). Compared with vehicle treatment, no significant difference in foot exrotation, base of support, or stride length was found when combining rolipram with Schwann cells, with or without cAMP (62).
Assessing gait using 3D video kinematic analysis, Costa et al. found significantly decreased hindlimb exrotation during the stance phase at initial contact in rolipram-treated animals (assessed at 8 weeks post-SCI) (45). Using CatWalk video analysis, Dai et al. identified no difference in standtime or swingtime when comparing rolipram and vehicle-treated animals (63).
Also using CatWalk video analysis, Koopmans et al. reported that neither gait coordination (quantified using the CatWalk regularity index) nor an integrated Catwalk-based BBB score was significantly different when comparing vehicle and rolipram-treated animals. Both metrics were significantly improved by 42 days post-SCI in rats treated with a combination of rolipram and thalidomide (compared with vehicle-treated rats) (48).
Reaching tasks
In total, two studies assessed animal reaching capabilities; there were no significant differences between rolipram and vehicle-treated animals in their ability to reach food through a small opening (with or without combination with db-cAMP and/or olfactory ensheathing cells) (64), or from vertical shelves (63).
Beam walking
In total, two studies included beam walking assessments (45, 62). Costa et al. reported that from 3 weeks post-SCI, rolipram-treated rats had significantly higher beam walk scores (i.e., fewer foot slips) than vehicle-treated rats (45). In ladder beam assessment, Sharp et al. found no significant difference in the number of hindlimb footfall errors between vehicle- and rolipram-treated mice (including mice treated with a combination of rolipram and Schwann cells, with or without cAMP) (62).
Allodynia
Bao et al. found mechanical allodynia elicited from hindpaws was significantly lower with PDE4 inhibitor treatment from 4 weeks post-SCI (55). Bretzner et al. reported that when applying thermal stimulation, the withdrawal latency in injured forepaws was not significantly different in rolipram-treated rats compared with vehicle-treated rats, but was significantly shorter in rats treated with rolipram and olfactory ensheathing cells from 4 weeks post-SCI (64).
Voluntary activity
Two studies assessed rates of voluntary activity (49, 61). No significant difference was found between rolipram-treated and vehicle-treated rats in voluntary movement inside the animal housing (with or without cAMP and/or glial restricted precursor cell transplant) (49), or between cilostazol-treated and vehicle-treated rats in voluntary exercise on a wheel inside the animal housing (61).
Additional measures
Yamamoto et al. found there was no significant difference in forced locomotor capability or forepaw grip strength between rats that received chronic compression and cilostazol treatment and rats that received a sham treatment. Forced locomotor capability and grip strength were significantly higher in sham-treated rats than those receiving chronic compression and vehicle treatment (61).
Downing et al. found, when compared to vehicle treatment, animals treated with low-dose rolipram patches had significantly higher Martinez forelimb open-field scores at 1–4, 6, and 8 weeks post-SCI, with significantly higher articular movement scores from 1 week post-SCI. There were no differences in scores between animals treated with high-dose rolipram and vehicle treatment. Animals treated with low-dose rolipram patches displayed the highest rates of coordinated forelimb–hindlimb behaviors of any group, while animals treated with high-dose rolipram patches displayed fewer coordinated behaviors than animals treated with unmedicated patches (57).
Discussion
The objective of this systematic review was to synthesize current literature evidence concerning the effect of PDE inhibitors on neurobehavioral outcomes in preclinical models of traumatic and non-traumatic SCI. Overall, PDE inhibitors were associated with statistically significant improvements in neurobehavioral outcomes in a majority of included studies. However, evidence was inconsistent with a high risk of bias, including inadequate or unreported allocation sequence and a lack of standardized methodologies.
Proposed mechanism of action
Mechanistic explanation for these results include rolipram antagonizing SCI-induced PDE4B1 and PDE4A5 production, PDE4A5 phosphorylation, and MCP-1 expression, reducing immune cell infiltration and preventing post-injury reduction in IL-10 (31). Furthermore, Bao et al. have demonstrated that the PDE4 inhibitor PDE4-I has anti-inflammatory and anti-oxidative effects, antagonizing free radical production, and reducing expression of nitric oxide synthase and cyclooxygenase (55). In addition, Moradi et al. suggest that the PDE4 inhibitor roflumilast increases the polarization of macrophages toward the anti-inflammatory M2 phenotype, resulting in increased IL-10 and decreased TNF-α production (56).
Neuroprotective effects have also been demonstrated by Pearse et al., in rolipram increasing oligodendrocyte survival in an acute SCI model (46). Similarly, Beaumont et al. have shown that rolipram significantly increases oligodendrocyte survival in the ventrolateral funiculus (VLF) of the spinal cord following acute SCI, with improved VLF conductivity and significantly fewer footfalls in grid walk testing (44).
Additional mechanistic insights are available from studies of combination therapies. Iannotti et al. demonstrated that administering rolipram with clodronate significantly increased axonal sparing and BBB locomotor scores (51). In addition, Koopmans et al. found that administering rolipram with thalidomide increased white matter sparing at the SCI lesion center and significantly increased BBB locomotor scores (48). Nikulina et al. demonstrated that the addition of rolipram to a post-SCI embryonic stem cell transplant improved axonal growth into the transplant post-SCI (54). A single study using PDE4-I found significantly higher BBB scores than in vehicle-treated animals, a finding comparable to that of studies using rolipram (55). Similarly, a single study using roflumilast found BBB scores to be significantly improved compared with vehicle-treated animals (56).
Evaluation of current methodologies and future perspectives
Significant heterogeneity exists between included studies. As a result, analysis of numerical effect estimates beyond study characteristics was not possible, and this review represents a qualitative synthesis of the literature. We have identified three key aspects within the methodologies of included studies that differed substantially: (1) the model of SCI utilized, (2) the intervention itself, including the PDE inhibitors that were delivered and the dosing-regimen, and (3) the neurobehavioral outcomes used to assess the efficacy of PDE inhibition in traumatic and non-traumatic SCI models.
Over the last 25 years, animal SCI models have become increasingly diverse. A range of injury mechanisms are now utilized, such as spinal cord contusion, compression, and transection (74). Differences exist even within individual SCI models; for example, spinal cord contusion may be induced using various types of impactor (75–78). As a result, the specific pattern of SCI induced, and subsequent pathophysiology, differs between the various models of SCI. This may in part explain inconsistencies between the results of included studies (79). No single model can replicate SCI in humans (80); researchers must therefore select an SCI model most suited to their research question. Most included studies that provided a rationale for SCI model choice, however, lacked detailed reporting of how the chosen model was implemented. This leads to difficulties in the replication of results (46, 62). A recent systematic review evaluating animal SCI models in the field of biomaterials similarly identified that poor reporting of methods and results had negatively impacted reproducibility in later studies (81). Comprehensive reporting of methodology in future studies would therefore aid result replication.
Significant variation between studies was also seen in the type of PDE inhibitor chosen and the mechanism of delivery. Currently, the relative merits of PDE inhibitors rolipram and cilostazol in the SCI context cannot be directly compared, as there is no overlap in the injury model or outcome measures for these two drugs in studies to date. Furthermore, in studies using the same PDE inhibitor, direct comparisons are hindered by variation in the route of administration and dosing regimens. It is also important to recognize the impact of differences in age, species and strain of animals. Not only would these factors have a significant impact on the pharmacodynamics of PDE inhibitors (82, 83), but they would also impact the pharmacokinetics, with differences in, for example, capillary permeability and local blood flow affecting drug absorption (84).
To aid clinical translation, it is important that routes of administration and dosing regimens amenable to the management of SCI in humans are considered when devising future experimental protocols. PDE inhibitor pharmacokinetic properties, including absorption, distribution, excretion, and metabolism, should also assessed (85). For example, rolipram readily crosses the blood–brain barrier (86), which is clinically advantageous in terms of being able to deliver the drug subcutaneously, whereas drugs that require a direct introduction to the site of injury may be less clinically translatable (54).
Finally, there was significant variation in the neurobehavioral outcomes used across included studies. Similar to models of SCI, each neurobehavioral outcome has its advantages and limitations; no single measurement can wholly assess the efficacy of PDE inhibitors in the context of SCI. For example, while the BBB score provides a simple and popular method to measure locomotion (87), identification of more subtle changes in motor recovery necessitates more intricate measures, such as 3D hindlimb kinematics (45). The most rigorous studies used a combination of neurobehavioral outcomes. Importantly, while our review focuses on neurobehavioral outcomes as a measure of PDE inhibitor efficacy, this is just one measure of efficacy. Other measures include immunohistochemistry, imaging, and neurophysiological parameters (79). While assessment of other forms of outcomes was beyond the scope of this review, it is important to acknowledge that the most robust studies assessed the efficacy of PDE inhibitors across multiple different outcome domains.
Strengths and limitations
This review is the first to synthesize the impact of phosphodiesterase inhibition on neurobehavioral outcomes in preclinical models of traumatic and non-traumatic SCI. The review involved an exhaustive systematic literature search, a robust methodology that adheres to PRISMA guidelines and includes a robust risk of bias assessment using the SYRCLE tool.
Despite significant results in a majority of included studies, there was significant inconsistency in findings between studies. This may be explained by the diversity of interventions, with a range of injury techniques and dosing parameters used. Moreover, comparison between studies is limited by a lack of uniformity in the domains, methods, and timings of neurobehavioral assessment. In addition, while a majority of included studies reported positive results this may well not reflect a majority of studies conducted due to underreporting of negative results.
This review has utility in raising awareness of this heterogeneity; standardization of laboratory protocols used in future studies will improve interpretability and aid future synthesis. In addition, this study provides the fundamental preclinical background to clinical trials of phosphodiesterase inhibition in spinal cord injury, including the RECEDE-Myelopathy trial, which is currently evaluating the PDE4 inhibitor ibudilast in patients with degenerative cervical myelopathy (39).
Conclusion
In preclinical models of traumatic and non-traumatic SCI, the exclusive administration of PDE inhibitors such as rolipram and cilostazol appeared to be associated with statistically significant improvements in neurobehavioral outcomes in a majority of included studies. However, evidence was inconsistent with a high risk of bias. Therefore, further evaluation of PDE inhibitors is required in the context of spinal cord injury to establish evidence of a repeatable and meaningful effect.
Data availability statement
The original contributions presented in the study are included in the article/Supplementary material, further inquiries can be directed to the corresponding author.
Author contributions
MB, OM, and BD: conceptualization, design, search strategy, and data interpretation. MB, OM, AB, UW, SR, TO, BW, LO, GS, IK, SV, RD, FB, F-E-MS, ARF, JT, BG, MA, and SA: screening. MB: data extraction and manuscript drafting. All authors: manuscript review. All authors contributed to the article and approved the submitted version.
Funding
MK was funded by an NIHR Clinician Scientist Award CS-2015-15-023. BD was supported by an NIHR Clinical Doctoral Research Fellowship. OM was supported by an NIHR Academic Clinical Fellowship.
Acknowledgments
We gratefully acknowledge support from the Cambridge NIHR Brain Injury MedTech Cooperative.
Conflict of interest
The authors declare that the research was conducted in the absence of any commercial or financial relationships that could be construed as a potential conflict of interest.
Publisher's note
All claims expressed in this article are solely those of the authors and do not necessarily represent those of their affiliated organizations, or those of the publisher, the editors and the reviewers. Any product that may be evaluated in this article, or claim that may be made by its manufacturer, is not guaranteed or endorsed by the publisher.
Author disclaimer
This report is independent research arising from a Clinician Scientist Award, CS-2015-15-023, supported by the National Institute for Health Research. The views expressed in this publication are those of the authors and not necessarily those of the NHS, the National Institute for Health Research, or the Department of Health.
Supplementary material
The Supplementary Material for this article can be found online at: https://www.frontiersin.org/articles/10.3389/fmed.2023.1237219/full#supplementary-material
References
1. Singh A, Tetreault L, Kalsi-Ryan S, Nouri A, Fehlings MG. Global prevalence and incidence of traumatic spinal cord injury. Clin Epidemiol. (2014) 6:309–31. doi: 10.2147/CLEP.S68889
2. Sekhon LH, Fehlings MG. Epidemiology, demographics, and pathophysiology of acute spinal cord injury. Spine. (1976).
3. Profyris C, Cheema SS, Zang D, Azari MF, Boyle K, Petratos S. Degenerative and regenerative mechanisms governing spinal cord injury. Neurobiol Dis. (2004) 15:415–36. doi: 10.1016/j.nbd.2003.11.015
4. Ahuja CS, Nori S, Tetreault L, Wilson J, Kwon B, Harrop J, et al. Traumatic spinal cord injury. Nat Rev Dis Primers. (2017) 3:17018. doi: 10.1038/nrdp.2017.18
5. Badhiwala JH, Ahuja CS, Akbar MA, Witiw CD, Nassiri F, Furlan JC, et al. Degenerative cervical myelopathy - update and future directions. Nat Rev Neurol. (2020) 16:108–24. doi: 10.1038/s41582-019-0303-0
6. Wilson JR, Tetreault LA, Kim J, Shamji MF, Harrop JS, Mroz T, et al. State of the art in degenerative cervical myelopathy: an update on current clinical evidence. Neurosurgery. (2017) 80:S33–45. doi: 10.1093/neuros/nyw083
7. Nouri, A, Tetreault, L, Côté, P, Zamorano JJ, Dalzell, K, Fehlings MG. Does magnetic resonance imaging improve the predictive performance of a validated clinical prediction rule developed to evaluate surgical outcome in patients with degenerative cervical myelopathy? Spine. (2015) 40:1092–100. doi: 10.1097/BRS.0000000000000919
8. Park E, Velumian AA, Fehlings MG. The role of excitotoxicity in secondary mechanisms of spinal cord injury: a review with an emphasis on the implications for white matter degeneration. J Neurotrauma. (2004) 21:754–74. doi: 10.1089/0897715041269641
9. Dietrich WD, Chatzipanteli K, Vitarbo E, Wada K, Kinoshita K. The role of inflammatory processes in the pathophysiology and treatment of brain and spinal cord trauma. Acta Neurochir Suppl. (2004) 89:69–74. doi: 10.1007/978-3-7091-0603-7_9
10. Nikoletopoulou V, Tavernarakis N. Calcium homeostasis in aging neurons. Front Gene. (2012) 3:200. doi: 10.3389/fgene.2012.00200
11. Tibbo AJ, Baillie GS. Phosphodiesterase 4B: master regulator of brain signaling. Cells. (2020) 9:1254. doi: 10.3390/cells9051254
12. Schafer PH, Parton A, Capone L. Apremilast is a selective PDE4 inhibitor with regulatory effects on innate immunity. Cell Signal. (2014) 26:2016–29. doi: 10.1016/j.cellsig.2014.05.014
13. Hervé R, Schmitz T, Evain-Brion D, Cabrol D, Leroy M-J, Méhats C. The PDE4 inhibitor rolipram prevents NF-kappaB binding activity and proinflammatory cytokine release in human chorionic cells. J Immunol. (2008) 181:2196–202. doi: 10.4049/jimmunol.181.3.2196
14. Hannila SS, Filbin MT. The role of cyclic AMP signaling in promoting axonal regeneration after spinal cord injury. Exp Neurol. (2008) 209:321–32. doi: 10.1016/j.expneurol.2007.06.020
15. Iona S, Cuomo M, Bushnik T. Characterization of the rolipram-sensitive, cyclic AMP-specific phosphodiesterases: identification and differential expression of immunologically distinct forms in the rat brain. Mol Pharmacol. (1998) 53:23–32. doi: 10.1124/mol.53.1.23
16. Verghese MW, McConnell RT, Lenhard JM, Hamacher L, Jin SL. Regulation of distinct cyclic AMP-specific phosphodiesterase (phosphodiesterase type 4) isozymes in human monocytic cells. Mol Pharmacol. (1995) 47:1164–71.
17. Li H, Zuo J, Tang W. Phosphodiesterase-4 inhibitors for the treatment of inflammatory diseases. Front Pharmacol. (2018) 9:1048. doi: 10.3389/fphar.2018.01048
18. Syed YA, Baer A, Hofer MP, González GA, Rundle J, Myrta S, et al. Inhibition of phosphodiesterase-4 promotes oligodendrocyte precursor cell differentiation and enhances CNS remyelination. EMBO Mol Med. (2013) 5:1918–34. doi: 10.1002/emmm.201303123
19. Chen R-W, Williams AJ, Liao Z, Yao C, Tortella FC, Dave JR. Broad spectrum neuroprotection profile of phosphodiesterase inhibitors as related to modulation of cell-cycle elements and caspase-3 activation. Neurosci Lett. (2007) 418:165–9. doi: 10.1016/j.neulet.2007.03.033
20. Griswold DE, Webb EF, Badger AM. SB 207499 (Ariflo), a second generation phosphodiesterase 4 inhibitor, reduces tumor necrosis factor alpha and interleukin-4 production in vivo. J Pharmacol Exp Ther. (1998) 287:705–11.
21. Sommer N, Martin R, McFarland HF. Therapeutic potential of phosphodiesterase type 4 inhibition in chronic autoimmune demyelinating disease. J Neuroimmunol. (1997) 79:54–61. doi: 10.1016/S0165-5728(97)00111-2
22. Yoshikawa M, Suzumura A, Tamaru T, Takayanagi T, Sawada M. Effects of phosphodiesterase inhibitors on cytokine production by microglia. Mult Scler. (1999) 5:126–33. doi: 10.1177/135245859900500210
23. Beshay E, Croze F, Prud'homme GJ. The phosphodiesterase inhibitors pentoxifylline and rolipram suppress macrophage activation and nitric oxide production in vitro and in vivo. Clin Immunol. (2001) 98:272–9. doi: 10.1006/clim.2000.4964
24. Block F, Schmidt W, Nolden-Koch M, Schwarz M. Rolipram reduces excitotoxic neuronal damage. Neuroreport. (2001) 12:1507–11. doi: 10.1097/00001756-200105250-00041
25. Bandtlow CE. Regeneration in the central nervous system. Exp Gerontol. (2003) 38:79–86. doi: 10.1016/S0531-5565(02)00165-1
26. Torphy TJ, Undem BJ. Phosphodiesterase inhibitors: new opportunities for the treatment of asthma. Thorax. (1991) 46:512–23. doi: 10.1136/thx.46.7.512
27. Francischi JN, Yokoro CM, Poole S, Tafuri WL, Cunha FQ, Teixeira MM. Anti-inflammatory and analgesic effects of the phosphodiesterase 4 inhibitor rolipram in a rat model of arthritis. Eur J Pharmacol. (2000) 399:243–9. doi: 10.1016/S0014-2999(00)00330-7
28. DeMarch Z, Giampà C, Patassini S, Martorana A, Bernardi G, Fusco FR. Beneficial effects of rolipram in a quinolinic acid model of striatal excitotoxicity. Neurobiol Dis. (2007) 25:266–73. doi: 10.1016/j.nbd.2006.09.006
29. Gong B, Vitolo OV, Trinchese F, Liu S, Shelanski M, Arancio O. Persistent improvement in synaptic and cognitive functions in an Alzheimer mouse model after rolipram treatment. J Clin Invest. (2004) 114:1624–34. doi: 10.1172/JCI22831
30. Navarro J, Punzon C, Jimenez JL. Inhibition of phosphodiesterase type IV suppresses human immunodeficiency virus type 1 replication and cytokine production in primary T cells: involvement of NF-kappaB and NFAT. J Virol. (1998) 72:4712–20. doi: 10.1128/JVI.72.6.4712-4720.1998
31. Schaal SM, Garg MS, Ghosh M. The therapeutic profile of rolipram, PDE target and mechanism of action as a neuroprotectant following spinal cord injury. PLoS ONE. (2012) 7:43634. doi: 10.1371/journal.pone.0043634
32. Atkins CM, Alonso OF, Pearse DD, Bramlett HM, Dietrich WD. Modulation of the cAMP signaling pathway after traumatic brain injury. Exp Neurol. (2007) 208:145–58. doi: 10.1016/j.expneurol.2007.08.011
33. Watanabe T, Zhang N, Liu M, Tanaka R, Mizuno Y, Urabe T. Cilostazol protects against brain white matter damage and cognitive impairment in a rat model of chronic cerebral hypoperfusion. Stroke. (2006) 37:1539–45. doi: 10.1161/01.STR.0000221783.08037.a9
34. Lee JH, Lee YK, Ishikawa M. Cilostazol reduces brain lesion induced by focal cerebral ischemia in rats–an MRI study. Brain Res. (2003) 994:91–8. doi: 10.1016/j.brainres.2003.09.021
35. Choi JM, Shin HK, Kim KY, Lee JH, Hong KW. Neuroprotective effect of cilostazol against focal cerebral ischemia via antiapoptotic action in rats. J Pharmacol Exp Ther. (2002) 300:787–93. doi: 10.1124/jpet.300.3.787
36. Iwama D, Miyamoto K, Miyahara S. Neuroprotective effect of cilostazol against retinal ischemic damage via inhibition of leukocyte-endothelial cell interactions. J Thromb Haemost. (2007) 5:818–25. doi: 10.1111/j.1538-7836.2007.02425.x
37. Uehara S, Hirayama A. Effects of cilostazol on platelet function. Arzneimittelforschung. (1989) 39:1531–4.
38. Fong M, Yoshitake M, Kambayashi J, Liu Y. Cilostazol increases tissue blood flow in contracting rabbit gastrocnemius muscle. Circ J. (2010) 74:181–7. doi: 10.1253/circj.CJ-09-0372
39. Davies B, Mowforth OD, Yordanov S, Alvarez-Berdugo D, Bond S, Nodale M, et al. Targeting patient recovery priorities in degenerative cervical myelopathy: design and rationale for the RECEDE-Myelopathy trial—study protocol. Br Med J Open. (2023) 13:e061294. doi: 10.1136/bmjopen-2022-061294
40. Moher D, Liberati A, Tetzlaff J, Altman DG, Group P. Preferred reporting items for systematic reviews and meta-analyses: the PRISMA statement. PLoS Med. (2009) 6:1000097. doi: 10.1371/journal.pmed.1000097
41. Campbell M, McKenzie JE, Sowden A, Katikireddi SV, Brennan SE, Ellis S, et al. Synthesis without meta-analysis (SWiM) in systematic reviews: reporting guideline. Br Med J. (2020) 2020:l6890. doi: 10.1136/bmj.l6890
42. Higgins JP, Altman DG, Gotzsche PC. The Cochrane Collaboration's tool for assessing risk of bias in randomised trials. Br Med J. (2011) 343:d5928. doi: 10.1136/bmj.d5928
43. Hooijmans CR, Rovers MM, de Vries RBM, Leenaars M, Ritskes-Hoitinga M, Lagendam MW. SYRCLE's risk of bias tool for animal studies. BMC Med Res Methodol. (2014) 14:43. doi: 10.1186/1471-2288-14-43
44. Beaumont E, Whitaker CM, Burke DA, Hetman M, Onifer SM. Effects of rolipram on adult rat oligodendrocytes and functional recovery after contusive cervical spinal cord injury. Neuroscience. (2009) 163:985–90. doi: 10.1016/j.neuroscience.2009.07.039
45. Costa LM, Pereira JE, Filipe VM. Rolipram promotes functional recovery after contusive thoracic spinal cord injury in rats. Behav Brain Res. (2013) 243:66–73. doi: 10.1016/j.bbr.2012.12.056
46. Pearse DD, Pereira FC, Marcillo AE. cAMP and Schwann cells promote axonal growth and functional recovery after spinal cord injury. Nat Med. (2004) 10:610–6. doi: 10.1038/nm1056
47. Wang X, Baughman KW, Basso DM, Strittmatter SM. Delayed Nogo receptor therapy improves recovery from spinal cord contusion. Ann Neurol. (2006) 60:540–9. doi: 10.1002/ana.20953
48. Koopmans GC, Deumens R, Buss A. Acute rolipram/thalidomide treatment improves tissue sparing and locomotion after experimental spinal cord injury. Exp Neurol. (2009) 216:490–8. doi: 10.1016/j.expneurol.2009.01.005
49. Nout YS, Culp E, Schmidt MH. Glial restricted precursor cell transplant with cyclic adenosine monophosphate improved some autonomic functions but resulted in a reduced graft size after spinal cord contusion injury in rats. Exp Neurol. (2011) 227:159–71. doi: 10.1016/j.expneurol.2010.10.011
50. Flora G, Joseph G, Patel S. Combining neurotrophin-transduced schwann cells and rolipram to promote functional recovery from subacute spinal cord injury. Cell Transplant. (2013) 22:2203–17. doi: 10.3727/096368912X658872
51. Iannotti CA, Clark M, Horn KP, van Rooijen N, Silver J, Steinmetz MP. A combination immunomodulatory treatment promotes neuroprotection and locomotor recovery after contusion SCI. Exp Neurol. (2011) 230:3–15. doi: 10.1016/j.expneurol.2010.03.010
52. Yin Y, Sun W, Li Z. Effects of combining methylprednisolone with rolipram on functional recovery in adult rats following spinal cord injury. Neurochem Int. (2013) 62:903–12. doi: 10.1016/j.neuint.2013.03.005
53. Grosso MJ, Matheus V, Clark M, van Rooijen N, Iannotti CA, Steinmetz MP. Effects of an immunomodulatory therapy and chondroitinase after spinal cord hemisection injury. Neurosurgery. (2014) 75:461–71. doi: 10.1227/NEU.0000000000000447
54. Nikulina E, Tidwell JL, Dai HN, Bregman BS, Filbin MT. The phosphodiesterase inhibitor rolipram delivered after a spinal cord lesion promotes axonal regeneration and functional recovery. Proc Natl Acad Sci USA. (2004) 101:8786–90. doi: 10.1073/pnas.0402595101
55. Bao F, Fleming JC, Golshani R. A selective phosphodiesterase-4 inhibitor reduces leukocyte infiltration, oxidative processes, and tissue damage after spinal cord injury. J Neurotrauma. (2011) 28:1035–49. doi: 10.1089/neu.2010.1575
56. Moradi K, Golbakhsh M, Haghighi F, Afshari K, Nikbakhsh R, Khavandi MM, et al. Inhibition of phosphodiesterase IV enzyme improves locomotor and sensory complications of spinal cord injury via altering microglial activity: introduction of Roflumilast as an alternative therapy. Int Immunopharmacol. (2020) 86:106743. doi: 10.1016/j.intimp.2020.106743
57. Downing TL, Wang A, Yan ZQ. Drug-eluting microfibrous patches for the local delivery of rolipram in spinal cord repair. J Control Release. (2012) 161:910–7. doi: 10.1016/j.jconrel.2012.05.034
58. Nazli Y, Colak N, Namuslu M. Cilostazol attenuates spinal cord ischemia-reperfusion injury in rabbits. J Cardiothorac Vasc Anesth. (2015) 29:351–9. doi: 10.1053/j.jvca.2014.06.028
59. Sahin MA, Onan B, Guler A. Cilostazol, a type III phosphodiesterase inhibitor, reduces ischemia/reperfusion-induced spinal cord injury. Heart Surg Forum. (2011) 14:1126. doi: 10.1532/HSF98.20101126
60. Kurtoglu T, Basoglu H, Ozkisacik EA. Effects of cilostazol on oxidative stress, systemic cytokine release, and spinal cord injury in a rat model of transient aortic occlusion. Ann Vasc Surg. (2014) 28:479–88. doi: 10.1016/j.avsg.2013.08.005
61. Yamamoto S, Kurokawa R, Kim P. Cilostazol, a selective Type III phosphodiesterase inhibitor: prevention of cervical myelopathy in a rat chronic compression model. J Neurosurg Spine. (2014) 20:93–101. doi: 10.3171/2013.9.SPINE121136
62. Sharp KG, Flanagan LA, Yee KM, Steward O. A re-assessment of a combinatorial treatment involving Schwann cell transplants and elevation of cyclic AMP on recovery of motor function following thoracic spinal cord injury in rats. Exp Neurol. (2012) 233:625–44. doi: 10.1016/j.expneurol.2010.12.020
63. Dai H, MacArthur L, McAtee M. Activity-based therapies to promote forelimb use after a cervical spinal cord injury. J Neurotrauma. (2009) 26:1719–32. doi: 10.1089/neu.2008.0592
64. Bretzner F, Plemel JR, Liu J, Richter M, Roskams AJ, Tetzlaff W. Combination of olfactory ensheathing cells with local vs. systemic cAMP treatment after a cervical rubrospinal tract injury. J Neurosci Res. (2010) 88:2833–46. doi: 10.1002/jnr.22440
65. Xu RX, Rocque WJ, Lambert MH, Vanderwall DE, Luther MA, Nolte RT. Crystal structures of the catalytic domain of phosphodiesterase 4B complexed with AMP, 8-Br-AMP, and Rolipram. J Mol Biol. (2004) 337:355–65. doi: 10.1016/j.jmb.2004.01.040
66. Lehenkari PP, Kellinsalmi M, Näpänkangas JP, Ylitalo KV, Mönkkönen J, Rogers MJ, et al. Further insight into mechanism of action of clodronate: inhibition of mitochondrial ADP/ATP translocase by a nonhydrolyzable, adenine-containing metabolite. Mol Pharmacol. (2002) 61:1255–62. doi: 10.1124/mol.61.5.1255
67. Hatzelmann A, Morcillo EJ, Lungarella G, Adnot S, Sanjar S, Beume R, et al. The preclinical pharmacology of roflumilast—a selective, oral phosphodiesterase 4 inhibitor in development for chronic obstructive pulmonary disease. Pulmonary Pharmacol Therapeut. (2010) 23:235–56. doi: 10.1016/j.pupt.2010.03.011
68. Mahajan R. Chondroitinase ABC enzyme: a potential treatment option for spinal cord injury. Int J App Basic Med Res. (2018) 8:203. doi: 10.4103/ijabmr.IJABMR_336_18
69. Zhao RR, Fawcett JW. Combination treatment with chondroitinase ABC in spinal cord injury—breaking the barrier. Neurosci Bull. (2013) 29:477–83. doi: 10.1007/s12264-013-1359-2
70. Sorkin EM, Markham A. Cilostazol. Drugs Aging. (1999) 14:63–71. doi: 10.2165/00002512-199914010-00005
71. Movsesian M, Ahmad F, Hirsch E. Functions of PDE3 Isoforms in Cardiac Muscle. JCDD. (2018) 5:10. doi: 10.3390/jcdd5010010
72. Sloka JS, Stefanelli M. The mechanism of action of methylprednisolone in the treatment of multiple sclerosis. Mult Scler. (2005) 11:425–32. doi: 10.1191/1352458505ms1190oa
73. Anderson KC. Lenalidomide and thalidomide: mechanisms of action—Similarities and differences. Semin Hematol. (2005) 42:S3–8. doi: 10.1053/j.seminhematol.2005.10.001
74. Cheriyan T, Ryan DJ, Weinrab JH, Cheriyan J, Paul JC, Lafage V, et al. Spinal cord injury models: a review. Spinal Cord. (2014) 52:588–95. doi: 10.1038/sc.2014.91
75. Gruner JAA. Monitored contusion model of spinal cord injury in the rat. J Neurotrauma. (1992) 9:123–8. doi: 10.1089/neu.1992.9.123
76. Scheff SW, Rabchevsky AG, Fugaccia I, Main JA, Lumpp JE. Experimental modeling of spinal cord injury: characterization of a force-defined injury device. J Neurotrauma. (2003) 20:179–93. doi: 10.1089/08977150360547099
77. Stokes BT. Experimental spinal cord injury: a dynamic and verifiable injury device. J Neurotrauma. (1992) 9:129–34. doi: 10.1089/neu.1992.9.129
78. Marcol W, Slusarczyk W, Gzik M, Larysz-Brysz M, Bobrowski M, Grynkiewicz-Bylina B, et al. Air gun impactor—A novel model of graded white matter spinal cord injury in rodents. J Reconstr Microsurg. (2012) 28:561–8. doi: 10.1055/s-0032-1315779
79. Sharif-Alhoseini M, Khormali M, Rezaei M, Safdarian M, Hajighadery A, Khalatbari MM, et al. Animal models of spinal cord injury: a systematic review. Spinal Cord. (2017) 55:714–21. doi: 10.1038/sc.2016.187
80. Zhang N, Fang M, Chen H, Gou F, Ding M. Evaluation of spinal cord injury animal models. Neural Regen Res. (2014) 9:2008. doi: 10.4103/1673-5374.143436
81. Verstappen K, Aquarius R, Klymov A, Wever KE, Damveld L, Leeuwenburgh SCG, et al. Systematic evaluation of spinal cord injury animal models in the field of biomaterials. Tissue Eng B. (2022) 28:1169–79. doi: 10.1089/ten.teb.2021.0194
82. Mangoni AA, Jackson SHD. Age-related changes in pharmacokinetics and pharmacodynamics: basic principles and practical applications: Age-related changes in pharmacokinetics and pharmacodynamics. Br J Clin Pharmacol. (2003) 57:6–14. doi: 10.1046/j.1365-2125.2003.02007.x
83. Toutain PL, Ferran A, Bousquet-Mélou A. Species differences in pharmacokinetics and pharmacodynamics. In:F Cunningham, J Elliott, P Lees, , editors, Comparative and Veterinary Pharmacology. Berlin; Heidelberg: Springer (2010). p. 19–48.
84. Martinez MN. Factors influencing the use and interpretation of animal models in the development of parenteral drug delivery systems. AAPS J. (2011) 13:632–49. doi: 10.1208/s12248-011-9303-8
85. Reichel A, Lienau P. Pharmacokinetics in drug discovery: an exposure-centred approach to optimising and predicting drug efficacy and safety. In:U Nielsch, U Fuhrmann, S Jaroch, , editors, New Approaches to Drug Discovery. Berlin: Springer International Publishing (2015). p. 235–60.
86. Krause W, KÜhne G. Pharmacokinetics of rolipram in the rhesus and cynomolgus monkeys, the rat and the rabbit. Studies on species differences. Xenobiotica. (1988) 18:561–71. doi: 10.3109/00498258809041693
Keywords: spinal cord, spinal cord injury, phosphodiesterase inhibitor, preclinical model, neurobehavioral outcomes
Citation: Butler MB, Vellaiyappan SK, Bhatti F, Syed F-E-M, Rafati Fard A, Teh JQ, Grodzinski B, Akhbari M, Adeeko S, Dilworth R, Bhatti A, Waheed U, Robinson S, Osunronbi T, Walker B, Ottewell L, Suresh G, Kuhn I, Davies BM, Kotter MRN and Mowforth OD (2023) The impact of phosphodiesterase inhibition on neurobehavioral outcomes in preclinical models of traumatic and non-traumatic spinal cord injury: a systematic review. Front. Med. 10:1237219. doi: 10.3389/fmed.2023.1237219
Received: 09 June 2023; Accepted: 31 July 2023;
Published: 22 August 2023.
Edited by:
Heping Yang, Cedars Sinai Medical Center, United StatesReviewed by:
Andrew Blight, Acorda Therapeutics, United StatesBing Yang, Cedars Sinai Medical Center, United States
Copyright © 2023 Butler, Vellaiyappan, Bhatti, Syed, Rafati Fard, Teh, Grodzinski, Akhbari, Adeeko, Dilworth, Bhatti, Waheed, Robinson, Osunronbi, Walker, Ottewell, Suresh, Kuhn, Davies, Kotter and Mowforth. This is an open-access article distributed under the terms of the Creative Commons Attribution License (CC BY). The use, distribution or reproduction in other forums is permitted, provided the original author(s) and the copyright owner(s) are credited and that the original publication in this journal is cited, in accordance with accepted academic practice. No use, distribution or reproduction is permitted which does not comply with these terms.
*Correspondence: Benjamin M. Davies, YmVuamFtaW4uZGF2aWVzNCYjeDAwMDQwO25ocy5uZXQ=
†These authors share first authorship
‡ORCID: Amir Rafati Fard orcid.org/0000-0003-1804-2586