- 1Institute of Orthopaedics and Traumatology, The First Affiliated Hospital of Zhejiang Chinese Medical University (Zhejiang Provincial Hospital of Traditional Chinese Medicine), Hangzhou, China
- 2Department of Pharmacy, The Second Affiliated Hospital, Zhejiang University School of Medicine, Hangzhou, China
- 3Hangzhou Fuyang Hospital of TCM Orthopedics and Traumatology, Hangzhou, China
- 4Guangdong Provincial Hospital of Chinese Medicine, Guangzhou University of Chinese Medicine, Guangzhou, China
Osteoporosis (OP) is a systemic skeletal disease prevalent in older adults, characterized by substantial bone loss and deterioration of microstructure, resulting in heightened bone fragility and risk of fracture. Traditional Chinese Medicine (TCM) herbs have been widely employed in OP treatment owing to their advantages, such as good tolerance, low toxicity, high efficiency, and minimal adverse reactions. Increasing evidence also reveals that many plant-based compounds (or secondary metabolites) from these TCM formulas, such as resveratrol, naringin, and ginsenoside, have demonstrated beneficial effects in reducing the risk of OP. Nonetheless, the comprehensive roles of these natural products in OP have not been thoroughly clarified, impeding the development of synergistic formulas for optimal OP treatment. In this review, we sum up the pathological mechanisms of OP based on evidence from basic and clinical research; emphasis is placed on the in vitro and preclinical in vivo evidence-based anti-OP mechanisms of TCM formulas and their chemically active plant constituents, especially their effects on imbalanced bone homeostasis regulated by osteoblasts (responsible for bone formation), osteoclasts (responsible for bone resorption), bone marrow mesenchymal stem cells as well as bone microstructure, angiogenesis, and immune system. Furthermore, we prospectively discuss the combinatory ingredients from natural products from these TCM formulas. Our goal is to improve comprehension of the pharmacological mechanisms of TCM formulas and their chemically active constituents, which could inform the development of new strategies for managing OP.
Introduction
Osteoporosis (OP) as a chronic systemic skeletal disease, is considered to be a growing silent epidemic in the 21st century, and it is estimated to affect approximately 200 million individuals worldwide (1, 2). It is characterized by a decline in bone mineral density (BMD) and degradation of bone tissue microstructure, which results in heightened bone fragility and a higher risk of fractures (3). Several factors, including hormonal imbalances, medication use (like glucocorticoids), smoking, lack of exercise, and insufficient calcium and vitamin D intake, contribute to bone loss in OP progression (4, 5). Epidemiology research has shown that 1/3 of women and 1/5 of men over the age of 50 are prone to osteoporotic fractures, with the risk increasing with age, particularly in women over 60 (6). Current OP treatments vary from non-pharmacological options (such as muscle tone and balance-improving exercises) to pharmacological therapies (such as bisphosphonates, denosumab, teriparatide, abaloparatide, and romosozumab) (7–9). Certain patients with low BMD or those who respond poorly to treatment may still have extremely low BMD despite receiving bone-formation therapy. The safety risks connected with these therapeutic agents have resulted in investigations into safer and more effective alternatives for treating OP.
Traditional Chinese medicine (TCM) has been used for over 2000 years to manage a broad spectrum of medical diseases or conditions and relies on plant-based natural products, including TCM formulas and their compounds and extracts (10–12). These products have been pivotal in maintaining the health of Chinese individuals, providing effective treatments for diseases and reducing adverse effects, compared to Western medicine or placebo, for instance, 5-Ling Granule produced significantly greater improvement in the severity of tic symptoms than placebo in an 8 weeks, double-blind, randomized, controlled trial and Pingchuan Yiqi granule significantly improves lung function and symptoms of acute asthma in a randomized, double-blind, placebo-controlled trial (13–17). As to OP, in clinical practice and animal experiments, TCM formulas, such as Bushen Huoxue decoction (BSHXD), Er Xian Decoction (EXD), and Liuwei Dihuang Pill (LWDHP), have been proven to be effective in treating OP (18–20).
Meanwhile, various natural compounds, which are present in TCM formulas, have exhibited diverse biological effects in treating osteoarticular degenerative diseases, including OP. Many of these compounds have been proven to have effects akin to those of the original formulas for treating OP (21–26). Of note, chemically active ingredients from plant-based compounds (or secondary metabolites), like resveratrol, naringin, and ginsenoside, have been extensively employed for preventing and treating OP, as corroborated by their beneficial effects in decreasing bone resorption, increasing bone formation, repairing bone microstructure, enhancing angiogenesis, and improving the immune system (27–29). Nevertheless, the full extent of the roles played by these TCM herb formulas and their natural products in OP remains to be systematically elucidated, which hampers the development of synergistic formulas for more effective OP treatment.
Based on evidence from clinical and basic research, this review outlines the underlying pathological mechanisms of OP, and offers a concise summary of the most recent discoveries on the anti-OP mechanism of TCM formulas and their chemically active ingredients, highlighting their detailed effects on osteoblasts and osteoclasts, bone marrow mesenchymal stem cells and bone microstructure, angiogenesis, and the immune system. Furthermore, through the prospective discussion of the unique ingredient combinations in these TCM formulas, we seek to offer valuable information for devising fresh OP treatment strategies.
Mechanism of OP
OP is diagnosed when an individual’s BMD T score falls at or below −2.5, according to WHO diagnostic criteria (30). Mechanistically, various factors contribute to OP progression, including bone and hormone metabolism disorders, modulation of signaling pathways, dysregulation of the vasculature, immune dysfunction, and other factors that can vary (31–33). Here, we mainly summarize the potential pathological mechanisms of OP from various aspects mentioned above (Figure 1).
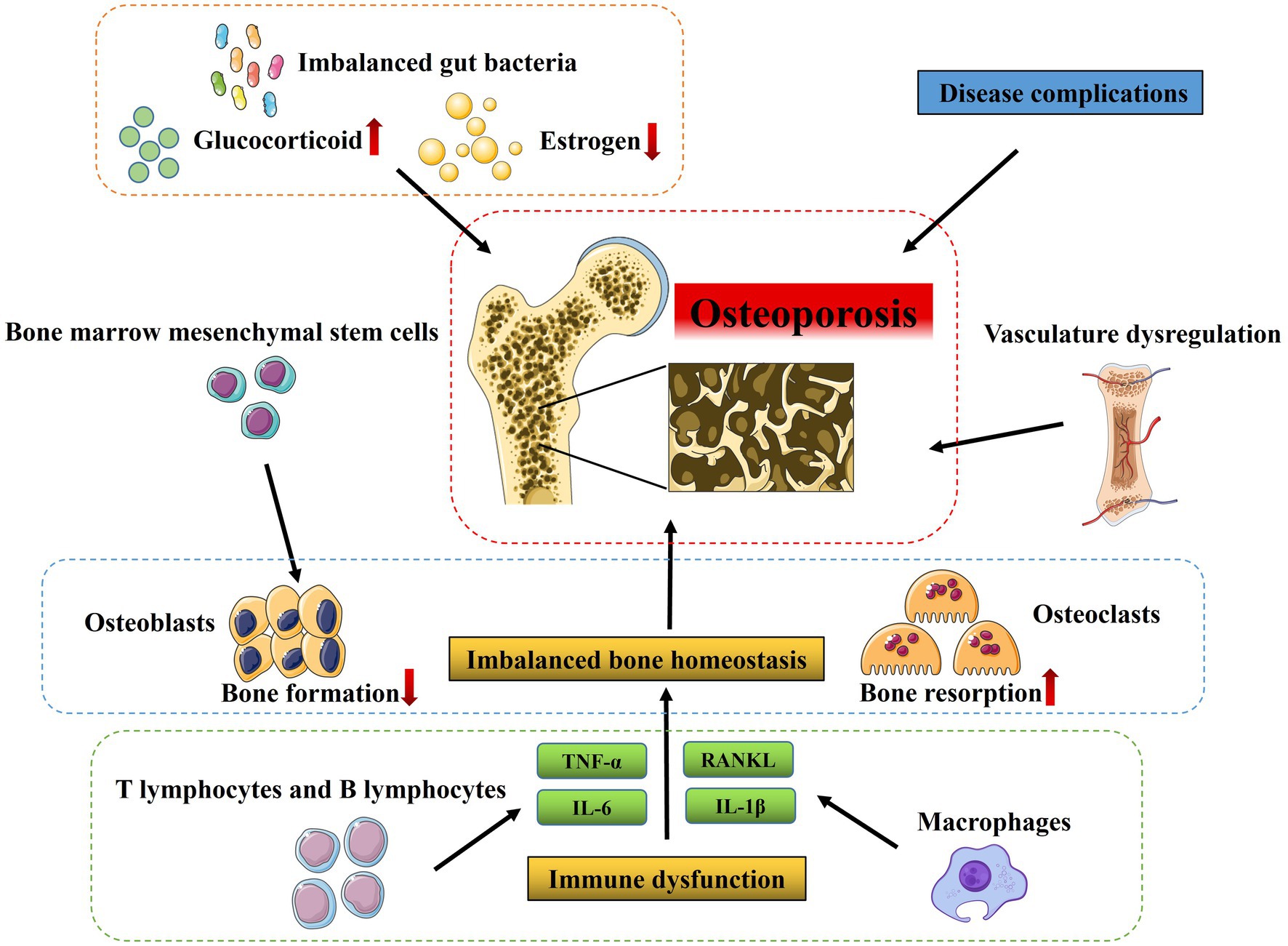
Figure 1. Pathological mechanisms of osteoporosis. Various factors contribute to OP progression, including imbalanced bone homeostasis regulated by osteoblasts (responsible for bone formation), osteoclasts (responsible for bone resorption), bone marrow mesenchymal stem cells as well as vasculature dysregulation, and immune dysfunction and many other factors such as estrogen deficiency, long-term and high-dose glucocorticoid treatment and imbalanced gut bacteria.
Bone homeostasis
The balance of bone-forming and bone-resorbing activities is essential to preserve bone homeostasis. Multiple bone cell types, including osteoblasts (OBs), osteoclasts (OCs), and bone marrow mesenchymal stem cells (BMSCs) are involved in the multifaceted process of bone homeostasis (34, 35). OBs and BMSCs are responsible for bone formation, whereas OCs are the primary functional cells involved in bone resorption (36). This interdependent mechanism is referred to as coupling, where OCs break down the organic matrix and dissolve bone minerals, followed by the recruitment of OBs to deposit fresh bone matrix that later mineralizes. An imbalance in the bone remodeling process, leading to excessive bone resorption relative to bone formation, is the principal factor contributing to the greater occurrence of OP (37, 38). The normal bone remodeling equilibrium can be disrupted by various factors, such as hormones, endocrine regulation, cytokines, and others, which interfere with OBs and OCs differentiation and activity (39, 40). Furthermore, the imbalance in bone remodeling also deteriorates the bone microstructure, making it a target for therapeutic approaches to improve bone strength and decrease the risk of fractures (41).
Besides, the regulation of OP initiation and development involves various signaling pathways, incorporating genetic elements, and modulatory molecules, like TGF-β, RANKL, as well as BMP, which have been studied in great detail over the past few decades (42). These signal transduction pathways are implicated in regulating the differentiation and growth of OBs, OCs, or BMSCs. For example, the Wnt/β-catenin signaling pathway plays a crucial role in the differentiation of BMSCs into OBs by determining their directionality, whereas knocking down β-catenin expression significantly elevates OCs numbers and accelerates bone resorption rates, resulting in the development of OP (42, 43). In addition, TGF-β signaling not only inhibits OBs maturation, mineralization, and transition into osteocytes, but also inhibits OCs differentiation by decreasing RANKL/OPG secretion ratio (44, 45). Other signaling pathways, including Notch, and BMP2/SMADs, also participate in bone homeostasis and are highly correlated to the differentiation, growth, and maturation of OBs, OCs, and BMSCs (46–48). In general, dysregulation of signaling pathways mentioned above is associated with the risk of OP.
Vasculature
The existence of blood vessels in the bone microenvironment is necessary for appropriate bone development and growth, post-fracture healing, and maintaining healthy bones. Angiogenesis in bone tissues involves the proliferation, migration, and tube formation of endothelial cells, which establishes blood flow conduits that deliver essential nourishment, vital oxygen, crucial growth-promoting substances, and hormones to bone cells (31). On the contrary, disruption in angiogenesis in bone tissues can disturb the equilibrium within the bone niche, which is intimately linked to the pathological progression of OP (49, 50). Emerging clinical evidence reveals that individuals with OP have comparatively decreased blood supply to the bone than those with normal bone density, suggesting a significant correlation between bone mineral density and bone vascularization (51). Thus, a significant hallmark of OP is a reduction in the number of sinusoidal and arterial capillaries located in the bone marrow, resulting in decreased delivery of blood to the bone tissue (52). Moreover, the reduction in blood perfusion within the bone marrow is also linked to a decline in BMD and an increased incidence of fractures in elderly women (53). Similarly, age and ovariectomized (OVX) mice show decreased levels of PDGF-B in serum and bone marrow, along with a marked decrease of type H vessels in long bones (54, 55). All these findings indicate a cause-and-effect connection between the number of type H vessels and OP, given the type H vessels’ capability to promote angiogenesis and bone formation, and maintain the delicate equilibrium between bone resorption and formation (54, 56). Therefore, activating angiogenesis might be a vital therapy strategy in the prevention of OP.
Immune system
Bone surrounds the bone marrow, which serves as an essential center for the immune system’s maturation and hematopoiesis. It is well-documented that there is a significant connection between the immune system and the skeletal system. T cells and B cells are the two types of lymphocytes responsible for adaptive immunity. They and their cytokines can impact the functions and activities of both OBs and OCs, with additional indirect implications on OCs due to an increase in RANKL expression via OBs proliferation (57–59). Moreover, these two systems share several regulatory molecules, including cytokines and signaling molecules. The innate immune cells are major producers of pro-inflammatory mediators, with several noteworthy cytokines such as IL-6, TNF-α, IL-1β, as well as ROS that have a crucial impact on OP (60). For instance, TNF-α can promote OCs formation by directly interacting with both OCs along with their precursors, synergistically with RANKL (61). Noteworthy, the regulation of bone homeostasis involves the contribution of multiple immune factors, such as T cells, B cells, NK cells, macrophages, and many other cytokines (37, 62). Different subsets of T cells, namely CD4+ T cells and CD8+ T cells, all exert crucial impacts in regulating bone health. Of these, Th17 cells stand out as a significant cause of bone loss since they produce significantly more RANKL and TNF-α (63, 64). Besides, the presence of B cells and their production of RANKL could be involved in the pathogenesis of ovariectomy-induced bone loss (65). Likewise, M1 macrophages exert a significant impact on promoting osteoclastogenesis by producing abundant ROS and pro-osteoclastogenic cytokines such as TNF-α and IL-1β (66). In contrast, unlike M1 macrophages which promote osteoclastogenesis, M2 macrophages contribute to bone mineralization by promoting the differentiation of MSCs and precursor OBs into mature OBs, thus supporting bone health (67).
Other factors
Accumulating evidence reveals that the development of OP is also affected by various other factors, such as disease complications, estrogen levels, and gut microbiota (68). Among them, endocrine systemic diseases, hematological diseases, or other metabolic diseases, have been shown to be closely related to the occurrence and development of OP (68). It has been reported that diabetic patients may suffer reduced bone turnover and bone loss due to deleterious impacts on bone metabolism and degradation of the extracellular matrix. And the development of brittle bone in diabetes is controlled by many contributing elements, such as obesity, insulin resistance, dysglycemia, myopathy, and the use of certain medications. In parallel, certain comorbidities, including thyroid dysfunction, gonadal disorders, and maldigestion, may disrupt bone metabolism and contribute to an imbalance in affected individuals (69, 70). Given the close relationships between bone and bone marrow, hematological diseases exert a significant influence on the secondary forms of OP; there is an increased rate of bone resorption among patients with hemophilia (71). Additionally, estrogen is considered to act directly on bone cells in preventing a decline of bone mass (72, 73), and it directly or indirectly influences immune cells to impact OBs and OCs via additional intermediaries like OPG/RANKL and chemokines such as TNF-α and IL-1β (74). Moreover, growing evidence indicates there is a connection between a balanced microbiome and stable bone health, and that an imbalance in gut bacteria might worsen the function of OCs, thus resulting in OP (68).
Furthermore, clinical evidence reveals that long-term and high-dose glucocorticoid treatment is another significant contributor to OP (75). Accumulating studies have demonstrated that glucocorticoids can inhibit OBs proliferation and increase rates of apoptosis of OBs, inhibit calcium uptake in the gastrointestinal tract, act as an antagonist to vitamin D, upregulate RANK ligand, and suppress OPG (76, 77). Importantly, glucocorticoid therapy also has been shown to adversely affect muscle mass and muscle strength, increasing the risk for OP fractures (78, 79).
Anti-OP effects of TCM and plant-based natural compounds
Compared with chemically synthesized drugs, TCM possesses beneficial characteristics such as fewer adverse reactions, long-term use, and stable treatment effect, therefore, TCM is increased in clinical trials to treat OP, among them, Bushen Zhuanggu tablet was demonstrated to be effective in the postmenopausal OP treatment by increasing the BMD, as well as regulating calcium and phosphorus metabolism in case-control studies (80–84). TCM formulas, including LWDHP, BSHXD, and other classic formulas, have demonstrated significant clinical effectiveness in both preventing and treating OP (20, 85–88). Given the multifaceted and diverse pathological mechanisms of OP, comprehensively understanding the pharmacological mechanisms of diverse TCM approaches for OP treatment is imperative. Here, we summarize in vitro and preclinical in vivo evidence-based anti-OP mechanisms of TCM formulas and their chemically active ingredients from various perspectives such as OBs and OCs, BMSCs, as well as bone microstructure, angiogenesis, and immune system regulation.
The anti-OP effects of TCM formulas
In general, many TCM formulas have the effect of improving OP phenotypes to some extent by promoting bone homeostasis, and angiogenesis, and regulating the immune system (Table 1). In terms of TCM formulas for the treatment of OP, LWDHP increases the BMD of femurs and improves the biomechanical capability of the vertebral body in OVX rats, mechanically, it could upregulate Runx2 and Osx expression and augment the number of calcified nodules in OBs by activating canonical Wnt/β-catenin cascade through the increase of Lrp-5 and β-catenin expressions (89). Bioinformatics analysis also provides potential therapeutic targets of LWDHP against OP such as AKT1, ATF2, and FBXW7 from a systemic perspective (90, 96). Qing’e Pill (QEP) inhibits OB ferroptosis and increases the transcription of osteogenesis-related genes, such as BMP2, Runx2 to increase the number of trabecula and trabecular connections by activating PI3K/AKT pathway and inhibiting ATM Serine/Threonine Kinase expression, thereby exerting a therapeutic role in OVX rats (97). In parallel, in vitro studies of OBs cultured with Danggui Buxue Decoction (DGBXD) led to a significant improvement in mitochondrial function, antioxidation, and anti-inflammatory, leading to increased OBs activity (91, 92).
As another part of bone homeostasis, OCs are also extensively studied in treated with TCM formulas. TCM formulas, BSHXD, and Jianpi Qingchang Bushen decoction (JQBD) can alleviate bone loss, decrease bone volume, and limit osteoclastogenesis by reducing the RANKL-stimulated NF-κB, ERK, JNK signal transduction pathways in OP model mice (93, 98). Bushen Tongluo decoction (BSTLD) and You Gui Yin (YGY) can promote bone generation and attenuate OC activity and bone resorption in the OVX-induced OP mouse model by reducing RANKL/OPG ratio (53, 99).
Meanwhile, numerous studies have demonstrated that TCM formulas can promote the BMSCs’ growth and migration to alleviate OP symptoms by enhancing the ability of BMSCs to differentiate into OBs. Duhuo Jisheng Decoction (DHJSD) was found to promote the activity of BMSCs, leading to upregulation of the expression of BMP2 and Runx2 genes, and OBs differentiation through activating SMAD1/5/8 and ERK signaling pathway (94). Taohong Siwu Decoction (THSWD) enhances the proliferation, and migration, together with bone-forming potentiality by upregulating VEGF expression and the phosphorylation of FAK and Src (100). Zuogui Pill (ZGP), another classical TCM prescription, could up-regulate let-7f and Runx2 mRNA expression and down-regulate Beclin-1, ATG12, ATG5, LC3, and CTSK mRNA expression in BMSCs to promote osteogenic differentiation of BMSCs, thus improving the OP progression (95), while another study indicated it can also slow down the senescence of BMSCs through modulating Wnt/β-catenin signaling pathway (101).
Moreover, EXD, which has been extensively used for the treatment of OP (102), can improve the bone microstructure and deterioration of bone loss in the OVX-induced OP model through lipid metabolism and the IGF1/PI3K/AKT pathway (103). BSTLD treatment results in a significant promotion in angiogenesis in OVX-induced OP rats, which could be associated with the promotion of HIF-1α/VEGF-regulated angiogenesis signal transduction and inhibition of the RANKL/OPG ratio (53). In a clinical trial, LWDHP has been found to promote the expression of immune-related cardiotrophin-like cytokine factor 1 (CLCF1) in the peripheral blood of postmenopausal osteoporosis patients with kidney Yin deficiency by activating the JAK/STAT signaling pathway, thereby improving immunocompetence, which provides a promising therapeutic approach for postmenopausal OP (104). Other classic Chinese herbal medicine like Xianling Gubao Capsule, Zhuanggu Zhitong Capsule, and Guilu Erxian Glue were also demonstrated to provide potential therapeutic benefits for the treatment of OP through in vivo and in vitro research (105–108).
The anti-OP effects of herbs and chemical ingredients in TCM formulas
Extensive in vivo studies have demonstrated the anti-OP effects of various Chinese herbs and their extracts, including Rhizoma Drynariae homogenous polysaccharide, mixed extracts of Fructus Corni and Radix Achyranthis Bidentatae (the main components of LWDHP and ZGP), Rhizoma Drynariae. These herbs have been shown to ameliorate the OP phenotype by increasing BMD and bone mineral content, which is closely related to the activity of OBs (109–111). Resveratrol, derived from Reynoutria japonica, has been found to exhibit significant antioxidant capacity in OBs by triggering the NRF2/SIRT1/FoxO1 signaling pathway and improving excessive iron-induced oxidative stress (112–114). Puerarin, another bioactive ingredient extracted from the root of Pueraria lobata (Willd.) Ohwi, has been found to significantly upregulate the expression of ALP and OPG mRNA levels while inhibiting OBs apoptosis (115, 116). Similarly, Icariin, a compound rich in TCM formulas like EXD, can also promote OBs proliferation, differentiation, and mineralization, as demonstrated by increased expression of ALP and Col I, and bone nodule formation but inhibit their apoptosis by activating ERK and JNK signaling but not p38 signaling (117). Intriguingly, in another study, MC3T3-E1 subclone 14 cell line administered with Icaritin, a hydrolytic product of Icariin, increased mRNA levels and protein expression of ALP, COL1, OC, OPN, and RUNX2 to enhance preosteoblastic cell differentiation, and upregulated the bone nodule formation and collagen synthesis to improve mineralization through the activation of ERK and p38 signalings but not the JNK signaling (118). These discrepancies in reported signaling activation can be attributed to several factors, including differences in the compounds used, varying concentrations, and inconsistent timing of signal detection between the studies (119).
OCs and a major osteoclastogenic molecule, RANKL, have also been studied in relation to the effective monomer and active ingredients from TCM formulas. For instance, it has been suggested that dendrobium, a traditional medicinal plant, exhibits potent suppression of OCs by inhibiting the increase in ROS, the expression of c-fos, and NFATC1, which are mediated through RANKL, and significantly blocking Mmp9 RNA transcription to reduce LPS-stimulated inflammatory bone loss (120). Moreover, Schisandrin A, which is rich in BSTLD, can inhibit the advantage of ROS induced by RANKL on OCs to improve bone resorption in OVX-induced OP mice via stimulating Nrf2 activity (121). Ellagic acid inhibits the expression of genes and proteins exclusive to OCs and hinders OCs differentiation, which limits bone loss, by blocking RANKL-RANK ligation, along with RANKL-conducted osteoclastogenesis (122, 123). In parallel, Aconitine, Berberine, and dihydro-artemisinin can inhibit RANKL-mediated osteoclastogenesis and bone resorption activity, leading to the promotion of osteogenic recovery (124–126).
Moreover, some TCM has been suggested to have dual effects. For example, Icariin can alleviate mitochondrial membrane potential dysfunction and oxygen-free radical production, promote Runx2, ALP, and OPN expression in OBs caused by excessive iron accumulation, and additionally inhibit the cellular differentiation and activity of OCs (127).
As for BMSCs, Artemisinin exerts antioxidant effects on BMSCs by inhibiting hydrogen peroxide-induced Caspase 3 activation and apoptosis of BMSCs (128). Likewise, Leonurine attenuates oxidative stress-induced COX2 and NOX4 mRNA expression in BMSCs and up-regulates mitochondrial membrane potential levels through the PI3K/Akt/mTOR signaling cascade to enhance the growth and differentiation capability of rat BMSCs (129, 130). Salvianolic acid B, extracted from Salvia miltiorrhiza, has been investigated in human BMSCs that can increase the expression of ALP, OPN, and Runx2 while promoting the formation of bone minerals (131). In addition, Panax notoginseng saponins dose-dependently increased ALP activity and ALP, Cbfa1, OC, and BSP mRNA levels of BMSCs, and decreased the mRNA and protein expression of PPARγ2, so as to accelerate proliferation and osteogenic differentiation of BMSCs (132). Meanwhile, it can also downregulate the number of OC precursor cells to inhibit bone resorption, together with enhancing BMSCs differentiation activity in the direction of osteogenesis (133). Oral administration of Ginkgolide has been identified as increasing the OPG-to-RANKL ratio and thus improving bone mass in three animal OP models, including the aging-, OVX-, and glucocorticoid-induced OP models (134, 135).
The destruction of bone microstructure is one of the typical characteristics of OP, and thus, the repair of bone microstructure is a standard to measure medicinal effectiveness. α-asarone, which is extracted from a TCM herb Acorus tatarinowii, is capable of inhibiting osteoclastogenesis and strengthening bone microstructure in OP models induced by estrogen deficiency (136). Cuscuta extract, which is from TCM formulas such as EXD and BSHXD, indicates an anti-OP effect by increasing bone density and bone microstructure via the c-fos/c-Src kinase/NFATC1 signaling pathway in OVX-induced OP mice (137). As mentioned above, the Wnt/β-catenin signaling pathway exerts a key role in the development of OP. Studies have shown that Ginsenoside Rc and Rhizoma drynariae total flavonoids noticeably hinder the decline of bone mass and bone volume, and promote osteogenesis and bone formation via the Wnt/β-catenin signaling pathway (138, 139). Moreover, Bionic Tiger-Bone Powder promotes osteogenesis, inhibits OCs, and increases collagen content in OVX-induced OP mice, improving bone microstructure and biomechanical strength. In line with the in vivo study, it also increases BMD in postmenopausal patients with OP in clinical (140). In different animal OP models, current evidence also suggests that TCM has preventive effects on OP by improving bone microstructure. Ganoderma lucidum contained in EXD can prevent extra bone loss and improve bone microstructure in OVX-induced OP mice by regulating bone and adipose tissue homeostasis, which can prevent bone loss caused by estrogen deficiency (141). Radix Achyranthis Bidentatae with Eucommiae Cortex herbal pair ameliorates glucocorticoid-induced OP by modulating the expressions of Runx2, OP-1, and β-catenin (142). In terms of the disuse-induced OP model, Scutellaria extract can significantly improve bone density and mechanical strength to prevent OP in the hindlimb unloading tail-suspended rat model (143). And oral administration of total flavonoid extracts from epimedium increase maximum BMD in normal-growing rats (144).
There is increasing evidence that angiogenesis is intimately associated with bone generation and remodeling to ensure adequate bone homeostasis. Thus, the promotion of angiogenesis-dependent osteogenesis and the reduction of bone loss are essential for preventing and managing OP (54). Studies have shown that Naringin derived from the traditional Chinese medicinal plant Drynaria regulated the function of endothelial cells and promoted angiogenesis, thereby exhibiting an anti-osteoporotic effect in postmenopausal osteoporotic rat models (145). As an active component found in many TCM herbs, vitexin modulates both osteogenesis and angiogenesis in an OVX-induced OP rat model through vitamin D receptor and endothelial NO synthase pathway (146). It is well-recognized that diabetes mellitus raises the risk of OP, and TCM also contributes to treating diabetic OP via angiogenesis. Curcumin prevents bone loss and promotes angiogenesis in diabetic OP mice by suppressing the hyper glucose-activated NF-κB pathway to restore osteogenic and angiogenic coupling of BMSC in hyperglycemia (147). In addition, Ginsenoside Rg1 facilitates the secretion of VEGF, triggers the Noggin/Notch signaling pathway, and enhances the interaction between H-type blood vessels and bone formation, leading to an improvement in bone structure in a mouse model of diabetic OP (148). Besides, Salvianolic acid B and Salidroside were also reported to prevent bone loss by enhancing angiogenesis in Glucocorticoid-induced and OVX-induced OP model, respectively, (149, 150).
The term “osteoimmunology” was coined in a commentary in Nature to highlight the connection point between osteology and immunology (151). In recent decades, mounting evidence suggests that TCM herbs and their extracts can regulate the immune system, which is essential for effective OP treatment. Ganoderma lucidum mycelium extract Ling-Zhi-8, an immunomodulatory protein, can effectively alleviate glucocorticoids-induced OP phenotypes in mice, as indicated by the increase in the OPG/RANKL ratio, suppression of osteoclastogenesis, and improvement in serum mineral metabolism, bone formation, and absorption markers expression, as well as expressions of hormone molecules such as estradiol and parathyroid hormone (152). Osthole, a type of coumarin compound present in various medicinal plants, including Cnidium monnieri, can enhance the immunosuppressive capacity of osteoporotic BMSCs to improve the therapeutic effect of BMSCs transplantation on colitis as well as OP (153). Likewise, Cissus quadrangularis markedly promotes the production of immune cells that counteract osteoclastogenesis, including Th1, Th2, and Tregs, in the bone marrow, while simultaneously decreasing the number of OC-promoting Th17 cells. This effectively inhibits RANKL’s ability to generate OCs and hinders the capacity of OCs in bone resorption (154).
Given the importance of proinflammatory cytokines, including TNF-α, IL-1β, as well as IL-17 in osteoimmunology, there is accruing evidence from recent studies suggesting that TCM herbs and their active ingredients such as Sinomenine (155), and Galanin (156) have therapeutical effects on OP by regulating the inflammatory reaction and inflammation-related signaling pathways. We have summarized the chemically active constituents in TCM herbs for treating OP in Table 2.
Conclusion and perspectives
The main feature of OP is the pathological decrease of BMD caused by the imbalance in bone remodeling, which involves a sophisticated interplay of endogenous, exogenous, mechanical, biological, and immunological mediators. TCM formulas are rich in natural compounds and display diverse biological effects in treating various diseases. Importantly, clinical trials as well as in vivo and in vitro findings demonstrate that TCM formulas such as LWDHP, QEP, EXD, YGY, and their chemically active constituents such as Resveratrol, Puerarin, Icariin, Schisandrin A possess the potential to deliver beneficial outcomes in the management of OP. Mechanically, they primarily reverse the OP progression by regulating bone remodeling, bone homeostasis, and angiogenesis, as well as OP-related signaling pathways and immune factors (Figure 2).
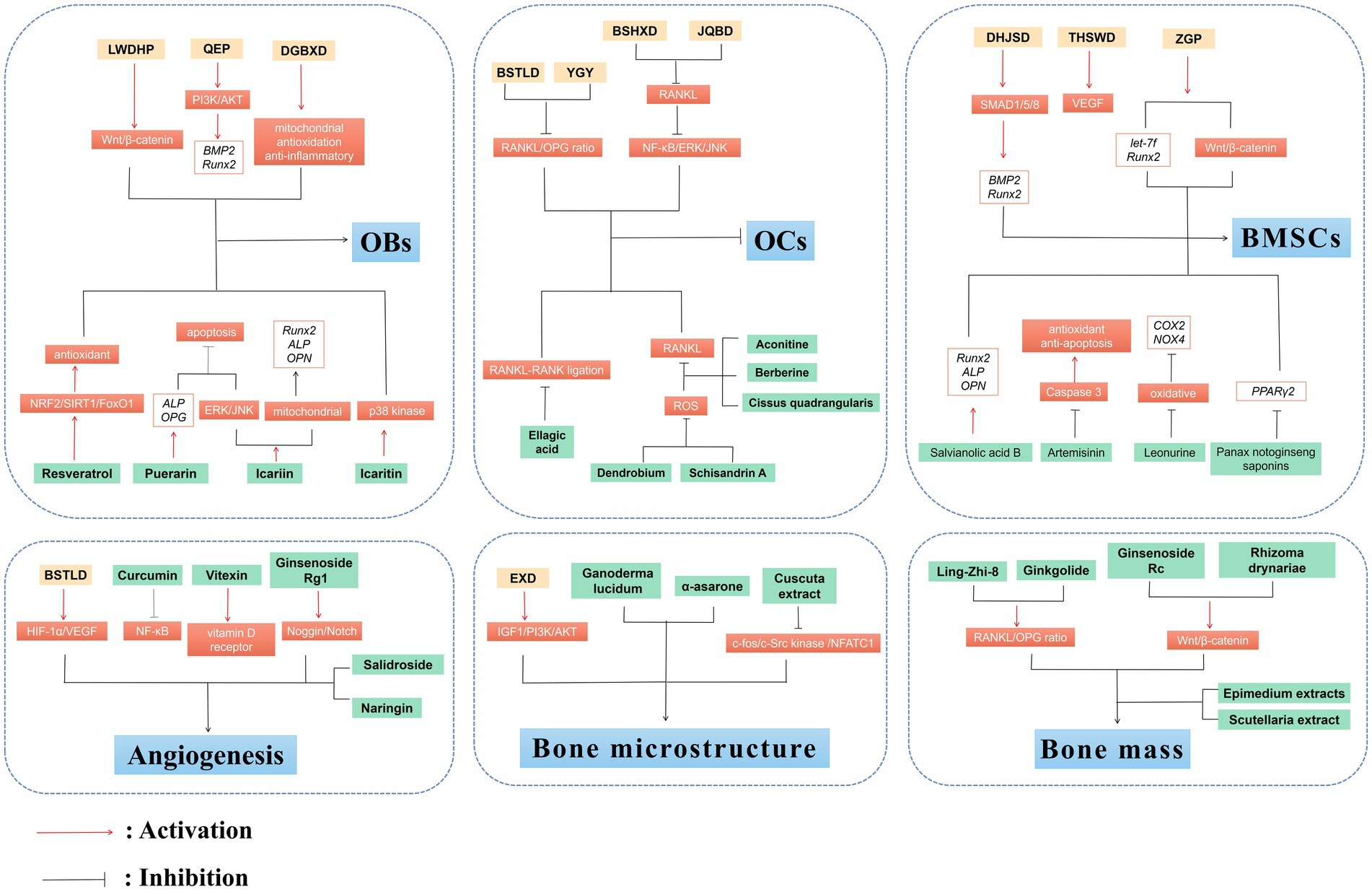
Figure 2. Anti-OP effects of TCM and plant-based natural compounds. TCM and plant-based natural compounds play a therapeutic role on OP from various perspectives such as OBs and OCs, BMSCs, bone microstructure, angiogenesis and bone mass.
Compared to chemical drugs, TCM formulas and their active ingredients possess the advantages of producing fewer adverse effects and being appropriate for sustained usage. TCM formulas containing multiple herbs, in which each single herb medicine often contains anti-OP constituents, have the potential to advance our understanding of traditional medicine’s role in modern healthcare and accelerate the screening of new anti-OP drugs. Also, the combination of chemical drugs and TCM formulas might contribute to discovering effective therapies for treating OP.
It should be noted that treating OP requires a comprehensive understanding of its etiology. Thus, various animal models of OP, including OVX-induced OP models, chemically-induced OP models, and disuse-induced OP models, are frequently utilized to study the underlying mechanisms of this bone disorder. TCM formulas and their chemically active ingredients should be tested in multiple models to comprehensively elucidate their anti-OP effects. Although estrogen substitution therapy can prevent OP, the anti-OP mechanisms of many estrogen-like TCM formulas (such as Shugan Liangxue decoction, Qibao Meiran formula) or monomers (such as Radix astragali, Curcuma comosa Roxb) are still unknown (157–160). Further studies elucidating the anti-OP mechanism of these formulas or monomers targeting estrogen-like effects will greatly promote the treatment of OP with TCM. Moreover, investigating the effects of TCM on the factors that regulate estrogens and their receptors will aid in screening new anti-OP drugs. In the past few years, non-coding RNAs, like LncRNA HOTAIR, LncRNA ROR, and miRNA-14 have been linked to the onset and progression of OP (161, 162), and the expression levels of these ncRNAs have been discovered to be modulated by various active components in TCM, such as ginsenoside, curcumin and baicalin (163). Therefore, ncRNAs may serve as promising molecular targets for clinical therapeutic options of OP with TCM.
Some clinical trials focused on TCM in the treatment of OP have been carried out (82, 164–167), however, most of the clinical studies only contain a small sample size and short treatment duration, as well as the clinical parameters and biomarkers for analysis differ from each other, which results in a limited amount of robust evidence from clinical trials regarding effective approaches for preventing and treating OP. Thus, a sequential combination of TCM and Western medicine is the only way to solve these problems. In fact, some clinical trials on the treatment of OP by the combination of TCM and Western medicine have been carried out, such as Dihuang Decoction plus alendronate (83), Bushen Zhuanggu tablet plus calcium supplement and vitamin D (84), EXD plus caltrate tablets and calcitriol (102). A diverse array of sources from TCM formulas and their chemically active ingredients, along with ample anti-OP mechanisms, further enhance our assurance, eagerness, and motivation to discover new anti-OP drugs, but screening is imminent.
Author contributions
YZ, CW, LZ, and HR: conceptualization. CZ, SS, and MZ: writing original draft preparation. All authors contributed to the article and approved the submitted version.
Funding
This work was financially supported by National Natural Science Foundation of China (Nos. 82174140, 82174401, 81973870), Natural Science Foundation of Zhejiang Province (Nos. LY22H270003 and LQ19H080001), the Joint Funds of the Zhejiang Provincial Natural Science Foundation of China under Grant No. LBY22H270008, Traditional Chinese Medical Administration of Zhejiang Province (Nos. 2022ZX005, 2022ZB119, 2021ZB090), Zhejiang Medical and Health Science and Technology Project (Nos. 2023RC194, 2021KY222), Scientific Research Project of Zhejiang Chinese Medical University (Nos. 2021JKZDZC02, 2021JKZKTS036A, 2021JKJNTZ022B, 2019ZG25), Research Project of Zhejiang Chinese Medical University Affiliated Hospital (Nos. 2022FSYYZZ05 and 2022FSYYZQ02), Zhejiang Chinese Medical University School-level Education and Teaching Reform Project (Nos. CY22001), National Undergraduate Innovation and Entrepreneurship Training Program (Nos. 202210344064, 202210344006, 202210344028, 202210344037).
Conflict of interest
The authors declare that the research was conducted in the absence of any commercial or financial relationships that could be construed as a potential conflict of interest.
Publisher’s note
All claims expressed in this article are solely those of the authors and do not necessarily represent those of their affiliated organizations, or those of the publisher, the editors and the reviewers. Any product that may be evaluated in this article, or claim that may be made by its manufacturer, is not guaranteed or endorsed by the publisher.
References
1. Qaseem, A, Forciea, MA, McLean, RM, Denberg, TD, Barry, MJ, Cooke, M, et al. Treatment of low bone density or osteoporosis to prevent fractures in men and women: a clinical practice guideline update from the American College of Physicians. Ann Intern Med. (2017) 166:818–39. doi: 10.7326/M15-1361
2. Letarouilly, JG, Broux, O, and Clabaut, A. New insights into the epigenetics of osteoporosis. Genomics. (2019) 111:793–8. doi: 10.1016/j.ygeno.2018.05.001
3. Li, Z, Li, D, Chen, R, Gao, S, Xu, Z, and Li, N. Cell death regulation: a new way for natural products to treat osteoporosis. Pharmacol Res. (2023) 187:106635. doi: 10.1016/j.phrs.2022.106635
4. Chandra, A, and Rajawat, J. Skeletal aging and osteoporosis: mechanisms and therapeutics. Int J Mol Sci. (2021) 22:3553. doi: 10.3390/ijms22073553
5. Lane, NE. Epidemiology, etiology, and diagnosis of osteoporosis. Am J Obstet Gynecol. (2006) 194:S3–S11. doi: 10.1016/j.ajog.2005.08.047
6. Noh, JY, Yang, Y, and Jung, H. Molecular mechanisms and emerging therapeutics for osteoporosis. Int J Mol Sci. (2020) 21:7623. doi: 10.3390/ijms21207623
7. Yong, EL, and Logan, S. Menopausal osteoporosis: screening, prevention and treatment. Singap Med J. (2021) 62:159–66. doi: 10.11622/smedj.2021036
8. Palacios, S. Medical treatment of osteoporosis. Climacteric J Int Menopause Soc. (2022) 25:43–9. doi: 10.1080/13697137.2021.1951697
9. Reid, IR, and Billington, EO. Drug therapy for osteoporosis in older adults. Lancet. (2022) 399:1080–92. doi: 10.1016/S0140-6736(21)02646-5
10. Wang, J, Wong, YK, and Liao, F. What has traditional Chinese medicine delivered for modern medicine? Expert Rev Mol Med. (2018) 20:e4. doi: 10.1017/erm.2018.3
11. Xu, W, Li, B, Xu, M, Yang, T, and Hao, X. Traditional Chinese medicine for precancerous lesions of gastric cancer: a review. Biomed Pharmacother. (2022) 146:112542. doi: 10.1016/j.biopha.2021.112542
12. Duan, T, Li, L, Yu, Y, Li, T, Han, R, Sun, X, et al. Traditional Chinese medicine use in the pathophysiological processes of intracerebral hemorrhage and comparison with conventional therapy. Pharmacol Res. (2022) 179:106200. doi: 10.1016/j.phrs.2022.106200
13. Zheng, Y, Zhang, ZJ, Han, XM, Ding, Y, Chen, YY, Wang, XF, et al. A proprietary herbal medicine (5-Ling granule) for Tourette syndrome: a randomized controlled trial. J Child Psychol Psychiatry. (2016) 57:74–83. doi: 10.1111/jcpp.12432
14. Gu, SC, Shi, R, Gaoag, C, Yuan, XL, Wu, Y, Zhang, Y, et al. Traditional Chinese medicine Pingchan granule for motor symptoms and functions in Parkinson’s disease: a multicenter, randomized, double-blind, placebo-controlled study. Phytomedicine Int J Phytother Phytopharmacol. (2023) 108:154497. doi: 10.1016/j.phymed.2022.154497
15. Lai, X, Dong, Z, Wu, S, Zhou, X, Zhang, G, Xiong, S, et al. Efficacy and safety of Chinese herbal medicine compared with losartan for mild essential hypertension: a randomized, multicenter, double-blind, noninferiority trial. Circ Cardiovasc Qual Outcomes. (2022) 15:e007923. doi: 10.1161/CIRCOUTCOMES.121.007923
16. Zhang, HP, Wang, L, Wang, Z, Xu, XR, Zhou, XM, Liu, G, et al. Chinese herbal medicine formula for acute asthma: a multi-center, randomized, double-blind, proof-of-concept trial. Respir Med. (2018) 140:42–9. doi: 10.1016/j.rmed.2018.05.014
17. Li, J, Xie, Y, Zhao, P, Qin, Y, Oliver, BG, Tian, Y, et al. A Chinese herbal formula ameliorates COPD by inhibiting the inflammatory response via downregulation of p65, JNK, and p38. Phytomed Int J Phytother Phytopharmacol. (2021) 83:153475. doi: 10.1016/j.phymed.2021.153475
18. Liu, ZW, Liu, B, Wu, Q, Chen, YF, Xu, P, Xie, HH, et al. Effect of Er-xian decoction on femur proteomics in ovariectomized osteoporosis rats. Zhongguo Zhong yao za zhi China J Chin Mater Med. (2017) 42:2558–63. doi: 10.19540/j.cnki.cjcmm.20170609.008
19. Wang, P, Wei, X, Zhang, F, Yang, K, Qu, C, Luo, H, et al. Ginsenoside Rg1 of Panax ginseng stimulates the proliferation, odontogenic/osteogenic differentiation and gene expression profiles of human dental pulp stem cells. Phytomed Int J Phytother Phytopharmacol. (2014) 21:177–83. doi: 10.1016/j.phymed.2013.08.021
20. Liu, Y, Fu, B, Li, X, Chen, C, Li, X, Xu, L, et al. Bushen huoxue decoction inhibits RANKL-stimulated osteoclastogenesis and glucocorticoid-induced bone loss by modulating the NF-κB, ERK, and JNK signaling pathways. Front Pharmacol. (2022) 13:1007839. doi: 10.3389/fphar.2022.1007839
21. Zhang, H, Yao, S, Zhang, Z, Zhou, C, Fu, F, Bian, Y, et al. Network pharmacology and experimental validation to reveal the pharmacological mechanisms of Liuwei Dihuang decoction against intervertebral disc degeneration. Drug Des Devel Ther. (2021) 15:4911–24. doi: 10.2147/DDDT.S338439
22. Yu, H, Yao, S, Zhou, C, Fu, F, Luo, H, Du, W, et al. Morroniside attenuates apoptosis and pyroptosis of chondrocytes and ameliorates osteoarthritic development by inhibiting NF-κB signaling. J Ethnopharmacol. (2021) 266:113447. doi: 10.1016/j.jep.2020.113447
23. Zhou, C, Yao, S, Fu, F, Bian, Y, Zhang, Z, Zhang, H, et al. Morroniside attenuates nucleus pulposus cell senescence to alleviate intervertebral disc degeneration via inhibiting ROS-hippo-p53 pathway. Front Pharmacol. (2022) 13:942435. doi: 10.3389/fphar.2022.1090857
24. Zhang, H, Zhou, C, Zhang, Z, Yao, S, Bian, Y, Fu, F, et al. Integration of network pharmacology and experimental validation to explore the pharmacological mechanisms of Zhuanggu Busui formula against osteoporosis. Front Endocrinol. (2021) 12:841668. doi: 10.3389/fendo.2021.766778
25. Huang, CY, Cheng, CJ, Chiou, WF, Chang, WC, Kang, YN, and Lee, MH. Efficacy and safety of Duhuo Jisheng decoction add-on bisphosphonate medications in patients with osteoporosis: a meta-analysis of randomized controlled trials. J Ethnopharmacol. (2022) 283:114732. doi: 10.1016/j.jep.2021.114732
26. Wen, J, Bao, Z, Li, L, Liu, Y, Wei, B, Ye, X, et al. Qiangguyin inhibited fat accumulation in OVX mice through the p38 MAPK signaling pathway to achieve anti-osteoporosis effects. Biomed Pharmacother. (2023) 158:114122. doi: 10.1016/j.biopha.2022.114122
27. Shi, S, Wang, F, Huang, Y, Chen, B, Pei, C, Huang, D, et al. Epimedium for osteoporosis based on western and eastern medicine: an updated systematic review and meta-analysis. Front Pharmacol. (2022) 13:782096. doi: 10.3389/fphar.2022.779942
28. Feng, R, Ding, F, Mi, XH, Liu, SF, Jiang, AL, Liu, BH, et al. Protective effects of Ligustroflavone, an active compound from Ligustrum lucidum, on diabetes-induced osteoporosis in mice: a potential candidate as calcium-sensing receptor antagonist. Am J Chin Med. (2019) 47:457–76. doi: 10.1142/S0192415X1950023X
29. Song, M, Jia, F, Cao, Z, Zhang, H, Liu, M, and Gao, L. Ginsenoside Rg3 attenuates aluminum-induced osteoporosis through regulation of oxidative stress and bone metabolism in rats. Biol Trace Elem Res. (2020) 198:557–66. doi: 10.1007/s12011-020-02089-9
30. Kanis, JA. Assessment of fracture risk and its application to screening for postmenopausal osteoporosis. Report of a WHO study group. World Health Organ Tech Rep Ser. (1994) 843:1–129. doi: 10.1007/BF01622200
31. Tong, X, Chen, X, Zhang, S, Huang, M, Shen, X, Xu, J, et al. The effect of exercise on the prevention of osteoporosis and bone angiogenesis. Biomed Res Int. (2019) 2019:8171897. doi: 10.1155/2019/8171897
32. Cui, J, Shibata, Y, Zhu, T, Zhou, J, and Zhang, J. Osteocytes in bone aging: advances, challenges, and future perspectives. Ageing Res Rev. (2022) 77:101608. doi: 10.1016/j.arr.2022.101608
33. Fischer, V, and Haffner-Luntzer, M. Interaction between bone and immune cells: implications for postmenopausal osteoporosis. Semin Cell Dev Biol. (2022) 123:14–21. doi: 10.1016/j.semcdb.2021.05.014
34. Wang, S, Hu, M, Song, D, Tang, L, and Jiang, H. Research progress on the role and mechanism of miR-671 in bone metabolism and bone-related diseases. Front Oncol. (2022) 12:1018308. doi: 10.3389/fonc.2022.1018308
35. Hou, J, He, C, He, W, Yang, M, Luo, X, and Li, C. Obesity and bone health: a complex link. Front Cell Dev Biol. (2020) 8:600181. doi: 10.3389/fcell.2020.600181
36. Hu, L, Yin, C, Zhao, F, Ali, A, Ma, J, and Qian, A. Mesenchymal stem cells: cell fate decision to osteoblast or adipocyte and application in osteoporosis treatment. Int J Mol Sci. (2018) 19:20360. doi: 10.3390/ijms19020360
37. Weitzmann, MN, and Ofotokun, I. Physiological and pathophysiological bone turnover – role of the immune system. Nat Rev Endocrinol. (2016) 12:518–32. doi: 10.1038/nrendo.2016.91
38. Zhan, Y, Liang, J, Tian, K, Che, Z, Wang, Z, Yang, X, et al. Vindoline inhibits RANKL-induced osteoclastogenesis and prevents ovariectomy-induced bone loss in mice. Front Pharmacol. (2019) 10:1587. doi: 10.3389/fphar.2019.01587
39. Liang, B, Burley, G, Lin, S, and Shi, YC. Osteoporosis pathogenesis and treatment: existing and emerging avenues. Cell Mol Biol Lett. (2022) 27:72. doi: 10.1186/s11658-022-00371-3
40. Yang, Y, Yujiao, W, Fang, W, Linhui, Y, Ziqi, G, Zhichen, W, et al. The roles of miRNA, lncRNA and circRNA in the development of osteoporosis. Biol Res. (2020) 53:40. doi: 10.1186/s40659-020-00309-z
41. Rizzoli, R, Chapurlat, RD, Laroche, JM, Krieg, MA, Thomas, T, Frieling, I, et al. Effects of strontium ranelate and alendronate on bone microstructure in women with osteoporosis. Results of a 2-year study. Osteoporosis Int J established as result of cooperation between the European Foundation for Osteoporosis and the National Osteoporosis Foundation of the USA. (2012) 23:305–15. doi: 10.1007/s00198-011-1758-z
42. Gao, Y, Chen, N, Fu, Z, and Zhang, Q. Progress of WNT signaling pathway in osteoporosis. Biomol Ther. (2023) 13:30483. doi: 10.3390/biom13030483
43. Zhang, XY, Li, HN, Chen, F, Chen, YP, Chai, Y, Liao, JZ, et al. Icariin regulates miR-23a-3p-mediated osteogenic differentiation of BMSCs via BMP-2/Smad5/Runx2 and WNT/β-catenin pathways in osteonecrosis of the femoral head. Saudi Pharmaceut J SPJ Off Publ Saudi Pharmaceut Soc. (2021) 29:1405–15. doi: 10.1016/j.jsps.2021.10.009
44. Wu, M, Chen, G, and Li, YP. TGF-β and BMP signaling in osteoblast, skeletal development, and bone formation, homeostasis and disease. Bone Res. (2016) 4:16009. doi: 10.1038/boneres.2016.9
45. Zhang, P, Zhang, H, Lin, J, Xiao, T, Xu, R, Fu, Y, et al. Insulin impedes osteogenesis of BMSCs by inhibiting autophagy and promoting premature senescence via the TGF-β1 pathway. Aging. (2020) 12:2084–100. doi: 10.18632/aging.102723
46. Chung, HJ, Kim, WK, Oh, J, Kim, MR, Shin, JS, Lee, J, et al. Anti-osteoporotic activity of harpagoside by upregulation of the BMP2 and WNT signaling pathways in osteoblasts and suppression of differentiation in osteoclasts. J Nat Prod. (2017) 80:434–42. doi: 10.1021/acs.jnatprod.6b00964
47. Yoshida, G, Kawabata, T, Takamatsu, H, Saita, S, Nakamura, S, Nishikawa, K, et al. Degradation of the NOTCH intracellular domain by elevated autophagy in osteoblasts promotes osteoblast differentiation and alleviates osteoporosis. Autophagy. (2022) 18:2323–32. doi: 10.1080/15548627.2021.2017587
48. Chai, S, Wan, L, Wang, JL, Huang, JC, and Huang, HX. Gushukang inhibits osteocyte apoptosis and enhances BMP-2/Smads signaling pathway in ovariectomized rats. Phytomed Int J Phytother Phytopharmacol. (2019) 64:153063. doi: 10.1016/j.phymed.2019.153063
49. Filipowska, J, Tomaszewski, KA, Niedźwiedzki, Ł, Walocha, JA, and Niedźwiedzki, T. The role of vasculature in bone development, regeneration and proper systemic functioning. Angiogenesis. (2017) 20:291–302. doi: 10.1007/s10456-017-9541-1
50. Wang, X, Li, X, Li, J, Zhai, L, Liu, D, Abdurahman, A, et al. Mechanical loading stimulates bone angiogenesis through enhancing type H vessel formation and downregulating exosomal miR-214-3p from bone marrow-derived mesenchymal stem cells. FASEB J. (2021) 35:e21150. doi: 10.1096/fj.202001080RR
51. Alagiakrishnan, K, Juby, A, Hanley, D, Tymchak, W, and Sclater, A. Role of vascular factors in osteoporosis. J Gerontol A Biol Sci Med Sci. (2003) 58:362–6. doi: 10.1093/gerona/58.4.m362
52. Zhao, Q, Shen, X, Zhang, W, Zhu, G, Qi, J, and Deng, L. Mice with increased angiogenesis and osteogenesis due to conditional activation of HIF pathway in osteoblasts are protected from ovariectomy induced bone loss. Bone. (2012) 50:763–70. doi: 10.1016/j.bone.2011.12.003
53. Yuan, H, Xiao, L, Min, W, Yuan, W, Lu, S, and Huang, G. Bu-Shen-Tong-Luo decoction prevents bone loss via inhibition of bone resorption and enhancement of angiogenesis in ovariectomy-induced osteoporosis of rats. J Ethnopharmacol. (2018) 220:228–38. doi: 10.1016/j.jep.2018.01.007
54. Xie, H, Cui, Z, Wang, L, Xia, Z, Hu, Y, Xian, L, et al. PDGF-BB secreted by preosteoclasts induces angiogenesis during coupling with osteogenesis. Nat Med. (2014) 20:1270–8. doi: 10.1038/nm.3668
55. Wang, Q, Zhou, J, Wang, X, Xu, Y, Liang, Z, Gu, X, et al. Coupling induction of osteogenesis and type H vessels by pulsed electromagnetic fields in ovariectomy-induced osteoporosis in mice. Bone. (2022) 154:116211. doi: 10.1016/j.bone.2021.116211
56. Shangguan, Y, Wu, Z, Xie, X, Zhou, S, He, H, Xiao, H, et al. Low-activity programming of the PDGFRβ/FAK pathway mediates H-type vessel dysplasia and high susceptibility to osteoporosis in female offspring rats after prenatal dexamethasone exposure. Biochem Pharmacol. (2021) 185:114414. doi: 10.1016/j.bcp.2021.114414
57. Takayanagi, H. Osteoimmunology and the effects of the immune system on bone. Nat Rev Rheumatol. (2009) 5:667–76. doi: 10.1038/nrrheum.2009.217
58. Wu, D, Cline-Smith, A, Shashkova, E, Perla, A, Katyal, A, and Aurora, R. T-cell mediated inflammation in postmenopausal osteoporosis. Front Immunol. (2021) 12:687551. doi: 10.3389/fimmu.2021.783362
59. Weitzmann, MN. T-cells and B-cells in osteoporosis. Curr Opin Endocrinol Diabetes Obes. (2014) 21:461–7. doi: 10.1097/MED.0000000000000103
60. Saxena, Y, Routh, S, and Mukhopadhaya, A. Immunoporosis: role of innate immune cells in osteoporosis. Front Immunol. (2021) 12:687037. doi: 10.3389/fimmu.2021.687037
61. Zhao, B, Grimes, SN, Li, S, Hu, X, and Ivashkiv, LB. TNF-induced osteoclastogenesis and inflammatory bone resorption are inhibited by transcription factor RBP-J. J Exp Med. (2012) 209:319–34. doi: 10.1084/jem.20111566
62. Limmer, A, and Wirtz, DC. Osteoimmunology: influence of the immune system on bone regeneration and consumption. Z Orth Unfallchirurgie. (2017) 155:273–80. doi: 10.1055/s-0043-100100
63. Sato, K, Suematsu, A, Okamoto, K, Yamaguchi, A, Morishita, Y, Kadono, Y, et al. Th17 functions as an osteoclastogenic helper T cell subset that links T cell activation and bone destruction. J Exp Med. (2006) 203:2673–82. doi: 10.1084/jem.20061775
64. Srivastava, RK, Dar, HY, and Mishra, PK. Immunoporosis: immunology of osteoporosis-role of T cells. Front Immunol. (2018) 9:657. doi: 10.3389/fimmu.2018.00657
65. Li, Y, Toraldo, G, Li, A, Yang, X, Zhang, H, Qian, WP, et al. B cells and T cells are critical for the preservation of bone homeostasis and attainment of peak bone mass in vivo. Blood. (2007) 109:3839–48. doi: 10.1182/blood-2006-07-037994
66. Zhang, B, Yang, Y, Yi, J, Zhao, Z, and Ye, R. Hyperglycemia modulates M1/M2 macrophage polarization via reactive oxygen species overproduction in ligature-induced periodontitis. J Periodontal Res. (2021) 56:991–1005. doi: 10.1111/jre.12912
67. Muñoz, J, Akhavan, NS, Mullins, AP, and Arjmandi, BH. Macrophage polarization and osteoporosis: a review. Nutrients. (2020) 12:102999. doi: 10.3390/nu12102999
68. Seely, KD, Kotelko, CA, Douglas, H, Bealer, B, and Brooks, AE. The human gut microbiota: a key mediator of osteoporosis and osteogenesis. Int J Mol Sci. (2021) 22:179452. doi: 10.3390/ijms22179452
69. Shanbhogue, VV, Mitchell, DM, Rosen, CJ, and Bouxsein, ML. Type 2 diabetes and the skeleton: new insights into sweet bones. Lancet Diabetes Endocrinol. (2016) 4:159–73. doi: 10.1016/S2213-8587(15)00283-1
70. Sobh, MM, Abdalbary, M, Elnagar, S, Nagy, E, Elshabrawy, N, Abdelsalam, M, et al. Secondary osteoporosis and metabolic bone diseases. J Clin Med. (2022) 11:92382. doi: 10.3390/jcm11092382
71. Rodriguez-Merchan, EC, and Valentino, LA. Increased bone resorption in hemophilia. Blood Rev. (2019) 33:6–10. doi: 10.1016/j.blre.2018.05.002
72. Miyauchi, Y, Sato, Y, Kobayashi, T, Yoshida, S, Mori, T, Kanagawa, H, et al. HIF1α is required for osteoclast activation by estrogen deficiency in postmenopausal osteoporosis. Proc Natl Acad Sci U S A. (2013) 110:16568–73. doi: 10.1073/pnas.1308755110
73. Streicher, C, Heyny, A, Andrukhova, O, Haigl, B, Slavic, S, Schüler, C, et al. Estrogen regulates bone turnover by targeting RANKL expression in bone lining cells. Sci Rep. (2017) 7:6460. doi: 10.1038/s41598-017-06614-0
74. Zhang, L, Zheng, YL, Wang, R, Wang, XQ, and Zhang, H. Exercise for osteoporosis: a literature review of pathology and mechanism. Front Immunol. (2022) 13:1005665. doi: 10.3389/fimmu.2022.1005665
75. Weinstein, RS, Jilka, RL, Parfitt, AM, and Manolagas, SC. Inhibition of osteoblastogenesis and promotion of apoptosis of osteoblasts and osteocytes by glucocorticoids. Potential mechanisms of their deleterious effects on bone. J Clin Invest. (1998) 102:274–82. doi: 10.1172/JCI2799
76. Kondo, T, Kitazawa, R, Yamaguchi, A, and Kitazawa, S. Dexamethasone promotes osteoclastogenesis by inhibiting osteoprotegerin through multiple levels. J Cell Biochem. (2008) 103:335–45. doi: 10.1002/jcb.21414
77. Hayat, S, and Magrey, MN. Glucocorticoid-induced osteoporosis: insights for the clinician. Cleve Clin J Med. (2020) 87:417–26. doi: 10.3949/ccjm.87a.19039
78. Natsui, K, Tanaka, K, Suda, M, Yasoda, A, Sakuma, Y, Ozasa, A, et al. High-dose glucocorticoid treatment induces rapid loss of trabecular bone mineral density and lean body mass. Osteoporosis Int J established as result of cooperation between the European Foundation for Osteoporosis and the National Osteoporosis Foundation of the USA. (2006) 17:105–8. doi: 10.1007/s00198-005-1923-3
79. Szulc, P, Beck, TJ, Marchand, F, and Delmas, PD. Low skeletal muscle mass is associated with poor structural parameters of bone and impaired balance in elderly men – the MINOS study. J Bone Miner Res Off J Am Soc Bone and Miner Res. (2005) 20:721–9. doi: 10.1359/JBMR.041230
80. Che, Q, Luo, T, Shi, J, He, Y, and Xu, DL. Mechanisms by which traditional Chinese medicines influence the intestinal flora and intestinal barrier. Front Cell Infect Microbiol. (2022) 12:863779. doi: 10.3389/fcimb.2022.863779
81. Li, T, Gao, S, Han, W, Gao, Z, Wei, Y, Wu, G, et al. Potential effects and mechanisms of Chinese herbal medicine in the treatment of psoriasis. J Ethnopharmacol. (2022) 294:115275. doi: 10.1016/j.jep.2022.115275
82. Ouyang, GL, Feng, XH, Xiao, LB, Huang, Z, Xia, Q, and Zhu, F. Effects of Chinese herbal medicine Qianggu capsule on patients with rheumatoid arthritis-induced osteoporosis: a report of 82 cases. Zhong Xi Yi Jie He Xue Bao J Chinese Integr Med. (2012) 10:1394–9. doi: 10.3736/jcim20121210
83. Wan, JM, Zhang, JF, Huang, K, Zhang, PL, and Zhu, SY. Comparison of the clinical effects between Dihuang decoction and alendronate sodium in the treatment of primary osteoporosis. Zhongguo gu shang China J Orthopaed Traumatol. (2019) 32:535–8. doi: 10.3969/j.issn.1003-0034.2019.06.010
84. Chen, T, Li, G, and Xu, Y. Study on the effect of Bushen Zhuanggu tablet combined with conventional regimen on bone mineral density improvement, functional recovery and fracture risk prevention in patients with postmenopausal osteoporosis. Comput Math Methods Med. (2023) 2023:4846392. doi: 10.1155/2023/4846392
85. Xie, H, Hua, Z, Guo, M, Lin, S, Zhou, Y, Weng, Z, et al. Gut microbiota and metabonomics used to explore the mechanism of Qing’e pills in alleviating osteoporosis. Pharm Biol. (2022) 60:785–800. doi: 10.1080/13880209.2022.2056208
86. Cheng, M, Wang, Q, Fan, Y, Liu, X, Wang, L, Xie, R, et al. A traditional Chinese herbal preparation, Er-Zhi-Wan, prevent ovariectomy-induced osteoporosis in rats. J Ethnopharmacol. (2011) 138:279–85. doi: 10.1016/j.jep.2011.09.030
87. Li, W, Liu, Z, Liu, L, Yang, F, Li, W, Zhang, K, et al. Effect of Zuogui pill and Yougui pill on osteoporosis: a randomized controlled trial. J Tradit Chinese Med Chung i tsa chih ying wen pan. (2018) 38:33–42. doi: 10.1016/j.jtcm.2018.01.005
88. Liu, MM, Dong, R, Hua, Z, Lv, NN, Ma, Y, Huang, GC, et al. Therapeutic potential of Liuwei Dihuang pill against KDM7A and Wnt/β-catenin signaling pathway in diabetic nephropathy-related osteoporosis. Biosci Rep. (2020) 40:201778. doi: 10.1042/BSR20201778
89. Xia, B, Xu, B, Sun, Y, Xiao, L, Pan, J, Jin, H, et al. The effects of Liuwei Dihuang on canonical Wnt/β-catenin signaling pathway in osteoporosis. J Ethnopharmacol. (2014) 153:133–41. doi: 10.1016/j.jep.2014.01.040
90. Wang, Q, Huang, P, Xia, C, and Fu, D. Network pharmacology-based strategy to investigate pharmacological mechanism of Liuwei Dihuang pill against postmenopausal osteoporosis. Medicine. (2022) 101:e31387. doi: 10.1097/MD.0000000000031387
91. Kwan, KKL, Dong, TTX, and Tsim, KWK. Danggui Buxue Tang, a Chinese herbal decoction containing Astragali Radix and Angelicae Sinensis Radix, improves mitochrondial bioenergetics in osteoblast. Phytomed Int J Phytother Phytopharmacol. (2021) 88:153605. doi: 10.1016/j.phymed.2021.153605
92. Wang, N, Xu, P, Wang, X, Yao, W, Wang, B, Wu, Y, et al. Timosaponin AIII attenuates inflammatory injury in AGEs-induced osteoblast and alloxan-induced diabetic osteoporosis zebrafish by modulating the RAGE/MAPK signaling pathways. Phytomedicine Int J Phytother Phytopharmacol. (2020) 75:153247. doi: 10.1016/j.phymed.2020.153247
93. Liu, Y, Fu, B, Li, X, Chen, C, Li, X, Xu, L, et al. Corrigendum: Bushen huoxue decoction inhibits RANKL-stimulated osteoclastogenesis and glucocorticoid-induced bone loss by modulating the NF-κB, ERK, and JNK signaling pathways. Front Pharmacol. (2023) 14:1148908. doi: 10.3389/fphar.2023.1148908
94. Wang, JY, Chen, WM, Wen, CS, Hung, SC, Chen, PW, and Chiu, JH. Du-Huo-Ji-sheng-Tang and its active component ligusticum chuanxiong promote osteogenic differentiation and decrease the aging process of human mesenchymal stem cells. J Ethnopharmacol. (2017) 198:64–72. doi: 10.1016/j.jep.2016.12.011
95. Shen, G, Shang, Q, Zhang, Z, Zhao, W, Chen, H, Mijiti, I, et al. Zuo-Gui-Wan aqueous extract ameliorates glucocorticoid-induced spinal osteoporosis of rats by regulating let-7f and autophagy. Front Endocrinol. (2022) 13:878963. doi: 10.3389/fendo.2022.878963
96. Gong, R, Ren, S, Chen, M, Wang, Y, Zhang, G, Shi, L, et al. Bioinformatics analysis reveals the altered gene expression of patients with postmenopausal osteoporosis using Liuweidihuang pills treatment. Biomed Res Int. (2019) 2019:1907906. doi: 10.1155/2019/1907906
97. Hao, J, Bei, J, Li, Z, Han, M, Ma, B, Ma, P, et al. Qing’e pill inhibits osteoblast Ferroptosis via ATM serine/threonine kinase (ATM) and the PI3K/AKT pathway in primary osteoporosis. Front Pharmacol. (2022) 13:902102. doi: 10.3389/fphar.2022.902102
98. Zhang, YL, Chen, Q, Zheng, L, Zhang, ZW, Chen, YJ, Dai, YC, et al. Jianpi Qingchang Bushen decoction improves inflammatory response and metabolic bone disorder in inflammatory bowel disease-induced bone loss. World J Gastroenterol. (2022) 28:1315–28. doi: 10.3748/wjg.v28.i13.1315
99. Zeng, Q, Xu, R, Ling, H, Zhao, S, Wang, X, Yuan, W, et al. N-butanol extract of modified You-Gui-Yin attenuates Osteoclastogenesis and ameliorates osteoporosis by inhibiting RANKL-mediated NF-κB signaling. Front Endocrinol. (2022) 13:925848. doi: 10.3389/fendo.2022.925848
100. Lu, X, Li, J, Zhou, B, Lu, X, Li, W, and Ouyang, J. Taohong Siwu decoction enhances human bone marrow mesenchymal stem cells proliferation, migration and osteogenic differentiation via VEGF-FAK signaling in vitro. J Ethnopharmacol. (2023) 307:116203. doi: 10.1016/j.jep.2023.116203
101. Kang, X, Chen, L, Yang, S, Gong, Z, Hu, H, Zhang, X, et al. Zuogui Wan slowed senescence of bone marrow mesenchymal stem cells by suppressing Wnt/β-catenin signaling. J Ethnopharmacol. (2022) 294:115323. doi: 10.1016/j.jep.2022.115323
102. Li, JY, Jia, YS, Chai, LM, Mu, XH, Ma, S, Xu, L, et al. Effects of Chinese herbal formula erxian decoction for treating osteoporosis: a systematic review. Clin Interv Aging. (2017) 12:45–53. doi: 10.2147/CIA.S117597
103. Ma, Y, Hu, J, Song, C, Li, P, Cheng, Y, Wang, Y, et al. Er-Xian decoction attenuates ovariectomy-induced osteoporosis by modulating fatty acid metabolism and IGF1/PI3K/AKT signaling pathway. J Ethnopharmacol. (2023) 301:115835. doi: 10.1016/j.jep.2022.115835
104. Ge, JR, Xie, LH, Chen, J, Li, SQ, Xu, HJ, Lai, YL, et al. Liuwei Dihuang pill () treats postmenopausal osteoporosis with Shen (kidney) Yin deficiency via Janus kinase/signal transducer and activator of transcription signal pathway by up-regulating Cardiotrophin-like cytokine factor 1 expression. Chin J Integr Med. (2018) 24:415–22. doi: 10.1007/s11655-016-2744-2
105. Si, Y, Li, S, Guo, Y, Wang, L, Ma, Y, and Yin, H. Chinese herbal medicine Guilu Erxian glue inhibits osteoclast formation and activity via Mc3t3-derived extracellular vesicles in vitro. Altern Ther Health Med. (2023)
106. Men, Z, Huang, C, Xu, M, Ma, J, Wan, L, Huang, J, et al. Zhuanggu Zhitong capsule alleviates postmenopausal osteoporosis in ovariectomized rats by regulating autophagy through AMPK/mTOR signaling pathway. Ann Transl Med. (2022) 10:900. doi: 10.21037/atm-22-3724
107. Chen, J, Zheng, J, Chen, M, Lin, S, and Lin, Z. The efficacy and safety of Chinese herbal medicine Xianling Gubao capsule combined with alendronate in the treatment of primary osteoporosis: a systematic review and meta-analysis of 20 randomized controlled trials. Front Pharmacol. (2021) 12:695832. doi: 10.3389/fphar.2021.804237
108. Wu, ZH, Zhu, X, Xu, CK, Chen, YJ, Zhang, L, and Zhang, CL. Effect of Xianling Gubao capsules on bone mineral density in osteoporosis patients. J Biol Regul Homeost Agents. (2017) 31:359–63.
109. Hung, TY, Chen, TL, Liao, MH, Ho, WP, Liu, DZ, Chuang, WC, et al. Promotes osteoblast maturation by inducing differentiation-related gene expression and protecting against oxidative stress-induced apoptotic insults. J Ethnopharmacol. (2010) 131:70–7. doi: 10.1016/j.jep.2010.05.063
110. Peng, CH, Lin, WY, Li, CY, Dharini, KK, Chang, CY, Hong, JT, et al. Gu Sui Bu (Drynaria fortunei J. SM.) antagonizes glucocorticoid-induced mineralization reduction in zebrafish larvae by modulating the activity of osteoblasts and osteoclasts. J Ethnopharmacol. (2022) 297:115565. doi: 10.1016/j.jep.2022.115565
111. Park, E, Lim, E, Yeo, S, Yong, Y, Yang, J, and Jeong, SY. Anti-menopausal effects of Cornus officinalis and Ribes fasciculatum extract in vitro and in vivo. Nutrients. (2020) 12:20369. doi: 10.3390/nu12020369
112. Xuan, Y, Wang, J, Zhang, X, Wang, J, Li, J, Liu, Q, et al. Resveratrol attenuates high glucose-induced osteoblast dysfunction via AKT/GSK3β/FYN-mediated NRF2 activation. Front Pharmacol. (2022) 13:862618. doi: 10.3389/fphar.2022.862618
113. Jiang, Y, Luo, W, Wang, B, Wang, X, Gong, P, and Xiong, Y. Resveratrol promotes osteogenesis via activating SIRT1/FoxO1 pathway in osteoporosis mice. Life Sci. (2020) 246:117422. doi: 10.1016/j.lfs.2020.117422
114. Zhao, L, Wang, Y, Wang, Z, Xu, Z, Zhang, Q, and Yin, M. Effects of dietary resveratrol on excess-iron-induced bone loss via antioxidative character. J Nutr Biochem. (2015) 26:1174–82. doi: 10.1016/j.jnutbio.2015.05.009
115. Wang, Y, Wang, WL, Xie, WL, Li, LZ, Sun, J, Sun, WJ, et al. Puerarin stimulates proliferation and differentiation and protects against cell death in human osteoblastic MG-63 cells via ER-dependent MEK/ERK and PI3K/Akt activation. Phytomedicine Int J Phytother Phytopharmacol. (2013) 20:787–96. doi: 10.1016/j.phymed.2013.03.005
116. Tiyasatkulkovit, W, Charoenphandhu, N, Wongdee, K, Thongbunchoo, J, Krishnamra, N, and Malaivijitnond, S. Upregulation of osteoblastic differentiation marker mRNA expression in osteoblast-like UMR106 cells by puerarin and phytoestrogens from Pueraria mirifica. Phytomed Int J Phytother Phytopharmacol. (2012) 19:1147–55. doi: 10.1016/j.phymed.2012.07.010
117. Song, L, Zhao, J, Zhang, X, Li, H, and Zhou, Y. Icariin induces osteoblast proliferation, differentiation and mineralization through estrogen receptor-mediated ERK and JNK signal activation. Eur J Pharmacol. (2013) 714:15–22. doi: 10.1016/j.ejphar.2013.05.039
118. Wu, Z, Ou, L, Wang, C, Yang, L, Wang, P, Liu, H, et al. Icaritin induces MC3T3-E1 subclone14 cell differentiation through estrogen receptor-mediated ERK1/2 and p38 signaling activation. Biomedicine & pharmacotherapy. (2017) 94:1–9. doi: 10.1016/j.biopha.2017.07.071
119. Bi, Z, Zhang, W, and Yan, X. Anti-inflammatory and immunoregulatory effects of icariin and icaritin. Biomed Pharmacother. (2022) 151:113180. doi: 10.1016/j.biopha.2022.113180
120. Deng, W, Ding, Z, Wang, Y, Zou, B, Zheng, J, Tan, Y, et al. Dendrobine attenuates osteoclast differentiation through modulating ROS/NFATc1/MMP9 pathway and prevents inflammatory bone destruction. Phytomed Int J Phytother Phytopharmacol. (2022) 96:153838. doi: 10.1016/j.phymed.2021.153838
121. Ni, S, Qian, Z, Yuan, Y, Li, D, Zhong, Z, Ghorbani, F, et al. Schisandrin a restrains osteoclastogenesis by inhibiting reactive oxygen species and activating Nrf2 signalling. Cell Prolif. (2020) 53:e12882. doi: 10.1111/cpr.12882
122. Xu, H, Chen, F, Liu, T, Xu, J, Li, J, Jiang, L, et al. Ellagic acid blocks RANKL-RANK interaction and suppresses RANKL-induced osteoclastogenesis by inhibiting RANK signaling pathways. Chem Biol Interact. (2020) 331:109235. doi: 10.1016/j.cbi.2020.109235
123. Rantlha, M, Sagar, T, Kruger, MC, Coetzee, M, and Deepak, V. Ellagic acid inhibits RANKL-induced osteoclast differentiation by suppressing the p38 MAP kinase pathway. Arch Pharm Res. (2017) 40:79–87. doi: 10.1007/s12272-016-0790-0
124. Han, SY, and Kim, YK. Berberine suppresses RANKL-induced osteoclast differentiation by inhibiting c-Fos and NFATc1 expression. Am J Chin Med. (2019) 47:439–55. doi: 10.1142/S0192415X19500228
125. Zeng, XZ, He, LG, Wang, S, Wang, K, Zhang, YY, Tao, L, et al. Aconine inhibits RANKL-induced osteoclast differentiation in RAW264.7 cells by suppressing NF-κB and NFATc1 activation and DC-STAMP expression. Acta Pharmacol Sin. (2016) 37:255–63. doi: 10.1038/aps.2015.85
126. Zhou, L, Liu, Q, Yang, M, Wang, T, Yao, J, Cheng, J, et al. Dihydroartemisinin, an anti-malaria drug, suppresses estrogen deficiency-induced osteoporosis, osteoclast formation, and RANKL-induced signaling pathways. J Bone Miner Res Off J Am Soc Bone Miner Res. (2016) 31:964–74. doi: 10.1002/jbmr.2771
127. Jing, X, Du, T, Chen, K, Guo, J, Xiang, W, Yao, X, et al. Icariin protects against iron overload-induced bone loss via suppressing oxidative stress. J Cell Physiol. (2019) 234:10123–37. doi: 10.1002/jcp.27678
128. Fang, J, Zhao, X, Li, S, Xing, X, Wang, H, Lazarovici, P, et al. Protective mechanism of artemisinin on rat bone marrow-derived mesenchymal stem cells against apoptosis induced by hydrogen peroxide via activation of c-Raf-Erk1/2-p90(rsk)-CREB pathway. Stem Cell Res Ther. (2019) 10:312. doi: 10.1186/s13287-019-1419-2
129. Zhao, B, Peng, Q, Poon, EHL, Chen, F, Zhou, R, Shang, G, et al. Leonurine promotes the osteoblast differentiation of rat BMSCs by activation of autophagy via the PI3K/Akt/mTOR pathway. Front Bioeng Biotechnol. (2021) 9:615191. doi: 10.3389/fbioe.2021.615191
130. Zhao, B, Peng, Q, Wang, D, Zhou, R, Wang, R, Zhu, Y, et al. Leonurine protects bone mesenchymal stem cells from oxidative stress by activating mitophagy through PI3K/Akt/mTOR pathway. Cells. (2022) 11:111724. doi: 10.3390/cells11111724
131. Xu, D, Xu, L, Zhou, C, Lee, WY, Wu, T, Cui, L, et al. Salvianolic acid B promotes osteogenesis of human mesenchymal stem cells through activating ERK signaling pathway. Int J Biochem Cell Biol. (2014) 51:1–9. doi: 10.1016/j.biocel.2014.03.005
132. Li, XD, Wang, JS, Chang, B, Chen, B, Guo, C, Hou, GQ, et al. Panax notoginseng saponins promotes proliferation and osteogenic differentiation of rat bone marrow stromal cells. J Ethnopharmacol. (2011) 134:268–74. doi: 10.1016/j.jep.2010.11.075
133. Wenxi, D, Shufang, D, Xiaoling, Y, and Liming, Y. Panax notoginseng saponins suppress radiation-induced osteoporosis by regulating bone formation and resorption. Phytomed Int J Phytother Phytopharmacol. (2015) 22:813–9. doi: 10.1016/j.phymed.2015.05.056
134. Zhu, B, Xue, F, Zhang, C, and Li, G. Ginkgolide B promotes osteoblast differentiation via activation of canonical WNT signalling and alleviates osteoporosis through a bone anabolic way. J Cell Mol Med. (2019) 23:5782–93. doi: 10.1111/jcmm.14503
135. Lee, CW, Lin, HC, Wang, BY, Wang, AY, Shin, RL, Cheung, SYL, et al. Ginkgolide B monotherapy reverses osteoporosis by regulating oxidative stress-mediated bone homeostasis. Free Radic Biol Med. (2021) 168:234–46. doi: 10.1016/j.freeradbiomed.2021.03.008
136. Tian, H, Jiang, T, Yang, K, Ning, R, Wang, T, Zhou, Q, et al. α-Asarone attenuates osteoclastogenesis and prevents against oestrogen-deficiency induced osteoporosis. Front Pharmacol. (2022) 13:780590. doi: 10.3389/fphar.2022.1053602
137. Yang, Y, Wei, Q, An, R, Zhang, HM, Shen, JY, Qin, XY, et al. Anti-osteoporosis effect of semen cuscutae in ovariectomized mice through inhibition of bone resorption by osteoclasts. J Ethnopharmacol. (2022) 285:114834. doi: 10.1016/j.jep.2021.114834
138. Yang, N, Zhang, X, Li, L, Xu, T, Li, M, Zhao, Q, et al. Ginsenoside RC promotes bone formation in ovariectomy-induced osteoporosis in vivo and osteogenic differentiation in vitro. Int J Mol Sci. (2022) 23:116187. doi: 10.3390/ijms23116187
139. Hu, Y, Mu, P, Ma, X, Shi, J, Zhong, Z, and Huang, L. Rhizoma drynariae total flavonoids combined with calcium carbonate ameliorates bone loss in experimentally induced osteoporosis in rats via the regulation of Wnt3a/β-catenin pathway. J Orthop Surg Res. (2021) 16:702. doi: 10.1186/s13018-021-02842-3
140. Ren, S, Jiao, G, Zhang, L, You, Y, and Chen, Y. Bionic Tiger-bone powder improves bone microstructure and bone biomechanical strength of ovariectomized rats. Orthop Surg. (2021) 13:1111–8. doi: 10.1111/os.12954
141. Qin, X, Wei, Q, An, R, Yang, Y, Cai, M, Han, X, et al. Regulation of bone and fat balance by Fructus Ligustri Lucidi in ovariectomized mice. Pharm Biol. (2023) 61:391–403. doi: 10.1080/13880209.2023.2168019
142. Lee, JH, Wei, YJ, Zhou, ZY, Hou, YM, Wang, CL, Wang, LB, et al. Efficacy of the herbal pair, radix achyranthis bidentatae and eucommiae cortex, in preventing glucocorticoid-induced osteoporosis in the zebrafish model. J Integr Med. (2022) 20:83–90. doi: 10.1016/j.joim.2021.11.003
143. Li, CR, Zhang, GW, Niu, YB, Pan, YL, Zhai, YK, and Mei, QB. Antiosteoporosis effect of radix scutellariae extract on density and microstructure of long bones in tail-suspended Sprague-dawley rats. Evidence-based complementary and alternative medicine: eCAM. (2013) 2013:753703. doi: 10.1155/2013/753703
144. Xi, HR, Ma, HP, Yang, FF, Gao, YH, Zhou, J, Wang, YY, et al. Total flavonoid extract of epimedium herb increases the peak bone mass of young rats involving enhanced activation of the AC10/cAMP/PKA/CREB pathway. J Ethnopharmacol. (2018) 223:76–87. doi: 10.1016/j.jep.2018.05.023
145. Shangguan, WJ, Zhang, YH, Li, ZC, Tang, LM, Shao, J, and Li, H. Naringin inhibits vascular endothelial cell apoptosis via endoplasmic reticulum stress- and mitochondrial-mediated pathways and promotes intraosseous angiogenesis in ovariectomized rats. Int J Mol Med. (2017) 40:1741–9. doi: 10.3892/ijmm.2017.3160
146. Liu, Y, Zhu, S, Liu, J, Chen, Y, Zhong, S, Lian, D, et al. Vitexin regulates angiogenesis and osteogenesis in ovariectomy-induced osteoporosis of rats via the VDR/PI3K/AKT/eNOS signaling pathway. J Agric Food Chem. (2023) 71:546–56. doi: 10.1021/acs.jafc.2c07005
147. Fan, D, Lu, J, Yu, N, Xie, Y, and Zhen, L. Curcumin prevents diabetic osteoporosis through promoting osteogenesis and angiogenesis coupling via NF-κB signaling. Evidence-based complementary and alternative medicine: eCAM. (2022) 2022:4974343. doi: 10.1155/2022/4974343
148. Chen, W, Jin, X, Wang, T, Bai, R, Shi, J, Jiang, Y, et al. Ginsenoside Rg1 interferes with the progression of diabetic osteoporosis by promoting type H angiogenesis modulating vasculogenic and osteogenic coupling. Front Pharmacol. (2022) 13:1010937. doi: 10.3389/fphar.2022.1010937
149. Cui, L, Li, T, Liu, Y, Zhou, L, Li, P, Xu, B, et al. Salvianolic acid B prevents bone loss in prednisone-treated rats through stimulation of osteogenesis and bone marrow angiogenesis. PLoS One. (2012) 7:e34647. doi: 10.1371/journal.pone.0034647
150. Li, L, Qu, Y, Jin, X, Guo, XQ, Wang, Y, Qi, L, et al. Protective effect of salidroside against bone loss via hypoxia-inducible factor-1α pathway-induced angiogenesis. Sci Rep. (2016) 6:32131. doi: 10.1038/srep32131
151. Okamoto, K, and Takayanagi, H. Osteoimmunology. Cold Spring Harbor Perspect Med. (2019) 9:a031245. doi: 10.1101/cshperspect.a031245
152. Yang, Y, Yu, T, Tang, H, Ren, Z, Li, Q, Jia, J, et al. Ganoderma lucidum immune modulator protein rLZ-8 could prevent and reverse bone loss in glucocorticoids-induced osteoporosis rat model. Front Pharmacol. (2020) 11:731. doi: 10.3389/fphar.2020.00731
153. Yu, Y, Chen, M, Yang, S, Shao, B, Chen, L, Dou, L, et al. Osthole enhances the immunosuppressive effects of bone marrow-derived mesenchymal stem cells by promoting the Fas/FasL system. J Cell Mol Med. (2021) 25:4835–45. doi: 10.1111/jcmm.16459
154. Azam, Z, Sapra, L, Baghel, K, Sinha, N, Gupta, RK, Soni, V, et al. Cissus quadrangularis (Hadjod) inhibits rankl-induced osteoclastogenesis and augments bone health in an estrogen-deficient preclinical model of osteoporosis via modulating the host Osteoimmune system. Cells. (2023, 20216) 12. doi: 10.3390/cells12020216
155. He, L, Duan, H, Li, X, Wang, S, Zhang, Y, Lei, L, et al. Sinomenine down-regulates TLR4/TRAF6 expression and attenuates lipopolysaccharide-induced osteoclastogenesis and osteolysis. Eur J Pharmacol. (2016) 779:66–79. doi: 10.1016/j.ejphar.2016.03.014
156. Li, X, Jiang, J, Yang, Z, Jin, S, Lu, X, and Qian, Y. Galangin suppresses RANKL-induced osteoclastogenesis via inhibiting MAPK and NF-κB signalling pathways. J Cell Mol Med. (2021) 25:4988–5000. doi: 10.1111/jcmm.16430
157. Gong, AG, Li, N, Lau, KM, Lee, PS, Yan, L, Xu, ML, et al. Calycosin orchestrates the functions of Danggui Buxue Tang, a Chinese herbal decoction composing of Astragali Radix and Angelica Sinensis Radix: An evaluation by using calycosin-knock out herbal extract. J Ethnopharmacol. (2015) 168:150–7. doi: 10.1016/j.jep.2015.03.033
158. Weerachayaphorn, J, Chuncharunee, A, Mahagita, C, Lewchalermwongse, B, Suksamrarn, A, and Piyachaturawat, P. A protective effect of Curcuma comosa Roxb. On bone loss in estrogen deficient mice. J Ethnopharmacol. (2011) 137:956–62. doi: 10.1016/j.jep.2011.06.040
159. Zheng, Y, Jin, Y, Zhu, HB, Xu, ST, Xia, YX, and Huang, Y. Effects of a Chinese medicinal plant Radix astragali on the ovariectomized female rats. Afric J Tradition Complement Alternative Med AJTCAM. (2012) 10:9–14.
160. Xu, Y, Ma, XP, Ding, J, Liu, ZL, Song, ZQ, Liu, HN, et al. Treatment with qibaomeiran, a kidney-invigorating Chinese herbal formula, antagonizes estrogen decline in ovariectomized rats. Rejuvenation Res. (2014) 17:372–81. doi: 10.1089/rej.2014.1557
161. Ko, NY, Chen, LR, and Chen, KH. The role of Micro RNA and long-non-coding RNA in osteoporosis. Int J Mol Sci. (2020) 21:144886. doi: 10.3390/ijms21144886
162. Hou, J, Liu, D, Zhao, J, Qin, S, Chen, S, and Zhou, Z. Long non-coding RNAs in osteoporosis: from mechanisms of action to therapeutic potential. Hum Cell. (2023) 36:950–62. doi: 10.1007/s13577-023-00888-5
163. Liu, TJ, Hu, S, Qiu, ZD, and Liu, D. Anti-tumor mechanisms associated with regulation of non-coding RNA by active ingredients of Chinese medicine: a review. Front Oncol. (2020) 10:634936. doi: 10.3389/fonc.2020.609512
164. Wang, ZQ, Li, JL, Sun, YL, Yao, M, Gao, J, Yang, Z, et al. Chinese herbal medicine for osteoporosis: a systematic review of randomized controlled trails. Evidence-based Complementary And Alternative Medicine: eCAM. (2013) 2013:356260. doi: 10.1155/2013/732562
165. Li, HM, Yang, W, Zhang, YL, and Zhi, YJ. Randomized controlled trial outcome indicators of postmenopausal osteoporosis treated by traditional Chinese medicine. Zhongguo Zhong yao za zhi China J Chinese Mater Med. (2021) 46:4274–86. doi: 10.19540/j.cnki.cjcmm.20210426.501
166. Wang, H, Yan, XP, and Kong, WP. Effect of bushen qiangdu recipe on osteoporosis and bone loss of patients with ankylosing spondylitis. Zhongguo Zhong xi yi jie he za zhi China J Integr Tradition Western Med. (2011) 31:471–5.
167. Leung, PC, Cheng, KF, and Chan, YH. An innovative herbal product for the prevention of osteoporosis. Chin J Integr Med. (2011) 17:744–9. doi: 10.1007/s11655-011-0876-y
Glossary
Keywords: osteoporosis, traditional Chinese medicine herbs, plant-based natural products, bone homeostasis, active ingredients, anti-osteoporosis drug
Citation: Zhou C, Shen S, Zhang M, Luo H, Zhang Y, Wu C, Zeng L and Ruan H (2023) Mechanisms of action and synergetic formulas of plant-based natural compounds from traditional Chinese medicine for managing osteoporosis: a literature review. Front. Med. 10:1235081. doi: 10.3389/fmed.2023.1235081
Edited by:
Ning Zhang, The Chinese University of Hong Kong, ChinaReviewed by:
Chaoran Liu, The Chinese University of Hong Kong, ChinaLiming Zhao, Stanford University, United States
Copyright © 2023 Zhou, Shen, Zhang, Luo, Zhang, Wu, Zeng and Ruan. This is an open-access article distributed under the terms of the Creative Commons Attribution License (CC BY). The use, distribution or reproduction in other forums is permitted, provided the original author(s) and the copyright owner(s) are credited and that the original publication in this journal is cited, in accordance with accepted academic practice. No use, distribution or reproduction is permitted which does not comply with these terms.
*Correspondence: Lingfeng Zeng, MTM0NzMwMTE3NUBxcS5jb20=; Hongfeng Ruan, cmhmQHpjbXUuZWR1LmNu
†These authors have contributed equally to this work