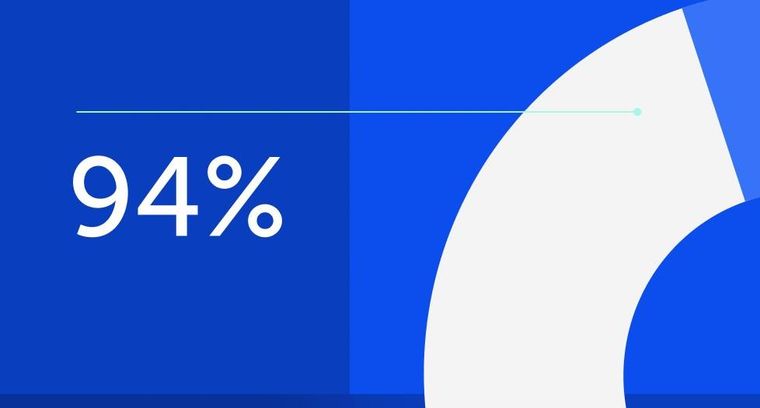
94% of researchers rate our articles as excellent or good
Learn more about the work of our research integrity team to safeguard the quality of each article we publish.
Find out more
PERSPECTIVE article
Front. Med., 03 August 2023
Sec. Pulmonary Medicine
Volume 10 - 2023 | https://doi.org/10.3389/fmed.2023.1232655
This article is part of the Research TopicEpidemiology and Risk Factors For Interstitial Lung DiseasesView all 13 articles
Within the wide scope of interstitial lung diseases (ILDs), familial pulmonary fibrosis (FPF) is being increasingly recognized as a specific entity, with earlier onset, faster progression, and suboptimal responses to immunosuppression. FPF is linked to heritable pathogenic variants in telomere-related genes (TRGs), surfactant-related genes (SRGs), telomere shortening (TS), and early cellular senescence. Telomere abnormalities have also been identified in some sporadic cases of fibrotic ILD. Air pollution and other environmental exposures carry additive risk to genetic predisposition in pulmonary fibrosis. We provide a perspective on how these features impact on screening strategies for relatives of FPF patients, interstitial lung abnormalities, ILD multi-disciplinary team (MDT) discussion, and disparities and barriers to genomic testing. We also describe our experience with establishing a familial interstitial pneumonia (FIP) clinic and provide guidance on how to identify patients with telomere dysfunction who would benefit most from genomic testing.
The scope of interstitial lung diseases (ILDs) is extremely wide (over 200 disorders). The most common fibrotic ILD is idiopathic pulmonary fibrosis (IPF) (1). Besides ILDs of a granulomatous origin (e.g., sarcoidosis) and those of a known cause (e.g., asbestosis), the classification for idiopathic interstitial pneumonias (IIPs) has been agreed 10 years ago and likely needs to be reviewed as new data emerges on the interplay between genetic and environmental factors (2).
The terminology to refer to cases of ILD with a familial predisposition can be confusing. While initial reports used the term “familial IPF,” this would not account for the heterogeneity of ILD diagnoses in affected family members (not all relatives being diagnosed with IPF) (3). A commonly used term is familial pulmonary fibrosis (FPF) which expands the definition to cases of fibrotic ILDs within the same family. In addition, familial interstitial pneumonia (FIP) applies to families in which two or more cases of IIPs are diagnosed; e.g. index case (also called proband) having IPF, and a sibling diagnosed with fibrotic non-specific interstitial pneumonia (NSIP) (3). Finally, we may even use “familial ILD” to expand the definition to pedigrees where some relatives have fibrotic IIPs, while others are diagnosed with non-IIP ILDs or non-fibrotic ILDs, as we have encountered such families in our practice.
Telomeres consist of non-coding DNA (TTAGGG) and associated proteins thought to maintain genomic integrity during repeated cell divisions. Critical TS can lead to diverse clinical syndromes (4). Dyskeratosis congenita is a prototype of a TS condition, which is described as a triad of oral leukoplakia, reticular pigmentation of the neck/upper chest and dysplastic nails (5). It can be associated with other features which may also be found in telomere-related FPF, such as bone marrow failure, myelodysplasia, and leukemia (6). TS syndrome can also be associated with immunodeficiency, neurological or retinal conditions (5, 7).
Telomere length decreases with age even in the absence of fibrotic lung pathology. However, this appears to be more pronounced in ILD patients (8). Those carrying a pathogenic variant (also called mutation) in a telomere-related gene (TRG) generally have shorter telomeres (<25th percentile) compared to age matched controls; while only 50% of ILD patients >60 years old and TERT, TERC or RTEL1 mutations had a telomere length > 10th percentile (9). Short telomere length is associated with worse clinical outcomes in fibrotic ILD (10–12) and poor tolerance to immunosuppressive treatments (13, 14).
Telomere abnormalities, including TS, have also been identified in sporadic cases of IPF and other forms of pulmonary fibrosis (PF) (10, 15, 16).
TRGs include TERT, TERC, RTEL1, PARN, DKC1, TINF2, NOP10, NHP2, ACD, NAF1, ZCCHC8, RPA, POT1 (5). TERT (telomerase reverse transcriptase) and TERC (telomerase RNA component) are components of the telomere complex which is a group of proteins and RNA that drive the addition of telomeric repeats (TTAGGG) to the ends of chromosomes and were the first TRGs identified in FPF (4).
The mean age of ILD diagnosis in patients with TRG mutation is approximately 58 years (16). Clinical features include a usual interstitial pneumonia (UIP) radiological pattern on chest CT which can be seen in up to 54–74% of ILD cases with TRG mutations although other radiological patterns can be present. Radiological and histological patterns can vary even within the same family (16–18). Hematological abnormalities, such as anemia (17–27%), macrocytosis (24–41%) or thrombocytopenia (8–54%) may be associated to ILD (16, 17). Patients with DKC1, TINF2 and TERC mutations are more likely to have hematological involvement compared to those with TERT, PARN or RTEL1 mutations (16). Asymptomatic liver function abnormalities (i.e., increased liver transaminases) can also be found in 5–27% TRG mutation patients and early hair greying is noted in 15–40% (11, 19).
Surfactant-related genes (SRGs) include SFTPC which has an autosomal dominant mode of inheritance with frequent de novo mutations, but low frequency in FPF cases (<5%) (5). ABCA3 is mostly associated with respiratory failure in the newborn but severe adult ILD has been reported. NKX2.1 encodes the protein TTF1 which regulates surfactant protein as well as ABCA3 gene transcription. While NKX2.1 mutation mostly causes neonatal disease it can result in an atypical UIP pattern in adults (5, 20–23). In childhood, SRG mutations are the most common, whereas in adults, TRG pathogenic variants are more common (5, 24).
The single-nucleotide polymorphism rs35705950 in the promoter of the gene encoding mucin 5B (MUC5B) was shown to be associated with both FIP and sporadic IPF (25). It showed a significant signal in large genome-wide association studies and has been associated with various fibrotic ILDs (10, 26–29). However, given the high prevalence of the MUC5B rs35705950 minor allele promoter variant and low penetrance, genotyping of the variant is not currently part of FPF gene investigations in most countries (5). Fortunately in the UK, MUC5B is listed in the FPF panel, as well as SRGs and TRGs, as part of the National Genomic Test Directory for rare and inherited disease (30).
In FPF, there is overall a 50% chance of inheriting a deleterious allele in first-degree relatives (autosomal dominant) (5). Penetrance is generally incomplete, higher for SRGs (24) compared to TRGs, and expressivity is variable. The absence of TRG mutations in a family member of an index case does not exclude risk of disease due to other telomere abnormalities. The average age of ILD onset is lower in SRG compared to TRG mutation carriers. Pulmonary-only disease is more common in patients with SRG versus TRG pathogenic variants (5).
Cellular senescence is characterized by a state of cell cycle arrest (31). PF is considered a disease of senescence (aging) with fibrogenesis requiring an interplay between genetic predisposition and repeated exogenous insults (i.e., environmental). Animal models have confirmed that increasing age and having mutations in TRGs (e.g., TERT) favor the development of fibrosis following infectious or noxious challenge. Immune processes can also be affected by cellular senescence (such as T cell dysfunction and shifting to Th17 responses leading to immune dysregulation and propensity to autoimmune responses) and environmental exposures can accelerate this process (31, 32). Genomic instability due to TERT or ABCA3 mutations and fibrogenic exposures can lead to lung epithelial cell phenotypes that correspond to age-induced senescence (33).
There is evidence that acute exacerbations of IPF may correlate to air pollution, possibly mediated by senescence in inflammatory cells (34, 35). Fibroblast senescence can be triggered directly by environmental stress as well as indirectly by neighboring senescent cells altering the cellular microenvironment (36, 37). The window when environmental challenge occurs, as well as the dose and repeated exposure, play a role in senescence and fibrotic responses (33, 38).
Air pollution may trigger alterations on the airway mucosal surface by overwhelming ciliary and macrophage clearance mechanisms leading to oxidative stress and transporting toxic metals and particulates into the blood stream (39). It has been associated with increased incidence and worse outcomes in IPF, but large cohorts are required to validate these findings (40). Tobacco smoke, ozone exposures leading to oxidative stress by generating reactive oxygen species, lead and nitrosamines have also been linked to TS (41). Some of these effects may occur prenatally, e.g., in mothers exposed to high PM2.5 (particulate matter with an aerodynamic diameter ≤ 2.5 μm) air pollution (42). The onset of acute exacerbations of IPF was linked to air pollution, notably increased ozone concentrations (35).
Long term cumulative exposure to air pollution can increase the incidence of IPF, which is additive to genetic predisposition (43). Single gene mutations are thought to have relatively small effects, hence polygenic risk scores (PRS) have been proposed (44). Data from a large epidemiological study of 433,738 participants seems to support a role for air pollution in the pathogenesis of IPF in individuals stratified by PRSs. Those with a high PRS (13 single nucleotide polymorphisms) exposed to airborne pollutants had the highest risk of IPF incidence compared to low air pollution and low PRS in terms of adjusted hazard ratios (95% CI): for NO2 = 3.94 (2.77–5.6), NOx = 3.08 (2.21–4.27), PM2.5 = 3.65 (2.6–5.13), and PM10 = 3.23 (2.32–4.5) (43).
Exposure of human bronchial epithelial cells to PM2.5 can lead to dose-dependent epigenomic modifications, altered telomerase activity and TS (45). IPF patients exhibit epigenomic modifications including widespread DNA methylation alterations in lung tissue which seem to occur near genes linked to PF (such as TOLLIP, NOTCH1, FBXO32) (46, 47). Resistance to fibroblast was linked to histone modifications, and fibroblast proliferation appears to be regulated by noncoding RNAs (40). Increased road traffic-derived PM10 exposure as measured by air pollution monitors in Beijing was associated with decreased histone H3 methylation (48). Increased PM10 exposure has also been associated with accelerated forced vital capacity (FVC) decline in IPF patients (34). Increased levels of both PM2.5 and PM10 were associated with higher IPF mortality (35).
Progressive pulmonary fibrosis (PPF) is the dominant phenotype seen in familial cases. There is evidence to suggest that FPF is worse, faster progressing and has higher mortality than sporadic fibrotic ILD, although data is incomplete and at times contradictory (5). In a small study, a 9.9% annual rate of FVC decline was seen in familial IPF patients compared to 4.9% in those with sporadic IPF (not statistically significant, p = 0.12) (49). The average annual rate of FVC decline amongst 115 ILD patients with TRG mutations (46% of whom had IPF) was found to be 300 ml (regardless of gene involved) (16). The mean survival from diagnosis in FPF appears to be between 2.4 and 7.3 years (18, 50). In a cohort of 1,262 ILD patients, survival was found to be worse in FPF compared to sporadic PF, both for IPF cases (HR for death or transplant of 1.8 [95% CI, 1.37–2.37]) and non-IPF ILD (HR for death or transplant of 2.08 [CI: 1.46–2.96]) (51). Regardless of the ILD diagnostic label, there was no difference in median survival comparing familial IPF to familial non-IPF (16).
As genomic tests can be expensive or only reimbursed when certain criteria are met, it is recommended to pre-screen patients based on family history and clinical features of TS prior to testing (Tables 1, 2) (5, 52). In our opinion, the easiest way to organize testing is by running dedicated FIP clinics. This allows to pool together patients with rare ILDs, screen relatives, offer personalized medicine and identify associated conditions (e.g., hematological diseases). More time for explanations can be given by a trained healthcare provider leading to a better patient experience. Genetic and psychological counselling, specialist ILD nursing and research can also be integrated within the FIP clinic structure.
Table 1. Clinical features of short telomere syndrome which can be elicited during consultations of patients affected by familial pulmonary fibrosis and their relatives.
Table 2. Eligibility for genomic testing for familial pulmonary fibrosis as per the latest version of UK National Genomic Test Directory for rare and inherited disease (53).
Appropriate counselling and a tactful approach are necessary because genomic testing can be seen as opening a “Pandora’s box.” It often leads to major changes in patients’ lives and those of their relatives. In our experience with the Manchester FIP clinic, most patients are extremely interested in genomic tests if the wider context is clearly explained, and a pathway exists to deal with possible results. Importantly, testing provides an answer to the question “Why did I get ILD?” which leads to better acceptance of the ILD diagnosis and treatment requirements. Many patients are also very interested in research projects.
In our experience, familial and sporadic cases with telomere dysfunction frequently have atypical ILD presentations, and this can delay referral to an ILD specialist center, accurate diagnosis, and timely treatment.
As FPF cases may progress faster than sporadic ones, more frequent follow-up with lung function monitoring and early lung transplant referral should be considered as part of the MDT discussion, ideally involving transplant specialists and genetic counsellors. Transplant outcomes between FPF and sporadic PF were comparable (54, 55), therefore knowledge of a familial aggregation, TS or identified mutation should not automatically be considered contraindications for lung transplantation (54). Special considerations should be given to personalizing immunosuppressive regimens and the post-transplant follow-up as patients with telomere dysfunction (TS and/or TRG mutation) may be at increased risk of complications (13, 14, 56, 57).
Antifibrotics are just as useful in FPF as in sporadic fibrotic ILDs (IPF and PPF). Immunosuppression can increase the risk of complications and efficacy may be limited in patients who require such treatments (e.g., to treat fibrotic hypersensitivity pneumonitis) (14). It would be reasonable to encourage smoking cessation and avoidance of other inhalational exposures.
Interstitial lung abnormalities (ILAs) on chest CT scanning are a common finding in relatives of FPF patients (5). They are defined as incidental findings of nondependent abnormalities which affect >5% of any lung zone (upper, middle, lower), where ILD was not previously suspected. Respiratory symptoms may be present, and this may indicate early ILD. Three subtypes of ILAs have been described: non-subpleural nonfibrotic, subpleural nonfibrotic, subpleural fibrotic (58, 59).
Previous studies have demonstrated a prevalence of ILAs on lung cancer screening of 4–20% (60–63). Recent data from Manchester found a prevalence of ILAs on low-dose chest CT scanning of 3.9%, and 40.7% of these individuals with ILAs were subsequently diagnosed with ILD within 5 years (64).
Interstitial lung abnormalities are associated with radiological progression and mortality (64). Having traction bronchiectasis or bronchiolectasis is an important radiological risk factor to predict adverse outcomes (65, 66).
There is no clear pathway or optimal age for screening relatives of FPF patients. As there is a 1–3-year delay between symptom onset and diagnosis for FPF patients, this represents an opportunity for early diagnosis (67).
It is recommended that all first-degree relatives of FPF patients should have a screening chest high-resolution CT (HRCT) scan especially if they have non-resolving respiratory symptoms (chronic cough and dyspnea) (5). The prevalence of interstitial lung changes on CT screening of first-degree relatives of FPF patients has been reported as 14–25% (5). Among first-degree relatives of FPF without overt ILD at screening, 19.4% developed extensive HRCT abnormalities or clinical ILD at 5 years. Also, 63.3% of patients with limited ILAs at enrolment experienced progression compared to only 6.1% of patients with normal HRCT at baseline (68).
Lung function is important for screening and follow-up, but simple spirometry may not identify early cases since FVC < 80% predicted in asymptomatic relatives is rare. However, relatives with ILAs have a lower diffusing capacity of the lung for carbon monoxide (DLCO) than those with normal HRCT (68, 69). Annual lung function testing may identify trends of decline. The role of home spirometry monitoring has not been explored in this population but may hold promise for the future.
Additionally, due to the high prevalence of other TS features, a full blood count (FBC) and liver function testing should also be performed in first-degree relatives (5).
Certain relatives may be more open to screening, while others would prefer to wait and clinicians should abide by their preferences, to not cause undue anxiety. Eligible relatives may also be offered genomic testing, usually via local/regional Clinical Genetics services who follow-up families of the proband.
To date, there is no consensus on the frequency and duration of clinical, functional, and radiological evaluations of first-degree relatives of FPF patients. Management could be customized through a risk assessment, considering resources available in each country/national health system.
Figure 1 outlines a potential screening strategy for relatives of FPF patients.
Figure 1. Proposed screening and monitoring pathway for first-degree relatives of familial pulmonary fibrosis (FPF) patients. HRCT, high resolution computed tomography; DLCO, diffusing capacity of the lung for carbon monoxide; FBC, full blood count; ILD, interstitial lung disease; ILA, interstitial lung abnormalities; IPF, idiopathic pulmonary fibrosis; NSIP, non-specific interstitial pneumonia.
Although patients with PF were found to have disproportionately short leukocyte telomere length (LTL), there was very little genetic variability in cohorts (to account for ethnic and socio-economic differences around the world). LTL decreased with age, more so in PF patients compared to controls (R = −0.28, p < 0.0001). It appears that sex-adjusted LTL below median is uniformly associated with chronological age and increased risk of mortality in all racial groups although in the Asian population it was not statistically significant (White = HR 2.21, [95%CI: 1.79–2.72], Black = HR 2.22 [1.05–4.66], Hispanic = HR 3.40 [1.88–6.14], Asian = HR 2.11 [0.55–8.23]) (8). Fibrotic lung disease seems to occur several years earlier in African American compared to White patients (70). Patients of Black ethnicity are less likely than those of White ethnicity to have lung transplantation and have worse outcomes even when controlling for confounding factors, socio-economic status (as per the area deprivation index – ADI), donor cause of death, blood type nor HLA mismatch (71, 72). Further research to determine if ethnic differences or socio-economic disparities drive outcomes in FPF are needed (73).
The availability of genomic testing, telomere length measurement and whole genome sequencing varies greatly around the world, making it difficult to standardize global recommendations. At the time of this writing, a 25-gene panel test for FPF is available in the UK, but telomere length measurement is not reimbursed by the health service (30). Local laws and practice regarding genetic counselling can also vary greatly around the world (74).
In our opinion, actively eliciting a family history of ILD and other rare conditions, as well as asking about TS features (Tables 1, 2) could be a cost-effective way of identifying relatives at risk of developing ILD. We hypothesize that FPF is more frequent than previously thought, but patients are rarely asked specifically about it during routine ILD consultations. We believe that dedicated FIP clinics can improve care through personalized medicine, early screening and diagnosis in “asymptomatic” relatives, genomic testing, rapid access to antifibrotic medication and early lung transplant referral. We also feel that environmental exposures should be elicited due to the additive risk in FPF (43), and this can be done by using standardized questionnaires (75).
The original contributions presented in the study are included in the article/supplementary material, further inquiries can be directed to the corresponding author.
SS and PR-O contributed equally to the conceptualization and literature search. JC contributed to summarizing the key points from the references. SS and PR-O structured the manuscript. SS, JC, and PR-O provided comments and contributed equally to finalizing the draft manuscript for submission. All authors contributed to the article and approved the submitted version.
The authors declare that the research was conducted in the absence of any commercial or financial relationships that could be construed as a potential conflict of interest.
All claims expressed in this article are solely those of the authors and do not necessarily represent those of their affiliated organizations, or those of the publisher, the editors and the reviewers. Any product that may be evaluated in this article, or claim that may be made by its manufacturer, is not guaranteed or endorsed by the publisher.
1. Wijsenbeek, M, Suzuki, A, and Maher, TM. Interstitial lung diseases. Lancet. (2022) 400:769–86. doi: 10.1016/S0140-6736(22)01052-2
2. Travis, WD, Costabel, U, Hansell, DM, King, TE Jr, Lynch, DA, Nicholson, AG, et al. An official American Thoracic Society/European Respiratory Society statement: update of the international multidisciplinary classification of the idiopathic interstitial pneumonias. Am J Respir Crit Care Med. (2013) 188:733–48. doi: 10.1164/rccm.201308-1483ST
3. Talbert, JL, Schwartz, DA, and Steele, MP. Familial interstitial pneumonia (FIP). Clin Pulm Med. (2014) 21:120–7. doi: 10.1097/CPM.0000000000000034
4. Armanios, M, and Blackburn, EH. The telomere syndromes. Nat Rev Genet. (2012) 13:693–704. doi: 10.1038/nrg3246
5. Borie, R, Kannengiesser, C, Antoniou, K, Bonella, F, Crestani, B, Fabre, A, et al. European Respiratory Society statement on familial pulmonary fibrosis. Eur Respir J. (2023) 61:2201383. doi: 10.1183/13993003.01383-2022
6. Savage SA NM. Dyskeratosis congenita (GeneReviews) GeneReviews (2009). Available at: https://www.ncbi.nlm.nih.gov/books/NBK22301/
7. Popescu, I, Mannem, H, Winters, SA, Hoji, A, Silveira, F, McNally, E, et al. Impaired cytomegalovirus immunity in idiopathic pulmonary fibrosis lung transplant recipients with short telomeres. Am J Respir Crit Care Med. (2019) 199:362–76. doi: 10.1164/rccm.201805-0825OC
8. Adegunsoye, A, Newton, CA, Oldham, JM, Ley, B, Lee, CT, Linderholm, AL, et al. Telomere length associates with chronological age and mortality across racially diverse pulmonary fibrosis cohorts. Nat Commun. (2023) 14:1489. doi: 10.1038/s41467-023-37193-6
9. Alder, JK, Hanumanthu, VS, Strong, MA, DeZern, AE, Stanley, SE, Takemoto, CM, et al. Diagnostic utility of telomere length testing in a hospital-based setting. Proc Natl Acad Sci U S A. (2018) 115:E2358–65. doi: 10.1073/pnas.1720427115
10. Ley, B, Newton, CA, Arnould, I, Elicker, BM, Henry, TS, Vittinghoff, E, et al. The MUC5B promoter polymorphism and telomere length in patients with chronic hypersensitivity pneumonitis: an observational cohort-control study. Lancet Respir Med. (2017) 5:639–47. doi: 10.1016/S2213-2600(17)30216-3
11. Alder, JK, Chen, JJ, Lancaster, L, Danoff, S, Su, SC, Cogan, JD, et al. Short telomeres are a risk factor for idiopathic pulmonary fibrosis. Proc Natl Acad Sci U S A. (2008) 105:13051–6. doi: 10.1073/pnas.0804280105
12. Stuart, BD, Lee, JS, Kozlitina, J, Noth, I, Devine, MS, Glazer, CS, et al. Effect of telomere length on survival in patients with idiopathic pulmonary fibrosis: an observational cohort study with independent validation. Lancet Respir Med. (2014) 2:557–65. doi: 10.1016/S2213-2600(14)70124-9
13. Newton, CA, Zhang, D, Oldham, JM, Kozlitina, J, Ma, SF, Martinez, FJ, et al. Telomere length and use of immunosuppressive medications in idiopathic pulmonary fibrosis. Am J Respir Crit Care Med. (2019) 200:336–47. doi: 10.1164/rccm.201809-1646OC
14. Adegunsoye, A, Morisset, J, Newton, CA, Oldham, JM, Vittinghoff, E, Linderholm, AL, et al. Leukocyte telomere length and mycophenolate therapy in chronic hypersensitivity pneumonitis. Eur Respir J. (2021) 57:2002872. doi: 10.1183/13993003.02872-2020
15. Cronkhite, JT, Xing, C, Raghu, G, Chin, KM, Torres, F, Rosenblatt, RL, et al. Telomere shortening in familial and sporadic pulmonary fibrosis. Am J Respir Crit Care Med. (2008) 178:729–37. doi: 10.1164/rccm.200804-550OC
16. Newton, CA, Batra, K, Torrealba, J, Kozlitina, J, Glazer, CS, Aravena, C, et al. Telomere-related lung fibrosis is diagnostically heterogeneous but uniformly progressive. Eur Respir J. (2016) 48:1710–20. doi: 10.1183/13993003.00308-2016
17. Diaz de Leon, A, Cronkhite, JT, Katzenstein, AL, Godwin, JD, Raghu, G, Glazer, CS, et al. Telomere lengths, pulmonary fibrosis and telomerase (TERT) mutations. PLoS One. (2010) 5:e10680. doi: 10.1371/journal.pone.0010680
18. Bennett, D, Mazzei, MA, Squitieri, NC, Bargagli, E, Refini, RM, Fossi, A, et al. Familial pulmonary fibrosis: clinical and radiological characteristics and progression analysis in different high resolution-CT patterns. Respir Med. (2017) 126:75–83. doi: 10.1016/j.rmed.2017.03.020
19. Diaz de Leon, A, Cronkhite, JT, Yilmaz, C, Brewington, C, Wang, R, Xing, C, et al. Subclinical lung disease, macrocytosis, and premature graying in kindreds with telomerase (TERT) mutations. Chest. (2011) 140:753–63. doi: 10.1378/chest.10-2865
20. Newton, CA, Oldham, JM, Applegate, C, Carmichael, N, Powell, K, Dilling, D, et al. The role of genetic testing in pulmonary fibrosis: a perspective from the Pulmonary Fibrosis Foundation genetic testing work group. Chest. (2022) 162:394–405. doi: 10.1016/j.chest.2022.03.023
21. Klay, D, Platenburg, M, van Rijswijk, R, Grutters, JC, and van Moorsel, CHM. ABCA3 mutations in adult pulmonary fibrosis patients: a case series and review of literature. Curr Opin Pulm Med. (2020) 26:293–301. doi: 10.1097/MCP.0000000000000680
22. Campo, I, Zorzetto, M, Mariani, F, Kadija, Z, Morbini, P, Dore, R, et al. A large kindred of pulmonary fibrosis associated with a novel ABCA3 gene variant. Respir Res. (2014) 15:43. doi: 10.1186/1465-9921-15-43
23. Nattes, E, Lejeune, S, Carsin, A, Borie, R, Gibertini, I, Balinotti, J, et al. Heterogeneity of lung disease associated with NK2 homeobox 1 mutations. Respir Med. (2017) 129:16–23. doi: 10.1016/j.rmed.2017.05.014
24. van Moorsel, CHM, van der Vis, JJ, and Grutters, JC. Genetic disorders of the surfactant system: focus on adult disease. Eur Respir Rev. (2021) 30:200085. doi: 10.1183/16000617.0085-2020
25. Seibold, MA, Wise, AL, Speer, MC, Steele, MP, Brown, KK, Loyd, JE, et al. A common MUC5B promoter polymorphism and pulmonary fibrosis. N Engl J Med. (2011) 364:1503–12. doi: 10.1056/NEJMoa1013660
26. Partanen, JJ, Häppölä, P, Zhou, W, Lehisto, AA, Ainola, M, Sutinen, E, et al. Leveraging global multi-ancestry meta-analysis in the study of idiopathic pulmonary fibrosis genetics. Cell Genom. (2022) 2:100181. doi: 10.1016/j.xgen.2022.100181
27. Juge, PA, Lee, JS, Ebstein, E, Furukawa, H, Dobrinskikh, E, Gazal, S, et al. MUC5B promoter variant and rheumatoid arthritis with interstitial lung disease. N Engl J Med. (2018) 379:2209–19. doi: 10.1056/NEJMoa1801562
28. Platenburg, M, Wiertz, IA, van der Vis, JJ, Crestani, B, Borie, R, Dieude, P, et al. The MUC5B promoter risk allele for idiopathic pulmonary fibrosis predisposes to asbestosis. Eur Respir J. (2020) 55:1902361. doi: 10.1183/13993003.02361-2019
29. Hunninghake, GM, Hatabu, H, Okajima, Y, Gao, W, Dupuis, J, Latourelle, JC, et al. MUC5B promoter polymorphism and interstitial lung abnormalities. N Engl J Med. (2013) 368:2192–200. doi: 10.1056/NEJMoa1216076
30. Pulmonary fibrosis familial. Pulmonary fibrosis familial (Version: 1.3). (2022). Genomics England. Available at: https://nhsgms-panelapp.genomicsengland.co.uk/panels/1174/v1.3
31. Goronzy, JJ, Hu, B, Kim, C, Jadhav, RR, and Weyand, CM. Epigenetics of T cell aging. J Leukoc Biol. (2018) 104:691–9. doi: 10.1002/JLB.1RI0418-160R
32. Baskara, I, Kerbrat, S, Dagouassat, M, Nguyen, HQ, Guillot-Delost, M, Surenaud, M, et al. Cigarette smoking induces human CCR6(+)Th17 lymphocytes senescence and VEGF-A secretion. Sci Rep. (2020) 10:6488. doi: 10.1038/s41598-020-63613-4
33. Venosa, A. Senescence in pulmonary fibrosis: between aging and exposure. Front Med (Lausanne). (2020) 7:606462. doi: 10.3389/fmed.2020.606462
34. Winterbottom, CJ, Shah, RJ, Patterson, KC, Kreider, ME, Panettieri, RA Jr, Rivera-Lebron, B, et al. Exposure to ambient particulate matter is associated with accelerated functional decline in idiopathic pulmonary fibrosis. Chest. (2018) 153:1221–8. doi: 10.1016/j.chest.2017.07.034
35. Sesé, L, Nunes, H, Cottin, V, Sanyal, S, Didier, M, Carton, Z, et al. Role of atmospheric pollution on the natural history of idiopathic pulmonary fibrosis. Thorax. (2018) 73:145–50. doi: 10.1136/thoraxjnl-2017-209967
36. Chen, X, Xu, H, Hou, J, Wang, H, Zheng, Y, Li, H, et al. Epithelial cell senescence induces pulmonary fibrosis through Nanog-mediated fibroblast activation. Aging (Albany NY). (2019) 12:242–59. doi: 10.18632/aging.102613
37. Waters, DW, Blokland, KEC, Pathinayake, PS, Wei, L, Schuliga, M, Jaffar, J, et al. STAT3 regulates the onset of oxidant-induced senescence in lung fibroblasts. Am J Respir Cell Mol Biol. (2019) 61:61–73. doi: 10.1165/rcmb.2018-0328OC
38. Chan, YL, Wang, B, Chen, H, Ho, KF, Cao, J, Hai, G, et al. Pulmonary inflammation induced by low-dose particulate matter exposure in mice. Am J Physiol Lung Cell Mol Physiol. (2019) 317:L424–30. doi: 10.1152/ajplung.00232.2019
39. Schraufnagel, DE, Balmes, JR, Cowl, CT, De Matteis, S, Jung, SH, Mortimer, K, et al. Air pollution and noncommunicable diseases: a review by the forum of international respiratory Societies' environmental committee, part 1: the damaging effects of air pollution. Chest. (2019) 155:409–16. doi: 10.1016/j.chest.2018.10.042
40. Goobie, GC, Nouraie, M, Zhang, Y, Kass, DJ, Ryerson, CJ, Carlsten, C, et al. Air pollution and interstitial lung diseases: defining Epigenomic effects. Am J Respir Crit Care Med. (2020) 202:1217–24. doi: 10.1164/rccm.202003-0836PP
41. Zhang, X, Lin, S, Funk, WE, and Hou, L. Environmental and occupational exposure to chemicals and telomere length in human studies. Occup Environ Med. (2013) 70:743–9. doi: 10.1136/oemed-2012-101350
42. Martens, DS, Cox, B, Janssen, BG, Clemente, DBP, Gasparrini, A, Vanpoucke, C, et al. Prenatal air pollution and Newborns' predisposition to accelerated biological aging. JAMA Pediatr. (2017) 171:1160–7. doi: 10.1001/jamapediatrics.2017.3024
43. Cui, F, Sun, Y, Xie, J, Li, D, Wu, M, Song, L, et al. Air pollutants, genetic susceptibility and risk of incident idiopathic pulmonary fibrosis. Eur Respir J. (2023) 61:2200777. doi: 10.1183/13993003.00777-2022
44. Huang, Y, Zhu, M, Ji, M, Fan, J, Xie, J, Wei, X, et al. Air pollution, genetic factors, and the risk of lung Cancer: a prospective study in the UK biobank. Am J Respir Crit Care Med. (2021) 204:817–25. doi: 10.1164/rccm.202011-4063OC
45. Leclercq, B, Platel, A, Antherieu, S, Alleman, LY, Hardy, EM, Perdrix, E, et al. Genetic and epigenetic alterations in normal and sensitive COPD-diseased human bronchial epithelial cells repeatedly exposed to air pollution-derived PM(2.5). Environ Pollut. (2017) 230:163–77. doi: 10.1016/j.envpol.2017.06.028
46. Alfano, R, Herceg, Z, Nawrot, TS, Chadeau-Hyam, M, Ghantous, A, and Plusquin, M. The impact of air pollution on our epigenome: how far is the evidence? (a systematic review). Curr Environ Health Rep. (2018) 5:544–78. doi: 10.1007/s40572-018-0218-8
47. Yang, IV, Pedersen, BS, Rabinovich, E, Hennessy, CE, Davidson, EJ, Murphy, E, et al. Relationship of DNA methylation and gene expression in idiopathic pulmonary fibrosis. Am J Respir Crit Care Med. (2014) 190:1263–72. doi: 10.1164/rccm.201408-1452OC
48. Zheng, Y, Sanchez-Guerra, M, Zhang, Z, Joyce, BT, Zhong, J, Kresovich, JK, et al. Traffic-derived particulate matter exposure and histone H3 modification: a repeated measures study. Environ Res. (2017) 153:112–9. doi: 10.1016/j.envres.2016.11.015
49. Krauss, E, Gehrken, G, Drakopanagiotakis, F, Tello, S, Dartsch, RC, Maurer, O, et al. Clinical characteristics of patients with familial idiopathic pulmonary fibrosis (f-IPF). BMC Pulm Med. (2019) 19:130. doi: 10.1186/s12890-019-0895-6
50. Lee, HL, Ryu, JH, Wittmer, MH, Hartman, TE, Lymp, JF, Tazelaar, HD, et al. Familial idiopathic pulmonary fibrosis: clinical features and outcome. Chest. (2005) 127:2034–41. doi: 10.1378/chest.127.6.2034
51. Cutting, CC, Bowman, WS, Dao, N, Pugashetti, JV, Garcia, CK, Oldham, JM, et al. Family history of pulmonary fibrosis predicts worse survival in patients with interstitial lung disease. Chest. (2021) 159:1913–21. doi: 10.1016/j.chest.2021.01.026
52. Molina-Molina, M, and Borie, R. Clinical implications of telomere dysfunction in lung fibrosis. Curr Opin Pulm Med. (2018) 24:440–4. doi: 10.1097/MCP.0000000000000506
53. Testing criteria for rare and inherited disease. (2023). Testing criteria for rare and inherited disease, version 5.1. NHS National Genomic Test Directory. Available at: https://www.england.nhs.uk/wp-content/uploads/2018/08/rare-and-inherited-disease-eligibility-criteria-v5.1.pdf.
54. Planas-Cerezales, L, Arias-Salgado, EG, Berastegui, C, Montes-Worboys, A, González-Montelongo, R, Lorenzo-Salazar, JM, et al. Lung transplant improves survival and quality of life regardless of telomere dysfunction. Front Med (Lausanne). (2021) 8:695919. doi: 10.3389/fmed.2021.695919
55. Alder, JK, Sutton, RM, Iasella, CJ, Nouraie, M, Koshy, R, Hannan, SJ, et al. Lung transplantation for idiopathic pulmonary fibrosis enriches for individuals with telomere-mediated disease. J Heart Lung Transplant. (2022) 41:654–63. doi: 10.1016/j.healun.2021.11.008
56. Stock, CJW, and Renzoni, EA. Telomeres in interstitial lung disease. J Clin Med. (2021) 10:1384. doi: 10.3390/jcm10071384
57. Phillips-Houlbracq, M, Mal, H, Cottin, V, Gauvain, C, Beier, F, Sicre de Fontbrune, F, et al. Determinants of survival after lung transplantation in telomerase-related gene mutation carriers: a retrospective cohort. Am J Transplant. (2022) 22:1236–44. doi: 10.1111/ajt.16893
58. Hata, A, Schiebler, ML, Lynch, DA, and Hatabu, H. Interstitial lung abnormalities: state of the art. Radiology. (2021) 301:19–34. doi: 10.1148/radiol.2021204367
59. Hatabu, H, Hunninghake, GM, Richeldi, L, Brown, KK, Wells, AU, Remy-Jardin, M, et al. Interstitial lung abnormalities detected incidentally on CT: a position paper from the Fleischner society. Lancet Respir Med. (2020) 8:726–37. doi: 10.1016/S2213-2600(20)30168-5
60. Jin, GY, Lynch, D, Chawla, A, Garg, K, Tammemagi, MC, Sahin, H, et al. Interstitial lung abnormalities in a CT lung cancer screening population: prevalence and progression rate. Radiology. (2013) 268:563–71. doi: 10.1148/radiol.13120816
61. Sverzellati, N, Guerci, L, Randi, G, Calabrò, E, La Vecchia, C, Marchianò, A, et al. Interstitial lung diseases in a lung cancer screening trial. Eur Respir J. (2011) 38:392–400. doi: 10.1183/09031936.00201809
62. Hoyer, N, Thomsen, LH, Wille, MMW, Wilcke, T, Dirksen, A, Pedersen, JH, et al. Increased respiratory morbidity in individuals with interstitial lung abnormalities. BMC Pulm Med. (2020) 20:67. doi: 10.1186/s12890-020-1107-0
63. Whittaker Brown, SA, Padilla, M, Mhango, G, Powell, C, Salvatore, M, Henschke, C, et al. Interstitial lung abnormalities and lung Cancer risk in the National Lung Screening Trial. Chest. (2019) 156:1195–203. doi: 10.1016/j.chest.2019.06.041
64. Balata, H, Punjabi, A, Chaudhuri, N, Greaves, M, Yorke, J, Booton, R, et al. The detection, assessment and clinical evolution of interstitial lung abnormalities identified through lung cancer screening. ERJ Open Res. (2023) 9:00632–2022. doi: 10.1183/23120541.00632-2022
65. Putman, RK, Gudmundsson, G, Axelsson, GT, Hida, T, Honda, O, Araki, T, et al. Imaging patterns are associated with interstitial lung abnormality progression and mortality. Am J Respir Crit Care Med. (2019) 200:175–83. doi: 10.1164/rccm.201809-1652OC
66. Hida, T, Nishino, M, Hino, T, Lu, J, Putman, RK, Gudmundsson, EF, et al. Traction bronchiectasis/bronchiolectasis is associated with interstitial lung abnormality mortality. Eur J Radiol. (2020) 129:109073. doi: 10.1016/j.ejrad.2020.109073
67. Fernandez, BA, Fox, G, Bhatia, R, Sala, E, Noble, B, Denic, N, et al. A Newfoundland cohort of familial and sporadic idiopathic pulmonary fibrosis patients: clinical and genetic features. Respir Res. (2012) 13:64. doi: 10.1186/1465-9921-13-64
68. Salisbury, ML, Hewlett, JC, Ding, G, Markin, CR, Douglas, K, Mason, W, et al. Development and progression of radiologic abnormalities in individuals at risk for familial interstitial lung disease. Am J Respir Crit Care Med. (2020) 201:1230–9. doi: 10.1164/rccm.201909-1834OC
69. Hunninghake, GM, Quesada-Arias, LD, Carmichael, NE, Martinez Manzano, JM, Poli De Frías, S, Baumgartner, MA, et al. Interstitial lung disease in relatives of patients with pulmonary fibrosis. Am J Respir Crit Care Med. (2020) 201:1240–8. doi: 10.1164/rccm.201908-1571OC
70. Adegunsoye, A, Oldham, JM, Bellam, SK, Chung, JH, Chung, PA, Biblowitz, KM, et al. African-American race and mortality in interstitial lung disease: a multicentre propensity-matched analysis. Eur Respir J. (2018) 51:1800255. doi: 10.1183/13993003.00255-2018
71. Lehr, CJ, Valapour, M, Gunsalus, PR, McKinney, WT, Berg, KA, Rose, J, et al. Association of Socioeconomic Position with Racial and Ethnic Disparities in survival after lung transplant. JAMA Netw Open. (2023) 6:e238306. doi: 10.1001/jamanetworkopen.2023.8306
72. Riley, LE, and Lascano, J. Gender and racial disparities in lung transplantation in the United States. J Heart Lung Transplant. (2021) 40:963–9. doi: 10.1016/j.healun.2021.06.004
73. Adegunsoye, A, and Saunders, MR. Racial disparities among lung transplant survivors-shadows and substance. JAMA Netw Open. (2023) 6:e238285. doi: 10.1001/jamanetworkopen.2023.8285
74. Abacan, M, Alsubaie, L, Barlow-Stewart, K, Caanen, B, Cordier, C, Courtney, E, et al. The global state of the genetic counselling profession. Eur J Hum Genet. (2019) 27:183–97. doi: 10.1038/s41431-018-0252-x
Keywords: familial pulmonary fibrosis, familial interstitial pneumonia, telomere shortening, interstitial lung disease, environment, genetics, FPF, FIP
Citation: Stanel SC, Callum J and Rivera-Ortega P (2023) Genetic and environmental factors in interstitial lung diseases: current and future perspectives on early diagnosis of high-risk cohorts. Front. Med. 10:1232655. doi: 10.3389/fmed.2023.1232655
Received: 31 May 2023; Accepted: 24 July 2023;
Published: 03 August 2023.
Edited by:
Niranjan Jeganathan, Loma Linda University, United StatesReviewed by:
Roberto Carbone, University of Genoa, ItalyCopyright © 2023 Stanel, Callum and Rivera-Ortega. This is an open-access article distributed under the terms of the Creative Commons Attribution License (CC BY). The use, distribution or reproduction in other forums is permitted, provided the original author(s) and the copyright owner(s) are credited and that the original publication in this journal is cited, in accordance with accepted academic practice. No use, distribution or reproduction is permitted which does not comply with these terms.
*Correspondence: Stefan Cristian Stanel, U3RlZmFuLlN0YW5lbEBtZnQubmhzLnVr
Disclaimer: All claims expressed in this article are solely those of the authors and do not necessarily represent those of their affiliated organizations, or those of the publisher, the editors and the reviewers. Any product that may be evaluated in this article or claim that may be made by its manufacturer is not guaranteed or endorsed by the publisher.
Research integrity at Frontiers
Learn more about the work of our research integrity team to safeguard the quality of each article we publish.