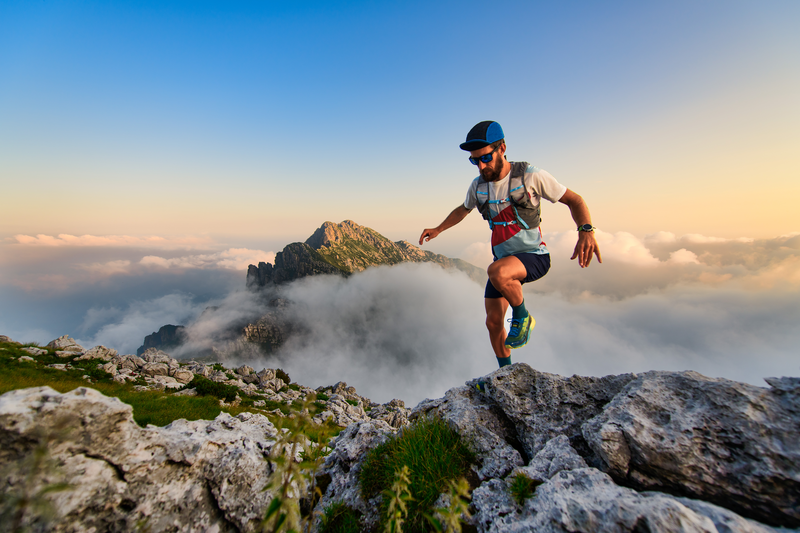
94% of researchers rate our articles as excellent or good
Learn more about the work of our research integrity team to safeguard the quality of each article we publish.
Find out more
REVIEW article
Front. Med. , 29 June 2023
Sec. Rheumatology
Volume 10 - 2023 | https://doi.org/10.3389/fmed.2023.1217147
This article is part of the Research Topic New insights into Interferons and Proinflammatory Cytokines in Lupus View all 7 articles
Aberrant activation of the Janus kinase (JAK) and signal transducer and activator of transcription (STAT) pathway is common in systemic lupus erythematosus (SLE), conferring immune-mediated properties in target tissues. Multiple cytokines activate different combinations of JAKs and STATs to alter the cell fate of target tissue and induce end-organ damage. Thus, the simultaneous blockade of several different cytokines by small molecules acting downstream intracellular signalling has gained traction. JAK inhibitors have been approved for the treatment of several rheumatic diseases, yet hitherto not for SLE. Nevertheless, JAK inhibitors including tofacitinib, baricitinib, and deucravacitinib have shown merit as treatments for SLE. Tofacitinib, a JAK1/3 inhibitor, reduced cholesterol levels, improved vascular function, and decreased the type I interferon signature in SLE patients. Baricitinib, a JAK1/2 inhibitor, demonstrated significant improvements in lupus rashes and arthritis in a phase 2 and a phase 3 randomised controlled trial, but the results were not replicated in another phase 3 trial. Deucravacitinib, a selective tyrosine kinase 2 (TYK2) inhibitor, yielded greater response rates than placebo in a phase 2 trial of SLE and will be investigated in larger phase 3 trials. TYK2 is activated in response to cytokines actively involved in lupus pathogenesis; this review highlights the potential of targeting TYK2 as a promising therapy for SLE.
Systemic lupus erythematosus (SLE) is a chronic autoimmune disease that affects multiple organs, leading to detrimental effects on patients’ quality of life and survival (1). Although its pathogenesis is complex and not yet fully understood, multiple cytokines are believed to underlie pathogenetic pathways. Due to the multifactorial aetiology and heterogeneity of the disease, numerous drugs have been tested in clinical trials with inadequate efficacy (2).
Over the last few decades, several biologic therapies have been developed to target specific inflammatory molecules and pathways. These drugs have shown encouraging results in rheumatic diseases, particularly rheumatoid arthritis (RA), psoriatic arthritis, and ankylosing spondylitis (3). However, many biologics fail to induce short- and long-term remission in rheumatic diseases, which combined with the potential adverse effects underscores the need for new therapies (4). In recent years, small molecules blocking intracellular downstream signalling molecules have shown capacity to change the natural history of several diseases (5).
Janus kinases (JAKs) are intracellular tyrosine kinases that mediate cytokine-mediated signalling via the phosphorylation of other proteins, particularly signal transducer and activator of transcription (STAT) proteins (6). There exist four JAK family members, i.e., JAK1, JAK2, JAK3, and tyrosine kinase 2 (TYK2), and seven different types of STAT proteins, i.e., STAT1, STAT2, STAT3, STAT4, STAT5a, STAT5b, and STAT6. Upon the binding of a ligand, e.g., a cytokine, to a receptor, JAKs activate STAT proteins through phosphorylation (dimers), allowing them to translocate to the nucleus and induce transcription of target genes; this describes the so-called JAK–STAT pathway (7) (Figure 1). The STAT dimers bind promoters of specific genes that control biological processes such as differentiation, proliferation, and apoptosis (6). Notably, each cytokine may activate different JAKs, while each JAK can interact with various STAT proteins, as shown in Table 1.
Figure 1. JAK–STAT pathway signalling and key cytokines involved in systemic lupus erythematosus pathogenesis.
Table 1. Pro-inflammatory cytokines involved in systemic lupus erythematosus pathogenesis and associated Janus kinase (JAK) and signal transduction and activator of transcription (STAT) proteins.
The JAK–STAT pathway is involved in the control of various biological processes, such as haematopoiesis, stem cell fate, and inflammatory response (6). In autoimmune diseases, multiple circulating cytokines activate different combinations of JAKs/STATs to alter the cell fate of target tissues and induce end-organ damage (3). We herein overview the involvement of the JAK–STAT pathway in the pathogenesis of SLE and discuss therapeutic options targeting this pathway, with an emphasis on TYK2 inhibition.
SLE is characterised by a plethora of circulating pro-inflammatory cytokines including interferon (IFN)-α, IFN-γ, interleukin (IL)-2, IL-6, IL-10, IL-12/IL-23, IL-19, IL-20, and IL-22 (8). These cytokines orchestrate autoimmunity by pleiotropic mechanisms, particularly through differentiation of immune cells to an autoreactive stage, e.g., IL-6 promoting Th17 formation (9), antibody production, e.g., IFN-α exaggerating formation of plasmablasts (10), and rapid proliferation of autoreactive cells. Although each one of these cytokines exerts several unique inflammatory functions, they use common JAKs and STAT proteins to transduce intracellular signals (3). Thus, the simultaneous blockade of many different cytokines by small molecules acting downstream on intracellular signalling gained traction a few years ago, and JAK inhibitors are now approved for the treatment of several rheumatic diseases, yet hitherto not for SLE (11).
To date, two targeted biologic therapies have been approved for the treatment of SLE, i.e., belimumab and anifrolumab (12). However, many biologics failed to reach the primary outcomes in a multitude of clinical trials, partly owing to the multiple and different immune-mediated pathways that are involved in lupus pathogenesis. Hence, the JAK–STAT pathway provides a rational target since it is activated by several cytokines in underlying aetiology, particularly interferons.
IFN-α is a key cytokine in SLE and other immune-mediated diseases, and its signalling is mediated by the intracellular kinases JAK1 and TYK2 (13) (Figure 1). Tofacitinib, a JAK1/JAK3 inhibitor, yielded some improvement in SLE patients in a phase 1 clinical trial (14). However, baricitinib, a JAK1/JAK2 inhibitor, failed to improve SLE disease activity in one of two randomised phase 3 trials (15, 16). To this end, a selective TYK2 inhibitor (deucravacitinib) has been developed and is being tested for its efficacy in autoimmune diseases, including SLE (17). Deucravacitinib selectively targets TYK2, which is activated in response to IFN-α, IL-6, IL-10, and IL-12/23. Importantly, all these cytokines are actively involved in lupus pathogenesis. Thus, TYK2 inhibition theoretically represents an intriguing therapeutic option for SLE.
New Zealand black/New Zealand white F1 (NZB/W-F1) and Murphy Roths Large/lymphoproliferation (MRL/lpr) murine models of SLE are commonly used to study pathogenetic aspects of lupus nephritis (LN). Experimental studies have shown that IFNs induce activation of STAT1 in glomerular mesangial cells (18), which is consistent with the increased expression of STAT1 seen in glomerular and tubular cells of LN kidney biopsies (19). The administration of a JAK2-STAT1 inhibitor in MRL/lpr mice has been shown to substantially improve lupus disease activity with respect to nephritis, vasculitis, and sialadenitis. Specifically, JAK2-STAT1 inhibition led to decreased levels of cytokines, chemokines, and IgG/C3 depositions in glomerular cells, along with improvements in serological markers of renal function (20). Early treatment with this inhibitor could prevent the establishment of LN in the same murine model, while the administration of JAK2-STAT1 inhibitors in NZB/W-F1 mice with established renal disease increased overall survival, decreased proteinuria, and improved histological renal features (21). Mechanistically, decreased expression of pSTAT3 and IFN-related genes (Mx1, Stat1, Isg15, and Ifit1) along with a profound reduction in the proportion of long-lived plasma cells in both spleen and bone marrow may account for the therapeutic efficacy of JAK2 inhibitors (22, 23).
Another experimental study tested the effectiveness of tofacitinib in the NZB/W-F1 model with regard to renal disease. Tofacitinib-treated mice showed renal histological improvements, decreased C3 and IgG glomerular deposits, significant reductions in the proportion of macrophages and T cells, and a decreased production of pro-inflammatory cytokines. The efficacy of tofacitinib was comparable to that of cyclophosphamide and mycophenolate mofetil (24). The potential therapeutic effect of tofacitinib in LN was validated in the MRL/lpr model of lupus (25). Another inhibitor targeting JAK1 and STAT3 showed ability to prevent LN development when given prophylactically and improve disease activity in established LN (26).
STAT3 is believed to play a pivotal role in LN pathogenesis. Indeed, STAT3 knock-out lupus-prone mice develop milder renal disease with regard to immune component infiltration and deposits in the kidneys (27). In addition, selective STAT3 inhibition has been shown to ameliorate LN both in terms of glomerulonephritis and tubulointerstitial inflammation in murine models of lupus (28). Although several experimental studies suggest that the JAK–STAT pathway represents a promising target in LN, clinical studies are required to confirm this hypothesis.
IFN signalling is an important mediator of cutaneous lupus (29). Ruxolitinib, a relatively specific inhibitor of JAK1 and JAK2, was administered to the MLR/lpr model to investigate its effect on lupus-related skin lesions. This study showed that ruxolitinib ameliorated cutaneous lupus by reducing the expression of IFN-related genes, immune cell infiltration (particularly T cells), and epidermal hyperplasia. However, no favourable effects were reported in other target organs (19). Ruxolitinib has also been successfully used to treat a patient with chilblain lupus (30).
Inhibition of the JAK–STAT pathway is associated with a decrease in autoantibody titres. More specifically, lupus-prone mice treated with JAK2-STAT1 inhibitors, JAK1-STAT3 inhibitors, selective STAT3 inhibitors, and tofacitinib showed reduced serum levels of anti-dsDNA antibodies (20, 24, 27). Across different murine models of lupus treated with JAK/STAT inhibitors, other antibodies such as antinuclear antibodies, anti-Smith, and anti-snRNP were also found to decrease (23, 26). Moreover, STAT3 and JAK2 inhibitors have been shown to increase serum C3 levels in the MRL/lpr model (22).
The TYK2 SNP rs34536443 (TYK2P) has been shown to confer a protective effect against autoimmunity (31). Humans expressing this SNP exhibit decreased switched memory B cells, T follicular helper cells, and IFN-mediated signalling. The underlying mechanism has been investigated in knock-out murine models for this allele (32). Homozygous mice for TYK2P have been shown to exhibit decreased T cell IL-12/IL-23-mediated signalling and Th1 and Th17 differentiation. These mice produce decreased proportions of IL-17+/IFN-γ + CD4+ T cells in draining lymph nodes. Taken together, this study confirms the protective role of TYK2P by reducing IL-12, IL-23, and IFN-α signalling.
Another interesting experimental study showed that the suppressor of T cell receptor signalling 1 (STS-1) tyrosine phosphatase was overexpressed in lupus B cells and indirectly activated the JAK–STAT pathway to induce IFN-α-mediated autophagy. Specifically, the activation of the JAK–STAT pathway was mediated by IFN-α-induced phosphorylation of TYK2 as a result of decreased phosphorylation of the E3 ubiquitin protein ligase c-cbl by STS-1. Thus, TYK2 seems to play an important role in the increased autophagy activity of SLE B cells (33).
TYK2 has also been implicated in neuropsychiatric SLE (NPSLE). Recent studies in the field demonstrated that microglia cells are activated towards an inflammatory state involving the IL12/IL23p40 pathway in diffuse NPSLE (34). Specifically, IL-12/23p40 signalling mediated neuropsychiatric changes in systemic lupus via TYK2 phosphorylation in the median prefrontal cortex (35). These findings have important clinical implications that point to TYK2 inhibitors as a potential drug for diffuse NPSLE.
Specific mutations or polymorphisms in JAK or STAT genes have been associated with various disorders, including immunodeficiencies, haematological conditions, and autoimmune diseases (3). This is an expected association since multiple cytokines and other mediators act through this pathway. Regarding rheumatic diseases, JAK2 polymorphisms are common in patients with Beçhet’s disease, while STAT3 and STAT6 polymorphisms have been associated with RA (36, 37). Notably, STAT4 polymorphisms have been linked to SLE susceptibility, and particularly with severe renal insufficiency in LN (38, 39).
Ex vivo studies in human SLE have shown that the JAK–STAT pathway upregulates the expression of IFN-regulated factor (IRF)-related genes in lupus T cells (40). Another study demonstrated that plasma cell niche cytokines, including IL-6, IL21, CXCL2, BAFF, and APRIL, enhance the production of autoantibodies and IgG in SLE-derived antibody-secreting cells in a STAT3-dependent manner (41). Further ex vivo studies are required to determine the role of the JAK–STAT pathway in human SLE.
TYK2 polymorphisms have been implicated as susceptibility factors for SLE, particularly cutaneous SLE manifestations (42, 43). More specifically, the TYK2 s2304256 polymorphism has been associated with SLE in individuals of European ancestry (44) while the rs280519 polymorphism has been linked to SLE in patients of both Asian and Caucasian ancestry. Other TYK2 single-nucleotide polymorphisms (SNPs) and gene–gene interactions between TYK2 and IRF5 have been detected in Han Chinese SLE patients (45). However, the association between TYK2 polymorphisms and susceptibility to SLE has not been confirmed in all populations (46, 47). Additionally, the SNP rs34536443 confers a protective role against autoimmunity (31).
Mechanistically, cutaneous lupus erythematosus (CLE) is initiated by keratinocytes producing IFN-κ, which further promotes IFN responses and increases the keratinocyte sensitivity to UV light. This mechanism is dependent on the expression of TYK2 (48). Authors have speculated that TYK2 mediates CLE via the regulation of autocrine IFN-κ activity, but further studies are required to prove this hypothesis. Another study showed that the STAT4 risk allele rs7574865[T] was associated with an elevated expression of IL-12 inducing IFN-γ production in lupus T cells. This mechanism was dependent on TYK2, as its inhibition reversed IFN-γ-induced activation of immune cells (49).
Numerous translational studies conducted on murine models of lupus have demonstrated the robust therapeutic efficacy of tofacitinib, particularly for various manifestations such as cardiovascular disease. These findings led to the first phase 1 double-blinded safety trial, which yielded encouraging results (14). In this study, thirty patients diagnosed with SLE were randomly assigned to receive tofacitinib 5 mg or placebo (2:1) twice daily. The primary endpoints of the trial were the safety and tolerability of the drug, while secondary outcomes focused on its clinical efficacy and mechanistic studies. The results showed that treatment with tofacitinib was associated with a decrease in high-density lipoprotein cholesterol levels, cholesterol efflux capacity, and lecithin, as well as improvements in endothelium-dependent vasorelaxation and arterial stiffness. Furthermore, the drug decreased the type I IFN signature, low-density granulocytes, and circulating neutrophil extracellular traps (NETs), which were considered as mechanistic outcomes. It is noteworthy that greater drug potency was observed in SLE patients with the STAT4 risk allele.
A case series comprising ten SLE patients with active skin and/or musculoskeletal disease demonstrated adequate efficacy of tofacitinib at a dose of 5 mg twice daily throughout a follow-up period of up to 12 months (50). Seven out of ten patients achieved clinical remission with a rapid response of lupus arthritis and a partial improvement of skin rashes. However, there was no significant change in serological markers. Nonetheless, it is essential to note that this study involved a limited number of patients and had a short observation period.
Baricitinib is an oral, selective inhibitor of JAK1/JAK2 that has been approved for the treatment of immune-mediated diseases, including COVID-19 (51). The first phase 2 randomised controlled trial (RCT) of baricitinib in SLE evaluated baricitinib in combination with standard of care and demonstrated efficacy in minimising lupus disease activity (52). The study included 314 SLE patients with active skin or joint disease, who were randomised to receive baricitinib at 2 different doses (2 mg or 4 mg) or placebo. The primary endpoint was the resolution of cutaneous or joint manifestations at week 24 according to the Systemic Lupus Erythematosus Disease Activity Index 2000 (SLEDAI-2K). In this study, the 4 mg dose of baricitinib exhibited clinical improvement with respect to lupus rashes and arthritis, with no new safety concerns raised during the trial. Early post-hoc analyses showed that baricitinib reduced both anti-dsDNA titres and key pro-inflammatory cytokines that are upregulated in SLE, suggesting a multitargeted mechanism of action (53, 54).
Based on the encouraging results of the phase 2 RTC of baricitinib, two phase 3 RTCs were designed to evaluate the potential efficacy of baricitinib in SLE, named SLE-BRAVE 1 and SLE-BRAVE 2, involving 760 and 775 SLE patients, respectively (15, 16). Participants were randomly assigned 1:1:1 to receive 2 mg or 4 mg of baricitinib or placebo once daily for 52 weeks. The primary endpoint was SLE Responder Index 4 (SRI-4) response at week 52 in the 4 mg baricitinib group compared with placebo. SLE-BRAVE 1 met its primary endpoint for the baricitinib 4 mg arm. However, the findings were not replicated in SLE-BRAVE 2. No safety concerns were reported in either one of the trials. Despite the mixed results from the phase 3 trials of baricitinib, JAK inhibitors still have merit as potential drugs for treating SLE, and further studies are needed to fully evaluate their efficacy and safety.
In a phase 1 clinical trial that involved both human samples and animal models of LN, the administration of an oral TYK2 inhibitor (BMS-986165) was shown to block multiple autoimmune pathways in human Th1, Th17, B cells, and myeloid cells, indicating a therapeutic potential (55). Increasing evidence for the efficacy of JAK inhibitors in other immune-mediated diseases and murine studies of lupus led to the first RCT of deucravacitinib, an oral, selective, allosteric TYK2 inhibitor, in SLE (17). This study was a phase 2, dose-determination trial that included 363 patients with active SLE. The primary endpoint was SRI-4 response at week 32, while secondary outcomes included attainment of Lupus Low Disease Activity State (LLDAS), British Isles Lupus Assessment Group-based Composite Lupus Assessment (BICLA) response, Cutaneous Lupus Erythematosus Disease Area and Severity Index 50 (CLASI-50), improvements in arthritis (swollen and tender joints), and SRI-4 response at week 48. Treatment with deucravacitinib was associated with higher SRI-4 response frequencies compared with placebo. Moreover, deucravacitinib was linked to better outcomes in terms of LLDAS, BICLA, CLASI-50, and joint counts. The optimal dose of deucravacitinib was found to be 3 mg twice daily, and the safety profile of the drug was acceptable. The potential efficacy of deucravacitinib will be investigated in larger phase 3 RTCs (NCT05620407, NCT05617677).
Design of RCTs and key outcomes are summarised in Table 2.
Table 2. Randomized clinical trials of Janus kinase (JAK) inhibitors in systemic lupus erythematosus.
In a post-hoc analysis of the ORAL surveillance trial, it was found that RA patients with cardiovascular risk factors who were treated with tofacitinib had a higher likelihood of exhibiting a cardiovascular event, including myocardial infarction, stroke, and death due to cardiovascular disease (56). Additionally, these patients had a higher risk of developing non-melanoma skin cancer compared to RA patients treated with TNF-α inhibitors (56). This observation led to several real-world studies assessing the potential side effects of JAK inhibitors. Notably, a large population-based study involving 102,263 participants found no enhanced risk for cardiovascular events in RA patients conferred from tofacitinib treatment compared to treatment with anti-TNF agents (58). The same study revealed no association between the use of tofacitinib and the occurrence of any type of cancer (excluding non-melanoma skin cancer) (58). A meta-analysis including 29 RCTs showed no increased likelihood of either cardiovascular events or all-cause mortality in patients with autoimmune diseases treated with tofacitinib (59). However, real-world data from the national Swedish registry indicated that RA patients treated with tofacitinib or baricitinib are at increased risk for venous thromboembolism compared with those treated with other biologic disease-modifying anti-rheumatic drugs (DMARDs) (57). While JAK inhibitors may hold promise as potential therapies for SLE, it is essential to approach these findings with prudence owing to the heightened propensity of such agents to trigger cardiovascular events as an adverse effect, particularly in view of established knowledge that SLE patients have an increased risk of atherosclerosis and cardiovascular disease (60). To this end, it is worth mentioning that the safety of JAK inhibitors used to treat various autoimmune diseases is currently under investigation by the Pharmacovigilance Risk Assessment Committee (PRAC).
Unfortunately, the SLE community has witnessed a multitude of failures of phase 2 and 3 clinical trials (61). However, during the last two decades we have also witnessed drug development that has resulted in licenced drugs (12). Recent advancements concerning JAK inhibition have yielded promising results for the treatment of autoimmune diseases. By targeting pro-inflammatory state-mediated signalling, these small molecules offer several advantages over biologics, such as oral administration, potentially lower costs, and a broad spectrum of mode of action that includes targeting multiple cytokines simultaneously. Importantly, JAK inhibitors including tofacitinib, baricitinib, and deucravacitinib have shown potential for treating SLE. Among them, the inhibition of TYK2 emerges as a promising therapeutic strategy due to its interference with key cytokines underlying lupus pathogenesis. We are cautiously optimistic about the results of the phase 3 trials of deucravacitinib for this challenging disease.
All authors listed have made a substantial, direct, and intellectual contribution to the work and approved it for publication.
IP is supported by grants from the Swedish Rheumatism Association (R-969696), King Gustaf V’s 80-year Foundation (FAI-2020-0741), Swedish Society of Medicine (SLS-974449), Nyckelfonden (OLL-974804), Professor Nanna Svartz Foundation (2021-00436), Ulla and Roland Gustafsson Foundation (2021–26), Region Stockholm (FoUI-955483), and Karolinska Institutet.
IP has received research funding and/or honoraria from Amgen, AstraZeneca, Aurinia, Elli Lilly, Gilead, GlaxoSmithKline, Janssen, Novartis, Otsuka, and Roche. The funders had no role in the design of the study, the analyses or interpretation of data, or the writing of the manuscript. DN declares that he has no conflicts of interest related to this work.
All claims expressed in this article are solely those of the authors and do not necessarily represent those of their affiliated organizations, or those of the publisher, the editors and the reviewers. Any product that may be evaluated in this article, or claim that may be made by its manufacturer, is not guaranteed or endorsed by the publisher.
1. Tsokos, GC. Systemic lupus erythematosus. N Engl J Med. (2011) 365:2110–21. doi: 10.1056/NEJMra1100359
2. Mahieu, MA, Strand, V, Simon, LS, Lipsky, PE, and Ramsey-Goldman, R. A critical review of clinical trials in systemic lupus erythematosus. Lupus. (2016) 25:1122–40. doi: 10.1177/0961203316652492
3. Banerjee, S, Biehl, A, Gadina, M, Hasni, S, and Schwartz, DM. JAK-STAT signaling as a target for inflammatory and autoimmune diseases: current and future prospects. Drugs. (2017) 77:521–46. doi: 10.1007/s40265-017-0701-9
4. Tao, MJ, Cheng, P, Jin, LR, Zhou, J, Shi, W, Peng, H, et al. The safety and efficacy of biologic agents in treatment of systemic lupus erythematosus: a network meta-analysis. Pak J Med Sci. (2019) 35:1680–6. doi: 10.12669/pjms.35.6.771
5. Nogueira, M, Puig, L, and Torres, T. JAK inhibitors for treatment of psoriasis: focus on selective TYK2 inhibitors. Drugs. (2020) 80:341–52. doi: 10.1007/s40265-020-01261-8
6. Thomas, SJ, Snowden, JA, Zeidler, MP, and Danson, SJ. The role of JAK/STAT signalling in the pathogenesis, prognosis and treatment of solid tumours. Br J Cancer. (2015) 113:365–71. doi: 10.1038/bjc.2015.233
7. Alunno, A, Padjen, I, Fanouriakis, A, and Boumpas, DT. Pathogenic and therapeutic relevance of JAK/STAT signaling in systemic lupus Erythematosus: integration of distinct inflammatory pathways and the Prospect of their inhibition with an Oral agent. Cells. (2019) 8:898. doi: 10.3390/cells8080898
8. Ohl, K, and Tenbrock, K. Inflammatory cytokines in systemic lupus erythematosus. J Biomed Biotechnol. (2011) 2011:432595:1–14. doi: 10.1155/2011/432595
9. Li, B, Jones, LL, and Geiger, TL. IL-6 promotes T cell proliferation and expansion under inflammatory conditions in association with low-level RORγt expression. J Immunol. (2018) 201:2934–46. doi: 10.4049/jimmunol.1800016
10. Manolakou, T, Nikolopoulos, D, Gkikas, D, Filia, A, Samiotaki, M, Stamatakis, G, et al. ATR-mediated DNA damage responses underlie aberrant B cell activity in systemic lupus erythematosus. Sci Adv. (2022) 8:eabo5840. doi: 10.1126/sciadv.abo5840
11. Tanaka, Y, Luo, Y, O’Shea, JJ, and Nakayamada, S. Janus kinase-targeting therapies in rheumatology: a mechanisms-based approach. Nat Rev Rheumatol. (2022) 18:133–45. doi: 10.1038/s41584-021-00726-8
12. Kostopoulou, M, Fanouriakis, A, Bertsias, G, and Boumpas, DT. Treatment of lupus: more options after a long wait. Ann Rheum Dis. (2022) 81:753–6. doi: 10.1136/annrheumdis-2021-221817
13. Lin, CM, Cooles, FA, and Isaacs, JD. Basic mechanisms of JAK inhibition. Mediterr J Rheumatol. (2020) 31:100–4. doi: 10.31138/mjr.31.1.100
14. Hasni, SA, Gupta, S, Davis, M, Poncio, E, Temesgen-Oyelakin, Y, Carlucci, PM, et al. Phase 1 double-blind randomized safety trial of the Janus kinase inhibitor tofacitinib in systemic lupus erythematosus. Nat Commun. (2021) 12:3391. doi: 10.1038/s41467-021-23361-z
15. Morand, EF, Vital, EM, Petri, M, van Vollenhoven, R, Wallace, DJ, Mosca, M, et al. Baricitinib for systemic lupus erythematosus: a double-blind, randomised, placebo-controlled, phase 3 trial (SLE-BRAVE-I). Lancet. (2023) 401:1001–10. doi: 10.1016/S0140-6736(22)02607-1
16. Petri, M, Bruce, IN, Dörner, T, Tanaka, Y, Morand, EF, Kalunian, KC, et al. Baricitinib for systemic lupus erythematosus: a double-blind, randomised, placebo-controlled, phase 3 trial (SLE-BRAVE-II). Lancet. (2023) 401:1011–9. doi: 10.1016/S0140-6736(22)02546-6
17. Morand, E, Pike, M, Merrill, JT, van Vollenhoven, R, Werth, VP, Hobar, C, et al. Deucravacitinib, a tyrosine kinase 2 inhibitor, in systemic lupus Erythematosus: a phase II, randomized, double-blind. Placebo-Controlled Trial Arthritis Rheumatol. (2023) 75:242–52. doi: 10.1002/art.42391
18. Dong, J, Wang, QX, Zhou, CY, Ma, XF, and Zhang, YC. Activation of the STAT1 signalling pathway in lupus nephritis in MRL/lpr mice. Lupus. (2007) 16:101–9. doi: 10.1177/0961203306075383
19. Martinez-Lostao, L, Ordi-Ros, J, Balada, E, Segarra-Medrano, A, Majó-Masferrer, J, Labrador-Horrillo, M, et al. Activation of the signal transducer and activator of transcription-1 in diffuse proliferative lupus nephritis. Lupus. (2007) 16:483–8. doi: 10.1177/0961203307079618
20. Wang, S, Yang, N, Zhang, L, Huang, B, Tan, H, Liang, Y, et al. Jak/STAT signaling is involved in the inflammatory infiltration of the kidneys in MRL/lpr mice. Lupus. (2010) 19:1171–80. doi: 10.1177/0961203310367660
21. Tagoe, C, and Putterman, C. JAK2 inhibition in murine systemic lupus erythematosus. Immunotherapy. (2012) 4:369–72. doi: 10.2217/imt.12.20
22. Lu, LD, Stump, KL, Wallace, NH, Dobrzanski, P, Serdikoff, C, Gingrich, DE, et al. Depletion of autoreactive plasma cells and treatment of lupus nephritis in mice using CEP-33779, a novel, orally active, selective inhibitor of JAK2. J Immunol. (2011) 187:3840–53. doi: 10.4049/jimmunol.1101228
23. Furumoto, Y, Smith, CK, Blanco, L, Zhao, W, Brooks, SR, Thacker, SG, et al. Tofacitinib ameliorates murine lupus and its associated vascular dysfunction. Arthritis Rheumatol. (2017) 69:148–60. doi: 10.1002/art.39818
24. Ripoll, È, de Ramon, L, Draibe Bordignon, J, Merino, A, Bolaños, N, Goma, M, et al. JAK3-STAT pathway blocking benefits in experimental lupus nephritis. Arthritis Res Ther. (2016) 18:134. doi: 10.1186/s13075-016-1034-x
25. Ikeda, K, Hayakawa, K, Fujishiro, M, Kawasaki, M, Hirai, T, Tsushima, H, et al. JAK inhibitor has the amelioration effect in lupus-prone mice: the involvement of IFN signature gene downregulation. BMC Immunol. (2017) 18:41. doi: 10.1186/s12865-017-0225-9
26. Ding, C, Chen, X, Dascani, P, Hu, X, Bolli, R, Zhang, HG, et al. STAT3 signaling in B cells is critical for germinal center maintenance and contributes to the pathogenesis of murine models of lupus. J Immunol. (2016) 196:4477–86. doi: 10.4049/jimmunol.1502043
27. Wu, T, Ye, Y, Min, SY, Zhu, J, Khobahy, E, Zhou, J, et al. Prevention of murine lupus nephritis by targeting multiple signaling axes and oxidative stress using a synthetic triterpenoid. Arthritis Rheumatol. (2014) 66:3129–39. doi: 10.1002/art.38782
28. Edwards, LJ, Mizui, M, and Kyttaris, V. Signal transducer and activator of transcription (STAT) 3 inhibition delays the onset of lupus nephritis in MRL/lpr mice. Clin Immunol. (2015) 158:221–30. doi: 10.1016/j.clim.2015.04.004
29. Georgakis, S, Gkirtzimanaki, K, Papadaki, G, Gakiopoulou, H, Drakos, E, Eloranta, ML, et al. NETs decorated with bioactive IL-33 infiltrate inflamed tissues and induce IFN-α production in patients with SLE. JCI Insight. (2021) 6:e147671. doi: 10.1172/jci.insight.147671
30. Wenzel, J, van Holt, N, Maier, J, Vonnahme, M, Bieber, T, and Wolf, D. JAK1/2 inhibitor Ruxolitinib controls a case of chilblain lupus Erythematosus. J Invest Dermatol. (2016) 136:1281–3. doi: 10.1016/j.jid.2016.02.015
31. Dendrou, CA, Cortes, A, Shipman, L, Evans, HG, Attfield, KE, Jostins, L, et al. Resolving TYK2 locus genotype-to-phenotype differences in autoimmunity. Sci Transl Med. (2016) 8:363ra149. doi: 10.1126/scitranslmed.aag1974
32. Gorman, JA, Hundhausen, C, Kinsman, M, Arkatkar, T, Allenspach, EJ, Clough, C, et al. The TYK2-P1104A autoimmune protective variant limits coordinate signals required to generate specialized T cell subsets. Front Immunol. (2019) 10:44. doi: 10.3389/fimmu.2019.00044
33. Dong, G, You, M, Fan, H, Ding, L, Sun, L, and Hou, Y. STS-1 promotes IFN-α induced autophagy by activating the JAK1-STAT1 signaling pathway in B cells. Eur J Immunol. (2015) 45:2377–88. doi: 10.1002/eji.201445349
34. Nikolopoulos, D, Manolakou, T, Polissidis, A, Filia, A, Bertsias, G, Koutmani, Y, et al. Microglia activation in the presence of intact blood-brain barrier and disruption of hippocampal neurogenesis via IL-6 and IL-18 mediate early diffuse neuropsychiatric lupus. Ann Rheum Dis. (2023) 82:646–57. doi: 10.1136/ard-2022-223506
35. Abe, N, Tarumi, M, Fujieda, Y, Takahashi, N, Karino, K, Uchida, M, et al. Pathogenic neuropsychiatric effect of stress-induced microglial interleukin 12/23 axis in systemic lupus erythematosus. Ann Rheum Dis. (2022) 81:1564–75. doi: 10.1136/ard-2022-222566
36. Duetsch, G, Illig, T, Loesgen, S, Rohde, K, Klopp, N, Herbon, N, et al. STAT6 as an asthma candidate gene: polymorphism-screening, association and haplotype analysis in a Caucasian sib-pair study. Hum Mol Genet. (2002) 11:613–21. doi: 10.1093/hmg/11.6.613
37. Schwartz, DM, Bonelli, M, Gadina, M, and O’Shea, JJ. Type I/II cytokines, JAKs, and new strategies for treating autoimmune diseases. Nat Rev Rheumatol. (2016) 12:25–36. doi: 10.1038/nrrheum.2015.167
38. Bolin, K, Sandling, JK, Zickert, A, Jönsen, A, Sjöwall, C, Svenungsson, E, et al. Association of STAT4 polymorphism with severe renal insufficiency in lupus nephritis. PLoS One. (2013) 8:e84450. doi: 10.1371/journal.pone.0084450
39. Remmers, EF, Plenge, RM, Lee, AT, Graham, RR, Hom, G, Behrens, TW, et al. STAT4 and the risk of rheumatoid arthritis and systemic lupus erythematosus. N Engl J Med. (2007) 357:977–86. doi: 10.1056/NEJMoa073003
40. Kawasaki, M, Fujishiro, M, Yamaguchi, A, Nozawa, K, Kaneko, H, Takasaki, Y, et al. Possible role of the JAK/STAT pathways in the regulation of T cell-interferon related genes in systemic lupus erythematosus. Lupus. (2011) 20:1231–9. doi: 10.1177/0961203311409963
41. de la Varga, MR, Rodríguez-Bayona, B, Añez, GA, Medina Varo, F, Pérez Venegas, JJ, Brieva, JA, et al. Clinical relevance of circulating anti-ENA and anti-dsDNA secreting cells from SLE patients and their dependence on STAT-3 activation. Eur J Immunol. (2017) 47:1211–9. doi: 10.1002/eji.201646872
42. Cunninghame Graham, DS, Morris, DL, Bhangale, TR, Criswell, LA, Syvänen, AC, Rönnblom, L, et al. Association of NCF2, IKZF1, IRF8, IFIH1, and TYK2 with systemic lupus erythematosus. PLoS Genet. (2011) 7:e1002341. doi: 10.1371/journal.pgen.1002341
43. Järvinen, TM, Hellquist, A, Koskenmies, S, Einarsdottir, E, Koskinen, LL, Jeskanen, L, et al. Tyrosine kinase 2 and interferon regulatory factor 5 polymorphisms are associated with discoid and subacute cutaneous lupus erythematosus. Exp Dermatol. (2010) 19:123–31. doi: 10.1111/j.1600-0625.2009.00982.x
44. Lee, YH, Choi, SJ, Ji, JD, and Song, GG. Associations between PXK and TYK2 polymorphisms and systemic lupus erythematosus: a meta-analysis. Inflamm Res. (2012) 61:949–54. doi: 10.1007/s00011-012-0486-y
45. Tang, L, Wan, P, Wang, Y, Pan, J, and Chen, B. Genetic association and interaction between the IRF5 and TYK2 genes and systemic lupus erythematosus in the Han Chinese population. Inflamm Res. (2015) 64:817–24. doi: 10.1007/s00011-015-0865-2
46. Kyogoku, C, Morinobu, A, Nishimura, K, Sugiyama, D, Hashimoto, H, Tokano, Y, et al. Lack of association between tyrosine kinase 2 (TYK2) gene polymorphisms and susceptibility to SLE in a Japanese population. Mod Rheumatol. (2009) 19:401–6. doi: 10.3109/s10165-009-0173-1
47. Li, P, Chang, YK, Shek, KW, and Lau, YL. Lack of association of TYK2 gene polymorphisms in Chinese patients with systemic lupus erythematosus. J Rheumatol. (2011) 38. Canada:177–8. doi: 10.3899/jrheum.100424
48. Sarkar, MK, Hile, GA, Tsoi, LC, Xing, X, Liu, J, Liang, Y, et al. Photosensitivity and type I IFN responses in cutaneous lupus are driven by epidermal-derived interferon kappa. Ann Rheum Dis. (2018) 77:1653–64. doi: 10.1136/annrheumdis-2018-213197
49. Hagberg, N, Joelsson, M, Leonard, D, Reid, S, Eloranta, ML, Mo, J, et al. The STAT4 SLE risk allele rs7574865[T] is associated with increased IL-12-induced IFN-γ production in T cells from patients with SLE. Ann Rheum Dis. (2018) 77:1070–7. doi: 10.1136/annrheumdis-2017-212794
50. You, H, Zhang, G, Wang, Q, Zhang, S, Zhao, J, Tian, X, et al. Successful treatment of arthritis and rash with tofacitinib in systemic lupus erythematosus: the experience from a single Centre. Ann Rheum Dis. (2019) 78. England:1441–3. doi: 10.1136/annrheumdis-2019-215455
51. Bieber, T, Feist, E, Irvine, AD, Harigai, M, Haladyj, E, Ball, S, et al. A review of safety outcomes from clinical trials of Baricitinib in rheumatology, dermatology and COVID-19. Adv Ther. (2022) 39:4910–60. doi: 10.1007/s12325-022-02281-4
52. Wallace, DJ, Furie, RA, Tanaka, Y, Kalunian, KC, Mosca, M, Petri, MA, et al. Baricitinib for systemic lupus erythematosus: a double-blind, randomised, placebo-controlled, phase 2 trial. Lancet. (2018) 392:222–31. doi: 10.1016/S0140-6736(18)31363-1
53. Dörner, T, van Vollenhoven, RF, Doria, A, Jia, B, Ross Terres, JA, Silk, ME, et al. Baricitinib decreases anti-dsDNA in patients with systemic lupus erythematosus: results from a phase II double-blind, randomized, placebo-controlled trial. Arthritis Res Ther. (2022) 24:112. doi: 10.1186/s13075-022-02794-x
54. Dörner, T, Tanaka, Y, Dow, ER, Koch, AE, Silk, M, Ross Terres, JA, et al. Mechanism of action of baricitinib and identification of biomarkers and key immune pathways in patients with active systemic lupus erythematosus. Ann Rheum Dis. (2022) 81:1267–72. doi: 10.1136/annrheumdis-2022-222335
55. Burke, JR, Cheng, L, Gillooly, KM, Strnad, J, Zupa-Fernandez, A, Catlett, IM, et al. Autoimmune pathways in mice and humans are blocked by pharmacological stabilization of the TYK2 pseudokinase domain. Sci Transl Med. (2019) 11:eaaw1736. doi: 10.1126/scitranslmed.aaw1736
56. Ytterberg, SR, Bhatt, DL, Mikuls, TR, Koch, GG, Fleischmann, R, Rivas, JL, et al. Cardiovascular and Cancer risk with Tofacitinib in rheumatoid arthritis. N Engl J Med. (2022) 386:316–26. doi: 10.1056/NEJMoa2109927
57. Molander, V, Bower, H, Frisell, T, Delcoigne, B, Di Giuseppe, D, Askling, J, et al. Venous thromboembolism with JAK inhibitors and other immune-modulatory drugs: a Swedish comparative safety study among patients with rheumatoid arthritis. Ann Rheum Dis. (2023) 82:189–97. doi: 10.1136/ard-2022-223050
58. Khosrow-Khavar, F, Kim, SC, Lee, H, Lee, SB, and Desai, RJ. Tofacitinib and risk of cardiovascular outcomes: results from the safety of TofAcitinib in routine care patients with rheumatoid arthritis (STAR-RA) study. Ann Rheum Dis. (2022) 81:798–804. doi: 10.1136/annrheumdis-2021-221915
59. Xie, W, Xiao, S, Huang, Y, Sun, X, and Zhang, Z. Effect of tofacitinib on cardiovascular events and all-cause mortality in patients with immune-mediated inflammatory diseases: a systematic review and meta-analysis of randomized controlled trials. Ther Adv Musculoskelet Dis. (2019) 11:1759720X1989549. doi: 10.1177/1759720X19895492
60. Kostopoulou, M, Nikolopoulos, D, Parodis, I, and Bertsias, G. Cardiovascular disease in systemic lupus Erythematosus: recent data on epidemiology, risk factors and prevention. Curr Vasc Pharmacol. (2020) 18:549–65. doi: 10.2174/1570161118666191227101636
Keywords: systemic lupus erythematosus, JAK inhibitors, TYK2, treatment, small molecules
Citation: Nikolopoulos D and Parodis I (2023) Janus kinase inhibitors in systemic lupus erythematosus: implications for tyrosine kinase 2 inhibition. Front. Med. 10:1217147. doi: 10.3389/fmed.2023.1217147
Received: 04 May 2023; Accepted: 13 June 2023;
Published: 29 June 2023.
Edited by:
João Eurico Fonseca, University of Lisbon, PortugalReviewed by:
Giacomo Cafaro, University of Perugia, ItalyCopyright © 2023 Nikolopoulos and Parodis. This is an open-access article distributed under the terms of the Creative Commons Attribution License (CC BY). The use, distribution or reproduction in other forums is permitted, provided the original author(s) and the copyright owner(s) are credited and that the original publication in this journal is cited, in accordance with accepted academic practice. No use, distribution or reproduction is permitted which does not comply with these terms.
*Correspondence: Ioannis Parodis, aW9hbm5pcy5wYXJvZGlzQGtpLnNl
Disclaimer: All claims expressed in this article are solely those of the authors and do not necessarily represent those of their affiliated organizations, or those of the publisher, the editors and the reviewers. Any product that may be evaluated in this article or claim that may be made by its manufacturer is not guaranteed or endorsed by the publisher.
Research integrity at Frontiers
Learn more about the work of our research integrity team to safeguard the quality of each article we publish.