- 1Division of Allergy, Clinical Immunology and Rheumatology, Department of Medicine, The University of Kansas Medical Center, Kansas City, KS, United States
- 2Department of Medicine, School of Medicine, University of Missouri, Kansas City, MO, United States
- 3Cardiology Section, Kansas City Veterans Affairs Medical Center, Kansas City, MO, United States
- 4Neurology Section, Kansas City Veterans Affairs Medical Center, Kansas City, MO, United States
- 5Research and Development Service, Kansas City Veterans Affairs Medical Center, Kansas City, MO, United States
- 6Midwest Veterans’ Biomedical Research Foundation (MVBRF), Kansas City VA Medical Center, Kansas, MO, United States
Mast cells are important components of the immune system, and they perform pro-inflammatory as well as anti-inflammatory roles in the complex process of immune regulation in health and disease. Because of their strategic perivascular localization, sensitivity and adaptability to the microenvironment, and ability to release a variety of preformed and newly synthesized effector molecules, mast cells perform unique functions in almost all organs. Additionally, Mast cells express a wide range of surface and cytoplasmic receptors which enable them to respond to a variety of cytokines, chemicals, and pathogens. The mast cell’s role as a cellular interface between external and internal environments as well as between vasculature and tissues is critical for protection and repair. Mast cell interactions with different immune and nonimmune cells through secreted inflammatory mediators may also turn in favor of disease promoting agents. First and forefront, mast cells are well recognized for their multifaceted functions in allergic diseases. Reciprocal communication between mast cells and endothelial cells in the presence of bacterial toxins in chronic/sub-clinical infections induce persistent vascular inflammation. We have shown that mast cell proteases and histamine induce endothelial inflammatory responses that are synergistically amplified by bacterial toxins. Mast cells have been shown to exacerbate vascular changes in normal states as well as in chronic or subclinical infections, particularly among cigarette smokers. Furthermore, a potential role of mast cells in SARS-CoV-2-induced dysfunction of the capillary-alveolar interface adds to the growing understanding of mast cells in viral infections. The interaction between mast cells and microglial cells in the brain further highlights their significance in neuroinflammation. This review highlights the significant role of mast cells as the interface that acts as sensor and early responder through interactions with cells in systemic organs and the nervous system.
1. Introduction
The immune system consists of macrophages, dendritic cells, monocytes, natural killer cells, basophils, eosinophils, neutrophils, T and B lymphocytes and mast cells, and a group of proteins that constitute the complement system (1). Among these cells, mast cells draw special attention due to their presence at strategic locations, expression of several stimulatory as well as inhibitory receptors, and their physiological and pathobiological functions based on the microenvironment. Mast cells are tissue-resident guard cells localized at strategic barrier locations, unlike monocytes, neutrophils, and T and B lymphocytes that circulate in the blood (2). Paul Ehrlich, who discovered mast cells, was also the first to describe their presence in the perivascular areas (3). Mast cells in rodents are grouped under two categories, namely the large connective tissue mast cells (CTMCs) and the mucosal mast cells (MMCs) although other organ specific types have been identified. Human mast cells (MCs) are also classified into two groups based on the type of protease(s), namely tryptase and chymase-positive (MCtc) and tryptase-only-positive mast cells (MCt). Classification of mast cells continues to be refined with advancing computing power for analysis (4).
Mast cells originate from hematopoietic progenitor cells which express c-kit (CD117) (5), the receptor for stem cells factor (SCF) and CD34 but lack FcεR1 (6–9). Circulating mast cell precursors migrate to different tissues through adhesion contacts to a network formed by integrins (10–12). Depending on the microenvironment, mast cells acquire specific phenotypic characteristics and expression of receptors. For example, FCγRIIb is expressed by mast cells of gastrointestinal tract but not by mast cells of skin (13). Mature mast cells are present in the areolar connective tissue space of many organs, such as the adventitial layer of blood vessels, skin, nerve fibers, smooth muscles, airways, gastrointestinal tract, and adipose tissue (14–16).
A characteristic feature of mast cells and other granulocytes (neutrophils, basophils, and eosinophils) is the regulated degranulation of cytoplasmic granular bodies (granule exocytosis) and their ability to regranulate (17). Mast cell degranulation is now a subject of intense investigation because of its therapeutic potential (18). Mast cells can also utilize the classical/constitutive secretory pathway to release mediators that modulate the innate and adaptive immune systems (19). Mast cells can also release DNA to form extracellular traps (MCET) in response to certain microorganisms (20, 21). MCET formed by mast cells have been shown to contain histones, tryptase and LL-37 (20, 22, 23). These characteristics enable mast cells to function as frontline responders to the toxins released by the invading viral, bacterial, or fungal pathogens, insects, and parasites. It is noteworthy that mast cell proteases are capable of degrading insect and snake venom toxins by releasing carboxypeptidase A (CPA3) and thus increasing resistance to their toxic effects (24). Mast cells may also act in synergy with exogenous toxins to amplify inflammatory responses. However, aging and senescence may significantly affect mast cell number and function (25).
In this article, we highlight mast cell receptors and mast cell-generated mediators that regulate inflammatory responses. These mediators enable mast cells to act as pro-and anti-inflammatory effectors to promote mobilization and proliferation of other immune cells. Next, using their perivascular localization as a point-of-reference, we address the role of mast cells under physiological conditions as well as in representative diseases where mast cells orchestrate cross-talks between different cell types and manage immune homeostasis. We discuss the role of mast cells in early vascular inflammation with and without co-morbidities (chronic/sub-clinical infections) and long-term progression to atherosclerosis. Lifestyle choices affect both the severity and outcome of vascular disease with or without infection. Using cigarette smoking as a lifestyle choice model, we outline recent research connecting cigarette smoking with mast cells, vascular inflammation, and bacterial toxins. Role of mast cells in virus-induced pathologies is an emerging field. Here, we discuss the evidence that links mast cells to changes in the capillary and alveolar structure–function following SARS-CoV-2 infection. Since mast cells are uniquely positioned to connect the central nervous system with changes in peripheral organs through the vasculature, we summarize growing evidence that demonstrates interaction between mast cells and brain (microglial) cells that influences neuro-inflammation.
2. Main classes of mediators released by mast cells
2.1. Overview of the mechanisms for release of mediators
Mast cells package pre-formed mediators in granules that are released upon activation. Mast cells also secrete several mediators through the secretory pathway leading to exocytosis of such molecules as large vesicles, microvesicles or exosomes (26, 27). Besides activation-induced secretion of mediators, human skin mast cells can constitutively and spontaneously secrete pro-angiogenic factors (28). Figure 1 provides a snapshot of pre-formed, cytokines/chemokines and de novo synthesized mediators released as well as some key receptors expressed by mast cells. Mediators stored in the granules are diverse chemical entities grouped as biogenic amines (histamine, serotonin, dopamine) (29–32), proteases (serine proteases, aspartic acid proteases, cysteine proteases, metalloproteinases) (33, 34), peptidoglycans (heparin, chondroitin sulfate) (35), many cytokines (TNF, IL-4 etc.) (36, 37), and growth factors (GM-CSF, bFGF, VEGF, NGF) (38). Newly synthesized inflammatory mediators include lipid mediators (LTB4, LTC4, PAF, PGD2), neuropeptides (CRH, VIP) (39), growth factors (PDGF, GnRH) (38), chemokines (MCP-1, eotaxin, TARC, RANTES) (40, 41), and cytokines (IL-1, IL-3, IL-6, IL-18, SCF, TGF-β) (27, 36, 37). Table 1 provides a list of mediators released by mast cells, their receptors, and their significance in disease. Such wide array of inflammatory mediators and the ability to release them through diverse mechanisms enable mast cells to perform a variety of functions including regulation of innate and adaptive immune mechanisms, participation in host defense against invading pathogens, parasites, venom detoxification and elimination of cancer cells (2, 172–178).
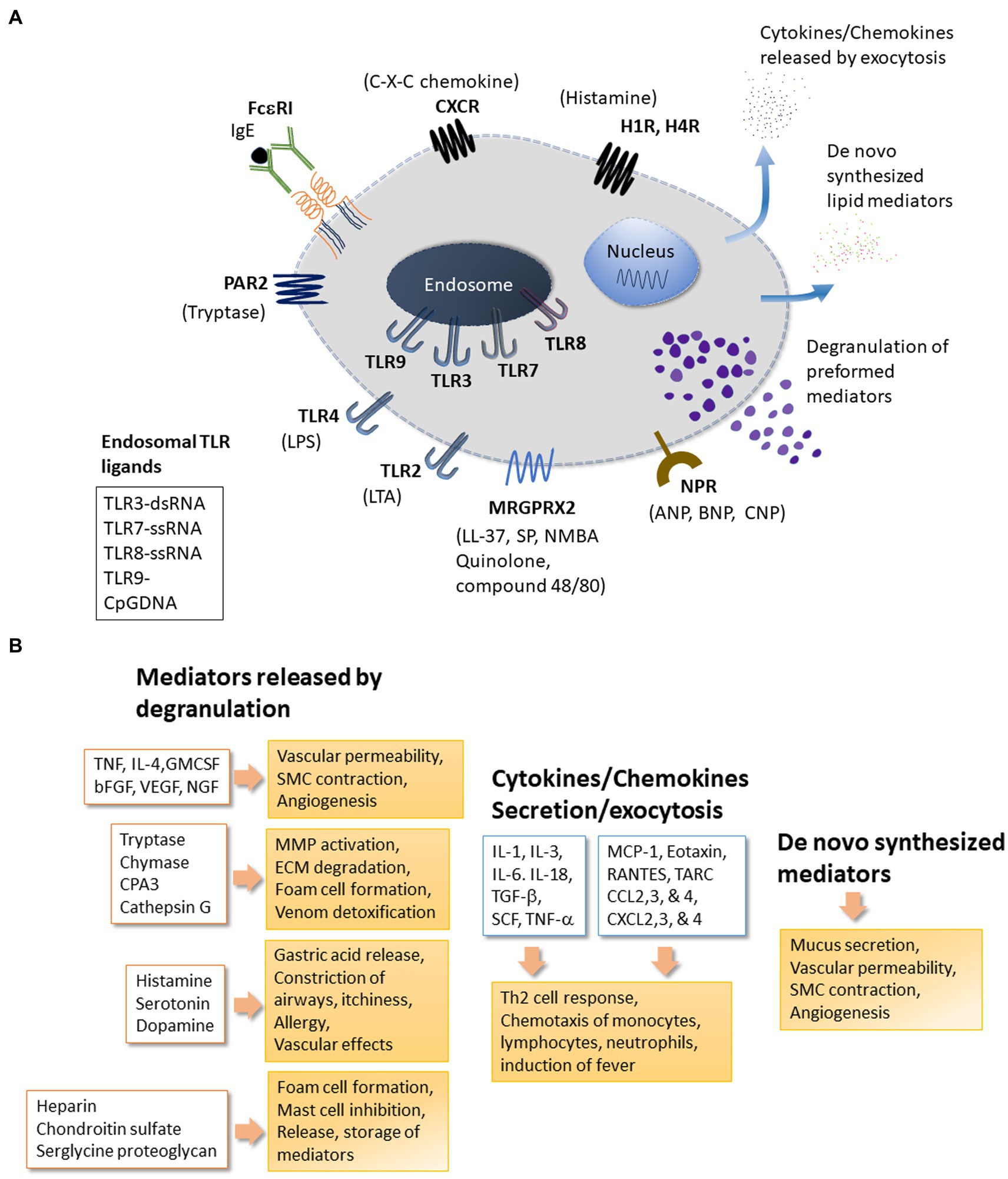
Figure 1. Mast cell receptors and products. Panel (A) depicts some of the major receptors expressed on mast cells. Respective ligands of these receptors are also shown. The receptors shown here include the high affinity FceRI for IgE; MRGPRX2: Mas-related G-protein coupled receptor-X2 that binds quinolones and compound 48/80 among other ligands; TLRs (Toll-like receptors) at the plasma membrane (TLR2 and TLR4) and at endosomes (TLR3 and TLR7-9) that bind to various exogenous and endogenous molecules in a pattern recognizing manner; NPR for neuropeptide ligands ANP, BNP or CNP; Protease Activated Receptors PARs; Histamine Receptors HRs for histamine; CXC-Chemokine Receptor CXCR. Other important receptors expressed on mast cells (not shown here) include receptors for Kit (stem cell factor receptor), Complement (C3a and C5a); alarmins IL-33 (ST2, IL-1 family receptor) and Epithelial cell derived Thymic stromal lymphopoietin (TSLP); the platelet-activating factor (PAF); vascular endothelial growth factor (VEGF); nerve growth factor (NGF); fibroblast growth factor (FGF); Interleukins (IL); Transforming growth factor beta (TGF-b); Thymus and activation-regulated chemokine (TARC); Prostaglandins (PGs); Cysteinyl leukotrienes (CysLT). Mast cell regulator/inhibitory receptors containing immunoregulatory tyrosine inhibition motifs (ITIMs) including IgE receptor Fc-gamma-RIIb, CD300a, CD200 R1, platelet-endothelial cell adhesion molecule 1 (PECAM-1), paired immunoglobulin-like receptor B (PIR-B), the c-lectin mast cell function-associated antigen (MAFA), sialic acid-binding immunoglobulin-like lectins (Siglecs), and leukocyte immunoglobulin-like receptor 4, subfamily B, member 4 (LILRB4). Panel (B) mast cells release many effector molecules through different mechanisms. Degranulation is a robust mechanism for mast cells to release pre-formed molecules of several chemical classes. However, release of mediators through degranulation is a complex process that may vary with regard to the composition of granules or the duration of release resulting in a fine control of mast cell response to their surroundings. Release of molecules including cytokines and chemokines may involve the classical secretory pathway or other mechanism for exocytosis. Molecules such as prostaglandins and leukotrienes are synthesized de novo in response to stimuli. Mediators released by mast cells enable mast cells to respond to many physiological and pathological conditions. bFGF, Basic Fibroblast Growth Factor; CCL2,3, and 4, Chemokine (C-C motif) Ligand 2, 3 and 4; CPA3, Carboxypeptidase-3; CXCL2,3 and 4-Chemokine (C-X-C motif) Ligand 2, 3 and 4; cysLTs, Cystinyl Leukotrienes; GM-CSF, Granulocyte Macrophage Colony-Stimulating Factor; IL-1, IL-3, IL-4, IL-6, IL-18, Interleukins 1, 3, 4, 6, 18; NGF, Nerve Growth Factor; MCP-1, Monocyte chemoattractant protein-1 (CCL2); PAF, Platelet Activating Factor; PGs, Prostaglandins; RANTES, Regulated upon Activation, Normal T cell Expressed and Secreted aka (CCL5); SCF, Stem Cell Factor; SMC, Smooth Muscle cells; TARC, Thymus and Activation Regulated Chemokine; TGF-β, Transforming Growth factor-β; TNF, Tumor Necrosis Factor; VEGF, Vascular Endothelial Growth Factor/vascular permeability factor.
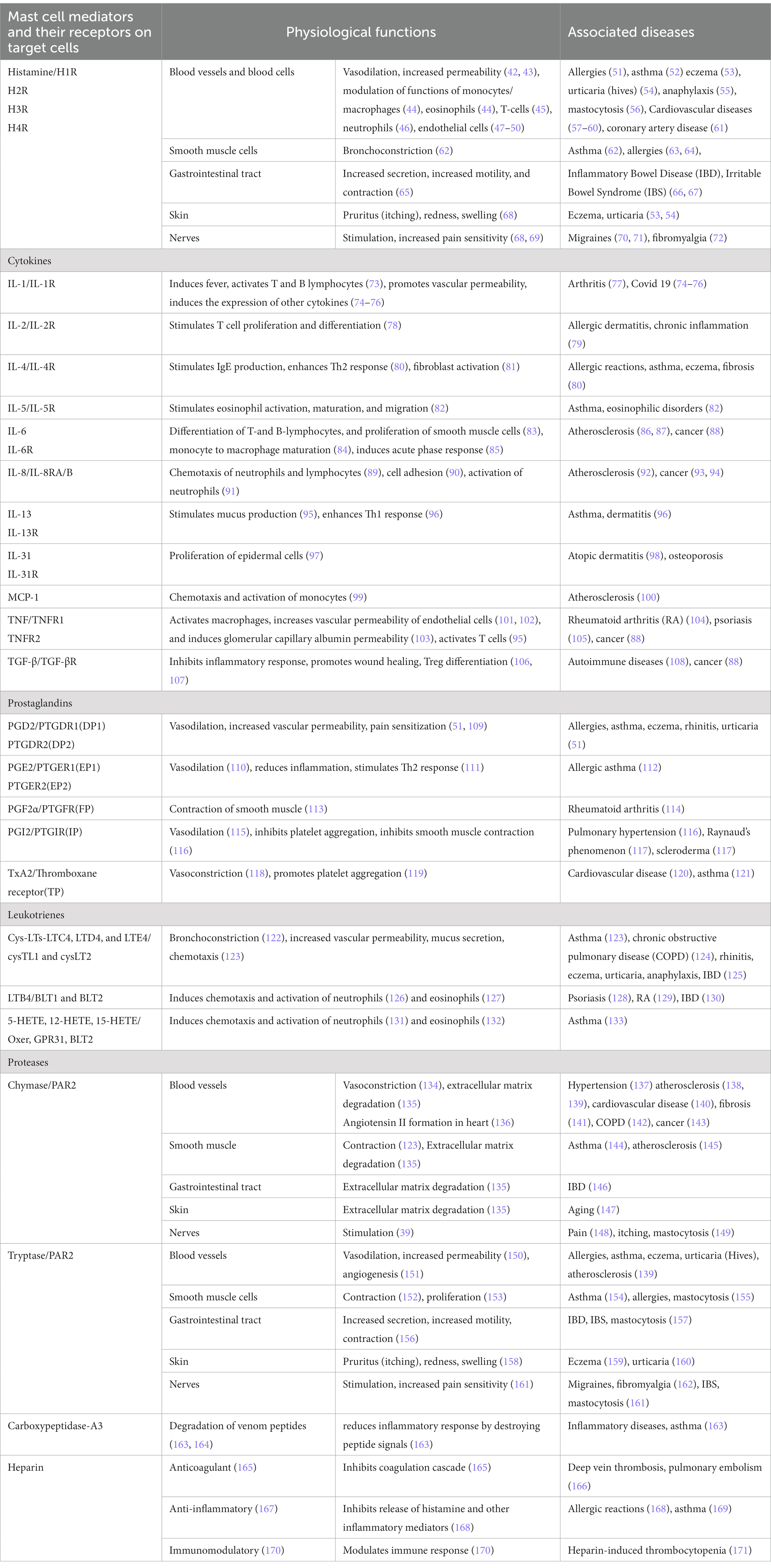
Table 1. Mast cell-released mediators act through their receptors on target cells in various tissues and contribute to physiological functions as well as pathophysiological changes.
Generally, the type of mediators released by mast cells are determined by the receptor [s] activated by specific ligands and the microenvironment. However, the composition, chronology of the release of granules, their cargo and multiple mechanisms for secreting mediators contribute to variations to this generalization (27). The pleiotropic nature of mast cells is also determined by the diversity of receptors, degree of ligand-specificity of receptors and receptor interactions and the effect of released mediators with the microenvironment (179). For example, IgE binds with high affinity to FcɛRI (tetrameric FcεRIαβγ2) expressed on mast cell, basophils, and gastrointestinal mucosa (180). However, IgE can also bind with lower affinity to FcεRII (or CD23, a C-type lectin) and to ε-binding protein εBP (or galectin 3) (181, 182). Such heterogeneity of the cells carrying different receptor isoforms for the same ligand creates varying response to IgE. Also, ligands that bind distinct receptor(s) may induce strong (allergy-type) responses without involving IgE. For example, quinolone containing tetrahydroisoquinoline (THIQ) motif (ciprofloxacin, vancomycin, morphine, rocuronium and others) bind to mast-cell specific Mas-related G protein-coupled receptor (MRGPRX2) and may cause severe allergy-type symptoms without involving IgE (183). In addition, mast cells may release mediators in a selective, piece-meal or discriminatory manner. Selective/differential release of mediators implies a preferential release of a cytokine or neurotransmitter without/before secretion of other mediators and/or without degranulation, e.g., serotonin release without histamine and without degranulation (74, 184, 185). The following sections outlines representative secretory products of the mast cell and their roles in inflammation with special emphasis on vascular inflammation.
2.2. Histamine
Histamine is a vasoactive amine synthesized and stored in the cytoplasmic granules of human basophils and mast cells. It is one of the major secretory products of mast cells that is well-recognized for its participation in allergic and hypersensitivity reactions. Histamine alone as well in combination with other mast cell products regulates vasodilation, bronchoconstriction (186, 187) and modulate the functions of monocytes/macrophages (188, 189), eosinophils (44, 188), T-cells (45), neutrophils (46), and endothelial cells (47–50).
Effects of histamine on physiological processes are mediated through a family of G-protein-coupled receptors, H1, H2, H3, and H4 (190). H1 receptors are highly expressed in many cell types including endothelial cells, smooth muscle cells, neuronal cells, respiratory epithelial cells, hepatic cells, dendritic cells, lymphocytes, and mast cells. Histamine contributes to vasodilation (through histamine-H1R-CycAdenosine axis), arteriolar constriction (through histamine-H1R-thromboxane axis) (118), angiogenesis, and vascular permeability. Histamine acting through the H1 receptor modulates inflammatory and hypersensitivity responses (47, 191–193). The H2 histamine receptor participates in the stimulation of gastric acid secretion in the gut and in the regulation of cytokine production by cells in cardiac, smooth muscle, and immune system (189, 194–197). The H2 receptor is expressed in a wide array of cells including B cells, T cells, dendritic cells, gastric parietal cells, smooth muscle cells as well as in the brain and cardiac tissues (192). The H3 histamine receptor is expressed in histamine-containing neurons of the brain (198) and functions by coupling to Gαi/0. The H4 receptor has approximately 40% homology to the H3 receptor and is highly expressed in bone marrow and leukocytes, and moderately expressed in spleen, thymus, lung, small intestine, colon, and heart (199–201). The H4 receptor is also expressed in the central nervous system and in certain cancer cells. The H4 receptor-signaling is associated with Gαi/0. It is noteworthy that the physiological outcomes of histamine action engaging different subtypes of histamine receptors are distinct and cell-specific.
The significance of histamine and its receptors in cardiovascular disease (CVD) has been proposed in several pioneering studies (57, 58, 202). Our studies have shown that histamine acting through H1 receptor stimulates the expression of IL-6 and IL-8 (47) and COX2 (49) in human coronary artery endothelial cells (HCAEC) in vitro which suggests that it can act as an important vascular inflammatory signal. Interestingly, the effects of histamine on these parameters were synergistically enhanced by lipopolysaccharide (LPS), peptidoglycan (PGN) and lipoteichoic acid (LTA) (47, 48). Our studies also demonstrated that LPS would enhance H1 receptor expression in endothelial cells (50). In addition, histamine can induce smooth muscle cell migration and proliferation (203, 204), and plays a role in intima thickening in a mouse model (205).
A direct relationship between histamine and vascular inflammation is evident from the observation that coronary arteries of patients with ischemic heart disease contain more mast cells and histamine than normal vessels (57). The role of histamine in vascular disease is further supported by the presence of elevated levels of histamine in the coronary circulation of patients with variant angina (206). Takagishi et al. have further shown increased expression of H1 receptor mRNA in smooth muscle cells of intima/media in the atheroma. Many recent reviews have focused on the role of histamine in coronary arterial disease (CAD) (61, 207–210).
2.3. Mast cell proteases
On a weight and molar basis, enzymatically active neutral proteases are the major protein constituents released from activated mast cells (175). Tryptase, chymase, and carboxypeptidase A, in various combinations, represent the three major proteases in the granules of mast cells (211). Expression of tryptases and chymases is highly specific for mast cells but proteases including cathepsins G, C, and L are present in various cells of inflammatory process. Both mast cells and basophils express carboxypeptidase A3 but proteases like mastin are mostly restricted to basophils (33). As mentioned earlier, the unique pattern of protease expression is the basis to classify heterogeneous mast cell populations broadly into MCTC and MCT (212–216). The role of mast cell chymase and tryptase in the progression of atherosclerosis has been extensively studied by Kovanen and colleagues (217–220) and by Bot and collaborators (221, 222).
Human tryptases are heterogeneous proteins expressed by alleles of α, β, and γ genes (223). Human mast cell tryptase can induce IL-8 and intercellular adhesion molecule 1 (ICAM-1) expression in bronchial epithelial cells (224), induce IL-8 production in endothelial cells (225), stimulate collagen synthesis and chemotaxis in fibroblasts (226), and initiate angiogenesis (227). Mast cells have been shown to modulate cardiomyocyte contractibility via release of tryptase, which activates protease-activated receptor 2 (PAR2) (228, 229). Tryptase by activating PAR2 also plays a prominent role in LPS-induced neutrophil recruitment and lung inflammation in a mouse model (230). Furthermore, increased numbers of mast cells and extracellular tryptase within the atherosclerotic plaques during initial stages of calcification have been demonstrated (231). Presence of mast cells in the human heart (232) and the recognition of mast cell chymase as a major pathway for the generation of angiotensin II (233, 234) support the role of mast cells in both myocardial and vascular functions. In addition, tryptase released from mast cells has been shown to activate sensory nerves to release substance P and mast cell chymase activates angiotensin-renin pathway (235). Mast cell chymase and carboxypeptidase A degrade low-density lipoprotein (236, 237). Recombinant mouse mast cell protease-6 independently induces infiltration of neutrophils in vivo and stimulates IL-8 production by cultured endothelial cells (238). These findings underscore the importance of mast cell-derived serine proteases in vascular inflammation and atherogenesis.
Our studies show that mast cell proteases and endotoxin synergistically activate human endothelial cells to generate IL-6 (239), and IL-8 (240). Chemokines and IL-6 play significant roles in the recruitment of inflammatory cells to the vessel wall. Mast cell activation leads to increased recruitment of leukocytes to the plaque with neutrophils as the predominant inflammatory cells in response to IL-8 (241). In addition, mast cell activation enhances the expression of adhesion molecules by endothelial cells which sets the stage for further increase in the transmigration of leukocytes to the plaque (242). Thus, endothelial activation induced by bacterial toxins and the subsequent overproduction of cytokines and chemokines can promote vascular inflammation independently as well as synergistically with mast cell proteases.
2.4. Heparin
Heparin is another component of mast cell granules with a wide range of biological functions including modulation of the release of mediators (243–245). In addition to its interaction with antithrombin III, heparin interacts with protease factor XII of the coagulation cascade. This interaction results in the production of bradykinin causing blood vessel dilatation, adhesion of various cell types to vascular endothelium, and increased vascular permeability leading to edema (246, 247). Heparin is also vital for the mast cell-mediated angiogenesis (248–250), and for vascular inflammation by binding to molecules such as polycationic peptides and chemokines (251, 252).
2.5. Major cytokines and chemokines
TNF is most abundant among the cytokines released by activated mast cells (101, 253). TNF causes immediate activation of macrophages, modulates the effects of other cytokines, increases vascular permeability of endothelial cells (101, 102), and causes increases in glomerular capillary albumin permeability in vitro (103). It is well-established that TNF facilitates vascular inflammation by increasing the expression of adhesion molecules resulting in increased binding of leukocytes and other immune cells to endothelial cells (254). In combination with IFNγ, TNF can increase vascular permeability by disrupting the cell-junction proteins (255). In addition, activated mast cells release other de novo synthesized cytokines including IL-1, IL-6, IL-8, and MCP-1 (256, 257).
Interleukin-6 (IL-6) is a pleiotropic cytokine secreted by a variety of cells including mast cells and endothelial cells and is known to stimulate the proliferation and differentiation of T-and B-lymphocytes, and smooth muscle cells (83). IL-6 has been shown to contribute to allergic conditions. In situ-derived human skin mast cells express functional membrane-bound IL-6 receptors. IL-6 enhances FcεRI-induced COX-2 expression and potentiates PGD2 biosynthesis through a STAT-3 dependent mechanism (258). In support of a pro-angiogenic role for mast cells, IL-6 was found to induce the expression of VEGF and MCP-1 in in situ-derived human skin mast cells (28, 258). It also initiates an acute phase response and is implicated in many inflammatory and autoimmune diseases (259). Elevated levels of IL-6 gene transcripts were found in atherosclerotic lesions of genetically hyperlipidemic rabbits (260). Atherosclerotic human arteries express 10 to 40-fold higher IL-6 mRNA than non-atherosclerotic arteries, and the thickened intimal layers of atherosclerotic vessels have higher number of IL-6 gene transcripts (86). Recently, IL-6 was shown to increase mast cell proliferation by down-regulation of suppressor of cytokine signaling 3 (SOCS3) and suppression of the hydrolysis of soluble IL-6 receptor resulting in a more reactive mast cell phenotype (261). Attenuation of IL-6 secretion has been proposed as a therapeutic approach for mast cell-related diseases such as mastocytosis (261).
Mast cells also release IL-8, a chemokine with a pivotal role in chemotaxis of neutrophils and inflammation. IL-8 was shown to be spontaneously secreted by human skin-derived mast cells (28). The diverse biological properties of IL-8 include chemotaxis of neutrophils and lymphocytes (89), regulation of cell adhesion (90), and activation of neutrophils (91). These pioneering studies implicate IL-8 as a key factor in the pathogenesis of vascular disease. In addition to its well-recognized chemoattractant properties, IL-8 is a potent angiogenic factor found at high concentrations in atherosclerotic lesions and macrophages collected from patients with the disease (92).
Monocyte chemoattractant protein-1 (MCP-1/CCL2) is a chemokine which is also released by mast cells. MCP-1 is a member of the CC chemokine family which attracts and activates monocytes (99). Mast cell degranulation induces production of MCP-1 and IL-8 in endothelial cells that is further amplified by tryptase (262). MCP-1 is important for allergen-specific activation of mast cell and acute phase inflammation and, it plays a critical role in the initiation and development of atherosclerotic lesions as it recruits monocytes into the sub-endothelial layers of the vessel wall (263, 264). Besides sub-endothelial macrophages and smooth muscle cells, endothelial cells are a major source of MCP-1 in atheromatous plaques (263, 264). Abundant amounts of MCP-1 have been detected in atherosclerotic lesions (265), and increased expression of MCP-1 mRNA has been detected in endothelial cells, macrophages, and vascular smooth muscle cells of human atherosclerotic arteries (86, 266). Studies using MCP-1-deficient and MCP-1 receptor-deficient mice have demonstrated decreased presence of macrophages and a reduction of atherosclerotic lesions (267).
Mast cells release pruritogenic cytokine IL-31 upon activation by antimicrobial peptides [e.g., human beta-defensins, cathelicidin, LL-37] (268, 269) and form extracellular traps by releasing DNA, like neutrophil extracellular traps, to inhibit bacterial growth (21). Overall, cytokines and chemokines released by mast cells enable rapid recruitment of neutrophils to the site of infection.
2.6. Major lipid mediators
Mast cells express both cyclooxygenase 1 and 2 (COX1 and COX2) (270) that catalyze the oxygenation of arachidonic acid to PGG2/PGH2 which are used by specific prostaglandin (PG) syntheses to generate PGE2, PGD2, PGF2α, PGI2, and TXA2. Among the PGs, PGI2, and TXA2 are well-recognized for their role in cardiovascular diseases (271–273). Prostacyclin (PGI2), is a potent vasodilator and an inhibitor of leukocyte adhesion, and platelet aggregation, and therefore, plays a protective role atherothrombosis (274). TXA2, in contrast, is a potent inducer of vasoconstriction, platelet activation and platelet adhesion (274). COX2 contributes significantly to systemic PGI2 synthesis in humans (275, 276), although COX1 also contributes to vascular PGI2 synthesis (272, 277). PGI2 has a protective role in vascular remodeling by smooth muscle cells and its absence leads to an increased intima/media ratio in response to vascular injury following disruption of the gene for the PGI2 receptor in mice (278). We have demonstrated that histamine induces the expression of COX2, but not COX1, in endothelial cells resulting in enhanced production of PGI2, indicating the vasodilatory effects of mast cells in vascular homeostasis (49). Since PGI2 and TXA2 act on vascular endothelium in opposing manners, their relative concentrations in the microenvironment and systemic circulation are critical for cardiovascular protection. These findings underscore the importance of mast cells in both cardiovascular health and cardiovascular disease.
Leukotrienes (LTs) belong to another group of arachidonic acid metabolites secreted by mast cells. LTs [LTA to LTE] are formed through a series of reactions initiated by 5-lipoxygenase. Due to the presence of 5-LO and LTC4 synthase, mast cells and eosinophils show a preferential production of LTC4 (279). Cysteinyl-leukotrienes, LTC4, LTD4 and LTE4, are important for causing bronchial constriction in asthma and play a role in CAD, rheumatoid arthritis, and allergic rhinitis. It has been noted that cysteinyl-leukotrienes are increased in ischemia–reperfusion injury in murine models and in patients (280, 281). On the other hand, LTB4 is secreted by mast cells, macrophages, and neutrophils. It functions as an autocrine chemoattractant of progenitor cells and immature cells (282). Atopic dermatitis patients express functional autoantibodies against IgE and/or FcεRI, and these antibodies have been shown to induce PGD2 and LTC4 by human cardiac mast cells to cause cardiac inflammation (283). Additionally, FcεRI-induced expression of COX-2, and presumably PGD2 biosynthesis but not LTC4, was shown to be, at least in part, controlled by miR-155 in human skin mast cells (284). Therefore, patients with autoimmune disease may be at increased risk of releasing vasoactive, proinflammatory mediators when cardiac mast cells get activated with autoantibodies against IgE or/and FcεR1 (283).
3. Mast cell receptors and the significance of toll-like receptors in inflammatory response
Mast cells express receptors for a variety of ligands (Figure 1). Some of the key receptors most relevant to this article are discussed here. Interaction of mast cell Fc receptors with anti-IgE or complement-bound pathogens is the most studied field of mast cell function through specific ligand binding (285–287). Additionally, mast cells utilize the TNF superfamily and TNF receptors (TNFR), CD30L-CD30, and pattern recognition receptors (PRRs) to engage in an array of interactions (288, 289).
PRRs enable mast cells to detect exogenous and endogenous signals and initiate immune surveillance. PRRs can detect, recognize, and neutralize pathogens by engaging the microbial pathogen-associated molecular patterns (PAMPs) and endogenous products containing damage-associated molecular patterns (DAMPs). PRRs include the C-lectin-type receptors (CLRs), retinoic acid-induced gene I-like receptors (RLRs), Nod-like receptors (NLRs) and Toll-like receptors (TLRs) (289, 290). Among these, certain TLR isoforms are relevant for the present discussion. Briefly, TLRs (isoforms 1–10 in humans, a total of 13 isoforms in murine species) are evolutionarily conserved, and their activation leads to an inflammatory response against microbial pathogens (291). TLRs are localized at the cell membrane or in the endosomes (TLR3, TLR7, and TLR9). Membrane-bound TLRs (TLR1, TLR2, TLR4, TLR5, TLR6, TLR10) contain a common intracytoplasmic domain that conveys signals by molecules that are shared by interleukin-1 (IL-1) receptor signaling to activate the NF-κB pathway and release pro-inflammatory cytokines (292).
Mast cells express most of the known TLR isoforms and among them TLR4 mediates responses to LPS (from the cell wall of Gram-negative bacteria) as well as heat shock protein (HSP60) (293–296). TLR2 recognizes several components of Gram-positive bacteria and mycobacteria, including peptidoglycan (PGN) and lipoteichoic acid (LTA). TLR2 activation results in release of cytokines like IL-4 but its activation by peptidoglycan (another TLR2 ligand) results in mast cell degranulation and histamine release (294, 297–300). Human endothelial cells constitutively express low levels of TLR2 and TLR4 and are activated by Gram-positive and Gram-negative bacterial components (48, 301, 302). The increased levels of TLR2 and TLR4 gene expression in the endothelium of human atherosclerotic lesions (303) and the reduced incidence of atherosclerosis in patients with TLR4 polymorphism (304), suggest a role for TLRs in vascular inflammation (305). The anecdotal observation that ‘acute coronary syndrome’ is common in patients following a viral or bacterial infection also supports a role for the TLR pathway in vascular inflammation and disease (306, 307).
4. Physiologically significant functions of mast cells
4.1. Overview of the physiological significance of mast cells
Mast cells are evolutionarily conserved cells dating back to thousands of years and their predecessor cells have been reported in non-vertebrate Ciona intestinalis (phylum chordata) prior to the evolution of adaptive immunity (308). Through their participation in both adaptive and innate immune systems by the virtue of their flexibility to change phenotypes with the microenvironment, mast cells participate in an array of physiological processes (Figure 2). Mast cells are present in almost all tissues, and are particularly notable in areas such as the mucosa in the respiratory, digestive, and urogenital systems, the dermis, blood vessels, lymph vessels, fibroblasts, and in the proximity of peripheral nerves, and this strategy allows them to function as sensors of changes in their local microenvironment (309). The localization of mast cells within the vessel wall highlights their involvement in the vasculature, specifically in vasodilatory functions and tissue-specific responses against circulating agents.
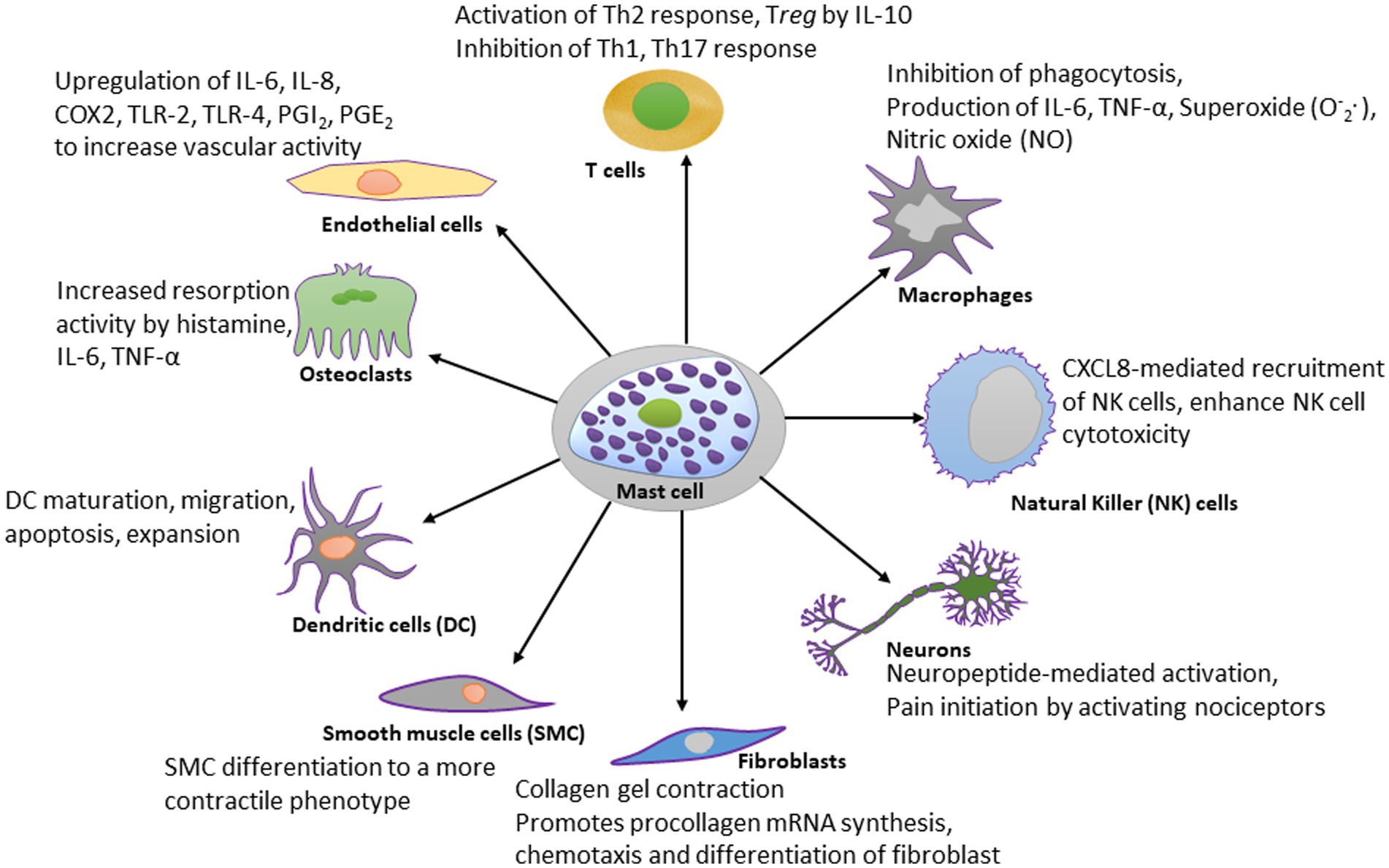
Figure 2. Schematic diagram showing the effect of mast cell-derived compounds on specific cell types. Mast cells have stimulatory or inhibitory actions on many types of cells. A few of those cell types are shown in this diagram. For the simplicity, the scheme shows only most studied effector molecules.
Mast cells play important roles in allergic and hypersensitivity reactions, vasodilatation, gastric acid production, angiogenesis, pathogen and parasite clearance, venom detoxification, mineral homeostasis, bone remodeling, wound healing, reproduction, and immune homeostasis, and each of these functions will be discussed.
Mast cells participate in immune homeostasis through multiple mechanisms of the innate and adaptive immune system (310–313). Although mast cells are not known as classic phagocytes, they are active participants in the elimination of bacteria through interactions with T and B cells and engaging ICAM-1, ICAM-3, CD43, CD80, CD86, and CD40L (73). Mast cells promote the development of Th2 cells and induce B cells to produce IgE through isotype switching (2).
The best recognized modulatory effects of mast cells are on dendritic cells, macrophages, T lymphocytes, natural killer (NK) cells, fibroblasts, neurons, endothelial cells, osteoclasts, and smooth muscle cells, and they are summarized in Figure 2. The significance of mast cells in neurodegenerative diseases is discussed separately.
4.2. Mast cells and infectious or toxic agents
4.2.1. Mast cells and bacteria
The evidence supporting the role of mast cells in fighting bacterial infections is based on the finding that transfer of wild-type mast cells (TLR2+/+) reduced the pathogen load and myeloid cell recruitment in TLR2-deficient (TLR2−/−) mice infected with Mycobacterium tuberculosis (314). Furthermore, mast cell-deficient mice were found to be more susceptible to Group B streptococcus infection (315). Anti-bacterial response of mast cells is shown to be mediated by release of inflammatory molecules resulting in increased vascular permeability and fluid accumulation, and recruitment of immune cells (eosinophils, NK cells, and neutrophils) (41, 316, 317). Different bacterial genera have been shown to elicit distinct secretory responses from mast cells in vitro (318). Such specificity in response is due to receptors that are expressed in a tissue-specific microenvironment (discussed above). Mast cells also generate antibacterial molecules, such as cathelicidins, defensins, and psidins (2). Interaction of mast cells with bacterial toxins (LPS) is discussed separately in this article. Mast cells are important for maintaining the gut microbiome. In the gastrointestinal tract mast cells contribute significantly to the homeostasis of the commensal bacteria through modulation of IgA maturation and follicular helper T cells maturation (2).
4.2.2. Mast cells and helminth infections
The controlled discharge of mast cell mediators through the activation of FcεRI by IgE is a vital component of the body’s defense against parasites, particularly helminths (319–322). In certain parasitic infections, mast cells are not significantly involved. Instead, the immune response relies on the binding of IgE to CD23 receptors found on eosinophils, platelets, macrophages, and dendritic cells. This leads to antigen presentation and the subsequent production of IgG antibodies (323, 324). In contrast, mast cell degranulation may be induced without involving IgE (322, 325). Despite its significance in some infections, mast cell involvement is neither crucial nor beneficial in all parasite-related responses. Mast cell degranulation can have harmful consequences, including tissue damage, and their activation is not always essential (326, 327). Nevertheless, parasitic helminths are sensed via tissue alarmins of the immune system that signal the presence of helminthic parasites leading to type 2 immune responses. Increased number of intestinal mast cells has been associated with Th2 immune response and certain cytokines (SCF, IL-3, IL-4, IL-9, IL-10, IL-18) (104). Notch 2 signaling pathway was found to mediate mobilization of mast cells to the area of parasite infection (328).
Mast cell degranulation following FcεRI activation by parasite-specific IgE and antigen is a strong response against certain parasites through several mediators. Mast cell proteases may directly act on parasites (329); glycosaminoglycans in mast cell granules may prevent adult worms from attaching to intestinal mucosa (330); cytokines/chemokines/mediators from mast cells modulate mobilization or function of other innate immune cells with various effects on parasites (331). Leukotriene LTB4 may recruit other immune cells to remove certain parasites (332). Mouse mast cell protease-6 (mMCP6) promotes eosinophil recruitment that may favor the parasite (333); mMCP1 released by mast cells may damage the intestinal barrier function thus promoting inflammation and pathological changes by degrading occludin and other tight junction proteins (334, 335).
Mast cells and basophils can release mediators that favor parasite expulsion through increasing vascular permeability (336–339). MC-mediated changes in the intestinal barrier function can promote the flow of fluid and blood-borne antibodies into the intestinal lumen creating an unfavorable environment for the parasites. Activation of IgE and/or IgG receptors creates cytotoxic milieu for parasite expulsion (340).
Overall, the response of mast cells to parasites depends on several factors including the type of parasite, presence of other parasites, genetic background of the host, status of intestinal mucosal cells and other surrounding cells, host microbiome, levels of parasite-specific vs. non-specific IgE, expression of high affinity (FcεRI) and low affinity (CD23) receptors for IgE, additional host defense cells including the granulating and non-granulating cells. Parasite-specific details, potential protective role of mast cells and treatment approaches are described in greater detail in more comprehensive reviews (24, 341).
4.2.3. Protozoan infections (malaria)
Mast cells play a role in protozoan infections, but their actions can be both protective or harmful depending on factors such as parasite species, host genetics, and timing of infection. Both innate and adaptive immune systems are involved in this process (342). Histamine and mast cells promote disease severity (343, 344) and intestinal permeability in malaria (345). Mast cell activation during mosquito feeding leads to inflammatory response (granulocyte recruitment, lymph node hyperplasia) (346). Mast cells release TNF in response to IgE binding to FcεRI (adaptive immune response) as well as TLR4 binding to peroxiredoxin from the parasite (innate immune) (347, 348). Mast cell activation by mosquito saliva down regulates antigen-specific immune response through MIP2 and IL-10 and promotes disease through activation of tissue damaging CD8 + T cells (349). Malarial parasite antigen induces VEGF release from mast cells in cerebral malaria (350), increases co-infection (351). Mast cells activation by uric acid released from plasmodium-infected RBS leads to activation of the tissue damaging CD8+ T cells (342).
4.2.4. Ixodid ticks
Mast cells along with basophils and IgE have been shown to contribute to host resistance to larval Ixodid ticks (352). These organisms can mediate the transmission of pathogens to the host. Such pathogens include the agents of Rocky Mountain spotted fever (Rickettsia rickettsia) (353), Q fever (Coxiella burnetii) (354), tularemia (Francisella tularensis) (355), granulocytic ehrlichiosis (Ehrlichia ewingii) (356), monocytotropic ehrlichiosis (Ehrlichia chaffeensis) (357).
4.2.5. Fungal infections, venoms
Mast cells are also important players in the host immune response to fungi (358, 359). Based on the discovery that activated IgE can trigger the release of carboxypeptidase A, it has been established that mast cells play a crucial role in both innate and adaptive immune responses to a range of venoms. Carboxypeptidase A breaks down snake and bee venoms, and endogenous toxins. Detailed discussion of the significance of mast cells and IgE in toxic reactions to venoms as well as research on their role in host protection can be found in recent reviews (321).
4.3. Mast cells in the reproductive system
The role of mast cell mediators in reproductive biology including spermatogenesis and pregnancy is currently being investigated and reviewed recently (360, 361). In males, two phenotypes of mast cells (MCTC, tryptase-positive, chymase-positive, and MCT, tryptase-positive, chymase-negative) are present in the testis and epididymis. Additionally, histamine influences steroidogenesis in Leydig cells through H1 or H2R (362, 363). In females, mast cell proteases activate MMP2 and MMP9, resulting in matrix degradation during the menstrual cycle (364). Mast cell products are important for fertility, pregnancy (365), and abortion (366). Mast cells release histamine and α-chymase when activated by estradiol and progesterone (367) and contribute to uterine remodeling for embryo implantation. During childbirth, before the onset of labor, the number of mast cells in the cervix increases which contributes to cervical ripening (360, 368). In addition, increased number of mast cells (indicated by increased levels of TNF-α) is associated with abortion (366, 369).
4.4. Mast cells in the skin and wound healing
Mast cells localize close to endothelial cells of the vasculature, hair follicles and nerves (370, 371). Dermal mast cells also play a critical role in all stages of wound healing. Additionally, mediators released by mast cells show pro-inflammatory effects that promote acute inflammation. Mast cell mediators also stimulate scarring, epithelialization and angiogenesis (372). Further evidence shows that in situ-derived human skin mast cells can spontaneously secrete several angiogenesis-related factors leading to the speculation that mast cells could be involved in vasculogenesis as well as angiogenesis and human development (28).
Considerable evidence suggests that mast cells join other cells as modulators of acute skin wounds. The role of mast cells in wound healing has been summarized in recent reviews (373, 374). The process of wound repair in man and experimental models involves overlapping events. Studies using rodent models show that mediators released by mast cells appear to influence the changes during the entire process of wound healing. Studies show that, (i) mast cells number and degranulation along the wound area increase within minutes and reaches to maximum within 1–3 h after injury corresponding with clot (fibrin network) formation during the immediate local response/the hemostatic phase (375, 376). Mast cells release histamine and TNF that promote endothelial expression of adhesion molecules for leukocyte adhesion (253). Leukotrienes and cytokines from mast cells promote chemotaxis of neutrophils, basophils, and eosinophils. Mast cell secreted tryptase and cathepsin G modulate leukocyte adhesion and function and chymase may activate eosinophils to release chemokines (377, 378). After the initial rise, mast cell numbers return to the base line around 6 h. (ii) Vasodilation and plasma exudation with activation of phagocytes and granulocytes to clean the wound during the inflammatory phase lasts up to 2-3 days and continues into the next phase. Some authors suggest that attracting neutrophils during this phase is the main function of mast cells (379). (iii) A second increase in the number of mast cells is believed to contribute to the proliferative phase (24-72 h). Mast cells secrete growth factors and cytokines (TNF, TGF-β, proteases) that modulate proliferation of fibroblasts to induce their transformation into myofibroblasts and their function leading to wound closure (380), basal epithelial proliferation and movement to close/contract the wound during the proliferative phase (24-72 h). Mast cell secreted transforming growth factor-β (TGF-β), vascular endothelial growth factor (VEGF), chymase and tryptase stimulate angiogenesis (381–386) while heparin may block pro-angiogenic factors (387) (iv) remodeling phase, fibroblasts removed, deposition of collagen matrix and ECM resembling skin, scar formation and closure of the wound (2–3 weeks and longer) (388). During this phase, mast cell tryptase has been found to stimulate collagen synthesis by fibroblasts (389).
In the later phases of wound healing, histamine and serotonin act in a stimulating manner on epidermal keratinocytes, while TNF has an inhibitory effect on them (390). Additionally, mast cell proteases MCP4 and MCP6 play a role in minimizing scar formation in the central nervous system after trauma (321). Another study found accumulated mast cells and their products chymase, fibroblast growth factor 2 (FGF2), TGF-β1 and VEGF at the edge of scald wound in mice during the proliferative and remodeling phases at days 14 and 21. Chymase activity in the injured tissues was decreased in the acute phase, but recovered to a no-injury level at days 14 and 21 (386). Thus, mast cells influence wound healing by promoting acute inflammation, proliferation/re-epithelialization, and angiogenesis, as well as scar contraction and collagen cross-linking (372). The available data suggests that mast cells may play a role in chronic wound healing, but there is not enough conclusive evidence to make any definitive conclusions at this time (391, 392).
5. Abnormal mast cell activation or expansion
Although mast cells have been extensively studied for their pro-inflammatory effects, they are also known to cause anti-inflammatory effects (393, 394). The number and activation of mast cells are strictly regulated under normal physiological conditions and the increased number of mast cells and the overproduction and release of mediators by certain mast cell clones reflect pathological changes. Two well-defined conditions, namely mastocytosis and mast cell activation syndrome (MCAS), are associated with high numbers of mast cells and excessive release of pro-inflammatory mediators that may affect several organs. Mastocytosis is a rare disorder [1 in 10,000–20,000] that is characterized by clonal proliferation and accumulation of mast cells. Two types of mastocytosis have been described, namely cutaneous and systemic mastocytosis. Cutaneous mastocytosis indicates accumulation of mast cells in the skin without much infiltration elsewhere in the body and mainly affects children (395). In contrast, systemic mastocytosis is generally found among adults who present with large numbers of mast cells in multiple tissues besides the skin, namely the bone marrow, gastrointestinal tract, and lymph nodes (396, 397). Recent studies report increased incidence of cardiovascular diseases in patients with mastocytosis (398). Mast cell activation syndrome (MCAS) represents overproduction or over activation of mast cells due to a variety of etiologies. It leads to repeated episodes of a spectrum of multisystem dysfunctions and several aspects of MACS remain unclear (399). MCAS is currently being discussed in the context of inflammation and loss of alveolar function in some patients with SARS-CoV-2 infection (76, 399–401).
6. Mast cells and allergic diseases
Mast cells play a pivotal role in the pathogenesis of allergic diseases. Since mast cells have been recognized as a major contributor in initiating hypersensitivity reactions and the aftermath of the episodes leading progression of chronic diseases like asthma, atopic dermatitis and urticaria, considerable focus has been given to the field of mast cell biology research (402). Since it is far beyond the scope of this article to accommodate the vast volume of work done on the role mast cells in allergic diseases, we are providing only a very brief commentary in this section.
By virtue of their strategic locations in the skin, mucosa, gut and lungs, mast cells are in constant contact with the external environment and allergens (403–407). Mast cells are the effector cells responsible for the IgE-mediated allergic reactions in which allergens are recognized and presented by antigen processing cells to naïve T lymphocytes. T cells recognize the antigen as a foreign material and differentiate into Th2 lymphocytes. Mast cells also can process and present antigens via MHC-I and MHC-II complexes. Because of this characteristic feature, mast cells contribute significantly to the sensitization process and in directing the adaptive immune system toward Th2 pathway in response to antigens (408, 409). Allergies develop when components of the immune system, particularly mast cells, respond to antigens and release many prestored and newly synthesized mediators which in turn interact with a variety of cell types including endothelial cells, macrophages, epithelial cells, T and B lymphocytes, cardiomyocytes, parietal cells, and neurons. As mentioned earlier, mast cell-released mediators include histamine, serine proteases and other enzymes, proteoglycans (heparin or chondroitin sulphate) and as well as prostaglandins and leukotrienes which are rapidly synthesized from arachidonic acid by the enzymes, cyclooxygenase, and lipoxygenase, respectively (see mast cell released mediators above).
Earlier studies on the association of mast cells in allergic reactions were focused on the acute phase of these reactions. In this regard, FceR1 stimulation by a polyvalent allergen recognized by the receptor-bound IgE initiates immediate hypersensitivity reactions by releasing pre-formed and newly synthesized mediators from the sensitized mast cells. These mediators are responsible for allergic symptoms such as erythema, edema, increased vascular permeability, smooth muscle contraction and increased mucus secretion (410). The mast cell mediator such as histamine, PGD2 and LTC4 contributes to asthmatic symptoms, causing bronchoconstriction, mucus secretion and respiratory mucosal edema.
It should be emphasized that allergic reactions are complex and multiphasic comprising of both acute and chronic outcomes. In later episodes, proinflammatory mediators secreted by mast cells induce the recruitment of eosinophils, basophils, and T cells to the sites of inflammation (410–412). The late phase of the allergic reaction is followed by a chronic phase which is associated with persistent inflammation, tissue remodeling and fibrosis. These phases are prevalent in allergic asthma, rhinitis, and atopic dermatitis (413–415).
It is noteworthy that mast cells are also activated through IgE-independent mechanisms in the initial phases of an allergic response. Existing evidence suggests that serine proteases can directly activate mast cells (416, 417). In addition, a multitude of agonists including complement, neuropeptides, cytokines, stress hormones and radiocontrast chemicals can activate mast cells directly (418–421).
As described earlier, FceRI cross-linking with polyvalent antigens is the primary component initiating allergic responses. The uncontrolled and persistent mast cell activation can lead to life threatening conditions such as anaphylaxis and chronic inflammatory diseases like asthma. Therefore, many recent therapeutic strategies are aimed at targeting this pathway to inhibit mast cell degranulation and mediator release (422).
7. Mast cells and atherosclerosis
The pathogenesis of atherosclerosis involves endothelial cell damage, persistent inflammatory response, increased expression of adhesion molecules and accumulation of a variety of cell types (423–426). There is ample evidence in the literature to demonstrate the role of mast cells in vascular inflammation and atherosclerosis progression (2, 57, 87, 120, 220, 221, 231, 236, 237, 427, 428). Pro-inflammatory and anti-inflammatory substances released by mast cells (175–178) are important modulators of vascular homeostasis particularly in relation to atherosclerosis and cardiovascular diseases (115, 116, 118, 120, 124, 134, 136–139, 429–433). Pro-inflammatory activation of vascular endothelium by mast cell mediators, cytokines such as TNF and IL1-β, bacterial cell wall components, viruses, and thrombin can induce local thrombosis, endothelial production of cytokines, loss of vessel barrier function, and enhanced leukocyte adhesion. Such chronic or subclinical inflammatory activation of the endothelium is a part of host defense mechanisms, and its dysfunctional response leads to early events in the onset and progression of atherosclerosis (434, 435).
7.1. Mast cells as mediators of inflammation during the initial stages of atherosclerosis
Mast cells play a critical role in the complex process of immune response during acute and chronic inflammatory states and they are implicated in the progression of atherosclerosis (59, 87, 217, 231, 236, 237, 423–426, 436). The adventitia of coronary arteries of patients with atherosclerotic plaques contain increased number of mast cells (57, 120, 231, 427, 437). Histological studies have demonstrated several hundred-fold increase in the number of activated mast cells in the atherosclerotic plaque rupture regions of coronary arteries. Mast cells in degranulated state were considered to have already undergone activation (438). Besides the adventitial layer, epicardial adipose tissue around the coronary artery also contains increased number of mast cells in CAD patients compared to controls (439). Increased number of mast cells were reported in the adventitia of the thrombosed veins, in vicinity of the vasa vasorum (440). Mast cells are considered, at least partially, to contribute to thrombosis through histamine release and by stimulating endothelial activation (441).
Mast cell granules have been identified within endothelial cells in vivo (442) and are known to cause proliferation of human microvascular endothelial cells (443). Furthermore, mast cell granule remnants have been shown to bind to low-density lipoproteins (LDL) and enhance their uptake by macrophages leading to the development of foam cells (236, 237).
7.2. A dual role for mast cells in infection-induced inflammation
A potential role for infection in the development of atherosclerosis has been considered for several decades. The first experimental evidence for infection-induced atherosclerosis was demonstrated in chickens using the herpes virus model (444). Later, many infectious agents were found to be associated with atherosclerosis. Interest in this topic has re-emerged because of several observations (445–451).
Vascular endothelial cells are critical targets for microbial pathogens and their activation by LPS results in the production of various inflammatory cytokines, chemokines, and cell adhesion molecules. The role of mast cells in these processes was demonstrated by a synergistic enhancement of LPS/LTA-induced production of IL-6 and IL-8 production by endothelial cells (48, 239). These amplifying effects have been shown to be due to histamine-induced overexpression of active TLR2 and TLR4 on endothelial cells (48). Furthermore, in a follow-up study, we demonstrated that LPS induces the expression of H1 receptor in endothelial cells (50). Collectively, these bidirectional effects of histamine and bacterial cell components lead to amplified inflammatory responses in the vascular endothelium via upregulation of the expression and TLR2, TLR4 and H1 receptors. Therefore, persistent infections together with mast cell degranulation provide ideal environments for the progression of atherosclerosis.
It is well-recognized that the immune response to bacterial products may recruit and sensitize inflammatory cells including mast cells, to potentiate localized inflammation. Microorganisms such as cytomegalovirus, Chlamydia pneumoniae and Helicobactor pylori are found in atherosclerotic lesions (426, 452, 453). The relationship between infection and inflammation to atherosclerosis has been further strengthened by the recognition of C. pneumoniae in patients with the disease (454–456). C. pneumoniae, which localizes in human atheroma, may contribute to inflammation during atherogenesis by activating endothelial cells, smooth muscle cells and macrophages (457–460). These findings are consistent with the risk of myocardial infarction and stroke observed in patients with systemic and respiratory tract infections (461). Therefore, it can be postulated that a cooperative action of bacterial products and mast cell mediators may amplify endothelial cell activation and progression of atherosclerosis.
7.3. Mast cells and inflammation linked to changes in microvessel integrity and, plaque growth and disruption
The development of atherosclerosis involves highly coordinated involvements of macrophages, mast cells and subsets of T cells (462–465). Mast cells strategically reside near microvessels, but very few are localized in the vascular intima. In contrast, the adventitia is densely populated with mast cells (466, 467). In conditions of persistent vascular inflammation and onset of atherosclerosis progression the mast cell population increases in the adventitia. Whether the increased mast cell population in the plaque region promotes atherogenesis or does it enhance plaque stability or both, is an open question. However, the available reports suggest that mast cells promote vascularization in atherosclerotic plaques by providing angiogenic factors such as VEGF-A and β-FGF as well as other proangiogenic mediators like heparin, tryptase and chymase (468, 469). Because neovascularization and vasodilation can improve oxygen supply to the affected hypoxic areas of the plaque, this process can stabilize the atherosclerotic plaque (470). It should be emphasized that mast cell proteases can degrade basement membranes of microvessels in the plaque causing intraplaque hemorrhage and plaque rupture (463).
Mast cells migrate into the plaque by the influence of chemokine CCL-1 present in the plaque, and CCR-2 expressed on the mast cell surface (217, 471). An increased number of tissue mast cells was suggested to be associated with thrombus formation mast cells (437). Mast cells in atherosclerotic plaques may also contribute to atherothrombosis through the formation of DNA extracellular traps (267, 472). Thus, the effects of activated mast cells in the neovascularized regions of atherosclerotic plaques appear to be both protective and harmful. This might be due to the unique characteristics of the mast cell to differentiate into different phenotypes based on the microenvironment. In this regard, mast cell mediators have been shown to play protective roles via immune homeostasis in a mouse model of systemic vasculitis (473).
Upon degranulation, mast cells release histamine and matrix-degrading proteases, which can cause microvessel leakage and rupture, leading to intraplaque hemorrhage. Mast cell activation during the progression of atherosclerosis has been shown to increase plaque size in the brachiocephalic artery of apoE-deficient mice (221). This response was prevented by administering cromolyn, a mast cell stabilizing agent. Role of mast cells in the progression of atherosclerosis has been further confirmed by findings that mast cell deficiency attenuated the development of atherosclerotic plaque in both LDL receptor-deficient LDLr−/− /KitW-sh/W−/sh mouse model (87) and apoE-deficient apoE−/−/KitW-sh/W−/sh mouse model (436).
The work summarized in this section supports a major role of mast cells in vascular inflammation, progression of atherosclerosis and plaque rupture. On the other hand, mast cells can exert protective effects in other vascular diseases such as systemic vasculitis. We believe that systemic vascular changes influence other organs. Tissue-specific resident mast cells participate in response to systemic vascular changes through sensing inflammation and then participate in the response mounted distally.
8. Mast cells mediate vascular inflammation caused by microbial infection and lifestyle choices such as cigarette smoking
Cigarette smoke extract-treated mast cells induce macrophage infiltration and M2 polarization (474). Recent studies have shown that cigarette smoke affects mast cell development and their response to activation in a TLR4-independent manner. Cigarette smoke attenuates the granularity and expression of surface c-kit and FcεRI in maturing mast cells resulting in decreased degranulation and release of Th1 and Th2 cytokines upon stimulation (475). Cigarette smoke induces the expression of chemokines and serine protease member S31 in mast cells (474).
Cigarette smoke increases susceptibility to bacterial infection and infection-related mortality among smokers (476–478) and mast cells respond to both cigarette smoke and microbial toxins. Since both endothelial cells and mast cells express TLRs that are activated by microbial toxins (48, 50, 479), pro-inflammatory constituents of cigarette smoke create conditions favorable for endothelial activation and mast cell degranulation. As mentioned previously, histamine activates endothelial cells via H1R (48, 436). These observations led us to propose that cigarette smoke amplifies activation of endothelial cells and inflammation through H1R-TLR2/4-COX2 axis (480). Thus, our results indicate a synergistic effect of cigarette smoke and the bacterial toxin LPS on endothelial cells in the presence of histamine.
9. Mast cells and viral infection with special reference to severe acute respiratory syndrome coronavirus-2 (SARS-CoV-2)
Research on the role of mast cells in viral infections is relatively new compared to the extensive work on bacterial infections and bacterial toxins. Viral infections are associated with mast cell-mediated aberrant inflammatory response, vascular leakage, and fibrosis (479, 481). Mast cells participate in host defense against viruses recruiting CD8+ T cells, which produce IFN-α and IFN-β. Dendritic cells function as antigen presenting cells and mast cells support dendritic cells for T-cell activation in adaptive immunity (321). Thus, while some of these actions are directly mediated by them, mast cells may also prompt or tune other innate, adaptive, or structural cells to engage in physiological actions. Influenza A virus infection was shown to activate mast cells leading to the release of histamine, proteases, leukotrienes, inflammatory cytokines, and antiviral chemokines (482). HIV-1 viral infection-mediated immunosuppression has been found to be linked to mast cell-released histamine (483).
The recent pandemic caused by SARS-CoV-2 appears to primarily affect the dense capillary network in the lungs causing high morbidity and mortality. Published data suggest a role for mast cells in the pulmonary complications associated with SARS-CoV-2 infection. Hyperactivation of proinflammatory cytokines [‘the cytokine storm’] is detected in nearly 20% patients with COVID-19 who experience a severe course of the infection for reasons [s] not well understood. Similar cytokine storms also characterize a multisystem disorder associated with idiopathic MCAS affecting approximately 17% population (301, 399). In line with that, higher numbers of mast cells were detected in the lungs of patients who died of COVID-19 infection (484).
SARS-CoV-2-triggered MC degranulation leading to alveolar epithelial inflammation and lung injury has been reported in ACE-1 humanized mice and rhesus macaques (485). Several molecules expressed/released by mast cells may contribute to the interaction between mast cells and SARS-CoV-2. Mast cells express the surface angiotensin converting enzyme 2 (ACE2) receptor of the renin-angiotensin system that is considered to be a candidate receptor for SARS-CoV-2 binding to cells (486, 487). A role for histamine released by mast cells in lung inflammation associated with SARS-CoV-2 infection was proposed almost immediately after the outbreak of the COVID-19 pandemic (75). Later, a report showed that histamine signaling through H2 receptor was essential for SARS-CoV-2 spike protein-mediated ACE2 internalization in endothelial cells (488). Mast cell-derived serine proteases, e.g., transmembrane serine protease 2 (TMPRSS2) facilitate the priming of the corona spike protein, and tryptase also has a role in SARS-CoV-2 infection (489, 490). Tryptase levels have been found to be associated with the severity of COVID-19 (491, 492). Tryptase secretion from mast cells activated via complement and IgE-dependent pathways is much higher compared to their direct activation via Mas-related G Protein coupled receptor X2 (MRGPRX2) (493). Tryptase hast been known to activate PAR2 on fibroblasts and induce collagen synthesis, fibroblast proliferation, and migration, airway remodeling/lung and development of fibrosis (494, 495). Although a direct link between mast cell proteases and matrix degrading metalloproteases (MMP) in COVID-19 remains to be established, elevated levels of plasma MMP2 and MMP9 have been shown to be associated with the severity and mortality of patients with COVID-19 (496). MMP2 and MMP9 were found to facilitate viral entry into the cell and were postulated to have a role in cytotoxicity of the virus and outcome of the disease (497). Increased MMPs (MMP8, MMP9, and MMP14) and matrix degradation in the lungs of mice infected with SARS-CoV-2 have been reported (497, 498). In support of activation of MMPs by tryptase, mast cell tryptase has been shown to activate lung MMP1 from pro-MMP1 in asthma and increase disease severity (499).
Aside from eicosanoids and other mast cell-released molecules, IL-1 is uniquely positioned to cause tissue damage due to its broad spectrum of biological effects and role in both innate and acquired immunity. Additionally, IL-1 induces multiple cytokines/chemokines in both macrophages and mast cells. Release of IL-1 following SARS-CoV-2 infection increases the levels of TNF, IL-6, and other cytokines. IL-1 also induces upregulation of nitric oxide, prostaglandins, and TXA2. Thus, IL-1 may be responsible for the ‘cytokine storm’ and inflammation, lung disease and death. Anti-IL-1 agents may be a valuable new line of treatment for SARS-CoV-2. In this regard, anti-inflammatory effects of IL-37, IL-1Rα are being studied to neutralize the effects and levels of IL-1 (75, 76).
Earlier studies have shown that, in addition to activation of monocytes/macrophages, dendritic cells, T cells, mast cells, and neutrophils, and the induction of cytokine storm in the lung, COVID-19 also affects other cells including neurons, glial cells, and endothelial cells thus causing neuroinflammation and psychological stress (500). A recent report showed the presence of CD117+ cells and IL-4-expressing cells in perivascular and alveolar septa that provide the interface between capillaries and the environment for gas exchange in the lungs in post-mortem biopsy samples of COVID-19 patients. The number of mast cells found in these biopsies were higher than previously reported in samples collected from patients with pandemic H1N1-induced pneumonia. Resulting upregulations of chemokine/cytokines cause alveolar injury and immune-thrombosis (484). However, it remains to be proven that endothelial dysfunction due to hyperactive mast cells results in interstitial edema in the alveolar septa triggering diffusion of pro-coagulative plasma factors leading to fibrin-dependent generation of the hyaline membrane. It is also noteworthy, that abnormally high mast cell activation has been observed in ‘long COVID/post-COVID syndrome’ where some patients experience lasting effects of SARS-CoV-2 infection in different organs (501, 502). A perspective on the involvement of mast cells in SARS-CoV-2-mediated organ damage is illustrated in Figure 3.
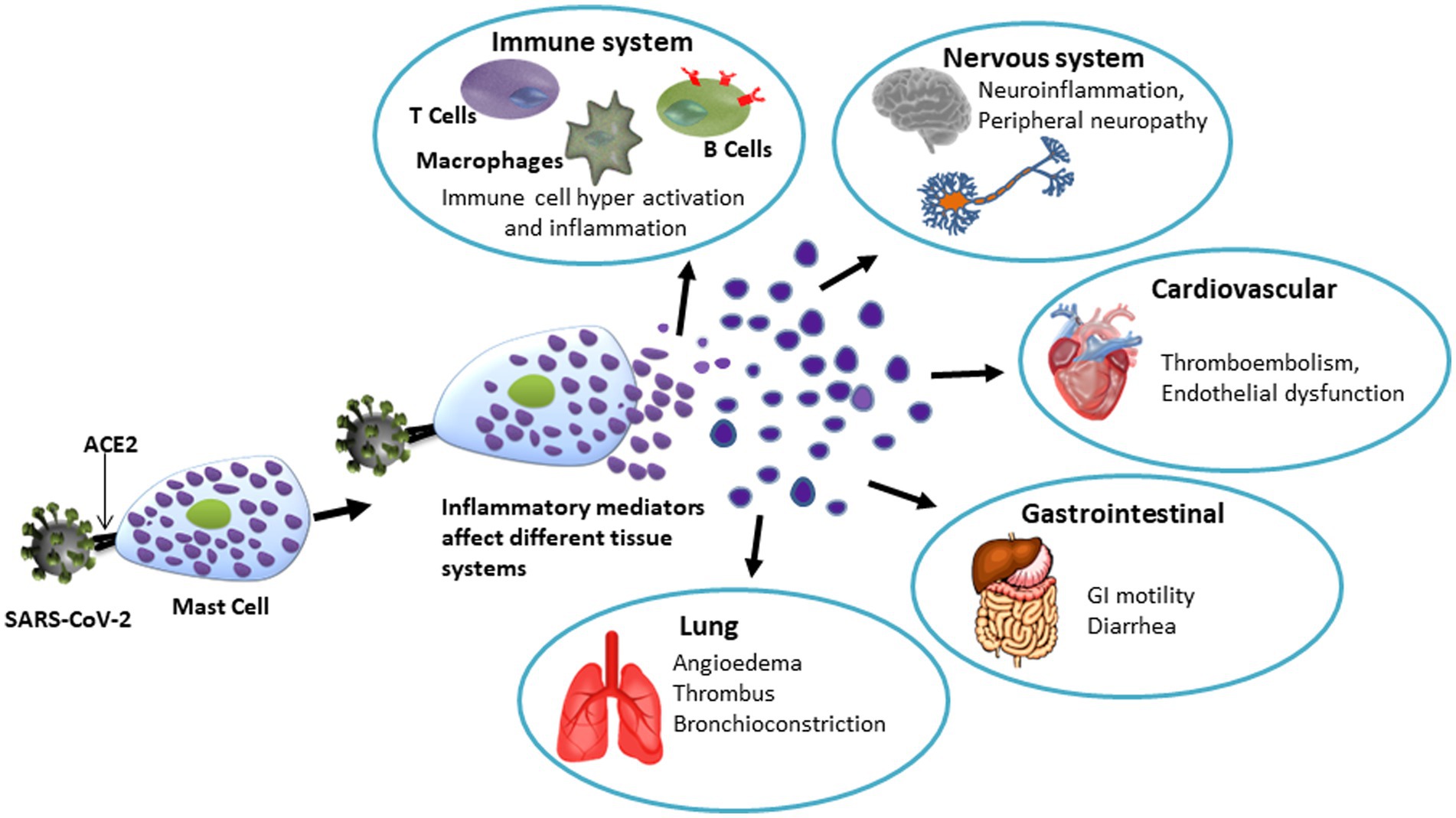
Figure 3. Schematic representation of the potential role of mast cells in SARS-CoV-12- induced tissue/organ damage. Spike protein of SARS-CoV-2 binds to ACE2 receptor on mast cells to cause degranulation. The hyperactive mast cells-derived granules contain various bioactive molecules that affect organ systems as mentioned. Notably, mast cell activation is now considered to be a key mechanism involved in various organ-related symptoms indicating long COVID.
10. Mast cells as effectors for integrating systemic inflammation, neuroinflammation, and neurodegenerative diseases
In the brain, mast cells are mostly localized in the immediate vicinity of blood vessels and cerebrospinal fluid (CSF)-containing spaces (503) notable in the hippocampus and thalamic hypothalamic region, 3rd ventricle, choroid plexus and innermost meninges (leptomeninges) (504–508). The number of mast cells in the human brain is low compared to the rodent brain and these cells are found mostly along meninges and perivascular regions (509–511). Studies using rat and human brain tissues show that majority of mast cells (>95%) localize on the abluminal side of blood vessel (brain side) during development (507, 512). However, peripherally injected mast cells were also found to enter the basal lamina (matrix) of blood vessels and localize close to astrocyte (astroglial) processes and microglia (506, 513, 514). Such localization allows mast cells to perform their role as transducers of signals and as first line defenders (515). Activation and proliferation of mast cells due to injury-induced derangement of blood–brain barrier in traumatic brain injury (TBI) suggests that mast cells may be potential targets for therapeutic approaches (516).
10.1. Mast cell-microglial interactions
Interactions between mast cells and microglia are a subject of growing research interest because unique structural and functional features of microglia allow mast cells to influence both physiological functions as well as pathological changes (511, 517). Structural and molecular features enable reciprocal interaction between mast cells and microglia (518) discussed under the following three categories (a-c).
10.1.1. Reciprocal interaction between mast cells and microglia
(a) P2 channel/receptor activation by ATP increases IL-33 release in activated microglia (by PAMPs via TLRs). In turn, IL-33 binding to mast cells induces IL-6, IL-13 and MCP-1 secretion that modulates microglial function (519–521). (b) activation of TLR2/4 induces phagocytosis and production of IL-6 and CCL5 in microglia that induce TLR2/4 expression in mast cells. In turn, activation of TLR2/4 in mast cells upregulates cytokine/chemokine formation. CCL5/RANTES activates microglia and promotes immune cell movement to the location of injury (522, 523). (c) Mast cell tryptase activates PAR2 in microglia causing increased synthesis and release of BDNP, increased release of pro-inflammatory molecules (IL-6 and TNF) by upregulating MAPK-NF-kB. In turn, IL-6 and TNF from microglia upregulate PAR2 on mast cells (524).
10.1.2. Mast cells and microglia as dual targets of inflammatory molecules/conditions
(a) Binding of chemokine (CXCL12) to its receptor (CXCR4) induces microglial activation and mobility under ischemia/hypoxia. CXCL12 is also a chemotaxin for mast cells (525). (b) Co-culture of mast cells with glial cells caused increased synthesis of CCL2 and IL-33 by mast cells and acceleration of Aβ oligomerization by microglia. CCL2 (monocyte chemoattractant protein-1 or MCP-1), and its receptor CCR2 are expressed on monocytes. Several recent studies suggest increased CCL2 in Alzheimer’s disease (AD) (516). Elucidation of the significance of CCL2-CCR2 axis in AD requires further studies. (c) Neuroinflammation induces release of complement protein C5a and upregulation of C5aR in microglia. Similarly, activation of mast cells is associated with C5aR upregulation attracting C5a peptides. Both cell types show crosstalk between C5a and TLR4 (526).
10.1.3. Activated mast cells modulate microglial function
(i) Experimental release of granules from mast cells upregulates histamine receptors, MAPK, AKT proteins and cytokine production in hypothalamic microglia (511, 527). (ii) Mast cell-released histamine elicits a pro-inflammatory response in quiescent microglia through H1 receptor and an anti-inflammatory response in activated microglia through the H4 receptor (528, 529).
10.2. Significance of mast cell-neural cell interactions in CNS pathophysiology
Neuroinflammation drives the processes leading to acute as well as progressive neurodegeneration. A growing number of studies suggest that neuroinflammation is not isolated from peripheral conditions and that mast cells may play a significant role through their interaction with neurons, astrocytes, and glia, particularly with microglia (see above).
Increased numbers and activation of brain mast cells are associated with various neuropathological changes starting with increased blood brain permeability and leading to neurodegenerative diseases. In addition, their activation in response to physical injury and inflammatory conditions, the role of mast cells in the pathophysiology of neuropsychiatric conditions is also a subject of growing interest (530). Due to their ability to respond in a very short time, mast cells have been considered as potential ‘first responders’ (531). The following outlines the significance of mast cells in selected neuropathological conditions including TBI, stroke, Alzheimer’s disease, and certain neuropsychological disorders.
10.2.1. Traumatic brain injury (TBI), hypoxia, ischemia, and intracranial hemorrhage
Neuroinflammation is associated with TBI that may lead to neurodegenerative changes often observed in AD-like dementias. Tissue injury due to hypoxia, ischemia (stroke) and intracranial hemorrhage also results in mast cell accumulation and degranulation with common features. Persistent inflammation due to moderate and severe TBI causes progressive tissue damage and has been linked to a greater susceptibility to AD-like dementia (532–534). Long term effects of TBI in humans and mouse models include cognitive deficit, deposition of amyloid precursor protein (APP), amyloid-beta peptide and tau protein intracellular neurofibrillary tangles (535, 536).
Immediate increases in the number and degranulation of mast cells in the injured brain area have been demonstrated in experimental models. Mast cell degranulation were shown to start immediately after TBI in a mouse model (537). Mast cell numbers increase rapidly along with the release of mediators including histamine, heparin, leukotrienes and tryptase and changes in histamine receptors (decreased H1, H3) and superoxide dismutase (SOD) in the brain (thalamic region) after TBI in rats (538). Persistent high number and degranulation of mast cells in the dura in a mouse model was correlated with post-traumatic headache in TBI patients (539).
Ischemia/hypoxia involve increased numbers and activation of mast cells indicated by increased brain histamine levels and increased cytokines (540, 541). These changes result in increased blood brain permeability (542, 543), interaction with microglia and influx of peripheral leukocyte leading to prolonged inflammation and consequent demyelination (508, 544, 545). Changes in the blood brain barrier characteristics are invariably linked to its constituents, namely endothelial cells and astrocytes (546). Due to shared tissue changes and injury, mast cells are considered as targets for treating TBI, hypoxia-ischemia, ischemic stroke, and intracranial hemorrhage (547).
10.2.2. Alzheimer’s disease (AD)
Inflammation in Alzheimer’s disease has been a subject of ongoing investigation and the role of mast cells in neuroinflammatory processes of AD continues to be studied (548–550). Higher numbers of tryptase positive mast cells and metallothionein positive astrocytes have been observed near amyloid plaques in patients with AD (551). Likewise, microglial clusters around plaques have been reported in human specimens as well as in mouse models (552). Glial clusters secrete proteins and chemoattractant for mast cells (552). However, the idea of mast cells being ‘first responders’ suggests that these cells reach the plaque site and activate microglia and other cells (553). In either case, as discussed above, a reciprocal interaction between the two cell types is clear. In a rodent model (APPswe/PS1dE9 mice) of AD, increased numbers of mast cells were reported in the hippocampus and cerebral cortex even before amyloid plaques were observed. This led the authors to hypothesize that mast cells act as early sensors of amyloid peptide and facilitate mobilization of other cells to the site of inflammation promoting the onset and progression of AD (554). Cytokines including TNF, IL-1β, and IL-6 released by mast cells can contribute to toxic neuroinflammation as well as to cyto-protective processes determined by their levels and duration of expression. These cytokines may affect tight junction proteins resulting in altered blood brain barrier permeability and mobilization of circulating pro-inflammatory cells and molecules. Attenuation of cognitive decline by tyrosine kinase inhibitor masitinib that targets mast cell through the receptor c-kit and Lyn tyrosine kinase was concluded to indicate a role of mast cell-released mediators in progression of AD (555, 556).
10.2.3. Anxiety, depression, and behavioral disorders
Cross-sectional human studies showed association of food allergies with anxiety (557) attention deficit and hyperactivity disorder (558) and anorexia nervosa (559, 560). Analysis of data on food allergies in children showed association of these conditions with changes in emotions and behavior. Using a mouse model of food allergy authors showed increased cortisone levels, changes in brain areas associated with emotional and affective behavior (anxiety and stress responses) that were partially mediated by C-sensitive afferent inputs and mast cells (561). Increased incidence of headache (migraine) anxiety, depression, and cognitive changes in human subjects have been reported to associate with mastocytosis (562, 563).
11. Concluding comments and perspectives
Mast cells are recognized for their role in maintaining immune homeostasis in health as well as in the pathobiology of many diseases. They are strategically residing throughout the body in almost all organs. Most of the published work has focused on their roles in skin, connective tissues, blood vessels, mucosa, lungs, and heart. Mast cells function as a cellular interface between the external and internal environments and initiate and coordinate innate and adaptive immune responses by interacting with a variety of cell types. The ability of the mast cell to act both as a sensor and responder while maintaining flexible mobility and adaptability enables this “master cell” to play a leading role in the complex process of immune homeostasis. In this article we highlighted selected functions of the mast cell to describe how these functional alterations affect the pathobiology of diseases.
Our work has been focused on understanding the mechanisms of mast-cell mediated immune alterations in cardiovascular diseases. In this regard, the synergy between mast cells and endothelial cells and the suppressive effects of mast cells on macrophage functions are intriguing findings. We have coined a novel term “H1R-TLR-COX2 axis” to describe these interactions. Chronic or sub-clinical infections and lifestyle choices such as cigarette smoking add additional dimensions to innate immune upregulation in cardiovascular diseases.
We also wish to bring attention to the role that mast cells play in angiogenesis which may have implications for tissue growth and wound healing. A brief review of their role in tissue repair processes points to future success of the ongoing efforts in exploring the potential using mast cells as target for developing novel treatments for diabetic wounds. On the flip side, inhibition of the pro-angiogenic role of mast cells is being explored for suppressing tumor growth. In this regard, we believe there will be much to gain by understanding the selective secretion of mediators by mast cells.
We hope that discussion on the role of mast cells as ‘first responders’ in neuroinflammation will stimulate greater interest in the field of pathobiology of neurological diseases. The significant influence of mast cells on both sides of the blood–brain barrier adds a new dimension to the field of developing therapeutic strategies for systemic vascular biology dysfunctions in cardiovascular and neurovascular diseases.
We believe that mast cells stand in the forefront of candidates for future work for exploring the impact of immunomodulatory dysfunctions in health and disease. It is nature’s rule that there are no “Good Guys” or “Bad Guys,” but it is the environment that influences the switch. Although a great amount of information has been gathered regarding the physiological role of mast cells, much remains to be learned about their participation as the ‘good guys’. Perhaps a better understanding at the epigenomic level will enable a greater utilization of their anti-inflammatory functions at the interface of tissues and the vasculature.
Author contributions
KND, RaS, VVR, and MS have collaborated on several projects and proposed the premise for this article. MS, VVR, and KND revised the manuscript and figures. MS, VVR, and KND completed the final version. All authors contributed specifically to individual area of specialty, participated in revising the manuscript, contributed to the article, and approved the submitted version.
Funding
Most of our research work cited in this article was supported by grants from NIH (R01-HL070101 and 3R01-HL070101-04S1), American Heart Association, Joseph and Elizabeth Carey Arthritis Fund, Lied Foundation, and the Audrey E. Smith Medical Research Fund from the University of Kansas Endowment Association (KD). MS received support, in part, from the Midwest Veterans’ Biomedical Research Foundation (MVBRF) and the Kansas City VA Medical Center, Kansas City, MO.
Acknowledgments
Authors gratefully acknowledge the scientific contributions of many postdoctoral fellows and laboratory staff for the past three decades. Their contributions are cited in this article. The views expressed in this article are those of the authors and do not necessarily reflect the position or policy of the Department of Veterans Affairs or the United States government. Authors thank Connie Wallace for critically reading the manuscript.
Conflict of interest
The authors declare that the research was conducted in the absence of any commercial or financial relationships that could be construed as a potential conflict of interest.
Publisher’s note
All claims expressed in this article are solely those of the authors and do not necessarily represent those of their affiliated organizations, or those of the publisher, the editors and the reviewers. Any product that may be evaluated in this article, or claim that may be made by its manufacturer, is not guaranteed or endorsed by the publisher.
References
1. Turvey, SE , and Broide, DH . Innate immunity. J Allergy Clin Immunol. (2010) 125:S24–32. doi: 10.1016/j.jaci.2009.07.016
2. Krystel-Whittemore, M , Dileepan, KN , and Wood, JG . Mast cell: a multi-functional master cell. Front Immunol. (2015) 6:620. doi: 10.3389/fimmu.2015.00620
3. Ehrlich, P . Beitraege Zur Kenntniss Der Anilinfaerbungen Und Ihrer Verwendung in Der Mikroskopischen Technik. Arch mikr anat u entwcklngsmech. (1877) 13:263–77. doi: 10.1007/BF02933937
4. Saito, H , Matsumoto, K , Okumura, S , Kashiwakura, J-i , Oboki, K , Yokoi, H, et al. Gene expression profiling of human mast cell subtypes: an in silico study. Allergol Int. (2006) 55:173–9. doi: 10.2332/allergolint.55.173
5. Quackenbush, EJ , Wershil, BK , Aguirre, V , and Gutierrez-Ramos, JC . Eotaxin modulates Myelopoiesis and mast cell development from embryonic hematopoietic progenitors. Blood. (1998) 92:1887–97. doi: 10.1182/blood.V92.6.1887
6. Butterfield, JH , Weiler, D , Dewald, G , and Gleich, GJ . Establishment of an immature mast cell line from a patient with mast cell leukemia. Leuk Res. (1988) 12:345–55. doi: 10.1016/0145-2126(88)90050-1
7. Kirshenbaum, AS , Akin, C , Wu, Y , Rottem, M , Goff, JP , Beaven, MA, et al. Characterization of novel stem cell factor responsive human mast cell lines Lad1 and 2 established from a patient with mast cell sarcoma/leukemia; activation following aggregation of Fcepsilonri or Fcgammari. Leuk Res. (2003) 27:677–82. doi: 10.1016/S0145-2126(02)00343-0
8. Rottem, M , Kirshenbaum, AS , and Metcalfe, DD . Early development of mast cells. Int Arch Allergy Appl Immunol. (1991) 94:9. doi: 10.1159/000235339
9. Nilsson, G , Blom, T , Kusche-Gullberg, M , Kjellen, L , Butterfield, JH , Sundstrom, C, et al. Phenotypic characterization of the human mast-cell line HMC-1. Scand J Immunol. (1994) 39:489–98. doi: 10.1111/j.1365-3083.1994.tb03404.x
10. Kaltenbach, L , Martzloff, P , Bambach, SK , Aizarani, N , Mihlan, M , Gavrilov, A, et al. Slow integrin-dependent migration organizes networks of tissue-resident mast cells. Nat Immunol. (2023) 24:915–24. doi: 10.1038/s41590-023-01493-2
11. Abonia, JP , Austen, KF , Rollins, BJ , Joshi, SK , Flavell, RA , Kuziel, WA, et al. Constitutive homing of mast cell progenitors to the intestine depends on autologous expression of the chemokine receptor CXCR2. Blood. (2005) 105:4308–13. doi: 10.1182/blood-2004-09-3578
12. Hallgren, J , and Gurish, MF . Mast cell progenitor trafficking and maturation In: AM Gilfillan and DD Metcalfe, editors. Mast cell biology: contemporary and emerging topics. Boston, MA: Springer US (2011). 14–28.
13. Burton, OT , Epp, A , Fanny, ME , Miller, SJ , Stranks, AJ , Teague, JE, et al. Tissue-specific expression of the low-affinity IgG receptor, FcγRIIb, on human mast cells. Front Immunol. (2018) 9:1244. doi: 10.3389/fimmu.2018.01244
14. Plotkin, JD , Elias, MG , Fereydouni, M , Daniels-Wells, TR , Dellinger, AL , Penichet, ML, et al. Human mast cells from adipose tissue target and induce apoptosis of breast Cancer cells. Front Immunol. (2019) 10:138. doi: 10.3389/fimmu.2019.00138
15. Fong, M , and Crane, JS . Histology, mast cells. Stat pearls publishing copyright © 2023. Treasure Island, FL: Stat pearls publishing LLC (2023).
16. Poglio, S , De Toni-Costes, F , Arnaud, E , Laharrague, P , Espinosa, E , Casteilla, L, et al. Adipose tissue as a dedicated reservoir of functional mast cell progenitors. Stem Cells. (2010) 28:2065–72. doi: 10.1002/stem.523
17. Burwen, SJ . Recycling of mast cells following degranulation in vitro: an ultrastructural study. Tissue Cell. (1982) 14:125–34. doi: 10.1016/0040-8166(82)90012-X
18. Raj, S , and Unsworth, LD . Targeting active sites of inflammation using inherent properties of tissue-resident mast cells. Acta Biomater. (2023) 159:21–37. doi: 10.1016/j.actbio.2023.01.024
19. Gaudenzio, N , Sibilano, R , Marichal, T , Starkl, P , Reber, LL , Cenac, N, et al. Different activation signals induce distinct mast cell degranulation strategies. J Clin Invest. (2016) 126:3981–98. doi: 10.1172/JCI85538
20. von Kockritz-Blickwede, M , Goldmann, O , Thulin, P , Heinemann, K , Norrby-Teglund, A , Rohde, M, et al. Phagocytosis-independent antimicrobial activity of mast cells by means of extracellular trap formation. Blood. (2008) 111:3070–80. doi: 10.1182/blood-2007-07-104018
21. Mollerherm, H , von Kockritz-Blickwede, M , and Branitzki-Heinemann, K . Antimicrobial activity of mast cells: role and relevance of extracellular DNA traps. Front Immunol. (2016) 7:265. doi: 10.3389/fimmu.2016.00265
22. Elieh Ali Komi, D , and Kuebler, WM . Significance of mast cell formed extracellular traps in microbial defense. Clin Rev Allergy Immunol. (2022) 62:160–79. doi: 10.1007/s12016-021-08861-6
23. Metz, M , Piliponsky, AM , Chen, CC , Lammel, V , Abrink, M , Pejler, G, et al. Mast cells can enhance resistance to Snake and honeybee venoms. Science. (2006) 313:526–30. doi: 10.1126/science.1128877
24. Galli, SJ , Gaudenzio, N , and Tsai, M . Mast cells in inflammation and disease: recent Progress and ongoing concerns. Annu Rev Immunol. (2020) 38:49–77. doi: 10.1146/annurev-immunol-071719-094903
25. Kundu, D , Kennedy, L , Meadows, V , Baiocchi, L , Alpini, G , and Francis, H . The dynamic interplay between mast cells, aging/cellular senescence and liver disease. Gene Expr. (2020) 20:77–88. doi: 10.3727/105221620X15960509906371
26. Vukman, KV , Forsonits, A , Oszvald, A , Toth, EA , and Buzas, EI . Mast cell Secretome: soluble and vesicular components. Semin Cell Dev Biol. (2017) 67:65–73. doi: 10.1016/j.semcdb.2017.02.002
27. Moon, TC , Befus, AD , and Kulka, M . Mast cell mediators: their differential release and the secretory pathways involved. Front Immunol. (2014) 5:569. doi: 10.3389/fimmu.2014.00569
28. McHale, C , Mohammed, Z , and Gomez, G . Human skin-derived mast cells spontaneously secrete several angiogenesis-related factors. Front Immunol. (2019) 10:1445. doi: 10.3389/fimmu.2019.01445
29. Riley, JF . Histamine in tissue mast cells. Science. (1953) 118:332–3. doi: 10.1126/science.118.3064.332
30. Rothschild, AM , and Schayer, RW . Characterization of histidine decarboxylase from rat peritoneal fluid mast cells. Biochim Biophys Acta. (1959) 34:392–8. doi: 10.1016/0006-3002(59)90291-4
31. Ringvall, M , Rönnberg, E , Wernersson, S , Duelli, A , Henningsson, F , Åbrink, M, et al. Serotonin and histamine storage in mast cell secretory granules is dependent on Serglycin proteoglycan. J Allergy Clin Immunol. (2008) 121:1020–6. doi: 10.1016/j.jaci.2007.11.031
32. Sjoerdsma, A , Waalkes, TP , and Weissbach, H . Serotonin and histamine in mast cells. Science. (1957) 125:1202–3. doi: 10.1126/science.125.3259.1202
33. Caughey, GH . Mast cell proteases as pharmacological targets. Eur J Pharmacol. (2016) 778:44–55. doi: 10.1016/j.ejphar.2015.04.045
34. Pejler, G , Åbrink, M , Ringvall, M , and Wernersson, S . Mast cell proteases. Adv Immunol. (2007) 95:167–255. doi: 10.1016/S0065-2776(07)95006-3
35. Rönnberg, E , Melo, FR , and Pejler, G . Mast cell proteoglycans. J Histochem Cytochem. (2012) 60:950–62. doi: 10.1369/0022155412458927
36. Galli, SJ , Gordon, JR , and Wershil, BK . Cytokine production by mast cells and basophils. Curr Opin Immunol. (1991) 3:865–72. doi: 10.1016/S0952-7915(05)80005-6
37. Galli, SJ , Gordon, JR , and Wershil, BK . Mast cell cytokines in allergy and inflammation. Agents Actions Suppl. (1993) 43:209–20. doi: 10.1007/978-3-0348-7324-6_18
38. Mukai, K , Tsai, M , Saito, H , and Galli, SJ . Mast cells as sources of cytokines, chemokines, and growth factors. Immunol Rev. (2018) 282:121–50. doi: 10.1111/imr.12634
39. Tore, F , and Tuncel, N . Mast cells: target and source of neuropeptides. Curr Pharm Des. (2009) 15:3433–45. doi: 10.2174/138161209789105036
40. Luu Quoc, Q , Moon, JY , Lee, DH , Ban, GY , Kim, SH , and Park, HS . Role of Thymus and activation-regulated chemokine in allergic asthma. J Asthma Allergy. (2022) 15:157–67. doi: 10.2147/JAA.S351720
41. Jimenez, M , Cervantes-Garcia, D , Cordova-Davalos, LE , Perez-Rodriguez, MJ , Gonzalez-Espinosa, C , and Salinas, E . Responses of mast cells to pathogens: beneficial and detrimental roles. Front Immunol. (2021) 12:685865. doi: 10.3389/fimmu.2021.685865
42. Dale, HH , and Richards, AN . The vasodilator action of histamine and of some other substances. J Physiol. (1918) 52:110–65. doi: 10.1113/jphysiol.1918.sp001825
43. Mortillaro, N , Granger, D , Kvietys, P , Rutili, G , and Taylor, A . Effects of histamine and histamine antagonists on intestinal capillary permeability. Am J Physiol. (1981) 240:G381–6. doi: 10.1152/ajpgi.1981.240.5.G381
44. Clark, RA , Gallin, JI , and Kaplan, AP . The selective eosinophil chemotactic activity of histamine. J Exp Med. (1975) 142:1462–76. doi: 10.1084/jem.142.6.1462
45. Ogden, BE , and Hill, HR . Histamine regulates lymphocyte Mitogenic responses through activation of specific H1 and H2 histamine receptors. Immunology. (1980) 41:107–14.
46. Beer, DJ , Matloff, SM , and Rocklin, RE . The influence of histamine on immune and inflammatory responses. Adv Immunol. (1984) 35:209–68. doi: 10.1016/s0065-2776(08)60577-5
47. Li, Y , Chi, L , Stechschulte, DJ , and Dileepan, KN . Histamine-induced production of interleukin-6 and interleukin-8 by human coronary artery endothelial cells is enhanced by endotoxin and tumor necrosis factor-alpha. Microvasc Res. (2001) 61:253–62. doi: 10.1006/mvre.2001.2304
48. Talreja, J , Kabir, MH , Bf, M , Stechschulte, DJ , and Dileepan, KN . Histamine induces toll-like receptor 2 and 4 expression in endothelial cells and enhances sensitivity to Gram-positive and Gram-negative bacterial cell wall components. Immunology. (2004) 113:224–33. doi: 10.1111/j.1365-2567.2004.01946.x
49. Tan, X , Essengue, S , Talreja, J , Reese, J , Stechschulte, DJ , and Dileepan, KN . Histamine directly and synergistically with lipopolysaccharide stimulates cyclooxygenase-2 expression and prostaglandin I(2) and E(2) production in human coronary artery endothelial cells. J Immunol. (2007) 179:7899–906. doi: 10.4049/jimmunol.179.11.7899
50. Raveendran, VV , Tan, X , Sweeney, ME , Levant, B , Slusser, J , Stechschulte, DJ, et al. Lipopolysaccharide induces H1 receptor expression and enhances histamine responsiveness in human coronary artery endothelial cells. Immunology. (2011) 132:578–88. doi: 10.1111/j.1365-2567.2010.03403.x
51. White, MV . The role of histamine in allergic diseases. J Allergy Clin Immunol. (1990) 86:599–605. doi: 10.1016/S0091-6749(05)80223-4
52. White, MV , Slater, JE , and Kaliner, MA . Histamine and asthma. Am Rev Respir Dis. (1987) 135:1165–76. doi: 10.1164/arrd.1987.135.5.1165
53. Behrendt, H , and Ring, J . Histamine, antihistamines and atopic eczema. Clin Exp Allergy. (1990) 20:25–30. doi: 10.1111/j.1365-2222.1990.tb02473.x
54. Zuberbier, T , Schwarz, S , Hartmann, K , Pfrommer, C , and Czarnetzki, B . Histamine releasability of basophils and skin mast cells in chronic Urticaria. Allergy. (1996) 51:24–8. doi: 10.1111/j.1398-9995.1996.tb04545.x
55. Lieberman, P , and Garvey, LH . Mast cells and anaphylaxis. Curr Allergy Asthma Rep. (2016) 16:1–7. doi: 10.1007/s11882-016-0598-5
56. Friedman, BS , Steinberg, SC , Meggs, WJ , Kaliner, MA , Frieri, M , and Metcalfe, DD . Analysis of plasma histamine levels in patients with mast cell disorders. Am J Med. (1989) 87:649–54. doi: 10.1016/S0002-9343(89)80398-5
57. Kalsner, S , and Richards, R . Coronary arteries of cardiac patients are Hyperreactive and contain Stores of Amines: a mechanism for coronary spasm. Science. (1984) 223:1435–7. doi: 10.1126/science.6701530
58. Cabanie, M , and Godfraind, T . The role of histamine in the cardiovascular system. Drugs Exp Clin Res. (1988) 14:141–7.
59. Smith, DD , Tan, X , Tawfik, O , Milne, G , Stechschulte, DJ , and Dileepan, KN . Increased aortic atherosclerotic plaque development in female apolipoprotein E-null mice is associated with elevated thromboxane A2 and decreased prostacyclin production. J Physiol Pharmacol. (2010) 61:309–16.
60. Raveendran, VV , Smith, DD , Tan, X , Sweeney, ME , Reed, GA , Flynn, CA, et al. Chronic ingestion of H1-antihistamines increase progression of atherosclerosis in apolipoprotein E−/− mice. PLoS One. (2014) 9:e102165. doi: 10.1371/journal.pone.0102165
61. Zhang, J , Cai, WK , Zhang, Z , Wang, P , Lin, XQ , Feng, J, et al. Cardioprotective effect of histamine H2 antagonists in congestive heart failure: a systematic review and meta-analysis. Medicine (Baltimore). (2018) 97:e0409. doi: 10.1097/MD.0000000000010409
62. Curry, JJ . The effect of antihistamine substances and other drugs on histamine bronchoconstriction in asthmatic subjects. J Clin Invest. (1946) 25:792–9. doi: 10.1172/JCI101765
63. Branco, ACCC , Yoshikawa, FSY , Pietrobon, AJ , and Sato, MN . Role of histamine in modulating the immune response and inflammation. Mediat Inflamm. (2018) 2018:9524075. doi: 10.1155/2018/9524075
64. Jutel, M , Blaser, K , and Akdis, CA . Histamine in chronic allergic responses. J Investig Allergol Clin Immunol. (2005) 15:1–8.
65. De Winter, BY , van den Wijngaard, RM , and de Jonge, WJ . Intestinal mast cells in gut inflammation and motility disturbances. Biochim Biophys Acta. (2012) 1822:66–73. doi: 10.1016/j.bbadis.2011.03.016
66. Robles, A , Perez Ingles, D , Myneedu, K , Deoker, A , Sarosiek, I , Zuckerman, MJ, et al. Mast cells are increased in the small intestinal mucosa of patients with irritable bowel syndrome: a systematic review and Meta-analysis. Neurogastroenterol Motil. (2019) 31:e13718. doi: 10.1111/nmo.13718
67. Panarelli, NC , Hornick, JL , and Yantiss, RK . What is the value of counting mast cells in gastrointestinal mucosal biopsies? Mod Pathol. (2023):100005. doi: 10.1016/j.modpat.2022.100005
68. Gupta, K , and Harvima, IT . Mast cell-neural interactions contribute to pain and itch. Immunol Rev. (2018) 282:168–87. doi: 10.1111/imr.12622
69. Bienenstock, J , Tomioka, M , Matsuda, H , Stead, RH , Quinonez, G , Simon, GT, et al. The role of mast cells in inflammatory processes: evidence for nerve/mast cell interactions. Int Arch Allergy Immunol. (1987) 82:238–43. doi: 10.1159/000234197
70. Yuan, H , and Silberstein, SD . Histamine and Migraine. Headache. (2018) 58:184–93. doi: 10.1111/head.13164
71. Guan, LC , Dong, X , and Green, DP . Roles of mast cells and their interactions with the trigeminal nerve in migraine headache. Mol Pain. (2023) 19:17448069231181358. doi: 10.1177/17448069231181358
72. Blanco, I , Béritze, N , Argüelles, M , Cárcaba, V , Fernández, F , Janciauskiene, S, et al. Abnormal overexpression of mastocytes in skin biopsies of fibromyalgia patients. Clin Rheumatol. (2010) 29:1403–12. doi: 10.1007/s10067-010-1474-7
73. Mekori, YA , and Metcalfe, DD . Mast cell-T cell interactions. J Allergy Clin Immunol. (1999) 104:517–23. doi: 10.1016/s0091-6749(99)70316-7
74. Theoharides, TC , Kempuraj, D , Tagen, M , Conti, P , and Kalogeromitros, D . Differential release of mast cell mediators and the pathogenesis of inflammation. Immunol Rev. (2007) 217:65–78. doi: 10.1111/j.1600-065X.2007.00519.x
75. Conti, P , Caraffa, A , Gallenga, CE , Ross, R , Kritas, SK , Frydas, I, et al. Coronavirus-19 (SARS-CoV-2) induces acute severe lung inflammation via IL-1 causing cytokine storm in COVID-19: a promising inhibitory strategy. J Biol Regul Homeost Agents. (2020) 34:1971–5. doi: 10.23812/20-1-E
76. Theoharides, TC . COVID-19, pulmonary mast cells, cytokine storms, and beneficial actions of Luteolin. Biofactors. (2020) 46:306–8. doi: 10.1002/biof.1633
77. Nigrovic, PA , Binstadt, BA , Monach, PA , Johnsen, A , Gurish, M , Iwakura, Y, et al. Mast cells contribute to initiation of autoantibody-mediated arthritis via IL-1. Proc Natl Acad Sci. (2007) 104:2325–30. doi: 10.1073/pnas.0610852103
78. Salamon, P , Shefler, I , Moshkovits, I , Munitz, A , Horwitz Klotzman, D , Mekori, Y, et al. IL-33 and IgE stimulate mast cell production of IL-2 and regulatory T cell expansion in allergic dermatitis. Clin Exp Allergy. (2017) 47:1409–16. doi: 10.1111/cea.13027
79. Hershko Alon, Y , Suzuki, R , Charles, N , Alvarez-Errico, D , Sargent Jennifer, L , Laurence, A, et al. Mast cell Interleukin-2 production contributes to suppression of chronic allergic dermatitis. Immunity. (2011) 35:562–71. doi: 10.1016/j.immuni.2011.07.013
80. Bradding, P , Feather, I , Wilson, S , Bardin, P , Heusser, C , Holgate, S, et al. Immunolocalization of cytokines in the nasal mucosa of normal and perennial Rhinitic subjects. The mast cell as a source of IL-4, IL-5, and IL-6 in human allergic mucosal inflammation. J Immunol. (1993) 151:3853–65.
81. Trautmann, A , Krohne, G , Bröcker, E-B , and Klein, CE . Human mast cells augment fibroblast proliferation by heterotypic cell-cell adhesion and action of IL-4. J Immunol. (1998) 160:5053–7. doi: 10.4049/jimmunol.160.10.5053
82. Shakoory, B , Fitzgerald, SM , Lee, SA , Chi, DS , and Krishnaswamy, G . The role of human mast cell-derived cytokines in eosinophil biology. J Interf Cytokine Res. (2004) 24:271–81. doi: 10.1089/107999004323065057
83. Choy, E , and Rose-John, S . Interleukin-6 as a multifunctional regulator: inflammation, immune response, and fibrosis. J Scleroderma Relat Disord. (2017) 2:S1–5. doi: 10.5301/jsrd.5000
84. Dahdah, A , Gautier, G , Attout, T , Fiore, F , Lebourdais, E , Msallam, R, et al. Mast cells aggravate Sepsis by inhibiting peritoneal macrophage phagocytosis. J Clin Invest. (2014) 124:4577–89. doi: 10.1172/JCI75212
85. Krüger-Krasagakes, S , Möller, A , Kolde, G , Lippert, U , Weber, M , and Henz, BM . Production of interleukin-6 by human mast cells and basophilic cells. J Investig Dermatol. (1996) 106:75–9. doi: 10.1111/1523-1747.ep12327815
86. Seino, Y , Ikeda, U , Ikeda, M , Yamamoto, K , Misawa, Y , Hasegawa, T, et al. Interleukin 6 gene transcripts are expressed in human atherosclerotic lesions. Cytokine. (1994) 6:87–91. doi: 10.1016/1043-4666(94)90013-2
87. Sun, J , Sukhova, GK , Wolters, PJ , Yang, M , Kitamoto, S , Libby, P, et al. Mast cells promote atherosclerosis by releasing Proinflammatory cytokines. Nat Med. (2007) 13:719–24. doi: 10.1038/nm1601
88. Huang, B , Lei, Z , Zhang, GM , Li, D , Song, C , Li, B, et al. SCF-mediated mast cell infiltration and activation exacerbate the inflammation and immunosuppression in tumor microenvironment. Blood. (2008) 112:1269–79. doi: 10.1182/blood-2008-03-147033
89. Baggiolini, M , Walz, A , and Kunkel, SL . Neutrophil-activating Peptide-1/interleukin 8, a novel cytokine that activates neutrophils. J Clin Invest. (1989) 84:1045–9. doi: 10.1172/JCI114265
90. Oppenheim, JJ , Zachariae, CO , Mukaida, N , and Matsushima, K . Properties of the novel proinflammatory supergene "Intercrine" cytokine family. Annu Rev Immunol. (1991) 9:617–48. doi: 10.1146/annurev.iy.09.040191.003153
91. Huber, AR , Kunkel, SL , Todd, RF , and Weiss, SJ . Regulation of rransendothelial neutrophil migration by endogenous interleukin-8. Science. (1991) 254:99–102. doi: 10.1126/science.1718038
92. Simonini, A , Moscucci, M , Muller, DWM , Bates, ER , Pagani, FD , Burdick, MD, et al. IL-8 is an Angiogenic factor in human coronary atherectomy tissue. Circulation. (2000) 101:1519–26. doi: 10.1161/01.CIR.101.13.1519
93. Visciano, C , Liotti, F , Prevete, N , Cali, G , Franco, R , Collina, F, et al. Mast cells induce epithelial-to-mesenchymal transition and stem cell features in human thyroid Cancer cells through an IL-8–AKT–slug pathway. Oncogene. (2015) 34:5175–86. doi: 10.1038/onc.2014.441
94. Ou, Z , He, Y , Qi, L , Zu, X , Wu, L , Cao, Z, et al. Infiltrating mast cells enhance benign prostatic hyperplasia through IL-6/STAT3/cyclin D1 signals. Oncotarget. (2017) 8:59156. doi: 10.18632/oncotarget.19465
95. Massey, O , and Suphioglu, C . Recent advances in the inhibition of the IL-4 cytokine pathway for the treatment of allergen-induced asthma. Int J Mol Sci. (2021) 22:13655. doi: 10.3390/ijms222413655
96. Leyva-Castillo, JM , Das, M , Artru, E , Yoon, J , Galand, C , and Geha, RS . Mast cell–derived IL-13 downregulates IL-12 production by skin dendritic cells to inhibit the TH1 cell response to cutaneous antigen exposure. J Allergy Clin Immunol. (2021) 147:2305–2315.e3. doi: 10.1016/j.jaci.2020.11.036
97. Singh, B , Jegga, AG , Shanmukhappa, KS , Edukulla, R , Khurana, GH , Medvedovic, M, et al. IL-31-driven skin remodeling involves epidermal cell proliferation and thickening that Lead to impaired skin-barrier function. PLoS One. (2016) 11:e0161877. doi: 10.1371/journal.pone.0161877
98. Cornelissen, C , Marquardt, Y , Czaja, K , Wenzel, J , Frank, J , Lüscher-Firzlaff, J, et al. IL-31 regulates differentiation and Filaggrin expression in human Organotypic skin models. J Allergy Clin Immunol. (2012) 129:426–433.e8. doi: 10.1016/j.jaci.2011.10.042
99. Deshmane, SL , Kremlev, S , Amini, S , and Sawaya, BE . Monocyte chemoattractant Protein-1 (MCP-1): an overview. J Interf Cytokine Res. (2009) 29:313–26. doi: 10.1089/jir.2008.0027
100. Lin, J , Kakkar, V , and Lu, X . Impact of MCP-1 in atherosclerosis. Curr Pharm Des. (2014) 20:4580–8. doi: 10.2174/1381612820666140522115801
101. Gordon, JR , and Galli, SJ . Mast cells as a source of both preformed and immunologically inducible TNF-α/cachectin. Nature. (1990) 346:274–6. doi: 10.1038/346274a0
102. Sato, N , Goto, T , Haranaka, K , Satomi, N , Nariuchi, H , Mano-Hirano, Y, et al. Actions of tumor necrosis factor on cultured vascular endothelial cells: morphologic modulation, growth inhibition, and cytotoxicity. J Natl Cancer Inst. (1986) 76:1113–21.
103. McCarthy, ET , Sharma, R , Sharma, M , Li, JZ , Ge, XL , Dileepan, KN, et al. TNF-alpha increases albumin permeability of isolated rat glomeruli through the generation of superoxide. J Am Soc Nephrol. (1998) 9:433–8. doi: 10.1681/ASN.V93433
104. Feldmann, M , Brennan, F , Foxwell, B , and Maini, R . The role of TNF-a and IL-1 in rheumatoid arthritis. Curr Dir Autoimmun. (2001) 3:188–99. doi: 10.1159/000060522
105. Harvima, IT , Nilsson, G , Suttle, M-M , and Naukkarinen, A . Is there a role for mast cells in psoriasis? Arch Dermatol Res. (2008) 300:461–78. doi: 10.1007/s00403-008-0874-x
106. Haque, TT , and Frischmeyer-Guerrerio, PA . The role of TGF& Beta; and other cytokines in regulating mast cell functions in allergic inflammation. Int J Mol Sci. (2022) 23:10864. doi: 10.3390/ijms231810864
107. Zhang, W , Wu, K , He, W , Gao, Y , Huang, W , Lin, X, et al. Transforming growth factor Beta 1 plays an important role in inducing CD4+ CD25+ Forhead box P3+ regulatory T cells by mast cells. Clin Exp Immunol. (2010) 161:490–6. doi: 10.1111/j.1365-2249.2010.04190.x
108. Elieh-Ali-Komi, D , and Cao, Y . Role of mast cells in the pathogenesis of multiple sclerosis and experimental autoimmune encephalomyelitis. Clin Rev Allergy Immunol. (2017) 52:436–45. doi: 10.1007/s12016-016-8595-y
109. Zhang, X-C , Strassman, AM , Burstein, R , and Levy, D . Sensitization and activation of intracranial meningeal nociceptors by mast cell mediators. J Pharmacol Exp Ther. (2007) 322:806–12. doi: 10.1124/jpet.107.123745
110. Neisius, U , Olsson, R , Rukwied, R , Lischetzki, G , and Schmelz, M . Prostaglandin E2 induces vasodilation and pruritus, but no protein extravasation in atopic dermatitis and controls. J Am Acad Dermatol. (2002) 47:28–32. doi: 10.1067/mjd.2002.120462
111. van der Pouw Kraan, TC , Boeije, LC , Smeenk, RJ , Wijdenes, J , and Aarden, LA . Prostaglandin-E2 is a potent inhibitor of human interleukin 12 production. J Exp Med. (1995) 181:775–9. doi: 10.1084/jem.181.2.775
112. Hartert, TV , Dworski, RT , Mellen, BG , Oates, JA , Murray, JJ , and Sheller, JR . Prostaglandin E(2) decreases allergen-stimulated release of prostaglandin D(2) in airways of subjects with asthma. Am J Respir Crit Care Med. (2000) 162:637–40. doi: 10.1164/ajrccm.162.2.9904038
113. Fujiwara, S , and Kuriyama, H . Hemolysate-induced contraction in smooth muscle cells of the Guinea pig basilar artery. Stroke. (1984) 15:503–10. doi: 10.1161/01.STR.15.3.503
114. Cho, C , Nguyen, A , Bryant, KJ , O'Neill, SG , and McNeil, HP . Prostaglandin D2 metabolites as a biomarker of in vivo mast cell activation in systemic Mastocytosis and rheumatoid arthritis. Immun Inflamm Dis. (2016) 4:64–9. doi: 10.1002/iid3.94
115. Raud, J . Vasodilatation and inhibition of mediator release represent two distinct mechanisms for prostaglandin modulation of acute mast cell-dependent inflammation. Br J Pharmacol. (1990) 99:449–54. doi: 10.1111/j.1476-5381.1990.tb12948.x
116. Casale, TB . Mast cell mediators and their effect on airway smooth muscle. Inflammatory cells and mediators in bronchial asthma In: DK Agrawal and RG Townley, editors. Inflammatory cells and mediators in bronchial asthma. Boca Raton, FL: CRC Press (1990). 57–76.
117. Moots, R , and Emery, P In: OS Savage and JD Pearson, editors. The role of the endothelium in rheumatoid arthritis and scleroderma. Immunological aspects of the vascular endothelium. Cambridge: Cambridge University Press (1995). 153–72.
118. Shepherd, RK , and Duling, BR . Inosine-induced vasoconstriction is mediated by histamine and thromboxane derived from mast cells. Am J Phys. (1996) 270:H560–6. doi: 10.1152/ajpheart.1996.270.2.H560
119. Chong, KW , Ruiz-Garcia, M , Patel, N , Boyle, RJ , and Turner, PJ . Reaction phenotypes in IgE-mediated food allergy and anaphylaxis. Ann Allergy Asthma Immunol. (2020) 124:473–8. doi: 10.1016/j.anai.2019.12.023
120. Forman, MB , Oates, JA , Robertson, D , Robertson, RM , Roberts, LJ 2nd, and Virmani, R . Increased adventitial mast cells in a patient with coronary spasm. N Engl J Med. (1985) 313:1138–41. doi: 10.1056/NEJM198510313131807
121. Iwamoto, I , Ra, C , Sato, T , Tomioka, H , and Yoshida, S . Thromboxane A2 production in allergen-induced immediate and late asthmatic responses: its possible role in inducing the late response. J Asthma. (1988) 25:117–24. doi: 10.3109/02770908809073198
122. Sladek, K , and Szczeklik, A . Cysteinyl leukotrienes overproduction and mast cell activation in aspirin-provoked bronchospasm in asthma. Eur Respir J. (1993) 6:391–9. doi: 10.1183/09031936.93.06030391
123. Hart, PH . Regulation of the inflammatory response in asthma by mast cell products. Immunol Cell Biol. (2001) 79:149–53. doi: 10.1046/j.1440-1711.2001.00983.x
124. de Prost, N , El-Karak, C , Avila, M , Ichinose, F , and Melo, MFV . Changes in Cysteinyl leukotrienes during and after cardiac surgery with cardiopulmonary bypass in patients with and without chronic obstructive pulmonary disease. J Thorac Cardiovasc Surg. (2011) 141:1496–502. e3. doi: 10.1016/j.jtcvs.2011.01.035
125. Sharma, JN , and Mohammed, LA . The role of leukotrienes in the pathophysiology of inflammatory disorders: is there a case for revisiting leukotrienes as therapeutic targets? Inflammopharmacology. (2006) 14:10–6. doi: 10.1007/s10787-006-1496-6
126. Ribeiro, RA , Souza-Filho, MVP , Souza, MHLP , Oliveira, SHP , Costa, CHS , Cunha, FQ, et al. Role of resident mast cells and macrophages in the neutrophil migration induced by LTB4, fMLP and C5a des arg. Int Arch Allergy Immunol. (2009) 112:27–35. doi: 10.1159/000237427
127. Oliveira, SHP , Costa, CHS , Ferreira, SH , and Cunha, FQ . Sephadex induces eosinophil migration to the rat and mouse peritoneal cavity: involvement of mast cells, LTB4, TNF-α, IL-8 and PAF. Inflamm Res. (2002) 51:144–53. doi: 10.1007/PL00000286
128. Honda, T , and Kabashima, K . Prostanoids and leukotrienes in the pathophysiology of atopic dermatitis and psoriasis. Int Immunol. (2019) 31:589–95. doi: 10.1093/intimm/dxy087
129. Min, HK , Kim, K-W , Lee, S-H , and Kim, H-R . Roles of mast cells in rheumatoid arthritis. Korean J Intern Med. (2020) 35:12. doi: 10.3904/kjim.2019.271
130. Nielsen, OH , and Rask-Madsen, J . Mediators of inflammation in chronic inflammatory bowel disease. Scand J Gastroenterol. (1996) 31:149–59. doi: 10.3109/00365529609094569
131. Poczobutt, JM , Nguyen, TT , Hanson, D , Li, H , Sippel, TR , Weiser-Evans, M, et al. Deletion of 5-lipoxygenase in the tumor microenvironment promotes lung Cancer progression and metastasis through regulating T cell recruitment. J Immunol. (2016) 196:891–901. doi: 10.4049/jimmunol.1501648
132. Powell, WS , Chung, D , and Gravel, S . 5-Oxo-6, 8, 11, 14-eicosatetraenoic acid is a potent stimulator of human eosinophil migration. J Immunol. (1995) 154:4123–32. doi: 10.4049/jimmunol.154.8.4123
133. Sanak, M , Gielicz, A , Bochenek, G , Kaszuba, M , Niżankowska-Mogilnicka, E , and Szczeklik, A . Targeted eicosanoid Lipidomics of exhaled breath condensate provide a distinct pattern in the aspirin-intolerant asthma phenotype. J Allergy Clin Immunol. (2011) 127:1141–1147. e2. doi: 10.1016/j.jaci.2010.12.1108
134. Park, S , Bivona, BJ , Ford, SM Jr, Xu, S , Kobori, H , de Garavilla, L, et al. Direct evidence for intrarenal chymase-dependent angiotensin ii formation on the diabetic renal microvasculature. Hypertension. (2013) 61:465–71. doi: 10.1161/HYPERTENSIONAHA.111.202424
135. Vartio, T , Seppä, H , and Vaheri, A . Susceptibility of soluble and matrix fibronectins to degradation by tissue proteinases, mast cell chymase and Cathepsin G. J Biol Chem. (1981) 256:471–7. doi: 10.1016/S0021-9258(19)70161-6
136. Balcells, E , Meng, QC , Johnson, WH Jr, Oparil, S , and Dell’Italia, LJ . Angiotensin II formation from ACE and chymase in human and animal hearts: methods and species considerations. Am J Phys Heart Circ Phys. (1997) 273:H1769–74. doi: 10.1152/ajpheart.1997.273.4.H1769
137. Roszkowska-Chojecka, MM , Walkowska, A , Gawryś, O , Baranowska, I , Kalisz, M , Litwiniuk, A, et al. Effects of chymostatin, a chymase inhibitor, on blood pressure, plasma and tissue angiotensin II, renal haemodynamics and renal excretion in two models of hypertension in the rat. Exp Physiol. (2015) 100:1093–105. doi: 10.1113/EP085325
138. Lindstedt, L , Lee, M , and Kovanen, PT . Chymase bound to heparin is resistant to its natural inhibitors and capable of Proteolyzing high density lipoproteins in aortic intimal fluid. Atherosclerosis. (2001) 155:87–97. doi: 10.1016/S0021-9150(00)00544-X
139. Ramalho, LS , Oliveira, LF , Cavellani, CL , da Fonseca Ferraz, ML , de Oliveira, FA , Corrêa, RRM, et al. Role of mast cell chymase and tryptase in the progression of atherosclerosis: study in 44 autopsied cases. Ann Diagn Pathol. (2013) 17:28–31. doi: 10.1016/j.anndiagpath.2012.04.007
140. Hamada, H , Terai, M , Kimura, H , Hirano, K , Oana, S , and Niimi, H . Increased expression of mast cell chymase in the lungs of patients with congenital heart disease associated with early pulmonary vascular disease. Am J Respir Crit Care Med. (1999) 160:1303–8. doi: 10.1164/ajrccm.160.4.9810058
141. Tomimori, Y , Muto, T , Saito, K , Tanaka, T , Maruoka, H , Sumida, M, et al. Involvement of mast cell chymase in bleomycin-induced pulmonary fibrosis in mice. Eur J Pharmacol. (2003) 478:179–85. doi: 10.1016/j.ejphar.2003.08.050
142. Liu, G , Jarnicki, AG , Paudel, KR , Lu, W , Wadhwa, R , Philp, AM, et al. Adverse roles of mast cell Chymase-1 in COPD. Eur Respir J. (2022) 60:2021. doi: 10.1183/13993003.01431-2021
143. Jiang, Y , Wu, Y , Hardie, WJ , and Zhou, X . Mast cell chymase affects the proliferation and metastasis of lung carcinoma cells in vitro. Oncol Lett. (2017) 14:3193–8. doi: 10.3892/ol.2017.6487
144. Brightling, CE , Bradding, P , Symon, FA , Holgate, ST , Wardlaw, AJ , and Pavord, ID . Mast-cell infiltration of airway smooth muscle in asthma. N Engl J Med. (2002) 346:1699–705. doi: 10.1056/NEJMoa012705
145. Wang, Y , Shiota, N , Leskinen, MJ , Lindstedt, KA , and Kovanen, PT . Mast cell chymase inhibits smooth muscle cell growth and collagen expression in vitro. Arterioscler Thromb Vasc Biol. (2001) 21:1928–33. doi: 10.1161/hq1201.100227
146. Liu, W-X , Wang, Y , Sang, L-X , Zhang, S , Wang, T , Zhou, F, et al. Chymase inhibitor TY-51469 in therapy of inflammatory bowel disease. World J Gastroenterol. (2016) 22:1826–33. doi: 10.3748/wjg.v22.i5.1826
147. Lind, T , Gustafson, A-M , Calounova, G , Hu, L , Rasmusson, A , Jonsson, KB, et al. Increased bone mass in female mice lacking mast cell chymase. PLoS One. (2016) 11:e0167964. doi: 10.1371/journal.pone.0167964
148. Hagermark, O , Rajka, G , and Bergvist, U . Experimental itch in human skin elicited by rat mast cell chymase. Acta Derm Venereol. (1972) 52:125–8. doi: 10.2340/0001555552125128
149. Castells, M . Mast cell mediators in allergic inflammation and mastocytosis. Immunol Aller Clin. (2006) 26:465–85. doi: 10.1016/j.iac.2006.05.005
150. Sala-Cunill, A , Guilarte, M , and Cardona, V . Phenotypes, endotypes and biomarkers in anaphylaxis: current insights. Curr Opin Allergy Clin Immunol. (2018) 18:370–6. doi: 10.1097/ACI.0000000000000472
151. Ribatti, D . Mast cells and macrophages exert beneficial and detrimental effects on tumor progression and angiogenesis. Immunol Lett. (2013) 152:83–8. doi: 10.1016/j.imlet.2013.05.003
152. Johnson, P , Ammit, A , Carlin, S , Armour, C , Caughey, G , and Black, J . Mast cell tryptase potentiates histamine-induced contraction in human sensitized bronchus. Eur Respir J. (1997) 10:38–43. doi: 10.1183/09031936.97.10010038
153. Berger, P , Perng, D-W , Thabrew, H , Compton, SJ , Cairns, JA , McEuen, AR, et al. Tryptase and agonists of PAR-2 induce the proliferation of human airway smooth muscle cells. J Appl Physiol. (2001) 91:1372–9. doi: 10.1152/jappl.2001.91.3.1372
154. Woodman, L , Siddiqui, S , Cruse, G , Sutcliffe, A , Saunders, R , Kaur, D, et al. Mast cells promote airway smooth muscle cell differentiation via autocrine up-regulation of TGF-beta 1. J Immunol. (2008) 181:5001–7. doi: 10.4049/jimmunol.181.7.5001
155. Brockow, K , Akin, C , Huber, M , Scott, LM , Schwartz, LB , and Metcalfe, DD . Levels of mast-cell growth factors in plasma and in suction skin blister fluid in adults with Mastocytosis: correlation with dermal mast-cell numbers and mast-cell tryptase. J Allergy Clin Immunol. (2002) 109:82–8. doi: 10.1067/mai.2002.120524
156. Vergnolle, N . Proteinase-activated receptors-novel signals for gastrointestinal pathophysiology. Aliment Pharmacol Ther. (2000) 14:257–66. doi: 10.1046/j.1365-2036.2000.00690.x
157. Crowe, SE , and Perdue, MH . Gastrointestinal food hypersensitivity: basic mechanisms of pathophysiology. Gastroenterology. (1992) 103:1075–95. doi: 10.1016/0016-5085(92)90047-3
158. Yosipovitch, G . Dry skin and impairment of barrier function associated with itch–new insights. Int J Cosmet Sci. (2004) 26:1–7. doi: 10.1111/j.0142-5463.2004.00199.x
159. Järvikallio, A , Naukkarinen, A , Harvima, I , Aalto, ML , and Horsmanheimo, M . Quantitative analysis of tryptase-and chymase-containing mast cells in atopic dermatitis and nummular eczema. Br J Dermatol. (1997) 136:871–7. doi: 10.1111/j.1365-2133.1997.tb03927.x
160. Ferrer, M , Nuñez-Córdoba, J , Luquin, E , Grattan, C , De la Borbolla, J , Sanz, M, et al. Serum total tryptase levels are increased in patients with active chronic Urticaria. Clin Exp Allergy. (2010) 40:1760–6. doi: 10.1111/j.1365-2222.2010.03582.x
161. Barbara, G , Stanghellini, V , De Giorgio, R , Cremon, C , Cottrell, GS , Santini, D, et al. Activated mast cells in proximity to colonic nerves correlate with abdominal pain in irritable bowel syndrome. Gastroenterology. (2004) 126:693–702. doi: 10.1053/j.gastro.2003.11.055
162. Torresani, C , Bellafiore, S , and De Panfilis, G . Chronic urticaria is usually associated with fibromyalgia syndrome. Acta Derm Venereol. (2009) 89:389–92. doi: 10.2340/00015555-0653
163. Pejler, G . The emerging role of mast cell proteases in asthma. Eur Respir J. (2019) 54:2019. doi: 10.1183/13993003.00685-2019
164. Schneider, LA , Schlenner, SM , Feyerabend, TB , Wunderlin, M , and Rodewald, H-R . Molecular mechanism of mast cell–mediated innate defense against endothelin and Snake venom Sarafotoxin. J Exp Med. (2007) 204:2629–39. doi: 10.1084/jem.20071262
165. Hook, M , Bjork, I , Hopwood, J , and Lindahl, U . Anticoagulant activity of heparin: separation of high-activity and low-activity heparin species by affinity chromatography on immobilized antithrombin. FEBS Lett. (1976) 66:90–3. doi: 10.1016/0014-5793(76)80592-3
166. Handoll, HH , Farrar, MJ , McBirnie, J , Tytherleigh-Strong, GM , Milne, AA , and Gillespie, WJ . Heparin, low molecular weight heparin and physical methods for preventing deep vein thrombosis and pulmonary embolism following surgery for hip fractures. Cochrane Database Syst Rev. (1996) 2021:305. doi: 10.1002/14651858.CD000305
167. Downing, LJ , Strieter, RM , Kadell, AM , Wilke, CA , Greenfield, LJ , and Wakefield, TW . Low-dose low–molecular-weight heparin is anti-inflammatory during venous thrombosis. J Vasc Surg. (1998) 28:848–54. doi: 10.1016/S0741-5214(98)70060-6
168. Carr, J . The anti-inflammatory action of heparin: heparin as an antagonist to histamine, bradykinin and prostaglandin E1. Thromb Res. (1979) 16:507–16. doi: 10.1016/0049-3848(79)90097-5
169. Brunnee, T , Reddigari, S , Shibayama, Y , Kaplan, A , and Silverberg, M . Mast cell derived heparin activates the contact system: a link to Kinin generation in allergic reactions. Clin Exp Allergy. (1997) 27:653–63. doi: 10.1159/000024284
170. Li, X , and Ma, X . The role of heparin in Sepsis: much more than just an anticoagulant. Br J Haematol. (2017) 179:389–98. doi: 10.1111/bjh.14885
171. Klinkhammer, B , and Gruchalla, M . Is there an association between heparin-induced thrombocytopenia (hit) and autoimmune disease? Wmj. (2018) 117:13–7.
172. Echtenacher, B , Mannel, DN , and Hultner, L . Critical protective role of mast cells in a model of acute septic peritonitis. Nature. (1996) 381:75–7. doi: 10.1038/381075a0
173. Wedemeyer, J , Tsai, M , and Galli, SJ . Roles of mast cells and basophils in innate and acquired immunity. Curr Opin Immunol. (2000) 12:624–31. doi: 10.1016/S0952-7915(00)00154-0
174. Gurish, MF , and Austen, KF . The diverse roles of mast cells. J Exp Med. (2001) 194:F1–5. doi: 10.1084/jem.194.1.F1
175. Schwartz, LB , Irani, AM , Roller, K , Castells, MC , and Schechter, NM . Quantitation of histamine, Tryptase, and Chymase in dispersed human T and TC mast cells. J Immunol. (1987) 138:2611–5. doi: 10.4049/jimmunol.138.8.2611
176. Reynolds, DS , Gurley, DS , Stevens, RL , Sugarbaker, DJ , Austen, KF , and Serafin, WE . Cloning of cDNAs that encode human mast cell carboxypeptidase a, and comparison of the protein with mouse mast cell carboxypeptidase A and rat pancreatic carboxypeptidases. Proc Natl Acad Sci U S A. (1989) 86:9480–4. doi: 10.1073/pnas.86.23.9480
177. Vanderslice, P , Ballinger, SM , Tam, EK , Goldstein, SM , Craik, CS , and Caughey, GH . Human mast cell Tryptase: multiple cDNAs and genes reveal a multigene serine protease family. Proc Natl Acad Sci U S A. (1990) 87:3811–5. doi: 10.1073/pnas.87.10.3811
178. Galli, SJ . New concepts about the mast cell. N Engl J Med. (1993) 328:257–65. doi: 10.1056/NEJM199301283280408
179. Gilfillan, AM , and Beaven, MA . Regulation of mast cell responses in health and disease. Crit Rev Immunol. (2011) 31:475–529. doi: 10.1615/CritRevImmunol.v31.i6.30
180. Bannert, C , Bidmon-Fliegenschnee, B , Stary, G , Hotzy, F , Stift, J , Nurko, S, et al. Fc-epsilon-RI, the high affinity IgE-receptor, is robustly expressed in the upper gastrointestinal tract and modulated by mucosal inflammation. PLoS One. (2012) 7:e42066. doi: 10.1371/journal.pone.0042066
181. Liu, FT , Hsu, DK , Zuberi, RI , Kuwabara, I , Chi, EY , and Henderson, WR Jr. Expression and function of galectin-3, a beta-galactoside-binding lectin, in human monocytes and macrophages. Am J Pathol. (1995) 147:1016–28.
182. Ezeamuzie, CI , Al-Attiyah, R , Shihab, PK , and Al-Radwan, R . Low-affinity IgE receptor (FcepsilonRII)-mediated activation of human monocytes by both monomeric IgE and IgE/anti-IgE immune complex. Int Immunopharmacol. (2009) 9:1110–4. doi: 10.1016/j.intimp.2009.05.009
183. McNeil, BD . MRGPRX2 and adverse drug reactions. Front Immunol. (2021) 12:676354. doi: 10.3389/fimmu.2021.676354
184. Theoharides, TC , Bondy, PK , Tsakalos, ND , and Askenase, PW . Differential release of serotonin and histamine from mast cells. Nature. (1982) 297:229–31. doi: 10.1038/297229a0
185. Kraeuter Kops, S , Theoharides, TC , Cronin, CT , Kashgarian, MG , and Askenase, PW . Ultrastructural characteristics of rat peritoneal mast cells undergoing differential release of serotonin without histamine and without degranulation. Cell Tissue Res. (1990) 262:415–24. doi: 10.1007/BF00305238
186. Barnes, PJ . Histamine receptors in the lung. Agents Actions Suppl. (1991) 33:103–22. doi: 10.1007/978-3-0348-7309-3_9
187. Hill, SJ . Multiple histamine receptors: properties and functional characteristics. Biochem Soc Trans. (1992) 20:122–5. doi: 10.1042/bst0200122
188. Dileepan, KN , Lorsbach, RB , and Stechschulte, DJ . Mast cell granules inhibit macrophage-mediated lysis of Mastocytoma cells (P815) and nitric oxide production. J Leukoc Biol. (1993) 53:446–53. doi: 10.1002/jlb.53.4.446
189. Elenkov, IJ , Webster, E , Papanicolaou, DA , Fleisher, TA , Chrousos, GP , and Wilder, RL . Histamine potently suppresses human IL-12 and stimulates IL-10 production via H2 receptors. J Immunol. (1998) 161:2586–93. doi: 10.4049/jimmunol.161.5.2586
190. Haas, HL , and Panula, P . Histamine Receptors. Neuropharmacology. (2016) 106:1–2. doi: 10.1016/j.neuropharm.2016.04.007
191. Walsh, GM , Annunziato, L , Frossard, N , Knol, K , Levander, SE , Nicolas, J-M, et al. New insights into the second generation antihistamines. Drugs. (2012) 61:207–36. doi: 10.2165/00003495-200161020-00006
192. Thangam, EB , Jemima, EA , Singh, H , Baig, MS , Khan, M , Mathias, CB, et al. The role of histamine and histamine receptors in mast cell-mediated allergy and inflammation: the Hunt for new therapeutic targets. Front Immunol. (2018) 9:1873. doi: 10.3389/fimmu.2018.01873
193. Akdis, CA , and Simons, FE . Histamine receptors are hot in Immunopharmacology. Eur J Pharmacol. (2006) 533:69–76. doi: 10.1016/j.ejphar.2005.12.044
194. Stechschulte, DJ Jr, Morris, DC , Jilka, RL , Stechschulte, DJ , and Dileepan, KN . Impaired gastric acid secretion in mast cell-deficient mice. Am J Phys. (1990) 259:G41–7. doi: 10.1152/ajpgi.1990.259.1.G41
195. Kohka, H , Nishibori, M , Iwagaki, H , Nakaya, N , Yoshino, T , Kobashi, K, et al. Histamine is a potent inducer of IL-18 and IFN-gamma in human peripheral blood mononuclear cells. J Immunol. (2000) 164:6640–6. doi: 10.4049/jimmunol.164.12.6640
196. Caron, G , Delneste, Y , Roelandts, E , Duez, C , Bonnefoy, JY , Pestel, J, et al. Histamine polarizes human dendritic cells into Th2 cell-promoting effector dendritic cells. J Immunol. (2001) 167:3682–6. doi: 10.4049/jimmunol.167.7.3682
197. Jutel, M , Watanabe, T , Akdis, M , Blaser, K , and Akdis, CA . Immune regulation by histamine. Curr Opin Immunol. (2002) 14:735–40. doi: 10.1016/S0952-7915(02)00395-3
198. Leurs, R , Blandina, P , Tedford, C , and Timmerman, H . Therapeutic potential of histamine H3 receptor agonists and antagonists. Trends Pharmacol Sci. (1998) 19:177–83. doi: 10.1016/S0165-6147(98)01201-2
199. Nakamura, T , Itadani, H , Hidaka, Y , Ohta, M , and Tanaka, K . Molecular cloning and characterization of a new human histamine receptor, HH4R. Biochem Biophys Res Commun. (2000) 279:615–20. doi: 10.1006/bbrc.2000.4008
200. Oda, T , Morikawa, N , Saito, Y , Masuho, Y , and Matsumoto, S . Molecular cloning and characterization of a novel type of histamine receptor preferentially expressed in leukocytes. J Biol Chem. (2000) 275:36781–6. doi: 10.1074/jbc.M006480200
201. Gantner, F , Sakai, K , Tusche, MW , Cruikshank, WW , Center, DM , and Bacon, KB . Histamine h(4) and h(2) receptors control histamine-induced Interleukin-16 release from human CD8(+) T cells. J Pharmacol Exp Ther. (2002) 303:300–7. doi: 10.1124/jpet.102.036939
202. Schneider, E , Rolli-Derkinderen, M , Arock, M , and Dy, M . Trends in histamine research: new functions during immune responses and hematopoiesis. Trends Immunol. (2002) 23:255–63. doi: 10.1016/S1471-4906(02)02215-9
203. Bottaro, D , Shepro, D , Peterson, S , and Hechtman, HB . Serotonin, histamine, and norepinephrine mediation of endothelial and vascular smooth muscle cell movement. Am J Phys. (1985) 248:C252–7. doi: 10.1152/ajpcell.1985.248.3.C252
204. Bell, L , and Madri, JA . Effect of platelet factors on migration of cultured bovine aortic endothelial and smooth muscle cells. Circ Res. (1989) 65:1057–65. doi: 10.1161/01.RES.65.4.1057
205. Miyazawa, N , Watanabe, S , Matsuda, A , Kondo, K , Hashimoto, H , Umemura, K, et al. Role of histamine H1 and H2 receptor antagonists in the prevention of intimal thickening. Eur J Pharmacol. (1998) 362:53–9. doi: 10.1016/S0014-2999(98)00716-X
206. Sakata, Y , Komamura, K , Hirayama, A , Nanto, S , Kitakaze, M , Hori, M, et al. Elevation of the plasma histamine concentration in the coronary circulation in patients with variant angina. Am J Cardiol. (1996) 77:1121–6. doi: 10.1016/S0002-9149(96)00147-6
207. Marone, G , Genovese, A , Varricchi, G , and Granata, F . Human heart as a shock organ in anaphylaxis. Allergo J Int. (2014) 23:60–6. doi: 10.1007/s40629-014-0007-3
208. Panula, P , Chazot, PL , Cowart, M , Gutzmer, R , Leurs, R , Liu, WL, et al. International Union of Basic and Clinical Pharmacology. XCVIII Histamine Receptors. Pharmacol Rev. (2015) 67:601–55. doi: 10.1124/pr.114.010249
209. Neumann, J , Kirchhefer, U , Dhein, S , Hofmann, B , and Gergs, U . The roles of cardiovascular H2-histamine receptors under Normal and pathophysiological conditions. Front Pharmacol. (2021):12. doi: 10.3389/fphar.2021.732842
210. Marino, A , and Levi, R . Salvaging the ischemic heart: Gi-coupled receptors in mast cells activate a PKCepsilon/ALDH2 pathway providing Anti-RAS cardioprotection. Curr Med Chem. (2018) 25:4416–31. doi: 10.2174/0929867325666180214115127
211. Pejler, G , Rönnberg, E , Waern, I , and Wernersson, S . Mast cell proteases: multifaceted regulators of inflammatory disease. Blood. (2010) 115:4981–90. doi: 10.1182/blood-2010-01-257287
212. Weidner, N , and Austen, KF . Heterogeneity of mast cells at multiple body sites. Fluorescent determination of Avidin binding and immunofluorescent determination of Chymase, Tryptase, and carboxypeptidase content. Pathol Res Pract. (1993) 189:156–62. doi: 10.1016/S0344-0338(11)80086-5
213. Irani, AA , Schechter, NM , Craig, SS , DeBlois, G , and Schwartz, LB . Two types of human mast cells that have distinct neutral protease compositions. Proc Natl Acad Sci U S A. (1986) 83:4464–8. doi: 10.1073/pnas.83.12.4464
214. Harvima, IT , Karkola, K , Harvima, RJ , Naukkarinen, A , Neittaanmäki, H , Horsmanheimo, M, et al. Biochemical and histochemical evaluation of Tryptase in various human tissues. Arch Dermatol Res. (1989) 281:231–7. doi: 10.1007/BF00431056
215. Harvima, IT , Naukkarinen, A , Harvima, RJ , and Fräki, JE . Immunoperoxidase and enzyme-histochemical demonstration of human skin tryptase in cutaneous mast cells in normal and mastocytoma skin. Arch Dermatol Res. (1988) 280:363–70. doi: 10.1007/BF00426615
216. Haas, N , Hamann, K , Grabbe, J , Algermissen, B , and Czarnetzki, BM . Phenotypic characterization of skin lesions in urticaria pigmentosa and mastocytomas. Arch Dermatol Res. (1995) 287:242–8. doi: 10.1007/BF01105073
217. Kaartinen, M , Penttila, A , and Kovanen, PT . Mast cells accompany microvessels in human coronary atheromas: implications for intimal neovascularization and hemorrhage. Atherosclerosis. (1996) 123:123–31. doi: 10.1016/0021-9150(95)05794-3
218. Leskinen, MJ , Lindstedt, KA , Wang, Y , and Kovanen, PT . Mast cell chymase induces smooth muscle cell apoptosis by a mechanism involving fibronectin degradation and disruption of focal adhesions. Arterioscler Thromb Vasc Biol. (2003) 23:238–43. doi: 10.1161/01.ATV.0000051405.68811.4D
219. Mayranpaa, MI , Heikkila, HM , Lindstedt, KA , Walls, AF , and Kovanen, PT . Desquamation of human coronary artery endothelium by human mast cell proteases: implications for plaque Erosion. Coron Artery Dis. (2006) 17:611–21. doi: 10.1097/01.mca.0000224420.67304.4d
220. Heikkila, HM , Latti, S , Leskinen, MJ , Hakala, JK , Kovanen, PT , and Lindstedt, KA . Activated mast cells induce endothelial cell apoptosis by a combined action of Chymase and tumor necrosis factor-alpha. Arterioscler Thromb Vasc Biol. (2008) 28:309–14. doi: 10.1161/ATVBAHA.107.151340
221. Bot, I , de Jager, SC , Zernecke, A , Lindstedt, KA , van Berkel, TJ , Weber, C, et al. Perivascular mast cells promote Atherogenesis and induce plaque destabilization in apolipoprotein E-deficient mice. Circulation. (2007) 115:2516–25. doi: 10.1161/CIRCULATIONAHA.106.660472
222. Bot, I . The mast cell: a novel actor in cardiac microvessel dysfunction. Arterioscler Thromb Vasc Biol. (2021) 41:1337–8. doi: 10.1161/ATVBAHA.121.316043
223. Caughey, GH , Raymond, WW , Blount, JL , Hau, LW , Pallaoro, M , Wolters, PJ, et al. Characterization of human gamma-tryptases, novel members of the chromosome 16p mast cell tryptase and prostasin gene families. J Immunol. (2000) 164:6566–75. doi: 10.4049/jimmunol.164.12.6566
224. Cairns, JA , and Walls, AF . Mast cell tryptase is a mitogen for epithelial cells. Stimulation of IL-8 production and intercellular adhesion Molecule-1 expression. J Immunol. (1996) 156:275–83. doi: 10.4049/jimmunol.156.1.275
225. Compton, SJ , Cairns, JA , Holgate, ST , and Walls, AF . The role of mast cell Tryptase in regulating endothelial cell proliferation, cytokine release, and adhesion molecule expression: Tryptase induces expression of mRNA for IL-1 Beta and IL-8 and stimulates the selective release of IL-8 from human umbilical vein endothelial cells. J Immunol. (1998) 161:1939–46. doi: 10.4049/jimmunol.161.4.1939
226. Gruber, BL , Kew, RR , Jelaska, A , Marchese, MJ , Garlick, J , Ren, S, et al. Human mast cells activate fibroblasts: tryptase is a fibrogenic factor stimulating collagen messenger ribonucleic acid synthesis and fibroblast chemotaxis. J Immunol. (1997) 158:2310–7. doi: 10.4049/jimmunol.158.5.2310
227. Blair, RJ , Meng, H , Marchese, MJ , Ren, S , Schwartz, LB , Tonnesen, MG, et al. Human mast cells stimulate vascular tube formation. Tryptase is a novel, potent Angiogenic factor. J Clin Invest. (1997) 99:2691–700. doi: 10.1172/JCI119458
228. Sabri, A , Muske, G , Zhang, H , Pak, E , Darrow, A , Andrade-Gordon, P, et al. Signaling properties and functions of two distinct cardiomyocyte protease-activated receptors. Circ Res. (2000) 86:1054–61. doi: 10.1161/01.RES.86.10.1054
229. Frungieri, MB , Weidinger, S , Meineke, V , Köhn, FM , and Mayerhofer, A . Proliferative action of mast-cell Tryptase is mediated by PAR2, COX2, prostaglandins, and PPARgamma: possible relevance to human fibrotic disorders. Proc Natl Acad Sci U S A. (2002) 99:15072–7. doi: 10.1073/pnas.232422999
230. de Almeida, AD , Silva, IS , Fernandes-Braga, W , Lima Filho, ACM , Florentino, ROM , Barra, A, et al. A role for mast cells and mast cell Tryptase in driving neutrophil recruitment in LPS-induced lung inflammation via protease-activated receptor 2 in mice. Inflamm Res. (2020) 69:1059–70. doi: 10.1007/s00011-020-01376-4
231. Jeziorska, M , McCollum, C , and Woolley, DE . Mast cell distribution, activation, and phenotype in atherosclerotic lesions of human carotid arteries. J Pathol. (1997) 182:115–22. doi: 10.1002/(SICI)1096-9896(199705)182:1<115::AID-PATH806>3.0.CO;2-9
232. Patella, V , Marino, I , Lamparter, B , Arbustini, E , Adt, M , and Marone, G . Human heart mast cells. Isolation, purification, ultrastructure, and immunologic characterization. J Immunol. (1995) 154:2855–65. doi: 10.4049/jimmunol.154.6.2855
233. Urata, H , Kinoshita, A , Misono, KS , Bumpus, FM , and Husain, A . Identification of a highly specific chymase as the major angiotensin ii-forming enzyme in the human heart. J Biol Chem. (1990) 265:22348–57. doi: 10.1016/S0021-9258(18)45712-2
234. Hoit, BD , Shao, Y , Kinoshita, A , Gabel, M , Husain, A , and Walsh, RA . Effects of angiotensin ii generated by an angiotensin converting enzyme-independent pathway on left ventricular performance in the conscious baboon. J Clin Invest. (1995) 95:1519–27. doi: 10.1172/JCI117824
235. Kleij, HP , and Bienenstock, J . Significance of conversation between mast cells and nerves. Allergy Asthma Clin Immunol. (2005) 1:65–80. doi: 10.1186/1710-1492-1-2-65
236. Kokkonen, JO , Vartiainen, M , and Kovanen, PT . Low density lipoprotein degradation by secretory granules of rat mast cells. Sequential degradation of apolipoprotein B by granule Chymase and carboxypeptidase A. J Biol Chem. (1986) 261:16067–72. doi: 10.1016/S0021-9258(18)66677-3
237. Lindstedt, KA . Inhibition of macrophage-mediated low density lipoprotein oxidation by stimulated rat serosal mast cells. J Biol Chem. (1993) 268:7741–6. doi: 10.1016/S0021-9258(18)53019-2
238. Huang, C , Friend, DS , Qiu, WT , Wong, GW , Morales, G , Hunt, J, et al. Induction of a selective and persistent extravasation of neutrophils into the peritoneal cavity by tryptase mouse mast cell protease 6. J Immunol. (1998) 160:1910–9. doi: 10.4049/jimmunol.160.4.1910
239. Li, Y , Stechschulte, AC , Smith, DD , Lindsley, HB , Stechschulte, DJ , and Dileepan, KN . Mast cell granules potentiate endotoxin-induced interleukin-6 production by endothelial cells. J Leukoc Biol. (1997) 62:211–6.
240. Jehle, AB , Li, Y , Stechschulte, AC , Stechschulte, DJ , and Dileepan, KN . Endotoxin and mast cell granule proteases synergistically activate human coronary artery endothelial cells to generate interleukin-6 and Interleukin-8. J Interf Cytokine Res. (2000) 20:361–8. doi: 10.1089/107999000312298
241. Wezel, A , Lagraauw, HM , van der Velden, D , de Jager, SC , Quax, PH , Kuiper, J, et al. Mast cells mediate neutrophil recruitment during atherosclerotic plaque progression. Atherosclerosis. (2015) 241:289–96. doi: 10.1016/j.atherosclerosis.2015.05.028
242. Zhang, J , Alcaide, P , Liu, L , Sun, J , He, A , Luscinskas, FW, et al. Regulation of endothelial cell adhesion molecule expression by mast cells, macrophages, and neutrophils. PLoS One. (2011) 6:e14525. doi: 10.1371/journal.pone.0014525
243. Forsberg, E , Pejler, G , Ringvall, M , Lunderius, C , Tomasini-Johansson, B , Kusche-Gullberg, M, et al. Abnormal mast cells in mice deficient in a heparin-synthesizing enzyme. Nature. (1999) 400:773–6. doi: 10.1038/23488
244. Humphries, DE , Wong, GW , Friend, DS , Gurish, MF , Qiu, W-T , Huang, C, et al. Heparin is essential for the storage of specific granule proteases in mast cells. Nature. (1999) 400:769–72. doi: 10.1038/23481
245. Henningsson, F , Ledin, J , Lunderius, C , Wilen, M , Hellman, L , and Pejler, G . Altered storage of proteases in mast cells from mice lacking heparin: a possible role for heparin in carboxypeptidase a processing. Biol Chem. (2002) 383:793–801. doi: 10.1515/BC.2002.083
246. Oschatz, C , Maas, C , Lecher, B , Jansen, T , Bjorkqvist, J , Tradler, T, et al. Mast cells increase vascular permeability by heparin-initiated bradykinin formation in vivo. Immunity. (2011) 34:258–68. doi: 10.1016/j.immuni.2011.02.008
247. Kaplan, AP , and Joseph, K . Pathogenic mechanisms of bradykinin mediated diseases: dysregulation of an innate inflammatory pathway. Adv Immunol. (2014) 121:41–89. doi: 10.1016/B978-0-12-800100-4.00002-7
248. Azizkhan, RG , Azizkhan, JC , Zetter, BR , and Folkman, J . Mast cell heparin stimulates migration of capillary endothelial cells in vitro. J Exp Med. (1980) 152:931–44. doi: 10.1084/jem.152.4.931
249. Folkman, J . Regulation of angiogenesis: a new function of heparin. Biochem Pharmacol. (1985) 34:905–9. doi: 10.1016/0006-2952(85)90588-X
250. Lagunoff, D , and Rickard, A . Mast cell granule heparin proteoglycan induces lacunae in confluent endothelial cell monolayers. Am J Pathol. (1999) 154:1591–600. doi: 10.1016/S0002-9440(10)65412-0
251. Jones, H , Paul, W , and Page, CP . The effects of heparin and related molecules on vascular permeability and neutrophil accumulation in rabbit skin. Br J Pharmacol. (2002) 135:469–79. doi: 10.1038/sj.bjp.0704505
252. Crijns, H , Vanheule, V , and Proost, P . Targeting chemokine-glycosaminoglycan interactions to inhibit inflammation. Front Immunol. (2020) 11:483. doi: 10.3389/fimmu.2020.00483
253. Bacci, S , Romagnoli, P , Norelli, GA , Forestieri, AL , and Bonelli, A . Early increase in TNF-alpha-containing mast cells in skin lesions. Int J Legal Med. (2006) 120:138–42. doi: 10.1007/s00414-005-0030-5
254. De Filippo, K , Dudeck, A , Hasenberg, M , Nye, E , van Rooijen, N , Hartmann, K, et al. Mast cell and macrophage chemokines CXCL1/CXCL2 control the early stage of neutrophil recruitment during tissue inflammation. Blood. (2013) 121:4930–7. doi: 10.1182/blood-2013-02-486217
255. Mehta, NN , Teague, HL , Swindell, WR , Baumer, Y , Ward, NL , Xing, X, et al. IFN-γ and TNF-α synergism may provide a link between psoriasis and inflammatory atherogenesis. Sci Rep. (2017) 7:13831. doi: 10.1038/s41598-017-14365-1
256. Kalesnikoff, J , and Galli, SJ . New developments in mast cell biology. Nat Immunol. (2008) 9:1215–23. doi: 10.1038/ni.f.216
257. da Silva, EZ , Jamur, MC , and Oliver, C . Mast cell function: a new vision of an old cell. J Histochem Cytochem. (2014) 62:698–738. doi: 10.1369/0022155414545334
258. McHale, C , Mohammed, Z , Deppen, J , and Gomez, G . Interleukin-6 potentiates FcεRI-induced PGD(2) biosynthesis and induces VEGF from human in situ-matured skin mast cells. Biochim Biophys Acta Gen Subj. (2018) 1862:1069–78. doi: 10.1016/j.bbagen.2018.01.020
259. Mihara, M , Hashizume, M , Yoshida, H , Suzuki, M , and Shiina, M . IL-6/IL-6 receptor system and its role in physiological and pathological conditions. Clin Sci. (2012) 122:143–59. doi: 10.1042/CS20110340
260. Ikeda, U , Ikeda, M , Seino, Y , Takahashi, M , Kano, S , and Shimada, K . Interleukin 6 gene transcripts are expressed in atherosclerotic lesions of genetically Hyperlipidemic rabbits. Atherosclerosis. (1992) 92:–8. doi: 10.1016/0021-9150(92)90280-t
261. Desai, A , Jung, MY , Olivera, A , Gilfillan, AM , Prussin, C , Kirshenbaum, AS, et al. IL-6 promotes an increase in human mast cell numbers and reactivity through suppression of suppressor of cytokine signaling 3. J Allergy Clin Immunol. (2016) 137:1863–71 e6. doi: 10.1016/j.jaci.2015.09.059
262. Kinoshita, M , Okada, M , Hara, M , Furukawa, Y , and Matsumori, A . Mast cell Tryptase in mast cell granules enhances MCP-1 and interleukin-8 production in human endothelial cells. Arterioscler Thromb Vasc Biol. (2005) 25:1858–63. doi: 10.1161/01.ATV.0000174797.71708.97
263. Nelken, NA , Coughlin, SR , Gordon, D , and Wilcox, JN . Monocyte chemoattractant protein-1 in human atheromatous plaques. J Clin Invest. (1991) 88:1121–7. doi: 10.1172/JCI115411
264. Takeya, M , Yoshimura, T , Leonard, EJ , and Takahashi, K . Detection of monocyte chemoattractant protein-1 in human atherosclerotic lesions by an anti-monocyte chemoattractant protein-1 monoclonal antibody. Hum Pathol. (1993) 24:534–9. doi: 10.1016/0046-8177(93)90166-E
265. Djeu, JY , Matsushima, K , Oppenheim, JJ , Shiotsuki, K , and Blanchard, DK . Functional activation of human neutrophils by recombinant monocyte-derived neutrophil chemotactic factor/IL-8. J Immunol. (1990) 144:2205–10. doi: 10.4049/jimmunol.144.6.2205
266. Yla-Herttuala, S , Lipton, BA , Rosenfeld, ME , Sarkioja, T , Yoshimura, T , Leonard, EJ, et al. Expression of monocyte chemoattractant protein 1 in macrophage-rich areas of human and rabbit atherosclerotic lesions. Proc Natl Acad Sci U S A. (1991) 88:5252–6. doi: 10.1073/pnas.88.12.5252
267. Gu, L , Okada, Y , Clinton, SK , Gerard, CJ , Sukhova, GK , Libby, P, et al. Absence of monocyte chemoattractant protein-1 reduces atherosclerosis in low density lipoprotein receptor-deficient mice. Mol Cell. (1998) 2:275–81. doi: 10.1016/S1097-2765(00)80139-2
268. Niyonsaba, F , Ushio, H , Hara, M , Yokoi, H , Tominaga, M , Takamori, K, et al. Antimicrobial peptides human beta-defensins and cathelicidin LL-37 induce the secretion of a pruritogenic cytokine IL-31 by human mast cells. J Immunol. (2010) 184:3526–34. doi: 10.4049/jimmunol.0900712
269. Di Nardo, A , Yamasaki, K , Dorschner, RA , Lai, Y , and Gallo, RL . Mast cell cathelicidin antimicrobial peptide prevents invasive group a streptococcus infection of the skin. J Immunol. (2008) 180:7565–73. doi: 10.4049/jimmunol.180.11.7565
270. Berenbaum, F , Humbert, L , Bereziat, G , and Thirion, S . Concomitant recruitment of ERK1/2 and p38 MAPK signalling pathway is required for activation of cytoplasmic phospholipase A2 via ATP in articular chondrocytes. J Biol Chem. (2003) 278:13680–7. doi: 10.1074/jbc.M211570200
271. Malaviya, R , and Abraham, SN . Role of mast cell leukotrienes in neutrophil recruitment and bacterial clearance in infectious peritonitis. J Leukoc Biol. (2000) 67:841. doi: 10.1002/jlb.67.6.841
272. Belton, O , Byrne, D , Kearney, D , Leahy, A , and Fitzgerald, DJ . Cyclooxygenase-1 and-2-dependent prostacyclin formation in patients with atherosclerosis. Circulation. (2000) 102:840–5. doi: 10.1161/01.CIR.102.8.840
273. Fitzgerald, GA , and Oates, JA . Selective and nonselective inhibition of thromboxane formation. Clin Pharmacol Therap. (1984) 35:633–40. doi: 10.1038/clpt.1984.87
274. Vane, JR , and Botting, RM . Pharmacodynamic profile of prostacyclin. Am J Cardiol. (1995) 75:3A–10A. doi: 10.1016/S0002-9149(99)80377-4
275. Catella-Lawson, F , McAdam, B , Morrison, BW , Kapoor, S , Kujubu, D , Antes, L, et al. Effects of specific inhibition of Cyclooxygenase-2 on sodium balance, hemodynamics, and vasoactive eicosanoids. J Pharmacol Exp Ther. (1999) 289:735–41.
276. McAdam, BF , Catella-Lawson, F , Mardini, IA , Kapoor, S , Lawson, JA , and Fitz Gerald, GA . Systemic biosynthesis of prostacyclin by cyclooxygenase (COX)-2: the human pharmacology of a selective inhibitor of COX-2. Proc Natl Acad Sci U S A. (1999) 96:272–7. doi: 10.1073/pnas.96.1.272
277. Wong, E , Huang, JQ , Tagari, P , and Riendeau, D . Effects of COX-2 inhibitors on aortic prostacyclin production in cholesterol-fed rabbits. Atherosclerosis. (2001) 157:393–402. doi: 10.1016/S0021-9150(00)00756-5
278. Cheng, Y , Austin, SC , Rocca, B , Koller, BH , Coffman, TM , Grosser, T, et al. Role of prostacyclin in the cardiovascular response to thromboxane A2. Science. (2002) 296:539–41. doi: 10.1126/science.1068711
279. Shaw, RJ , Cromwell, O , and Kay, AB . Preferential generation of leukotriene C4 by human eosinophils. Clin Exp Immunol. (1984) 56:716–22.
280. Adamek, A , Jung, S , Dienesch, C , Laser, M , Ertl, G , Bauersachs, J, et al. Role of 5-lipoxygenase in myocardial ischemia-reperfusion injury in mice. Eur J Pharmacol. (2007) 571:51–4. doi: 10.1016/j.ejphar.2007.05.040
281. Nobili, E , Salvado, MD , Folkersen, L , Castiglioni, L , Kastrup, J , Wetterholm, A, et al. Cysteinyl leukotriene signaling aggravates myocardial hypoxia in experimental atherosclerotic heart disease. PLoS One. (2012) 7:e41786. doi: 10.1371/journal.pone.0041786
282. Weller, CL , Collington, SJ , Brown, J , Miller, HRP , Al-Kashi, A , Clark, PC, et al. Leukotriene B4, an activation product of mast cells, is a chemoattractant for their progenitors. J Exp Med. (2005) 201:1961–71. doi: 10.1084/jem.20042407
283. Poto, R , Patella, V , Criscuolo, G , Marone, G , Coscioni, E , and Varricchi, G . Autoantibodies to IgE can induce the release of proinflammatory and vasoactive mediators from human cardiac mast cells. Clin Exp Med. (2022) 23:1265–76. doi: 10.1007/s10238-022-00861-w
284. Mohammed, Z , McHale, C , Kubinak, JL , Dryer, S , and Gomez, G . miR-155 is a positive regulator of FcεRI-induced Cyclooxygenase-2 expression and cytokine production in mast cells. Front Allergy. (2022) 3:835776. doi: 10.3389/falgy.2022.835776
285. Nagata, Y , and Suzuki, R . FcεRI: a master regulator of mast cell functions. Cells. (2022) 11:622. doi: 10.3390/cells11040622
286. Jönsson, F , and Daëron, M . Mast cells and company. Front Immunol. (2012) 3:16. doi: 10.3389/fimmu.2012.00016
287. Schäfer, B , Piliponsky, AM , Oka, T , Song, CH , Gerard, NP , Gerard, C, et al. Mast cell anaphylatoxin receptor expression can enhance IgE-dependent skin inflammation in mice. J Allergy Clin Immunol. (2013) 131:541.e1–8.e9. doi: 10.1016/j.jaci.2012.05.009
288. Kneilling, M , Mailhammer, R , Hültner, L , Schönberger, T , Fuchs, K , Schaller, M, et al. Direct crosstalk between mast cell-TNF and TNFR1-expressing endothelia mediates local tissue inflammation. Blood. (2009) 114:1696–706. doi: 10.1182/blood-2008-11-187682
289. Agier, J , Pastwinska, J , and Brzezinska-Blaszczyk, E . An overview of mast cell pattern recognition receptors. Inflamm Res. (2018) 67:737–46. doi: 10.1007/s00011-018-1164-5
290. Li, D , and Wu, M . Pattern recognition receptors in health and diseases. Signal Transduct Target Ther. (2021) 6:291. doi: 10.1038/s41392-021-00687-0
291. Medzhitov, R , and Janeway, CA Jr. Decoding the patterns of self and nonself by the innate immune system. Science. (2002) 296:298–300. doi: 10.1126/science.1068883
292. Sandig, H , and Bulfone-Paus, S . TLR signaling in mast cells: common and unique features. Front Immunol. (2012) 3:185. doi: 10.3389/fimmu.2012.00185
293. Poltorak, A , He, XP , Smirnova, II , Liu, MY , van Huffel, C , Du, X, et al. Defective LPS signaling in C3H/HeJ and C57BL/10ScCr mice: mutations in TLR4 gene. Science. (1998) 282:2085–8. doi: 10.1126/science.282.5396.2085
294. Takeuchi, O , Hoshino, K , Kawai, T , Sanjo, H , Takada, H , Ogawa, T, et al. Differential roles of TLR2 and TLR4 in recognition of gram-negative and gram-positive bacterial cell wall components. Immunity. (1999) 11:443–51. doi: 10.1016/S1074-7613(00)80119-3
295. Beutler, B . Toll-like receptors: how they work and what they do. Curr Opin Hematol. (2002) 9:2–10. doi: 10.1097/00062752-200201000-00002
296. Hoshino, K , Takeuchi, O , Kawai, T , Sanjo, H , Ogawa, T , Takeda, Y, et al. Pillars article: cutting edge: toll-like receptor 4 (TLR4)-deficient mice are hyporesponsive to lipopolysaccharide: evidence for TLR4 as the Lps gene product. J Immunol. (1999) 162:3749–52. doi: 10.4049/jimmunol.162.7.3749
297. Brightbill, HD , Libraty, DH , Krutzik, SR , Yang, RB , Belisle, JT , Bleharski, JR, et al. Host defense mechanisms triggered by microbial lipoproteins through toll-like receptors. Science. (1999) 285:732–6. doi: 10.1126/science.285.5428.732
298. Hirschfeld, M , Kirschning, CJ , Schwandner, R , Wesche, H , Weis, JH , Wooten, RM, et al. Cutting edge: inflammatory signaling by Borrelia Burgdorferi lipoproteins is mediated by toll-like receptor 2. J Immunol. (1999) 163:2382–6. doi: 10.4049/jimmunol.163.5.2382
299. Hertz, CJ , Kiertscher, SM , Godowski, PJ , Bouis, DA , Norgard, MV , Roth, MD, et al. Microbial Lipopeptides stimulate dendritic cell maturation via toll-like receptor 2. J Immunol. (2001) 166:2444–50. doi: 10.4049/jimmunol.166.4.2444
300. Lehner, MD , Morath, S , Michelsen, KS , Schumann, RR , and Hartung, T . Induction of cross-tolerance by lipopolysaccharide and highly purified Lipoteichoic acid via different toll-like receptors independent of paracrine mediators. J Immunol. (2001) 166:5161–7. doi: 10.4049/jimmunol.166.8.5161
301. Faure, E , Equils, O , Sieling, PA , Thomas, L , Zhang, FX , Kirschning, CJ, et al. Bacterial lipopolysaccharide activates Nf-Kappab through toll-like receptor 4 (TLR-4) in cultured human dermal endothelial cells. Differential expression of TLR-4 and TLR-2 in endothelial cells. J Biol Chem. (2000) 275:11058–63. doi: 10.1074/jbc.275.15.11058
302. Zeuke, S , Ulmer, AJ , Kusumoto, S , Katus, HA , and Heine, H . TLR4-mediated inflammatory activation of human coronary artery endothelial cells by Lps. Cardiovasc Res. (2002) 56:126–34. doi: 10.1016/S0008-6363(02)00512-6
303. Edfeldt, K , Swedenborg, J , Hansson, GK , and Yan, ZQ . Expression of toll-like receptors in human atherosclerotic lesions: a possible pathway for plaque activation. Circulation. (2002) 105:1158–61. doi: 10.1161/circ.105.10.1158
304. Kiechl, S , Lorenz, E , Reindl, M , Wiedermann, CJ , Oberhollenzer, F , Bonora, E, et al. Toll-like receptor 4 polymorphisms and atherogenesis. N Engl J Med. (2002) 347:185–92. doi: 10.1056/NEJMoa012673
305. Pasterkamp, G , Van Keulen, JK , and De Kleijn, DP . Role of toll-like receptor 4 in the initiation and progression of atherosclerotic disease. Eur J Clin Investig. (2004) 34:328–34. doi: 10.1111/j.1365-2362.2004.01338.x
306. Heger, LA , Hortmann, M , Albrecht, M , Colberg, C , Peter, K , Witsch, T, et al. Inflammation in acute coronary syndrome: expression of TLR2 mRNA is increased in platelets of patients with ACS. PLoS One. (2019) 14:e0224181. doi: 10.1371/journal.pone.0224181
307. Li, B , Xia, Y , and Hu, B . Infection and atherosclerosis: TLR-dependent pathways. Cell Mol Life Sci. (2020) 77:2751–69. doi: 10.1007/s00018-020-03453-7
308. Wong, GW , Zhuo, L , Kimata, K , Lam, BK , Satoh, N , and Stevens, RL . Ancient origin of mast cells. Biochem Biophys Res Commun. (2014) 451:314–8. doi: 10.1016/j.bbrc.2014.07.124
309. Maurer, M , Theoharides, T , Granstein, RD , Bischoff, SC , Bienenstock, J , Henz, B, et al. What is the physiological function of mast cells? Exp Dermatol. (2003) 12:886. doi: 10.1111/j.0906-6705.2003.0109a.x
310. Galli, SJ , Maurer, M , and Lantz, CS . Mast cells as sentinels of innate immunity. Curr Opin Immunol. (1999) 11:53–9. doi: 10.1016/S0952-7915(99)80010-7
311. Abraham, SN , Thankavel, K , and Malaviya, R . Mast cells as modulators of host defense in the lung. Front Biosci. (1997) 2:d78–87. doi: 10.2741/A176
312. Henz, BM , Maurer, M , Lippert, U , Worm, M , and Babina, M . Mast cells as initiators of immunity and host defense. Exp Dermatol. (2001) 10:1–10. doi: 10.1034/j.1600-0625.2001.100101.x
313. Krishnaswamy, G , Ajitawi, O , and Chi, DS . The human mast cell: an overview. Methods Mol Biol. (2006) 315:13–34. doi: 10.1385/1-59259-967-2:013
314. Carlos, D , Frantz, FG , Souza-Júnior, DA , Jamur, MC , Oliver, C , Ramos, SG, et al. TLR2-dependent mast cell activation contributes to the control of Mycobacterium Tuberculosis infection. Microbes Infect. (2009) 11:770–8. doi: 10.1016/j.micinf.2009.04.025
315. Gendrin, C , Shubin, NJ , Boldenow, E , Merillat, S , Clauson, M , Power, D, et al. Mast cell chymase decreases the severity of group B Streptococcus infections. J Allergy Clin Immunol. (2018) 142:120–9.e6. doi: 10.1016/j.jaci.2017.07.042
316. Chen, X , Niyonsaba, F , Ushio, H , Hara, M , Yokoi, H , Matsumoto, K, et al. Antimicrobial peptides human Β-Defensin (hBD)-3 and hBD-4 activate mast cells and increase skin vascular permeability. Eur J Immunol. (2007) 37:434–44. doi: 10.1002/eji.200636379
317. Theoharides, TC , Alysandratos, K-D , Angelidou, A , Delivanis, D-A , Sismanopoulos, N , Zhang, B, et al. Mast cells and inflammation. Biochim Biophys Acta. (2012) 1822:21–33. doi: 10.1016/j.bbadis.2010.12.014
318. Garcia-Rodriguez, KM , Bahri, R , Sattentau, C , Roberts, IS , Goenka, A , and Bulfone-Paus, S . Human mast cells exhibit an individualized pattern of antimicrobial responses. Immun Inflamm Dis. (2020) 8:198–210. doi: 10.1002/iid3.295
319. Pennock, JL , and Grencis, RK . The mast cell and gut nematodes: damage and defence. Chem Immunol Allergy. (2006) 90:128–40. doi: 10.1159/000088885
320. Vukman, KV , Lalor, R , Aldridge, A , and O'Neill, SM . Mast cells: new therapeutic target in helminth immune modulation. Parasite Immunol. (2016) 38:45–52. doi: 10.1111/pim.12295
321. Dudeck, A , Koberle, M , Goldmann, O , Meyer, N , Dudeck, J , Lemmens, S, et al. Mast cells as protectors of health. J Allergy Clin Immunol. (2019) 144:S4–S18. doi: 10.1016/j.jaci.2018.10.054
322. Hepworth, MR , Daniłowicz-Luebert, E , Rausch, S , Metz, M , Klotz, C , Maurer, M, et al. Mast cells orchestrate type 2 immunity to helminths through regulation of tissue-derived cytokines. Proc Natl Acad Sci. (2012) 109:6644–9. doi: 10.1073/pnas.1112268109
323. Linnemann, LC , Reitz, M , Feyerabend, TB , Breloer, M , and Hartmann, W . Limited role of mast cells during infection with the parasitic nematode Litomosoides Sigmodontis. PLoS Negl Trop Dis. (2020) 14:e0008534. doi: 10.1371/journal.pntd.0008534
324. Burton, OT , and Oettgen, HC . Beyond immediate hypersensitivity: evolving roles for IgE antibodies in immune homeostasis and allergic diseases. Immunol Rev. (2011) 242:128–43. doi: 10.1111/j.1600-065X.2011.01024.x
325. Hepworth, MR , Maurer, M , and Hartmann, S . Regulation of type 2 immunity to helminths by mast cells. Gut Microbes. (2012) 3:476–81. doi: 10.4161/gmic.21507
326. Mukai, K , Tsai, M , Starkl, P , Marichal, T , and Galli, SJ . IgE and mast cells in host defense against parasites and venoms. Semin Immunopathol. (2016) 38:581–603. doi: 10.1007/s00281-016-0565-1
327. Lu, F , and Huang, S . The roles of mast cells in parasitic protozoan infections. Front Immunol. (2017) 8:363. doi: 10.3389/fimmu.2017.00363
328. Sakata-Yanagimoto, M , Sakai, T , Miyake, Y , Saito, TI , Maruyama, H , Morishita, Y, et al. Notch2 signaling is required for proper mast cell distribution and mucosal immunity in the intestine. Blood. (2011) 117:128–34. doi: 10.1182/blood-2010-07-289611
329. McKean, PG , and Pritchard, DI . The action of a mast cell protease on the cuticular collagens of Necator Americanus. Parasite Immunol. (1989) 11:293–7. doi: 10.1111/j.1365-3024.1989.tb00667.x
330. Maruyama, H , Yabu, Y , Yoshida, A , Nawa, Y , and Ohta, N . A role of mast cell Glycosaminoglycans for the immunological expulsion of intestinal nematode. Strongyloides Venezuelensis J Immunol. (2000) 164:3749–54. doi: 10.4049/jimmunol.164.7.3749
331. Ryan Nathan, M , and Oghumu, S . Role of mast cells in the generation of a T-helper type 2 dominated anti-helminthic immune response. Biosci Rep. (2019) 39:1771. doi: 10.1042/BSR20181771
332. Machado, ER , Ueta, MT , Lourenço, EV , Anibal, FF , Sorgi, CA , Soares, EG, et al. Leukotrienes play a role in the control of parasite burden in murine Strongyloidiasis. J Immunol. (2005) 175:3892–9. doi: 10.4049/jimmunol.175.6.3892
333. Huang, L , Gebreselassie, NG , Gagliardo, LF , Ruyechan, MC , Lee, NA , Lee, JJ, et al. Eosinophil-derived IL-10 supports chronic nematode infection. J Immunol. (2014) 193:4178–87. doi: 10.4049/jimmunol.1400852
334. Farid, AS , Jimi, F , Inagaki-Ohara, K , and Horii, Y . Increased intestinal endotoxin absorption during enteric nematode but not protozoal infections through a mast cell-mediated mechanism. Shock. (2008) 29:709–16. doi: 10.1097/SHK.0b013e31815c3f36
335. Lawrence, CE , Paterson, YY , Wright, SH , Knight, PA , and Miller, HR . Mouse mast cell protease-1 is required for the enteropathy induced by gastrointestinal helminth infection in the mouse. Gastroenterology. (2004) 127:155–65. doi: 10.1053/j.gastro.2004.04.004
336. McDermott, JR , Bartram, RE , Knight, PA , Miller, HR , Garrod, DR , and Grencis, RK . Mast cells disrupt epithelial barrier function during enteric nematode infection. Proc Natl Acad Sci U S A. (2003) 100:7761–6. doi: 10.1073/pnas.1231488100
337. Bertaccini, G , Morini, G , and Coruzzi, G . Different mechanisms are responsible for the contractile effects of histaminergic compounds on isolated intestinal smooth muscle cells. J Physiol Paris. (1997) 91:199–202. doi: 10.1016/S0928-4257(97)89484-3
338. Anthony, RM , Rutitzky, LI , Urban, JF Jr, Stadecker, MJ , and Gause, WC . Protective immune mechanisms in helminth infection. Nat Rev Immunol. (2007) 7:975–87. doi: 10.1038/nri2199
339. Fitzsimmons, CM , Falcone, FH , and Dunne, DW . Helminth allergens, parasite-specific IgE, and its protective role in human immunity. Front Immunol. (2014) 5:61. doi: 10.3389/fimmu.2014.00061
340. Gounni, AS , Lamkhioued, B , Ochiai, K , Tanaka, Y , Delaporte, E , Capron, A, et al. High-affinity IgE receptor on eosinophils is involved in defence against parasites. Nature. (1994) 367:183–6. doi: 10.1038/367183a0
341. Prakash, PS , Weber, MHW , van Hellemond, JJ , and Falcone, FH . Are humanized IgE reporter systems potential game changers in serological diagnosis of human parasitic infection? Parasitol Res. (2022) 121:1137–44. doi: 10.1007/s00436-021-07352-z
342. Guermonprez, P , Helft, J , Claser, C , Deroubaix, S , Karanje, H , Gazumyan, A, et al. Inflammatory Flt3l is essential to mobilize dendritic cells and for T cell responses during plasmodium infection. Nat Med. (2013) 19:730–8. doi: 10.1038/nm.3197
343. Mecheri, S . Contribution of allergic inflammatory response to the pathogenesis of malaria disease. Biochim Biophys Acta. (2012) 1822:49–56. doi: 10.1016/j.bbadis.2011.02.005
344. Beghdadi, W , Porcherie, A , Schneider, BS , Dubayle, D , Peronet, R , Huerre, M, et al. Inhibition of histamine-mediated signaling confers significant protection against severe malaria in mouse models of disease. J Exp Med. (2008) 205:395–408. doi: 10.1084/jem.20071548
345. Potts, RA , Tiffany, CM , Pakpour, N , Lokken, KL , Tiffany, CR , Cheung, K, et al. Mast cells and histamine alter intestinal permeability during malaria parasite infection. Immunobiology. (2016) 221:468–74. doi: 10.1016/j.imbio.2015.11.003
346. Demeure, CE , Brahimi, K , Hacini, F , Marchand, F , Péronet, R , Huerre, M, et al. Anopheles mosquito bites activate cutaneous mast cells leading to a local inflammatory response and lymph node hyperplasia. J Immunol. (2005) 174:3932–40. doi: 10.4049/jimmunol.174.7.3932
347. Körner, H , McMorran, B , Schlüter, D , and Fromm, P . The role of TNF in parasitic diseases: still more questions than answers. Int J Parasitol. (2010) 40:879–88. doi: 10.1016/j.ijpara.2010.03.011
348. Furuta, T , Imajo-Ohmi, S , Fukuda, H , Kano, S , Miyake, K , and Watanabe, N . Mast cell-mediated immune responses through IgE antibody and toll-like receptor 4 by malarial Peroxiredoxin. Eur J Immunol. (2008) 38:1341–50. doi: 10.1002/eji.200738059
349. Depinay, N , Hacini, F , Beghdadi, W , Peronet, R , and Mécheri, S . Mast cell-dependent Down-regulation of antigen-specific immune responses by mosquito bites. J Immunol. (2006) 176:4141–6. doi: 10.4049/jimmunol.176.7.4141
350. Furuta, T , Kimura, M , and Watanabe, N . Elevated levels of vascular endothelial growth factor (VEGF) and soluble vascular endothelial growth factor receptor (VEGFR)-2 in human malaria. Am J Trop Med Hyg. (2010) 82:136–9. doi: 10.4269/ajtmh.2010.09-0203
351. Chau, JY , Tiffany, CM , Nimishakavi, S , Lawrence, JA , Pakpour, N , Mooney, JP, et al. Malaria-associated L-arginine deficiency induces mast cell-associated disruption to intestinal barrier defenses against nontyphoidal Salmonella bacteremia. Infect Immun. (2013) 81:3515–26. doi: 10.1128/IAI.00380-13
352. Brossard, M , and Wikel, SK . Immunology of interactions between ticks and hosts. Med Vet Entomol. (1997) 11:270–6. doi: 10.1111/j.1365-2915.1997.tb00406.x
353. Kim, HK . Rickettsia-host-tick interactions: knowledge advances and gaps. Infect Immun. (2022) 90:e0062121. doi: 10.1128/iai.00621-21
354. Parola, P , and Raoult, D . Ticks and Tickborne bacterial diseases in humans: an emerging infectious threat. Clin Infect Dis. (2001) 32:897–928. doi: 10.1086/319347
355. Duzlu, O , Yildirim, A , Inci, A , Gumussoy, KS , Ciloglu, A , and Onder, Z . Molecular investigation of Francisella-like endosymbiont in ticks and Francisella Tularensis in Ixodid ticks and mosquitoes in Turkey. Vector Borne Zoonotic Dis. (2016) 16:26–32. doi: 10.1089/vbz.2015.1818
356. Magnarelli, LA , Stafford, KC 3rd, Mather, TN , Yeh, MT , Horn, KD , and Dumler, JS . Hemocytic Rickettsia-like organisms in ticks: serologic reactivity with antisera to Ehrlichiae and detection of DNA of agent of human granulocytic Ehrlichiosis by PCR. J Clin Microbiol. (1995) 33:2710–4. doi: 10.1128/jcm.33.10.2710-2714.1995
357. Mendell, NL , Reynolds, ES , Blanton, LS , Hermance, ME , Londoño, AF , Hart, CE, et al. Detection of Rickettsiae, Borreliae, and Ehrlichiae in ticks collected from Walker County, Texas, 2017-2018. Insects. (2019) 10:315. doi: 10.3390/insects10100315
358. Zelechowska, P , Pastwinska, J , Brzezinska-Blaszczyk, E , and Agier, J . Do mast cells contribute to the antifungal host defense? Cells. (2021) 10:2510. doi: 10.3390/cells10102510
359. Saluja, R , Metz, M , and Maurer, M . Role and relevance of mast cells in fungal infections. Front Immunol. (2012) 3:146. doi: 10.3389/fimmu.2012.00146
360. Elieh Ali Komi, D , Shafaghat, F , and Haidl, G . Significance of mast cells in spermatogenesis, implantation, pregnancy, and abortion: cross talk and molecular mechanisms. Am J Reprod Immunol. (2020) 83:e13228. doi: 10.1111/aji.13228
361. Di Persio, S , and Neuhaus, N . Human Spermatogonial stem cells and their niche in male (in)fertility: novel concepts from single-cell RNA-sequencing. Hum Reprod. (2023) 38:1–13. doi: 10.1093/humrep/deac245
362. Mondillo, C , Patrignani, Z , Reche, C , Rivera, E , and Pignataro, O . Dual role of histamine in modulation of Leydig cell steroidogenesis via HRH1 and HRH2 receptor subtypes. Biol Reprod. (2005) 73:899–907. doi: 10.1095/biolreprod.105.041285
363. Khan, UW , and Rai, U . Differential effects of histamine on Leydig cell and testicular macrophage activities in wall lizards: precise role of H1/H2 receptor subtypes. J Endocrinol. (2007) 194:441–8. doi: 10.1677/JOE-06-0225
364. Godbole, G , Suman, P , Gupta, SK , and Modi, D . Decidualized endometrial stromal cell derived factors promote trophoblast invasion. Fertil Steril. (2011) 95:1278–83. doi: 10.1016/j.fertnstert.2010.09.045
365. Ueshima, C , Kataoka, TR , Hirata, M , Sugimoto, A , Iemura, Y , Minamiguchi, S, et al. Possible involvement of human mast cells in the establishment of pregnancy via killer cell Ig-like receptor 2DL4. Am J Pathol. (2018) 188:1497–508. doi: 10.1016/j.ajpath.2018.02.012
366. Derbala, Y , Elazzamy, H , Bilal, M , Reed, R , Salazar Garcia, MD , Skariah, A, et al. Mast cell–induced immunopathology in recurrent pregnancy losses. Am J Reprod Immunol. (2019) 82:e13128. doi: 10.1111/aji.13128
367. Jensen, F , Woudwyk, M , Teles, A , Woidacki, K , Taran, F , Costa, S, et al. Estradiol and progesterone regulate the migration of mast cells from the periphery to the uterus and induce their maturation and degranulation. PLoS One. (2010) 5:e14409. doi: 10.1371/journal.pone.0014409
368. Norstrom, A , Vukas Radulovic, N , Bullarbo, M , and Ekerhovd, E . Mast cell involvement in human cervical ripening. Eur J Obstet Gynecol Reprod Biol. (2019) 238:157–63. doi: 10.1016/j.ejogrb.2019.05.010
369. Marx, L , Arck, P , Kieslich, C , Mitterlechner, S , Kapp, M , and Dietl, J . Decidual mast cells might be involved in the onset of human first-trimester abortion. Am J Reprod Immunol. (1999) 41:34–40. doi: 10.1111/j.1600-0897.1999.tb00073.x
370. Komi, DEA , Khomtchouk, K , and Santa Maria, PL . A review of the contribution of mast cells in wound healing: involved molecular and cellular mechanisms. Clin Rev Allergy Immunol. (2020) 58:298–312. doi: 10.1007/s12016-019-08729-w
371. Dudeck, J , Kotrba, J , Immler, R , Hoffmann, A , Voss, M , Alexaki, VI, et al. Directional mast cell degranulation of tumor necrosis factor into blood vessels primes neutrophil extravasation. Immunity. (2021) 54:468–83.e5. doi: 10.1016/j.immuni.2020.12.017
372. Ud-Din, S , Wilgus, TA , and Bayat, A . Mast cells in skin scarring: a review of animal and human research. Front Immunol. (2020) 11:552205. doi: 10.3389/fimmu.2020.552205
373. Rodrigues, M , Kosaric, N , Bonham, CA , and Gurtner, GC . Wound healing: a cellular perspective. Physiol Rev. (2019) 99:665–706. doi: 10.1152/physrev.00067.2017
374. Bacci, S . Fine regulation during wound healing by mast cells, a physiological role not yet clarified. Int J Mol Sci. (2022) 23:1820. doi: 10.3390/ijms23031820
375. Bonelli, A , Bacci, S , and Norelli, GA . Affinity Cytochemistry analysis of mast cells in skin lesions: a possible tool to assess the timing of lesions after death. Int J Legal Med. (2003) 117:331–4. doi: 10.1007/s00414-003-0396-1
376. Weller, K , Foitzik, K , Paus, R , Syska, W , and Maurer, M . Mast cells are required for normal healing of skin wounds in mice. FASEB J. (2006) 20:2366–8. doi: 10.1096/fj.06-5837fje
377. Steinhoff, M , Buddenkotte, J , Shpacovitch, V , Rattenholl, A , Moormann, C , Vergnolle, N, et al. Proteinase-activated receptors: transducers of proteinase-mediated signaling in inflammation and immune response. Endocr Rev. (2005) 26:1–43. doi: 10.1210/er.2003-0025
378. Terakawa, M , Tomimori, Y , Goto, M , and Fukuda, Y . Mast cell chymase induces expression of chemokines for neutrophils in eosinophilic EoL-1 cells and mouse peritonitis eosinophils. Eur J Pharmacol. (2006) 538:175–81. doi: 10.1016/j.ejphar.2006.03.053
379. Egozi, EI , Ferreira, AM , Burns, AL , Gamelli, RL , and Dipietro, LA . Mast cells modulate the inflammatory but not the proliferative response in healing wounds. Wound Repair Regen. (2003) 11:46–54. doi: 10.1046/j.1524-475X.2003.11108.x
380. Gailit, J , Marchese, MJ , Kew, RR , and Gruber, BL . The differentiation and function of Myofibroblasts is regulated by mast cell mediators. J Invest Dermatol. (2001) 117:1113–9. doi: 10.1046/j.1523-1747.2001.15211.x
381. Norrby, K , Jakobsson, A , and Sörbo, J . Mast-cell-mediated angiogenesis: a novel experimental model using the rat mesentery. Virchows Arch B Cell Pathol Incl Mol Pathol. (1986) 52:195–206. doi: 10.1007/BF02889963
382. Abdel-Majid, RM , and Marshall, JS . Prostaglandin E2 induces degranulation-independent production of vascular endothelial growth factor by human mast cells. J Immunol. (2004) 172:1227–36. doi: 10.4049/jimmunol.172.2.1227
383. Muramatsu, M , Katada, J , Hattori, M , Hayashi, I , and Majima, M . Chymase mediates mast cell-induced angiogenesis in Hamster sponge granulomas. Eur J Pharmacol. (2000) 402:181–91. doi: 10.1016/S0014-2999(00)00350-2
384. Doggrell, SA , and Wanstall, JC . Vascular chymase: pathophysiological role and therapeutic potential of inhibition. Cardiovasc Res. (2004) 61:653–62. doi: 10.1016/j.cardiores.2003.11.029
385. Somasundaram, P , Ren, G , Nagar, H , Kraemer, D , Mendoza, L , Michael, LH, et al. Mast cell tryptase may modulate endothelial cell phenotype in healing myocardial infarcts. J Pathol. (2005) 205:102–11. doi: 10.1002/path.1690
386. Shiota, N , Nishikori, Y , Kakizoe, E , Shimoura, K , Niibayashi, T , Shimbori, C, et al. Pathophysiological role of skin mast cells in wound healing after scald injury: study with mast cell-deficient W/WV mice. Int Arch Allergy Immunol. (2009) 151:80–8. doi: 10.1159/000232573
387. Presta, M , Leali, D , Stabile, H , Ronca, R , Camozzi, M , Coco, L, et al. Heparin derivatives as angiogenesis inhibitors. Curr Pharm Des. (2003) 9:553–66. doi: 10.2174/1381612033391379
388. Almadani, YH , Vorstenbosch, J , Davison, PG , and Murphy, AM . Wound healing: a comprehensive review. Semin Plast Surg. (2021) 35:141–4. doi: 10.1055/s-0041-1731791
389. Abe, M , Kurosawa, M , Ishikawa, O , Miyachi, Y , and Kido, H . Mast cell Tryptase stimulates both human dermal fibroblast proliferation and type I collagen production. Clin Exp Allergy. (1998) 28:1509–17. doi: 10.1046/j.1365-2222.1998.00360.x
390. Maurer, M , Opitz, M , Henz, BM , and Paus, R . The mast cell products histamine and serotonin stimulate and TNF-alpha inhibits the proliferation of murine epidermal keratinocytes in situ. J Dermatol Sci. (1997) 16:79–84. doi: 10.1016/S0923-1811(97)00043-1
391. Wulff, BC , and Wilgus, TA . Mast cell activity in the healing wound: more than meets the eye? Exp Dermatol. (2013) 22:507–10. doi: 10.1111/exd.12169
392. Bacci, S . Cellular mechanisms and therapies in wound healing: looking toward the future. Biomedicine. (2021) 9:611. doi: 10.3390/biomedicines9111611
393. Beghdadi, W , Madjene, LC , Benhamou, M , Charles, N , Gautier, G , Launay, P, et al. Mast cells as cellular sensors in inflammation and immunity. Front Immunol. (2011) 2:37. doi: 10.3389/fimmu.2011.00037
394. Springer, JM , Raveendran, VV , Zhang, M , Funk, R , Smith, DD , Maz, M, et al. Mast cell degranulation decreases lipopolysaccharide-induced aortic gene expression and systemic levels of Interleukin-6 in vivo. Mediat Inflamm. (2019) 2019:3856360. doi: 10.1155/2019/3856360
395. Klaiber, N , Kumar, S , and Irani, AM . Mastocytosis in children. Curr Allergy Asthma Rep. (2017) 17:80. doi: 10.1007/s11882-017-0748-4
396. Pardanani, A . Systemic Mastocytosis in adults: 2021 update on diagnosis, risk stratification and management. Am J Hematol. (2021) 96:508–25. doi: 10.1002/ajh.26118
397. Indhirajanti, S , van Daele, PLA , Bos, S , Mulder, MT , Bot, I , and Roeters van Lennep, JE . Systemic Mastocytosis associates with cardiovascular events despite lower plasma lipid levels. Atherosclerosis. (2018) 268:152–6. doi: 10.1016/j.atherosclerosis.2017.11.030
398. Hermans, M , Lennep, JRV , van Daele, P , and Bot, I . Mast cells in cardiovascular disease: from bench to bedside. Int J Mol Sci. (2019) 20:3395. doi: 10.3390/ijms20143395
399. Akin, C . Mast cell activation syndromes. J Allergy Clin Immunol. (2017) 140:349–55. doi: 10.1016/j.jaci.2017.06.007
400. Afrin, LB , Weinstock, LB , and Molderings, GJ . COVID-19 Hyperinflammation and post-COVID-19 illness may be rooted in mast cell activation syndrome. Int J Infect Dis. (2020) 100:327–32. doi: 10.1016/j.ijid.2020.09.016
401. Arun, S , Storan, A , and Myers, B . Mast cell activation syndrome and the link with long COVID. Br J Hosp Med (Lond). (2022) 83:1–10. doi: 10.12968/hmed.2022.0123
402. Galli, SJ , and Tsai, M . Mast cells in allergy and infection: versatile effector and regulatory cells in innate and adaptive immunity. Eur J Immunol. (2010) 40:1843–51. doi: 10.1002/eji.201040559
403. Tikoo, S , Barki, N , Jain, R , Zulkhernain, NS , Buhner, S , Schemann, M, et al. Imaging of mast cells. Immunol Rev. (2018) 282:58–72. doi: 10.1111/imr.12631
404. Heard, BE , Nunn, AJ , and Kay, AB . Mast cells in human lungs. J Pathol. (1989) 157:59–63. doi: 10.1002/path.1711570108
405. Traina, G . The role of mast cells in the gut and brain. J Integr Neurosci. (2021) 20:185–96. doi: 10.31083/j.jin.2021.01.313
406. Enerbäck, L . Mucosal mast cells in the rat and in man. Int Arch Allergy Appl Immunol. (1987) 82:249–55. doi: 10.1159/000234199
407. Church, MK , and Clough, GF . Human skin mast cells: in vitro and in vivo studies. Ann Allergy Asthma Immunol. (1999) 83:471–5. doi: 10.1016/S1081-1206(10)62853-0
408. Eisenbarth, SC , Piggott, DA , Huleatt, JW , Visintin, I , Herrick, CA , and Bottomly, K . Lipopolysaccharide-enhanced, toll-like receptor 4-dependent T helper cell type 2 responses to inhaled antigen. J Exp Med. (2002) 196:1645–51. doi: 10.1084/jem.20021340
409. Nigo, YI , Yamashita, M , Hirahara, K , Shinnakasu, R , Inami, M , Kimura, M, et al. Regulation of allergic airway inflammation through toll-like receptor 4-mediated modification of mast cell function. Proc Natl Acad Sci U S A. (2006) 103:2286–91. doi: 10.1073/pnas.0510685103
410. Hofmann, AM , and Abraham, SN . New roles for mast cells in modulating allergic reactions and immunity against pathogens. Curr Opin Immunol. (2009) 21:679–86. doi: 10.1016/j.coi.2009.09.007
411. Durham, SR , Varney, VA , Gaga, M , Jacobson, MR , Varga, EM , Frew, AJ, et al. Grass pollen immunotherapy decreases the number of mast cells in the skin. Clin Exp Allergy. (1999) 29:1490–6. doi: 10.1046/j.1365-2222.1999.00678.x
412. Tsicopoulos, A , Hamid, Q , Haczku, A , Jacobson, MR , Durham, SR , North, J, et al. Kinetics of cell infiltration and cytokine messenger RNA expression after intradermal challenge with allergen and tuberculin in the same atopic individuals. J Allergy Clin Immunol. (1994) 94:764–72. doi: 10.1016/0091-6749(94)90185-6
413. Williams, CM , and Galli, SJ . The diverse potential effector and Immunoregulatory roles of mast cells in allergic disease. J Allergy Clin Immunol. (2000) 105:847–59. doi: 10.1067/mai.2000.106485
414. Grimbaldeston, MA , Metz, M , Yu, M , Tsai, M , and Galli, SJ . Effector and potential Immunoregulatory roles of mast cells in IgE-associated acquired immune responses. Curr Opin Immunol. (2006) 18:751–60. doi: 10.1016/j.coi.2006.09.011
415. Gregory, GD , and Brown, MA . Mast cells in allergy and autoimmunity: implications for adaptive immunity. Methods Mol Biol. (2006) 315:35–50. doi: 10.1385/1-59259-967-2:035
416. Montealegre, F , Quiñones, C , Torres, N , and Goth, K . Detection of serine proteases in extracts of the domestic mite Blomia Tropicalis. Exp Appl Acarol. (2002) 26:87–100. doi: 10.1023/a:1020931221953
417. Miike, S , and Kita, H . Human eosinophils are activated by cysteine proteases and release inflammatory mediators. J Allergy Clin Immunol. (2003) 111:704–13. doi: 10.1067/mai.2003.1332
418. Galli, SJ . The Paul Kallos memorial lecture. The mast cell: a versatile effector cell for a challenging world. Int Arch Allergy Immunol. (1997) 113:14–22. doi: 10.1159/000237497
419. Füreder, W , Agis, H , Willheim, M , Bankl, HC , Maier, U , Kishi, K, et al. Differential expression of complement receptors on human basophils and mast cells. Evidence for mast cell heterogeneity and Cd88/C5ar expression on skin mast cells. J Immunol. (1995) 155:3152–60. doi: 10.4049/jimmunol.155.6.3152
420. Wojta, J , Kaun, C , Zorn, G , Ghannadan, M , Hauswirth, AW , Sperr, WR, et al. C5a stimulates production of plasminogen activator Inhibitor-1 in human mast cells and basophils. Blood. (2002) 100:517–23. doi: 10.1182/blood.V100.2.517
421. Ansel, JC , Brown, JR , Payan, DG , and Brown, MA . Substance P selectively activates TNF-alpha gene expression in murine mast cells. J Immunol. (1993) 150:4478–85. doi: 10.4049/jimmunol.150.10.4478
422. Gomez, G . Current strategies to inhibit high affinity Fcεri-mediated signaling for the treatment of allergic disease. Front Immunol. (2019) 10:175. doi: 10.3389/fimmu.2019.00175
423. Davies, MJ . The composition of coronary-artery plaques. N Engl J Med. (1997) 336:1312–4. doi: 10.1056/NEJM199705013361809
424. Ross, R . Atherosclerosis--an inflammatory disease. N Engl J Med. (1999) 340:115–26. doi: 10.1056/NEJM199901143400207
425. Glass, CK , and Witztum, JL . Atherosclerosis. Road Ahead Cell. (2001) 104:503–16. doi: 10.1016/s0092-8674(01)00238-0
426. Hansson, GK , Libby, P , Schonbeck, U , and Yan, ZQ . Innate and adaptive immunity in the pathogenesis of atherosclerosis. Circ Res. (2002) 91:281–91. doi: 10.1161/01.RES.0000029784.15893.10
427. Atkinson, JB , Harlan, CW , Harlan, GC , and Virmani, R . The Association of mast cells and atherosclerosis: a morphologic study of early atherosclerotic lesions in young people. Hum Pathol. (1994) 25:154–9. doi: 10.1016/0046-8177(94)90271-2
428. Dvorak, AM . Mast-cell degranulation in human hearts. N Engl J Med. (1986) 315:969–70. doi: 10.1056/nejm198610093151515
429. Clejan, S , Japa, S , Clemetson, C , Hasabnis, SS , David, O , and Talano, JV . Blood histamine is associated with coronary artery disease, cardiac events and severity of inflammation and atherosclerosis. J Cell Mol Med. (2002) 6:583–92. doi: 10.1111/j.1582-4934.2002.tb00456.x
430. Deng, L , Hong, T , Lin, J , Ding, S , Huang, Z , Chen, J, et al. Histamine deficiency exacerbates myocardial injury in acute myocardial infarction through impaired macrophage infiltration and increased cardiomyocyte apoptosis. Sci Rep. (2015) 5:13131. doi: 10.1038/srep13131
431. Colazzo, F , Gelosa, P , Tremoli, E , Sironi, L , and Castiglioni, L . Role of the Cysteinyl leukotrienes in the pathogenesis and progression of cardiovascular diseases. Mediat Inflamm. (2017) 2017:2432958. doi: 10.1155/2017/2432958
432. Williams, JW . Huang L-h, Randolph GJ. Cytokine Circuits in Cardiovascular Disease Immunity. (2019) 50:941–54. doi: 10.1016/j.immuni.2019.03.007
433. Mukherjee, D , and Topol, EJ . The role of low-molecular-weight heparin in cardiovascular diseases. Prog Cardiovasc Dis. (2002) 45:139–56. doi: 10.1053/pcad.2002.127679
434. Esper, RJ , Nordaby, RA , Vilarino, JO , Paragano, A , Cacharron, JL , and Machado, RA . Endothelial dysfunction: a comprehensive appraisal. Cardiovasc Diabetol. (2006) 5:4. doi: 10.1186/1475-2840-5-4
435. Favero, G , Paganelli, C , Buffoli, B , Rodella, LF , and Rezzani, R . Endothelium and its alterations in cardiovascular diseases: life style intervention. Biomed Res Int. (2014) 2014:801896. doi: 10.1155/2014/801896
436. Smith, DD , Tan, X , Raveendran, VV , Tawfik, O , Stechschulte, DJ , and Dileepan, KN . Mast cell deficiency attenuates progression of atherosclerosis and hepatic steatosis in apolipoprotein E-null mice. Am J Physiol Heart Circ Physiol. (2012) 302:H2612–21. doi: 10.1152/ajpheart.00879.2011
437. Fernex, M. The mast-cell system: its relationship to atherosclerosis, fibrosis and eosinophils. New York: S. Karger AG (1968). 112–128 p.
438. Kovanen, PT , Kaartinen, M , and Paavonen, T . Infiltrates of activated mast cells at the site of coronary atheromatous Erosion or rupture in myocardial infarction. Circulation. (1995) 92:1084–8. doi: 10.1161/01.CIR.92.5.1084
439. Rozsívalová, K , Pierzynová, A , Kratochvílová, H , Lindner, J , Lipš, M , Kotulák, T, et al. Increased number of mast cells in Epicardial adipose tissue of cardiac surgery patients with coronary artery disease. Physiol Res. (2020) 69:621–31. doi: 10.33549/physiolres.934344
440. Bankl, HC , Grobschmidt, K , Pikula, B , Bankl, H , Lechner, K , and Valent, P . Mast cells are augmented in deep vein thrombosis and express a Profibrinolytic phenotype. Hum Pathol. (1999) 30:188–94. doi: 10.1016/S0046-8177(99)90274-5
441. Ponomaryov, T , Payne, H , Fabritz, L , Wagner, DD , and Brill, A . Mast cells granular contents are crucial for deep vein thrombosis in mice. Circ Res. (2017) 121:941–50. doi: 10.1161/CIRCRESAHA.117.311185
442. DeSchryver-Kecskemeti, K , Williamson, JR , Jakschik, BA , Clouse, RE , and Alpers, DH . Mast cell granules within endothelial cells: a possible signal in the inflammatory process? Mod Pathol. (1992) 5:343–7.
443. Marks, RM , Roche, WR , Czerniecki, M , Penny, R , and Nelson, DS . Mast cell granules cause proliferation of human microvascular endothelial cells. Lab Investig. (1986) 55:289–94.
444. Fabricant, CG , and Fabricant, J . Atherosclerosis induced by infection with Marek’s disease herpesvirus in chickens. Am Heart J. (1999) 138:S465–8. doi: 10.1016/S0002-8703(99)70276-0
445. Pussinen, PJ , Paju, S , Koponen, J , Viikari, JSA , Taittonen, L , Laitinen, T, et al. Association of childhood oral infections with cardiovascular risk factors and subclinical atherosclerosis in adulthood. JAMA Netw Open. (2019) 2:e192523. doi: 10.1001/jamanetworkopen.2019.2523
446. Xia, X , Zhang, L , Chi, J , Li, H , Liu, X , Hu, T, et al. Helicobacter Pylori infection impairs endothelial function through an exosome-mediated mechanism. J Am Heart Assoc. (2020) 9:e014120. doi: 10.1161/JAHA.119.014120
447. Schumski, A , Ortega-Gómez, A , Wichapong, K , Winter, C , Lemnitzer, P , Viola, JR, et al. Endotoxinemia accelerates atherosclerosis through electrostatic charge–mediated monocyte adhesion. Circulation. (2021) 143:254–66. doi: 10.1161/CIRCULATIONAHA.120.046677
448. Huaman, MA , Qualls, JE , Jose, S , Schmidt, SM , Moussa, A , Kuhel, DG, et al. Mycobacterium Bovis Bacille-Calmette-Guérin infection aggravates atherosclerosis. Front Immunol. (2020):11. doi: 10.3389/fimmu.2020.607957
449. Krupa, A , Gonciarz, W , Rusek-Wala, P , Rechciński, T , Gajewski, A , Samsel, Z, et al. Helicobacter Pylori infection acts synergistically with a high-fat diet in the development of a Proinflammatory and potentially Proatherogenic endothelial cell environment in an experimental model. Int J Mol Sci. (2021) 22:3394. doi: 10.3390/ijms22073394
450. Miao, G , Zhao, X , Wang, B , Zhang, L , Wang, G , Zheng, N, et al. TLR2/CXCR4 coassociation facilitates Chlamydia Pneumoniae infection-induced atherosclerosis. Am J Phys Heart Circ Phys. (2020) 318:H1420–35. doi: 10.1152/ajpheart.00011.2020
451. Zhao, X , Miao, G , Zhang, L , Zhang, Y , Zhao, H , Xu, Z, et al. Chlamydia Pneumoniae infection induces vascular smooth muscle cell migration and atherosclerosis through mitochondrial reactive oxygen species-mediated JunB-Fra-1 activation. Front Cell Dev Biol. (2022) 10:879023. doi: 10.3389/fcell.2022.879023
452. Grayston, JT , Campbell, LA , Kuo, CC , Mordhorst, CH , Saikku, P , Thom, DH, et al. A new respiratory tract pathogen: Chlamydia Pneumoniae strain TWAR. J Infect Dis. (1990) 161:618–25. doi: 10.1093/infdis/161.4.618
453. Kol, A , and Libby, P . The mechanisms by which infectious agents may contribute to atherosclerosis and its clinical manifestations. Trends Cardiovasc Med. (1998) 8:191–9. doi: 10.1016/S1050-1738(98)00010-3
454. Saikku, P , Leinonen, M , Tenkanen, L , Linnanmäki, E , Ekman, M , Manninen, V, et al. Chronic Chlamydia Pneumoniae infection as a risk factor for coronary heart disease in the Helsinki heart study. Ann Intern Med. (1992) 116:273–8. doi: 10.7326/0003-4819-116-4-273
455. Gupta, S , and Camm, AJ . Chronic infection in the etiology of atherosclerosis--the case for Chlamydia Pneumoniae. Clin Cardiol. (1997) 20:829–36. doi: 10.1002/clc.4960201008
456. Gaydos, CA , and Quinn, TC . The role of Chlamydia Pneumoniae in cardiovascular disease. Adv Intern Med. (2000) 45:139–73.
457. Kuo, CC , Grayston, JT , Campbell, LA , Goo, YA , Wissler, RW , and Benditt, EP . Chlamydia Pneumoniae (TWAR) in coronary arteries of young adults (15-34 years old). Proc Natl Acad Sci U S A. (1995) 92:6911–4. doi: 10.1073/pnas.92.15.6911
458. Kol, A , Bourcier, T , Lichtman, AH , and Libby, P . Chlamydial and human heat shock protein 60s activate human vascular endothelium, smooth muscle cells, and macrophages. J Clin Invest. (1999) 103:571–7. doi: 10.1172/JCI5310
459. Krull, M , Klucken, AC , Wuppermann, FN , Fuhrmann, O , Magerl, C , Seybold, J, et al. Signal transduction pathways activated in endothelial cells following infection with Chlamydia Pneumoniae. J Immunol. (1999) 162:4834–41. doi: 10.4049/jimmunol.162.8.4834
460. Smeeth, L , Thomas, SL , Hall, AJ , Hubbard, R , Farrington, P , and Vallance, P . Risk of myocardial infarction and stroke after acute infection or vaccination. N Engl J Med. (2004) 351:2611–8. doi: 10.1056/NEJMoa041747
461. Davidson, JA , Banerjee, A , Smeeth, L , McDonald, HI , Grint, D , Herrett, E, et al. Risk of acute respiratory infection and acute cardiovascular events following acute respiratory infection among adults with increased cardiovascular risk in England between 2008 and 2018: a retrospective. Populat Based Cohort Study Lancet Digit Health. (2021) 3:e773–83. doi: 10.1016/s2589-7500(21)00203-x
462. Fernandez, DM , Rahman, AH , Fernandez, NF , Chudnovskiy, A , Amir, ED , Amadori, L, et al. Single-cell immune landscape of human atherosclerotic plaques. Nat Med. (2019) 25:1576–88. doi: 10.1038/s41591-019-0590-4
463. Varricchi, G , Marone, G , and Kovanen, PT . Cardiac mast cells: underappreciated immune cells in cardiovascular homeostasis and disease. Trends Immunol. (2020) 41:734–46. doi: 10.1016/j.it.2020.06.006
464. Kovanen, PT . Mast cells as potential accelerators of human atherosclerosis-from early to late lesions. Int J Mol Sci. (2019) 20:4479. doi: 10.3390/ijms20184479
465. Kovanen, PT , and Bot, I . Mast cells in atherosclerotic cardiovascular disease-activators and actions. Eur J Pharmacol. (2017) 816:37–46. doi: 10.1016/j.ejphar.2017.10.013
466. Laine, P , Kaartinen, M , Penttilä, A , Panula, P , Paavonen, T , and Kovanen, PT . Association between myocardial infarction and the mast cells in the adventitia of the infarct-related coronary artery. Circulation. (1999) 99:361–9. doi: 10.1161/01.CIR.99.3.361
467. Laine, P , Naukkarinen, A , Heikkilä, L , Penttilä, A , and Kovanen, PT . Adventitial mast cells connect with sensory nerve fibers in atherosclerotic coronary arteries. Circulation. (2000) 101:1665–9. doi: 10.1161/01.CIR.101.14.1665
468. Bot, I , van der Velden, D , Bouwman, M , Kröner, MJ , Kuiper, J , Quax, PHA, et al. Local mast cell activation promotes neovascularization. Cells. (2020) 9:701. doi: 10.3390/cells9030701
469. Willems, S , Vink, A , Bot, I , Quax, PHA , de Borst, GJ , de Vries, J-PPM, et al. Mast cells in human carotid atherosclerotic plaques are associated with Intraplaque microvessel density and the occurrence of future cardiovascular events. Eur Heart J. (2013) 34:3699–706. doi: 10.1093/eurheartj/eht186
470. Camaré, C , Pucelle, M , Nègre-Salvayre, A , and Salvayre, R . Angiogenesis in the atherosclerotic plaque. Redox Biol. (2017) 12:18–34. doi: 10.1016/j.redox.2017.01.007
471. Kamat, BR , Galli, SJ , Barger, AC , Lainey, LL , and Silverman, KJ . Neovascularization and coronary atherosclerotic plaque: cinematographic localization and quantitative histologic analysis. Hum Pathol. (1987) 18:1036–42. doi: 10.1016/S0046-8177(87)80220-4
472. Grammas, P . Neurovascular dysfunction, inflammation and endothelial activation: implications for the pathogenesis of Alzheimer's disease. J Neuroinflammation. (2011) 8:26. doi: 10.1186/1742-2094-8-26
473. Springer, JM , Raveendran, VV , Gierer, SA , Maz, M , and Dileepan, KN . Protective role of mast cells in primary systemic Vasculitis: a perspective. Front Immunol. (2017) 8:990. doi: 10.3389/fimmu.2017.00990
474. Li, H , Yang, T , Ning, Q , Li, F , Chen, T , Yao, Y, et al. Cigarette smoke extract-treated mast cells promote alveolar macrophage infiltration and polarization in experimental chronic obstructive pulmonary disease. Inhal Toxicol. (2015) 27:822–31. doi: 10.3109/08958378.2015.1116644
475. Givi, ME , Blokhuis, BR , Da Silva, CA , Adcock, I , Garssen, J , Folkerts, G, et al. Cigarette smoke suppresses the surface expression of C-kit and FcepsilonRI on mast cells. Mediat Inflamm. (2013) 2013:813091. doi: 10.1155/2013/813091
476. Arcavi, L , and Benowitz, NL . Cigarette smoking and infection. Arch Intern Med. (2004) 164:2206–16. doi: 10.1001/archinte.164.20.2206
477. Bagaitkar, J , Demuth, DR , and Scott, DA . Tobacco use increases susceptibility to bacterial infection. Tob Induc Dis. (2008) 4:12. doi: 10.1186/1617-9625-4-12
478. Carter, BD , Freedman, ND , and Jacobs, EJ . Smoking and mortality--beyond established causes. N Engl J Med. (2015) 372:2170. doi: 10.1056/NEJMc1503675
479. Marshall, JS , Portales-Cervantes, L , and Leong, E . Mast cell responses to viruses and pathogen products. Int J Mol Sci. (2019) 20:4241. doi: 10.3390/ijms20174241
480. Barua, RS , Sharma, M , and Dileepan, KN . Cigarette smoke amplifies inflammatory response and atherosclerosis progression through activation of the H1R-TLR2/4-COX2 Axis. Front Immunol. (2015) 6:572. doi: 10.3389/fimmu.2015.00572
481. Rathore, AP , and St John, AL . Protective and pathogenic roles for mast cells during viral infections. Curr Opin Immunol. (2020) 66:74–81. doi: 10.1016/j.coi.2020.05.003
482. Graham, AC , Hilmer, KM , Zickovich, JM , and Obar, JJ . Inflammatory response of mast cells during influenza a virus infection is mediated by active infection and RIG-I signaling. J Immunol. (2013) 190:4676–84. doi: 10.4049/jimmunol.1202096
483. Song, ST , Wu, ML , Zhang, HJ , Su, X , and Wang, JH . Mast cell activation triggered by retrovirus promotes acute viral infection. Front Microbiol. (2022) 13:798660. doi: 10.3389/fmicb.2022.798660
484. Motta Junior, JDS , Miggiolaro, A , Nagashima, S , de Paula, CBV , Baena, CP , Scharfstein, J, et al. Mast cells in alveolar septa of COVID-19 patients: a pathogenic pathway that may link interstitial edema to immunothrombosis. Front Immunol. (2020) 11:574862. doi: 10.3389/fimmu.2020.574862
485. Wu, ML , Liu, FL , Sun, J , Li, X , He, XY , Zheng, HY, et al. SARS-CoV-2-triggered mast cell rapid degranulation induces alveolar epithelial inflammation and lung injury. Signal Transduct Target Ther. (2021) 6:428. doi: 10.1038/s41392-021-00849-0
486. Scialo, F , Daniele, A , Amato, F , Pastore, L , Matera, MG , Cazzola, M, et al. ACE2: the major cell entry receptor for SARS-CoV-2. Lung. (2020) 198:867–77. doi: 10.1007/s00408-020-00408-4
487. Kim, HY , Kang, HG , Kim, HM , and Jeong, HJ . Expression of SARS-CoV-2 receptor angiotensin-converting enzyme 2 by activating protein-1 in human mast cells. Cell Immunol. (2023) 386:104705. doi: 10.1016/j.cellimm.2023.104705
488. Raghavan, S , and Leo, MD . Histamine potentiates SARS-CoV-2 spike protein entry into endothelial cells. Front Pharmacol. (2022) 13:872736. doi: 10.3389/fphar.2022.872736
489. Gebremeskel, S , Schanin, J , Coyle, KM , Butuci, M , Luu, T , Brock, EC, et al. Mast cell and eosinophil activation are associated with COVID-19 and TLR-mediated viral inflammation: implications for an anti-Siglec-8 antibody. Front Immunol. (2021) 12:650331. doi: 10.3389/fimmu.2021.650331
490. Gioia, M , Ciaccio, C , Calligari, P , De Simone, G , Sbardella, D , Tundo, G, et al. Role of proteolytic enzymes in the COVID-19 infection and promising therapeutic approaches. Biochem Pharmacol. (2020) 182:114225. doi: 10.1016/j.bcp.2020.114225
491. Krysko, O , Bourne, JH , Kondakova, E , Galova, EA , Whitworth, K , Newby, ML, et al. Severity of SARS-CoV-2 infection is associated with high numbers of alveolar mast cells and their degranulation. Front Immunol. (2022) 13:968981. doi: 10.3389/fimmu.2022.968981
492. Karimi, N , Morovati, S , Chan, L , Napoleoni, C , Mehrani, Y , Bridle, BW, et al. Mast cell tryptase and implications for SARS-CoV-2 pathogenesis. Bio Med. (2021) 1:136–49. doi: 10.3390/biomed1020013
493. Kounis, NG , Koniari, I , de Gregorio, C , Velissaris, D , Petalas, K , Brinia, A, et al. Allergic reactions to current available COVID-19 vaccinations: pathophysiology, causality, and therapeutic considerations. Vaccines (Basel). (2021) 9:221. doi: 10.3390/vaccines9030221
494. Bagher, M , Larsson-Callerfelt, AK , Rosmark, O , Hallgren, O , Bjermer, L , and Westergren-Thorsson, G . Mast cells and mast cell tryptase enhance migration of human lung fibroblasts through protease-activated receptor 2. Cell Commun Signal. (2018) 16:59. doi: 10.1186/s12964-018-0269-3
495. Akers, IA , Parsons, M , Hill, MR , Hollenberg, MD , Sanjar, S , Laurent, GJ, et al. Mast cell tryptase stimulates human lung fibroblast proliferation via protease-activated receptor-2. Am J Physiol Lung Cell Mol Physiol. (2000) 278:L193–201. doi: 10.1152/ajplung.2000.278.1.L193
496. Carolina, DA-M , Couto, AES , Campos, LCB , Vasconcelos, TF , Michelon-Barbosa, J , Corsi, CAC, et al. MMP-2 and MMP-9 levels in plasma are altered and associated with mortality in COVID-19 patients. Biomed Pharmacother. (2021) 142:112067. doi: 10.1016/j.biopha.2021.112067
497. Benlarbi, M , Laroche, G , Fink, C , Fu, K , Mulloy, RP , Phan, A, et al. Identification of a SARS-CoV-2 host metalloproteinase-dependent entry pathway differentially used by SARS-CoV-2 and variants of concern alpha, Delta, and omicron. bioRxiv. (2022). doi: 10.1101/2022.02.19.481107
498. Gutman, H , Aftalion, M , Melamed, S , Politi, B , Nevo, R , Havusha-Laufer, S, et al. Matrix metalloproteinases expression is associated with SARS-CoV-2-induced lung pathology and extracellular-matrix remodeling in K18-HACE2 mice. Viruses. (2022) 14:1627. doi: 10.3390/v14081627
499. Naveed, SU , Clements, D , Jackson, DJ , Philp, C , Billington, CK , Soomro, I, et al. Matrix Metalloproteinase-1 activation contributes to airway smooth muscle growth and asthma severity. Am J Respir Crit Care Med. (2017) 195:1000–9. doi: 10.1164/rccm.201604-0822OC
500. Lei, Y , Zhang, J , Schiavon, CR , He, M , Chen, L , Shen, H, et al. SARS-CoV-2 spike protein impairs endothelial function via downregulation of ACE2. Circ Res. (2021) 128:1323–6. doi: 10.1161/CIRCRESAHA.121.318902
501. Weinstock, LB , Brook, JB , Walters, AS , Goris, A , Afrin, LB , and Molderings, GJ . Mast cell activation symptoms are prevalent in long-COVID. Int J Infect Dis. (2021) 112:217–26. doi: 10.1016/j.ijid.2021.09.043
502. Batiha, GE , Al-Kuraishy, HM , Al-Gareeb, AI , and Welson, NN . Pathophysiology of post-COVID syndromes: a new perspective. Virol J. (2022) 19:158. doi: 10.1186/s12985-022-01891-2
503. Grigorev, IP , and Korzhevskii, DE . Mast cells in the vertebrate brain: localization and functions. J Evol Biochem Physiol. (2021) 57:16–32. doi: 10.1134/S0022093021010026
504. Silver, R , Silverman, AJ , Vitković, L , and Lederhendler, II . Mast cells in the brain: evidence and functional significance. Trends Neurosci. (1996) 19:25–31. doi: 10.1016/0166-2236(96)81863-7
505. Florenzano, F , and Bentivoglio, M . Degranulation, density, and distribution of mast cells in the rat thalamus: a light and Electron microscopic study in basal conditions and after Intracerebroventricular Administration of Nerve Growth Factor. J Comp Neurol. (2000) 424:651–69. doi: 10.1002/1096-9861(20000904)424:4<651::AID-CNE7>3.0.CO;2-G
506. Khalil, M , Ronda, J , Weintraub, M , Jain, K , Silver, R , and Silverman, AJ . Brain mast cell relationship to Neurovasculature during development. Brain Res. (2007) 1171:18–29. doi: 10.1016/j.brainres.2007.07.034
507. Porzionato, A , Macchi, V , Parenti, A , and De Caro, R . The distribution of mast cells in the human area Postrema. J Anat. (2004) 204:141–7. doi: 10.1111/j.1469-7580.2004.00256.x
508. Ribatti, D . The crucial role of mast cells in blood-brain barrier alterations. Exp Cell Res. (2015) 338:119–25. doi: 10.1016/j.yexcr.2015.05.013
509. Nautiyal, KM , Liu, C , Dong, X , and Silver, R . Blood-borne donor mast cell precursors migrate to mast cell-rich brain regions in the adult mouse. J Neuroimmunol. (2011) 240-241:142–6. doi: 10.1016/j.jneuroim.2011.09.003
510. Bañuelos-Cabrera, I , Valle-Dorado, MG , Aldana, BI , Orozco-Suárez, SA , and Rocha, L . Role of histaminergic system in blood-brain barrier dysfunction associated with neurological disorders. Arch Med Res. (2014) 45:677–86. doi: 10.1016/j.arcmed.2014.11.010
511. Dong, H , Zhang, X , and Qian, Y . Mast cells and Neuroinflammation. Med Sci Monit Basic Res. (2014) 20:200–6. doi: 10.12659/MSMBR.893093
512. Lambracht-Hall, M , Dimitriadou, V , and Theoharides, TC . Migration of mast cells in the developing rat brain. Brain Res Dev Brain Res. (1990) 56:151–9. doi: 10.1016/0165-3806(90)90077-C
513. Silverman, AJ , Sutherland, AK , Wilhelm, M , and Silver, R . Mast cells migrate from blood to brain. J Neurosci. (2000) 20:401–8. doi: 10.1523/JNEUROSCI.20-01-00401.2000
514. Hough, LB . Cellular localization and possible functions for brain histamine: recent Progress. Prog Neurobiol. (1988) 30:469–505. doi: 10.1016/0301-0082(88)90032-9
515. Tsai, M , Grimbaldeston, M , and Galli, SJ . Mast cells and immunoregulation/immunomodulation. Adv Exp Med Biol. (2011) 716:186–211. doi: 10.1007/978-1-4419-9533-9_11
516. Kempuraj, D , Mentor, S , Thangavel, R , Ahmed, ME , Selvakumar, GP , Raikwar, SP, et al. Mast cells in stress, pain, blood-brain barrier, Neuroinflammation and Alzheimer's disease. Front Cell Neurosci. (2019) 13:54. doi: 10.3389/fncel.2019.00054
517. Jones, MK , Nair, A , and Gupta, M . Mast cells in neurodegenerative disease. Front Cell Neurosci. (2019) 13:171. doi: 10.3389/fncel.2019.00171
518. Skaper, SD , Giusti, P , and Facci, L . Microglia and mast cells: two tracks on the road to Neuroinflammation. FASEB J. (2012) 26:3103–17. doi: 10.1096/fj.11-197194
519. Burnstock, G , Krugel, U , Abbracchio, MP , and Illes, P . Purinergic signalling: from normal behaviour to pathological brain function. Prog Neurobiol. (2011) 95:229–74. doi: 10.1016/j.pneurobio.2011.08.006
520. Chakraborty, S , Kaushik, DK , Gupta, M , and Basu, A . Inflammasome signaling at the heart of central nervous system pathology. J Neurosci Res. (2010) 88:1615–31. doi: 10.1002/jnr.22343
521. Bulanova, E , and Bulfone-Paus, S . P2 receptor-mediated signaling in mast cell biology. Purinergic Signal. (2010) 6:3–17. doi: 10.1007/s11302-009-9173-z
522. Ribes, S , Adam, N , Ebert, S , Regen, T , Bunkowski, S , Hanisch, UK, et al. The viral TLR3 agonist poly (I: C) stimulates phagocytosis and intracellular killing of Escherichia Coli by microglial cells. Neurosci Lett. (2010) 482:17–20. doi: 10.1016/j.neulet.2010.06.078
523. Skuljec, J , Sun, H , Pul, R , Benardais, K , Ragancokova, D , Moharregh-Khiabani, D, et al. CCL5 induces a pro-inflammatory profile in microglia in vitro. Cell Immunol. (2011) 270:164–71. doi: 10.1016/j.cellimm.2011.05.001
524. Zhang, X , Wang, Y , Dong, H , Xu, Y , and Zhang, S . Induction of microglial activation by mediators released from mast cells. Cell Physiol Biochem. (2016) 38:1520–31. doi: 10.1159/000443093
525. Wang, Y , Huang, J , Li, Y , and Yang, GY . Roles of chemokine CXCL12 and its receptors in ischemic stroke. Curr Drug Targets. (2012) 13:166–72. doi: 10.2174/138945012799201603
526. Gasque, P , Singhrao, SK , Neal, JW , Gotze, O , and Morgan, BP . Expression of the receptor for complement C5a (CD88) is up-regulated on reactive astrocytes, microglia, and endothelial cells in the inflamed human central nervous system. Am J Pathol. (1997) 150:31–41.
527. Dong, H , Wang, Y , Zhang, X , Zhang, X , Qian, Y , Ding, H, et al. Stabilization of brain mast cells alleviates LPS-induced neuroinflammation by inhibiting microglia activation. Front Cell Neurosci. (2019) 13:191. doi: 10.3389/fncel.2019.00191
528. Rocha, SM , Saraiva, T , Cristovao, AC , Ferreira, R , Santos, T , Esteves, M, et al. Histamine induces microglia activation and dopaminergic neuronal toxicity via H1 receptor activation. J Neuroinflammation. (2016) 13:137. doi: 10.1186/s12974-016-0600-0
529. Barata-Antunes, S , Cristovao, AC , Pires, J , Rocha, SM , and Bernardino, L . Dual role of histamine on microglia-induced neurodegeneration. Biochim Biophys Acta Mol basis Dis. (2017) 1863:764–9. doi: 10.1016/j.bbadis.2016.12.016
530. Hendriksen, E , van Bergeijk, D , Oosting, RS , and Redegeld, FA . Mast cells in Neuroinflammation and brain disorders. Neurosci Biobehav Rev. (2017) 79:119–33. doi: 10.1016/j.neubiorev.2017.05.001
531. Abraham, SN , and St John, AL . Mast cell-orchestrated immunity to pathogens. Nat Rev Immunol. (2010) 10:440–52. doi: 10.1038/nri2782
533. Ramos-Cejudo, J , Wisniewski, T , Marmar, C , Zetterberg, H , Blennow, K , de Leon, MJ, et al. Traumatic brain injury and Alzheimer's disease: the cerebrovascular link. EBioMedicine. (2018) 28:21–30. doi: 10.1016/j.ebiom.2018.01.021
534. Gu, D , Ou, S , and Liu, G . Traumatic brain injury and risk of dementia and Alzheimer's disease: a systematic review and meta-analysis. Neuroepidemiology. (2022) 56:4–16. doi: 10.1159/000520966
535. Johnson, VE , Stewart, W , and Smith, DH . Widespread Τ and amyloid-Β pathology many years after a single traumatic brain injury in humans. Brain Pathol. (2012) 22:142–9. doi: 10.1111/j.1750-3639.2011.00513.x
536. Kokiko-Cochran, O , Ransohoff, L , Veenstra, M , Lee, S , Saber, M , Sikora, M, et al. Altered Neuroinflammation and behavior after traumatic brain injury in a mouse model of Alzheimer's disease. J Neurotrauma. (2016) 33:625–40. doi: 10.1089/neu.2015.3970
537. Moretti, R , Chhor, V , Bettati, D , Banino, E , De Lucia, S , Le Charpentier, T, et al. Contribution of mast cells to injury mechanisms in a mouse model of pediatric traumatic brain injury. J Neurosci Res. (2016) 94:1546–60. doi: 10.1002/jnr.23911
538. Lozada, A , Maegele, M , Stark, H , Neugebauer, EM , and Panula, P . Traumatic brain injury results in mast cell increase and changes in regulation of central histamine receptors. Neuropathol Appl Neurobiol. (2005) 31:150–62. doi: 10.1111/j.1365-2990.2004.00622.x
539. Levy, D , Edut, S , Baraz-Goldstein, R , Rubovitch, V , Defrin, R , Bree, D, et al. Responses of Dural mast cells in concussive and blast models of mild traumatic brain injury in mice: potential implications for post-traumatic headache. Cephalalgia. (2016) 36:915–23. doi: 10.1177/0333102415617412
540. Biran, V , Cochois, V , Karroubi, A , Arrang, JM , Charriaut-Marlangue, C , and Héron, A . Stroke induces histamine accumulation and mast cell degranulation in the neonatal rat brain. Brain Pathol. (2008) 18:1–9. doi: 10.1111/j.1750-3639.2007.00092.x
541. Ziemka-Nalecz, M , Jaworska, J , and Zalewska, T . Insights into the Neuroinflammatory responses after neonatal hypoxia-ischemia. J Neuropathol Exp Neurol. (2017) 76:644–54. doi: 10.1093/jnen/nlx046
542. Schilling, L , and Wahl, M . Opening of the blood-brain barrier during cortical Superfusion with histamine. Brain Res. (1994) 653:289–96. doi: 10.1016/0006-8993(94)90403-0
543. Shelestak, J , Singhal, N , Frankle, L , Tomor, R , Sternbach, S , McDonough, J, et al. Increased blood-brain barrier hyperpermeability coincides with mast cell activation early under Cuprizone administration. PLoS One. (2020) 15:e0234001. doi: 10.1371/journal.pone.0234001
544. Berghoff, SA , Düking, T , Spieth, L , Winchenbach, J , Stumpf, SK , Gerndt, N, et al. Blood-brain barrier hyperpermeability precedes demyelination in the Cuprizone model. Acta Neuropathol Commun. (2017) 5:94. doi: 10.1186/s40478-017-0497-6
545. McKittrick, CM , Lawrence, CE , and Carswell, HV . Mast cells promote blood brain barrier breakdown and neutrophil infiltration in a mouse model of focal cerebral ischemia. J Cereb Blood Flow Metab. (2015) 35:638–47. doi: 10.1038/jcbfm.2014.239
546. Abbott, NJ . Astrocyte-endothelial interactions and blood-brain barrier permeability. J Anat. (2002) 200:629–38. doi: 10.1046/j.1469-7580.2002.00064.x
547. Strbian, D , Kovanen, PT , Karjalainen-Lindsberg, ML , Tatlisumak, T , and Lindsberg, PJ . An emerging role of mast cells in cerebral ischemia and hemorrhage. Ann Med. (2009) 41:438–50. doi: 10.1080/07853890902887303
548. Akiyama, H , Barger, S , Barnum, S , Bradt, B , Bauer, J , Cole, GM, et al. Inflammation and Alzheimer's disease. Neurobiol Aging. (2000) 21:383–421. doi: 10.1016/S0197-4580(00)00124-X
549. Park, JC , Han, SH , and Mook-Jung, I . Peripheral inflammatory biomarkers in Alzheimer's disease: a brief review. BMB Rep. (2020) 53:10–9. doi: 10.5483/BMBRep.2020.53.1.309
550. Lassmann, H . Neuroinflammation: 2021 Update. Free Neuropathol. (2021):2. doi: 10.17879/freeneuropathology-2021-3166
551. Maslinska, D , Laure-Kamionowska, M , Maslinski, KT , Gujski, M , and Maslinski, S . Distribution of tryptase-containing mast cells and metallothionein reactive astrocytes in human brains with amyloid deposits. Inflamm Res. (2007) 56:S17–8. doi: 10.1007/s00011-006-0508-8
552. Skaper, SD , Facci, L , Zusso, M , and Giusti, P . An inflammation-centric view of neurological disease: beyond the neuron. Front Cell Neurosci. (2018) 12:72. doi: 10.3389/fncel.2018.00072
553. Shaik-Dasthagirisaheb, YB , and Conti, P . The role of mast cells in Alzheimer's disease. Adv Clin Exp Med. (2016) 25:781–7. doi: 10.17219/acem/61914
554. Harcha, PA , Vargas, A , Yi, C , Koulakoff, AA , Giaume, C , and Sáez, JC . Hemichannels are required for amyloid Β-peptide-induced degranulation and are activated in brain mast cells of APPswe/PS1dE9 mice. J Neurosci. (2015) 35:9526–38. doi: 10.1523/JNEUROSCI.3686-14.2015
555. Piette, F , Belmin, J , Vincent, H , Schmidt, N , Pariel, S , Verny, M, et al. Masitinib as an adjunct therapy for mild-to-moderate Alzheimer's disease: a randomised, placebo-controlled phase 2 trial. Alzheimers Res Ther. (2011) 3:16. doi: 10.1186/alzrt75
556. Folch, J , Petrov, D , Ettcheto, M , Abad, S , Sánchez-López, E , García, ML, et al. Current research therapeutic strategies for Alzheimer's disease treatment. Neural Plast. (2016) 2016:8501693. doi: 10.1155/2016/8501693
557. Shanahan, L , Zucker, N , Copeland, WE , Costello, EJ , and Angold, A . Are children and adolescents with food allergies at increased risk for psychopathology? J Psychosom Res. (2014) 77:468–73. doi: 10.1016/j.jpsychores.2014.10.005
558. Topal, E , Catal, F , Soylu, N , Ozcan, OO , Celiksoy, MH , Babayiğit, A, et al. Psychiatric disorders and symptoms severity in pre-school children with Cow's Milk allergy. Allergol Immunopathol (Madr). (2016) 44:445–9. doi: 10.1016/j.aller.2016.03.001
559. Hayashi, T , Cottam, HB , Chan, M , Jin, G , Tawatao, RI , Crain, B, et al. Mast cell-dependent anorexia and hypothermia induced by mucosal activation of toll-like receptor 7. Am J Physiol Regul Integr Comp Physiol. (2008) 295:R123–32. doi: 10.1152/ajpregu.00527.2007
560. Shaker, M , Yuan, I , Kennedy, KL , Capucilli, P , and Spergel, JM . Idiopathic anaphylaxis and undiagnosed anorexia nervosa. Ann Allergy Asthma Immunol. (2019) 122:215–7. doi: 10.1016/j.anai.2018.10.017
561. Costa-Pinto, FA , and Basso, AS . Neural and behavioral correlates of food allergy. Chem Immunol Allergy. (2012) 98:222–39. doi: 10.1159/000336525
562. Moura, DS , Georgin-Lavialle, S , Gaillard, R , and Hermine, O . Neuropsychological features of adult Mastocytosis. Immunol Allergy Clin N Am. (2014) 34:407–22. doi: 10.1016/j.iac.2014.02.001
Keywords: cardiovascular disease, neuroinflammation, cigarette smoking, bacterial infection, SARS-CoV-2 disease, atherosclerosis, allergic disease, skin and wound-healing
Citation: Dileepan KN, Raveendran VV, Sharma R, Abraham H, Barua R, Singh V, Sharma R and Sharma M (2023) Mast cell-mediated immune regulation in health and disease. Front. Med. 10:1213320. doi: 10.3389/fmed.2023.1213320
Edited by:
Stefano Bacci, University of Florence, ItalyReviewed by:
Gregorio Gomez, University of Houston, United StatesDaniel Elieh-Ali-Komi, Charité University Medicine Berlin, Germany
Copyright © 2023 Dileepan, Raveendran, Sharma, Abraham, Barua, Singh, Sharma and Sharma. This is an open-access article distributed under the terms of the Creative Commons Attribution License (CC BY). The use, distribution or reproduction in other forums is permitted, provided the original author(s) and the copyright owner(s) are credited and that the original publication in this journal is cited, in accordance with accepted academic practice. No use, distribution or reproduction is permitted which does not comply with these terms.
*Correspondence: Kottarappat N. Dileepan, kdileepan@kumc.edu; Mukut Sharma, Mukut.Sharma@va.gov
†Present address: Vineesh V. Raveendran, Department of Cell Biology, King Faisal Specialist Hospital and Research Centre, Riyadh, Saudi Arabia