- 1Microbiota Research Group, Endocrinology and Metabolism Research Center, Endocrinology and Metabolism Clinical Sciences Institute, Tehran University of Medical Sciences, Tehran, Iran
- 2Faculty of Medicine, Qeshm International Medical Sciences Branch, Islamic Azad University, Qeshm, Iran
- 3Young Researchers and Elite Club, Qeshm International Medical Sciences Branch, Islamic Azad University, Qeshm, Iran
- 4Obesity and Eating Habits Research Center, Endocrinology and Metabolism Clinical Sciences Institute, Tehran University of Medical Sciences, Tehran, Iran
- 5Endocrinology and Metabolism Research Center, Endocrinology and Metabolism Clinical Sciences Institute, Tehran University of Medical Sciences, Tehran, Iran
Objectives: Gut dysbiosis is believed to be one of the several mechanisms that are involved in the pathogenesis of gout. This systematic review aimed to summarize the role of gut dysbiosis in gout disease and uncover the underlying mechanisms.
Methods: A comprehensive search was conducted on PubMed, Web of Science, and Scopus databases up to October 2021. Animal studies and human observational studies, including case-control, cross-sectional, and cohort studies assessing the association between gut microbiota composition and gout were included. The quality of included studies has been evaluated using the Newcastle–Ottawa Quality Assessment scale (NOS) and the SYRCLE's risk of bias tool.
Results: Initially, we found 274 studies among which 15 studies were included in this systematic review. Of them, 10 studies were conducted on humans and 5 studies were conducted on animals. Increased abundance of Alistipes and decreased abundance of Enterobacteriaceae alters purine metabolism, thereby aggravating gout condition. Moreover, a higher abundance of Phascolarctobacterium and Bacteroides in gout modulates enzymatic activity in purine metabolism. Butyrate-producing bacteria such as Faecalibacterium, prausnitzii, Oscillibacter, Butyricicoccus, and Bifidobacterium have higher abundance in healthy controls compared to gout patients, suggesting the anti-inflammatory and anti-microbial role of short-chain fatty acids (SCFAs). Lipopolysaccharides (LPS)-releasing bacteria, such as Enterobacteriaceae, Prevotella, and Bacteroides, are also involved in the pathogenesis of gout disease by stimulating the innate immune system.
Conclusion: Exploring the role of gut dysbiosis in gout and the underlying mechanisms can help develop microbiota-modulating therapies for gout.
Introduction
Gout is an inflammatory arthritis disease caused by purine metabolism disorder and characterized by elevated levels of serum uric acid (SU) and deposition of monosodium urate (MSU) in and around the joints (1–3). Due to the changes in diet and lifestyle, the prevalence and incidence of gout have gradually increased worldwide (1, 4, 5). Hyperuricemia (HUA) is a major risk factor for MSU crystal deposition and gout complications such as acute gouty arthritis, joint deformity, and uric acid nephropathy (6, 7). Both gout and HUA are critical risk factors for different metabolic diseases such as hypertension, chronic kidney disease (CKD), obesity, cardiovascular disease, stroke, and diabetes mellitus (5, 8, 9).
Gut microbiota refers to the community of microorganisms residing in human intestines. Gut microbiota plays crucial roles in the physiological functions of the gastrointestinal tract such as food digestion, anti-microbial defense, and metabolism (2, 10–13). Several metabolomics and metagenomics studies described the association between gut dysbiosis and gout to differentiate between gout patients and healthy individuals and provide a novel insight for disease treatment (8, 11).
HUA is associated with purine abnormal metabolism and decreased UA excretion. In a healthy person, about 70% of the UA excretion occurs in the kidney, whereas the remaining is excreted through the intestines and is metabolized by gut microbiota. Several studies have indicated that gut microbiota and their metabolites contribute to purine and UA metabolism (10, 14). However, to date, the mechanisms linking host purine and UA metabolism to gut microbiota are not completely determined. Therefore, recent studies have focused on the involvement of gut microbiota in hyperuricemia to uncover the mediating mechanisms linking gut dysbiosis to gout (1, 2, 7, 15).
Some studies revealed that the abundance of Bacteroides is remarkably increased in gout patients while the abundance of Faecalibacterium is decreased. These alterations in the composition of gut microbiota are the hallmark of gout disease and can accelerate disease progression (1, 11). Another study revealed that gut dysbiosis can alter intestinal immunity and increase bacterial penetration into the systemic circulation, thereby inducing a systemic inflammatory response and aggravating gout disease (16, 17). Moreover, studies have found that gut microbiota is an important target for treating HUA by enhancing purine and UA catabolism, increasing UA excretion, and modulating intestinal inflammatory response (8, 15, 18). In this systematic review, we aimed to provide a better insight into the association of gut dysbiosis with gout disease.
Methods
This systematic review was done according to the Preferred Reporting Items for Systematic Reviews and Meta-analysis (PRISMA) statement (19).
Search strategy
PubMed, Web of Science, and Scopus databases were searched for observational studies on the relationship between gout and changes in gut microbiota composition up to October 2021. The search terms were “gout” OR “hyperuricemia” OR (“arthritis” AND “gouty”) AND “microbiome” OR “microbiota” OR “dysbiosis” OR “gut microbial composition” OR “intestinal microbial composition” OR “Fecal microbial composition” OR “gut bacterial composition” OR “intestinal bacterial composition” OR “Fecal bacterial composition” OR “intestinal microflora” OR “gut microflora.” Furthermore, the reference list of related review articles in this field was screened. All retrieved articles were checked and duplicates were removed manually by two independent researchers.
Eligibility criteria and study selection
Initially, all documents were screened according to the titles and abstracts by two independent researchers. Thereafter, the full-text version of the articles was reviewed based on the inclusion and exclusion criteria. In case of any disagreements between the two researchers, they continued discussing until reaching a consensus.
Animal studies or human observational studies including case-control, cross-sectional, and cohort studies assessing the correlation between gut microbiota and gout were included.
Regarding “PECOS” of this systematic review:
Population (P): gout patients
Exposure (E): microbiota dysbiosis
Controls (C): healthy subjects
Outcomes (O): gout symptoms and disease intensity
Study design (S): observational
Reviews, case reports, experimental studies, interventional studies, protocols, conference papers, and letters to the editor were excluded.
Data extraction
Data were extracted from both human and animal studies by two independent researchers. For animal studies, authors' names, publication year, characteristics of animals (sample size, age, and sex), HUA induction method, microbiota and biochemical analysis method, and microbiota and biochemical changes in the HUA group were collected. For human observational studies, authors' names, country and year of publication, participants' characteristics (sample size and age), diagnostic tools for gout, medications, microbiota analysis method, main findings regarding microbiota profile, and biochemical changes were collected.
Quality assessment
The Newcastle–Ottawa Quality Assessment scale (NOS) has been used to evaluate the quality of cohort and case-control studies, and its modified version has been used to evaluate the quality of cross-sectional studies (20, 21). The maximum score was 9 for cohort and case-control studies and 7 for cross-sectional studies. The quality of studies was defined good if the studies got 3 or 4 stars in the selection domain AND 1 or 2 stars in the comparability domain AND 2 or 3 stars in the outcome/exposure domain. Fair quality was defined as 2 stars in the selection domain AND 1 or 2 stars in the comparability domain AND 2 or 3 stars in the outcome/exposure domain. Poor quality was defined as 0 or 1 star in the selection domain OR 0 stars in the comparability domain OR 0 or 1 stars in the outcome/exposure domain (20). Besides, the quality of animal studies was measured based on the SYRCLE's risk of bias tool (22).
All procedures including searching, study selection, data extracting, and quality assessment were conducted by two separate and independent researchers and they discussed the conflicting points until they reached a consensus.
Results
Primarily, we identified 274 studies, and 127 articles were removed because of duplication. Then, 147 studies were screened using their title/abstract and full text. Finally, 15 studies were included in this systematic review (Figure 1).
Human studies
Data from ten included articles are presented in Table 1. Among them, 8 were case-control (2–5, 8, 10, 11, 14), 1 was a cohort (1) and 1 was a cross-sectional study (24). Totally, there were 477 participants with gout disease and 494 participants as the control group. Henson et al. (5) Liu et al. (11) and Yang et al. (24) included both genders whereas Ning et al., Shao et al., and Xing et al. only included men (2, 3, 10). The gender of participants was not mentioned in other studies (1, 4, 8, 14). The average age of participants ranged from 28 to 75 years (1–5, 10, 11, 24). Two studies did not mention the age of participants (8, 14).
The 2015 version of ACR/EULAR classification criteria, hematologic examination, and clinical symptoms were used to recognize and verify gout disease. Five studies used 2015 ACR/EULAR classification criteria (3, 4, 8, 10, 14), and other studies used hematologic examination (1, 2, 8, 11, 24). In addition to hematologic examination, clinical symptoms were used for diagnosis in 4 studies (1, 2, 8, 11). Diagnostic tool was not defined in one study (5). In 7 studies, participants did not use any medications including antibiotics (2–4, 8, 10, 14, 24), while 3 studies did not mention whether their participants used anti-gout medications or antibiotics (1, 5, 11). All studies analyzed the diversity and composition of microbiota by 16s rRNA gene sequencing (1–3, 5, 8, 10, 11, 14, 24), except one study that used Metagenomic shotgun sequencing (4). Lin et al. also used both 16s rRNA gene sequencing and Metagenomic shotgun sequencing for analyzing microbiota composition and function (8). Four studies targeted the V3–V4 region of 16S rRNA genes (2, 3, 14, 24), whereas Guo et al. targeted the V1–V3 region of 16S rRNA genes (1).
Gut microbial composition in gout disease
Alterations of gut microbial profile in patients with gout disease are summarized in Table 1. In the study conducted by MéndezSalazar et al. richness indices were increased in the control group. At the genus level, Ruminococcus_1, Clostridium_sensu_stricto_1, Oscillibacter, Butyricicoccus, Ruminococcaceae_UCG_010, Bifidobacterium, Lachnospiraceae_ND3007_group, Haemophilus and Ruminococcaceae_UGC_013 were more abundant in the control group (14). Furthermore, Chu et al. exhibited higher abundance of phylum Bacteroidetes and Fusobacteria and lower abundance of phylum Proteobacteria (4) and Yang et al. revealed increased alpha diversity in the asymptomatic hyperuricemic group compared with controls. At genus level, unclassified_Ruminococcaceae, Alistipes, Dialister, unidentified_Ruminococcaceae, Roseburia, Gemmiger and Faecalibacterium had higher abundance in the asymptomatic hyperuricemic group, while unclassified_Enterobacteriaceae, Bifidobacterium, Klebsiella, Ruminococcus, unidentified_Lactobacillales, unclassified_Enterococcaceae, Eubacterium, unidentified_Enterobacteriaceae and Clostridium had lower abundance in the control group (24). Additionally, Lin et al. found that phylum Actinobacteria has higher abundance in healthy controls and Firmicutes has higher abundance in untreated patients than in healthy controls. They also reported that gerenra Fecalibacterium, Lachnospiraceae Clostridium, Roseburia, Cytophaga, Ruminococcaceae Clostridium, Alistipes, Pseudomonas, Butyricicoccus, Clostridiaceae Clostridium, Sporobacter, Campylobacter, Desulfotomaculum, Halomonas and Succinispira have higher abundance in untreated patients than in healthy controls and Millisia, Bifidobacterium, Paracoccus, Aeromonas, Enterococcus and Leifsonia have higher abundance in healthy controls than in untreated patients. Moreover, Ning et al. reported that ACE, Shannon, Chao1, Simpson, and observed-species indices decrease in gout patients. This study also revealed an increased abundance of Firmicutes and Actinobacteria and a decreased abundance of Proteobacteria at phylum level in gout patients. At genus level, the study showed higher numbers of Corynebacterium_1 and Prevotella and lower numbers of Novosphingobium, Derxia, Curvibacter, Methylobacterium, Caulobacter, Skermanella, unidentified, Chloroplast and Rikenellaceae_RC9_gut_group in gout patients (3). Furthermore, Shao et al. showed that Chao1, observed species, Simpson, and Shannon indices decrease in gout patients. In addition, this study exhibited the increased population of phylum Chloroflexi and Bacteroidetes, Class Erysipelotrichia, Negativicutes, Anaerolineae and Bacteroidia, Order Bacteroidales, Anaerolineales, Selenomonadales, Corynebacteriales and Erysipelotrichales and Family Nocardiaceae, Bacteroidacea, Anaerolineaceae, Porphyromonadaceae, Erysipelotrichaceae, Vibrionaceae in gout patients. At genus level, the study reported higher abundance of Rhodococcus and Erysipelatoclostridium and lower abundance of Photobacterium, Vibrio, Coprococcus 3, Lachnospiraceae NC2004 group, Lachnospiraceae UCG_005, Ruminococcaceae NK4A214 group and Ruminococcaceae UCG_011 in gout patients (2). Guo et al. found that Faecalibacterium prausnitzii, Bifidobacterium and pseudocatenulatum were more abundant in healthy individuals whereas Bacteroides caccae and Bacteroides xylanisolvens were more abundant in gout patients. At genus level, this study also reported higher abundance of Coprococcus, Faecalibacterium, Alistipes, Dialister, Robinsoniella, Subdoligranulum, Odoribacter, and Oscillibacter in healthy controls and higher abundance of Barnesiella, Parasporobacterium, Paraprevotella, Anaerotruncus, Pseudobutyrivibrio, Bacteroides, Holdemania, and Acetanaerobacterium in gout patients (1). Xing et al. performed diversity analysis and clustering analysis on genera Bacteroides and Clostridium. Compared with normal cases, the number of bands and Shannon–Weaver (H') of Clostridium but not Bacteroides significantly decreased in patients with primary gout. Furthermore, the intra-group and inter-group similarity of both Bacteroides and Clostridium were lower (10).
Except two studies, all other studies conducted biochemical analysis (8, 24). In the study conducted by Méndez-Salazar et al., the level of urea carboxylase and urease accessory protein were positively correlated in the control group. Compared with the control group, gout patients without tophi had higher levels of glycine reductase complex component B subunits alpha, beta, and gamma and glycine dehydrogenase subunit, while gout patients with tophi had higher levels of vitamin B12, nucleoside permease, xanthine phosphorylases, nucleoside phosphorylases, methionine transaminase, glycine cleavage system, transcriptional repressor, xanthine dehydrogenase, iron-sulfur-binding subunits, 5-hydroxyisourate hydrolase, (S)-ureidoglycine aminohydralase, and purine nucleosidase (14). Three studies reported higher levels of uric acid in gout patients (2, 4, 10). Besides, Chu et al. and Shao et al. studies observed higher levels of erythrocyte sedimentation rate and blood urea nitrogen in gout patients (1, 2, 4).
Quality assessment of the included human studies by the Newcastle–Ottawa Quality Assessment scale (20, 21) revealed that only 1 study had good quality (total score: 7) (10), 5 studies had fair quality (total core: 5–7) (1, 3, 8, 14, 24) and 4 studies had poor quality (2, 4, 5, 11) (Table 1).
Animal studies
Data from five animal studies are presented in Table 2. The age of animals ranged from 4 to 26 weeks (7, 15–17, 25). Target species were Wistar rats in one study (7), wild-type mice in two studies (16, 17), and Sprague Dawley rats in two studies (15, 25). Three studies used male animals (7, 15, 25), while the others did not mention the gender of the species (16, 17). HUA induction methods were different among studies. One study used 100 mg/Kg/day of yeast-rich forage and purine (7). Other studies used 10% yeast and 15% adenine diet (25) and a high-fat diet containing 10% yeast extract (15) while some studies did not mention the details (16, 17). These studies investigated the association between gut microbiota composition and gout disease (7, 15–17, 25). In all studies, microbiota diversity was analyzed by 16srRNA gene sequencing, using frozen fecal samples (7, 15–17, 25). Three studies targeted the V3–V4 region of 16S rRNA genes (7, 15, 25), while 2 studies targeted the V1–V3 region of 16S rRNA genes (16, 17).
Gut microbial composition in HUA animal models
At the genus level, studies exhibited an increase in the abundance of Parabacteroides and Bacteroides (7, 15, 16) and a decrease in the abundance of Prevotella (7, 15) and Ruminococcaceae in hyperuricemic animals (15, 25). Four studies showed conflicting results regarding Alloprevotella, Clostridium, Lactobacillus and Blautia (7, 15–17, 25). Liu et al. observed a lower abundance of Alloprevotella, while Lv et al. reported a higher abundance of this genus (7, 16). Additionally, Liu et al. and Xu et al. revealed a higher frequency of Clostridium, whereas Lv et al. and Yu et al. exhibited a lower abundance of this genus (7, 15–17). Lv et al. and Xu et al. also showed an increased number of Lactobacillus in hyperuricemic animals, while in Yu et al. reported a decreased abundance of Lactobacillus in hyperuricemic animals (15–17). Moreover, Pan et al. found a decreased abundance of Blautia and Ruminococcaceae when Liu et al. observed an increased abundance of Blautia. Xu et al. reported a higher abundance of Ruminococcaceae (7, 17, 25). At the phylum level, studies also reported controversial results regarding the abundance of Bacteroidetes and Firmicutes (15, 17, 25). Pan et al. reported a higher abundance of Bacteroidetes in hyperuricemic animals, while in Yu et al. reported a lower abundance of this phylum in hyperuricemic animals (15, 25). Furthermore, Xu et al. showed a lower number of Firmicutes, while Yu et al. reported a higher number of this phylum (15, 17).
Biochemical analysis was also performed in these studies (7, 15–17, 25). All studies used plasma samples for analysis of biochemical indicators except one study that assessed biochemical indicators in both plasma and urine (25). In 2 studies hematoxylin-eosin staining, immunohistochemical analysis, and enzyme-linked immunosorbent assay were performed (16, 17). All studies reported increased levels of uric acid in hyperuricemic animal models (7, 15–17, 25). Three studies reported higher levels of creatinine (7, 15, 25) and 4 studies reported increased levels of total cholesterol in animal models (7, 15–17). In 2 studies, blood urea nitrogen was increased in hyperuricemic mice (7, 16). Pan et al. also reported higher levels of plasma amino acids such as serine glutamate and glutamine. Urine analysis showed lower levels of phenol, p-cresol, p-hydroxypheny lacetic acid, and indol-5-ol in this study (25). An increased level of TNF-α was reported in 2 studies (16, 17). IL-1β did not significantly change in the study conducted by Xu et al. while it significantly increased in the study conducted by Lv et al. (16, 17).
SYRCLE's tool for assessing the risk of bias was used in animal studies (22) and showed that most of the studies had attrition bias, reporting bias, and weakness in sequencing generation in selection bias (Supplementary Table).
Discussion
Main findings
This systematic review summarizes gut dysbiosis in gout to illustrate possible correlations. From all studies, just 5 of them reported changes for alpha diversity and richness indices (2, 3, 7, 14, 24). Three studies showed lower richness among gout patients which can be related to intestinal dysbiosis and inflammation in gout (2, 3, 14). In contrast, 2 studies reported higher richness. These contradictions may be related to differences between humans and animals (7) or disease condition (asymptomatic hyperuricemia) (24). At the phylum level, Bacteroidetes abundance increased in three studies (2, 4, 15) and decreased in one study (25). Firmicutes abundance increased in three studies (3, 7, 8) and decreased in 2 studies (15, 17). Actinobacteria abundance decreased in one study (7) and increased in 3 studies (3, 8, 25). In addition, Actinobacteria abundance increased among healthy controls in one study (8). Proteobacteria abundance increased in two studies (14, 25) and decreased in 2 studies (3, 4). At the phylum level, there were contradictions and heterogeneities among the studies. Differences in characteristics of the population, severity of gout, and methodologies could be the reason for these contradictions. At the genus level, increased Oscillibacter (1, 14), Butyricicoccus (8, 14), and Dialister (1, 24) were observed in two studies. Increased abundance of Bacteroides among patients was reported in 5 studies (1, 7, 14–16) while Roseburia (8, 14, 24), and Alistipes abundance (8, 16, 24) were increased in 3 studies. Studies were contradictory regarding Faecalibacterium (11). Faecalibacterium was higher in AH (1, 24) contrary to its higher frequency identified in controls. In individuals with a higher abundance of Faecalibacterium, increased production of butyrate can partly prevent gout. In this study, healthy individuals had 230% higher abundance of Faecalibacterium and 550% higher production of butyrate compared with gout patients (11). Additionally, two studies revealed that at the genus level, Bifidobacterium was decreased in gout patients (8, 24).
Underlying mechanisms
Gout is associated with higher levels of uric acid in the blood, which is called hyperuricemia. Many mechanisms are involved in the pathogenesis of hyperuricemia one of which is gut dysbiosis. Accumulating evidence shows that purine metabolism plays a key role in gout by degrading purine to urea or uric acid (1). In gout patients, purine mostly degrades to uric acid causing hyperuricemia (10). Xanthine dehydrogenase degrades purine to uric acid which is highly expressed in gout patients leading to hyperuricemia. Increased abundance of Alistipes in AH and its possible effect on purine metabolism, hypothetically may upregulate xanthine dehydrogenase. Therefore, Alistipes can be involved in the pathogenesis of gout (1, 24). It is worth noting that Enterobacteriaceae acts like allantoinase, an enzyme in purine metabolism, which degrades uric acid to urea (1, 26). Allantoinase is upregulated in treated patients and downregulated in gout patients. Decreased abundance of Enterobacteriaceae in gout patients suggests a connection between Enterobacteriaceae and Allantoinase. Perhaps, changes in microbial composition can change enzyme levels, thereby ameliorating or aggravating gout. Moreover, Phascolarctobacterium and Bacteroides had higher abundance in gout patients and convert urate into allantoin. It is proposed that Phascolarctobacterium and Bacteroides are involved in gout through enzyme modulation (14, 27).
Acetate, propionate, and butyrate, are short-chain fatty acids (SCFAs) with substantial advantages for health (28). Butyrate possesses protective roles against gout through numerous mechanisms. Butyrate-producing bacteria such as Faecalibacterium prausnitzii, Oscillibacter, and Butyricicoccus are increased in healthy controls compared to gout patients, suggesting the possible role of SCFAs in gout (1, 14). SCFAs, especially butyrate, maintain the stability and integrity of the epithelial barrier by regulating the expression of tight junction proteins (TJP) such as claudin-1 and Zonula Occludens-1 (ZO-1) (29–31). SCFAs, particularly butyrate, have anti-inflammatory functions by downregulating inflammatory cytokines such as IL-1β, IL-6, and IL-8 or exerting a direct anti-inflammatory effect (Figure 2) (31–33). Moreover, butyrate is an energy source for cells, stimulates the proliferation of healthy cells, and promotes intestinal villus repair (1, 31, 34).
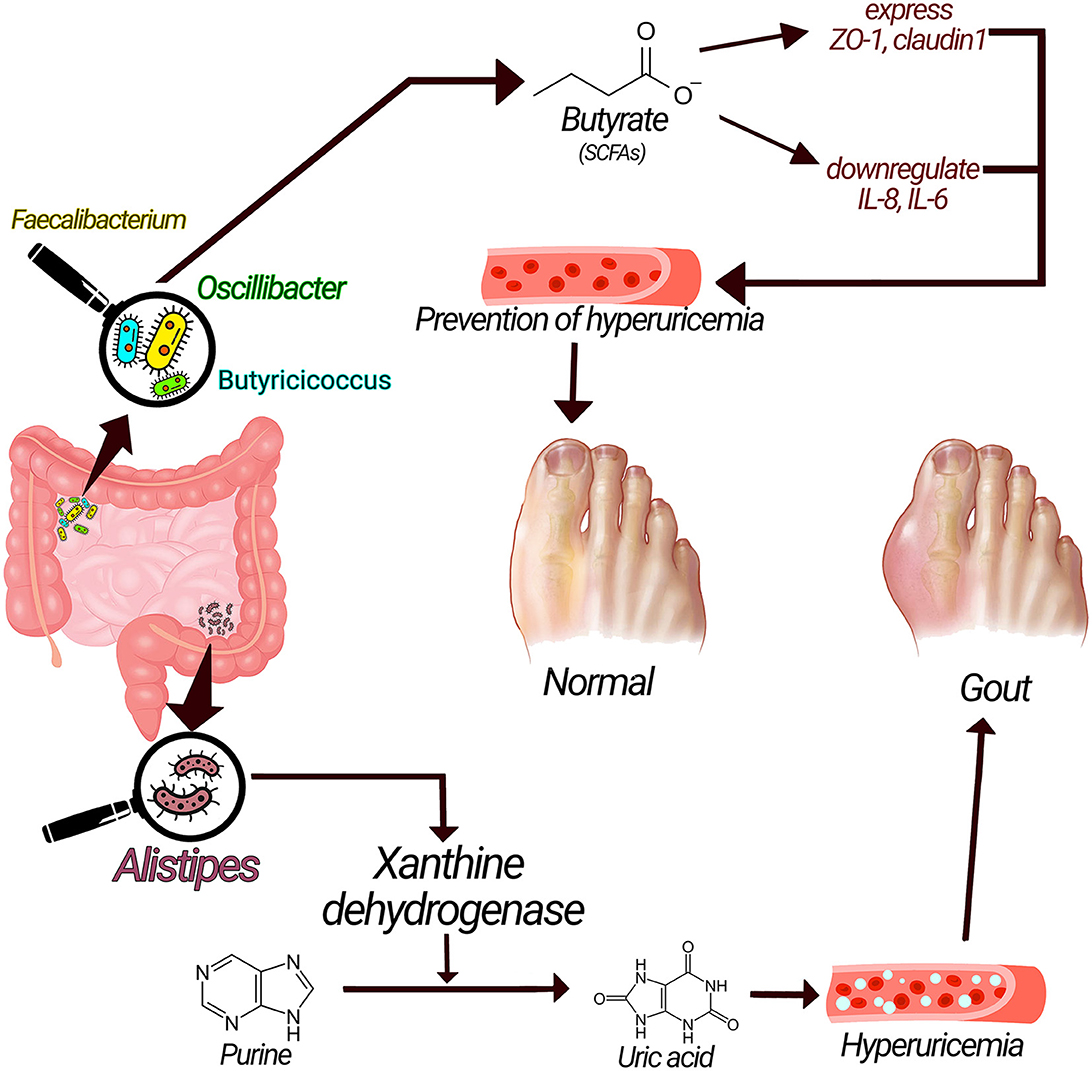
Figure 2. Underlying mechanisms related gut microbiota with gout pathogenesis. Xanthine dehydrogenase produced by alistipes could convert purine to uric acid and cause hyperuricemia and increase the risk of gout; on the other hand, SCFAs have anti-inflammatory effect via enhancing the expression of tight junction proteins and have preventive effects on gout.
At the genus level, Bifidobacterium showed a decreased abundance in gout patients (1, 8, 24, 35). We already know that Bifidobacterium has Several protective functions such as reinforcing immune response, acting as a biological barrier (24, 36, 37), preventing aging, and promoting gastrointestinal functionality (8, 38). Furthermore, Bifidobacterium can produce butyric acid which has many effects. Furthermore, Bifidobacterium can ameliorate constipation and prevent the growth of pathogens (24). The simultaneous presence of Bifidobacterium and butyrate-producing bacteria can attenuate inflammation and improve the function of the intestinal barrier (14).
Higher abundance of Bacteroides genus (1, 7, 14–16), Bacteroides caccae and Bacteroides xylanisolvens species (1), Prevotella genus and Prevotella intermedia species (3, 5) in gout patients (24) supports their role in gout via biosynthesis of LPS or lipid A. LPS is a stimulator of the innate immune system. LPS produced by some species can be transferred into the cytoplasm by interferon-inducible GTPases. The hexa-acylated lipid A component of LPS attaches to caspase 4, caspase 5, caspase 11, and non-canonical NLRP3 inflammasome and activates them (39). The structure of LPS is important in activating the immune system, meaning that only some LPSs can induce the inflammatory response (40). Immune activation depends on the type of acyl chains in LPSs (4). Prevotella and Bacteroides generally generate LPSs with 4 or 5 acyl chains. Furthermore, they carry two phosphate groups (4, 41, 42) while LPS generated by Enterobacteriaceae has six acyl chains and one phosphate group (4, 41). LPSs produced by Bacteroides cannot induce the production of cytokines. In contrast, LPS produced by E. coli can strongly provoke the production of various cytokines such as IL-10, TNF-α, IL-1β, and IL-6 (43). At the phylum level, Proteobacteria showed contradictory results. It had decreased abundance in two studies (3, 4) and increased abundance in one study (14). It was the most abundant phylum in gout patients and also in healthy controls (3). Some species of Proteobacteria, especially E.coli, can generate LPS which activates immune response (4). Proteobacteria downregulates urate oxidase but increases nitrogen fixation capacity in gout (14), which could be an effect of some species other than E.coli.
Therapeutic strategies
Our study aims to reveal microbiota dysbiosis in gout patients and after that, interventional approaches such as probiotics, special dietary patterns, and fecal microbiota transplantation (FMT) may be helpful to practically modulate the gut microbiota composition and amendment of symptoms. So these interventions are needed to be studied in future. Recommended dietary changes decrease SU and lower lipid levels in gout patients (44), and it has been determined that diet partly ameliorates gout by modulating gut microbiota composition (3).
It was observed that clostridium can lower uric acid levels, suggesting that clostridium can be added to gout-specific probiotic combinations (24, 45). Moreover, SCFAs such as butyrate and acetate have many beneficial effects for gout patients including anti-inflammatory effects and enhancing the intestinal barrier. So, SCFAs-producing bacteria such as Faecalibacterium prausnitzii, Oscillibacter, and Butyricicoccus could be great targets for intervention (1, 14). Furthermore, using Bifidobacterium can improve the anti-inflammatory effects (14).
Strengths and limitations
The present study has some strengths and limitations. The strength of our systematic review is the comprehensive search of all documents with high accuracy and precision to investigate the correlation between gut microbiota composition and gout. Besides, we evaluated the quality of animal and human studies. Diverse methods used for microbiota analysis, diagnostic tools and outcome measures in different studies are the main limitations of this systematic review which make it challenging to compare and combine the results. Besides, all included studies in this paper were observational in nature so it is hard to establish assured causality but instead correlations were acknowledged. Further studies are needed to generate basic knowledge for clarifying possible underlying mechanisms and any probable causal relationship.
Conclusion
Microbiota is deeply associated with inflammatory disorders like gout via many mechanisms. Some contradictory results prevent us to determine the role of some special bacterial taxa. There was no clear evidence regarding which bacteria are more protective. Different designs and methodologies of studies could be a reason for those controversies. Generally, studies were different in the eligibility criteria, sample size, diagnostic tools, microbiota and biochemical analysis techniques, which may warrant the controversies. Further studies are needed to improve gout through microbiota-modulating-based therapies.
Data availability statement
The original contributions presented in the study are included in the article/Supplementary material, further inquiries can be directed to the corresponding author.
Author contributions
SS-R is contributed as first author. H-SE and BL conceived and coordinated the study. H-SE and BL participated in design of study and cooperated to write final draft. SS-R and NK-N extracted information from the articles and drafted the manuscript. H-SE and SS-R edited the final draft of manuscript. H-SE reviewed the manuscript with a fault-finding view and SS-R did the quality assessment. The title is chosen by BL. All authors read and approved the final manuscript.
Conflict of interest
The authors declare that the research was conducted in the absence of any commercial or financial relationships that could be construed as a potential conflict of interest.
Publisher's note
All claims expressed in this article are solely those of the authors and do not necessarily represent those of their affiliated organizations, or those of the publisher, the editors and the reviewers. Any product that may be evaluated in this article, or claim that may be made by its manufacturer, is not guaranteed or endorsed by the publisher.
Supplementary material
The Supplementary Material for this article can be found online at: https://www.frontiersin.org/articles/10.3389/fmed.2023.1163778/full#supplementary-material
References
1. Guo Z, Zhang J, Wang Z, Ang KY, Huang S, Hou Q, et al. Intestinal microbiota distinguish gout patients from healthy humans. Sci Rep. (2016) 6:20602. doi: 10.1038/srep20602
2. Shao TJ, Shao L, Li HC, Xie ZJ, He ZX, Wen CP. Combined signature of the fecal microbiome and metabolome in patients with gout. Front Microbiol. (2017) 8:268. doi: 10.3389/fmicb.2017.00268
3. Ning Y, Yang GM, Chen YC, Zhao X, Qian HY, Liu Y, et al. Characteristics of the urinary microbiome from patients with gout: a prospective study. Front Endocrinol. (2020) 11. doi: 10.3389/fendo.2020.00272
4. Chu Y, Sun S, Huang Y, Gao Q, Xie X, Wang P, et al. Metagenomic analysis revealed the potential role of gut microbiome in gout. npj Biofilms Microbiomes. (2021) 7:2. doi: 10.1038/s41522-021-00235-2
5. Liu J, Cui L, Yan XM, Zhao XY, Cheng JL, Zhou L, et al. Analysis of oral microbiota revealed high abundance of prevotella intermedia in gout patients. Cell Physiol Biochem. (2018) 49:1804–12. doi: 10.1159/000493626
6. Dalbeth N, Choi H. Joosten LAB, Khanna PP, Matsuo H, Perez-Ruiz F, Stamp LK. Gout Nat Rev Dis Primers. (2019) 5:69. doi: 10.1038/s41572-019-0115-y
7. Liu X, Lv Q, Ren H, Gao L, Zhao P, Yang X, et al. The altered gut microbiota of high-purine-induced hyperuricemia rats and its correlation with hyperuricemia. PeerJ. (2020) 8:e8664. doi: 10.7717/peerj.8664
8. Lin S, Zhang T, Zhu L, Pang K, Lu S, Liao X, et al. Characteristic dysbiosis in gout and the impact of a uric acid-lowering treatment, febuxostat on the gut microbiota. J Agric Food Chem. (2021) 3:9. doi: 10.1016/j.jgg.2021.06.009
9. Robinson PC. Gout–An update of aetiology, genetics, co-morbidities and management. Maturitas. (2018) 118:67–73. doi: 10.1016/j.maturitas.2018.10.012
10. Xing SC, Meng DM, Chen Y, Jiang G, Liu XS Li N, et al. Study on the diversity of bacteroides and clostridium in patients with primary gout. Cell Biochem Biophys. (2015) 71:707–15. doi: 10.1007/s12013-014-0253-5
11. Henson MA. Interrogation of the perturbed gut microbiota in gouty arthritis patients through in silico metabolic modeling. NPJ Biofilms Microbiomes. (2021) 21:489–501. doi: 10.1002/elsc.202100003
12. Cassotta M, Forbes-Hernández TY, Calderón Iglesias R, Ruiz R, Elexpuru Zabaleta M, Giampieri F, et al. Links between nutrition, infectious diseases, and microbiota: emerging technologies and opportunities for human-focused research. Nutrients. (2020) 12:1827. doi: 10.3390/nu12061827
13. Ejtahed H-S, Oveissi V, Tabatabaei-Malazy O, Shirvani Rad S, Razi F, Larijani B. Analysis of scientific products about microbiota and COVID-19: a scientometric study. J Mazandaran Univ Med Sci. (2021) 31:200–11.
14. Méndez-Salazar EO, Vázquez-Mellado J, Casimiro-Soriguer CS, Dopazo J, Çubuk C, Zamudio-Cuevas Y, et al. Taxonomic variations in the gut microbiome of gout patients with and without tophi might have a functional impact on urate metabolism. Mol Med. (2021) 27:50. doi: 10.1186/s10020-021-00311-5
15. Yu YR, Liu QP, Li HC, Wen CP, He ZX. Alterations of the gut microbiome associated with the treatment o f hyperuricaemia in male rats. Front Microbiol. (2018) 9:2233. doi: 10.3389/fmicb.2018.02233
16. Lv Q, Xu D, Zhang X, Yang X, Zhao P, Cui X, et al. Association of hyperuricemia with immune disorders and intestinal barrier dysfunction. Front Physiol. (2020) 11:4236. doi: 10.3389/fphys.2020.524236
17. Xu DX, Lv QL, Wang XF, Cui XN, Zhao P, Yang XM, et al. Hyperuricemia is associated with impaired intestinal permeability in mice. Am J Physiol Gastroin Liver Physiol. (2019) 317:G484–G92. doi: 10.1152/ajpgi.00151.2019
18. Wang J, Chen Y, Zhong H, Chen F, Regenstein J, Hu X, et al. The gut microbiota as a target to control hyperuricemia pathogenesis: potential mechanisms and therapeutic strategies. Crit Rev Food Sci Nutri. (2021) 3:1–11. doi: 10.1080/10408398.2021.1874287
19. Moher D, Shamseer L, Clarke M, Ghersi D, Liberati A, Petticrew M, et al. Preferred reporting items for systematic review and meta-analysis protocols (PRISMA-P) 2015 statement. Syst Rev. (2015) 4:1–9. doi: 10.1186/2046-4053-4-1
20. Peterson J, Welch V, Losos M, Tugwell P. The Newcastle-Ottawa scale (NOS) for Assessing the Quality of Non-randomised Studies in Meta-Analyses. Ottawa: Ottawa Hospital Research Institute (2011) 2:1–12.
21. Ma LL, Wang YY, Yang ZH, Huang D, Weng H, Zeng XT. Methodological quality (risk of bias) assessment tools for primary and secondary medical studies: what are they and which is better? Milit Med Res. (2020) 7:1–11. doi: 10.1186/s40779-020-00238-8
22. Hooijmans CR, Rovers MM, de Vries R, Leenaars M, Ritskes-Hoitinga M, Langendam MW. SYRCLE's risk of bias tool for animal studies. BMC Med Res Methodol. (2014) 14:1–9. doi: 10.1186/1471-2288-14-43
23. Neogi T, Jansen TL, Dalbeth N, Fransen J, Schumacher HR, Berendsen D, et al. 2015 gout classification criteria: an American College of Rheumatology/European League Against Rheumatism collaborative initiative. Arthritis Rheumatol. (2015) 67:2557–68. doi: 10.1002/art.39254
24. Yang HT, Xiu WJ, Liu JK, Yang Y, Hou XG, Zheng YY, et al. Gut microbiota characterization in patients with asymptomatic hyperuricemia: probiotics increased. Bioengineered. (2021) 12:7263–75. doi: 10.1080/21655979.2021.1976897
25. Pan LB, Han P, Ma SR, Peng R, Wang C, Kong WJ, et al. Abnormal metabolism of gut microbiota reveals the possible molecular mechanism of nephropathy induced by hyperuricemia. Acta Pharmaceutica Sinica B. (2020) 10:249–61. doi: 10.1016/j.apsb.2019.10.007
26. Vogels Gvd, Van der Drift C. Degradation of purines and pyrimidines by microorganisms. Bacteriol Rev. (1976) 40:403–68. doi: 10.1128/br.40.2.403-468.1976
27. Lim MY, Rho M, Song YM, Lee K, Sung J, Ko G. Stability of gut enterotypes in Korean monozygotic twins and their association with biomarkers and diet. Sci Rep. (2014) 4:1–7. doi: 10.1038/srep07348
28. Shirvani-Rad S, Ejtahed H-S, Ettehad Marvasti F, Taghavi M, Sharifi F, Arzaghi SM, et al. The role of gut microbiota-brain axis in pathophysiology of ADHD: a systematic review. J Atten Disord. (2022) 3:10870547211073474. doi: 10.1177/10870547211073474
29. Cani PD, Bibiloni R, Knauf C, Waget A, Neyrinck AM, Delzenne NM, et al. Changes in gut microbiota control metabolic endotoxemia-induced inflammation in high-fat diet–induced obesity and diabetes in mice. Diabetes. (2008) 57:1470–81. doi: 10.2337/db07-1403
30. Wang HB, Wang PY, Wang X, Wan YL, Liu YC. Butyrate enhances intestinal epithelial barrier function via up-regulation of tight junction protein Claudin-1 transcription. Dig Dis Sci. (2012) 57:3126–35. doi: 10.1007/s10620-012-2259-4
31. Morrison DJ, Preston T. Formation of short chain fatty acids by the gut microbiota and their impact on human metabolism. Gut Microbes. (2016) 7:189–200. doi: 10.1080/19490976.2015.1134082
32. Chu Y, Huang Y, Huang Q, Xie X, Wang P, Li J, et al. Metagenomic study revealed the potential role of the gut microbiome in gout. medRxiv. (2019) 4:4142. doi: 10.1101/2019.12.21.19014142
33. Fossati G, Jansen T, Dinarello C, Netea M, Joosten L, Cleophas MC, et al. Mechanisms of Autoinflammation and Therapy Targets in Gout. Radboud University Nijmegen (2016) 75:179.
34. Den Besten G, Van Eunen K, Groen AK, Venema K, Reijngoud D-J, Bakker BM. The role of short-chain fatty acids in the interplay between diet, gut microbiota, and host energy metabolism. J Lipid Res. (2013) 54:2325–40. doi: 10.1194/jlr.R036012
35. Bian M, Wang J, Wang Y, Nie A, Zhu C, Sun Z, et al. Chicory ameliorates hyperuricemia via modulating gut microbiota and alleviating LPS/TLR4 axis in quail. Biomed Pharmacotherapy. (2020) 131:110719. doi: 10.1016/j.biopha.2020.110719
36. Fukuda S, Toh H, Hase K, Oshima K, Nakanishi Y, Yoshimura K, et al. Bifidobacteria can protect from enteropathogenic infection through production of acetate. Nature. (2011) 469:543–7. doi: 10.1038/nature09646
37. Ghadimi D, Hassan MFY, Fölster-Holst R, Röcken C, Ebsen M, de Vrese M, et al. Regulation of hepcidin/iron-signalling pathway interactions by commensal bifidobateria plays an important role for the inhibition of metaflammation-related biomarkers. Immunobiology. (2020) 225:151874. doi: 10.1016/j.imbio.2019.11.009
38. Yu D, Nguyen SM, Yang Y, Xu W, Cai H, Wu J, et al. Long-term diet quality is associated with gut microbiome diversity and composition among urban Chinese adults. Am J Clin Nutr. (2021) 113:684–94. doi: 10.1093/ajcn/nqaa350
39. Man SM. Inflammasomes in the gastrointestinal tract: infection, cancer and gut microbiota homeostasis. Nat Rev Gastroenterol Hepatol. (2018) 15:721–37. doi: 10.1038/s41575-018-0054-1
40. Whitfield C, Trent MS. Biosynthesis and export of bacterial lipopolysaccharides. Annu Rev Biochem. (2014) 83:99–128. doi: 10.1146/annurev-biochem-060713-035600
41. Vatanen T, Kostic AD, d'Hennezel E, Siljander H, Franzosa EA, Yassour M, et al. Variation in microbiome LPS immunogenicity contributes to autoimmunity in humans. Cell. (2016) 165:842–53. doi: 10.1016/j.cell.2016.04.007
42. Herath TD, Wang Y, Seneviratne CJ, Lu Q, Darveau RP, Wang CY, et al. lipopolysaccharide lipid A heterogeneity differentially modulates the expression of IL-6 and IL-8 in human gingival fibroblasts. J Clin Periodontol. (2011) 38:694–701. doi: 10.1111/j.1600-051X.2011.01741.x
43. Hofstad T, Sveen K, Dahlen G. Chemical composition, serological reactivity and endotoxicity of lipopolysaccharides extracted in different ways from Bacteroides fragilis, Bacteroides melaninogenicus and Bacteroides oralis. Acta Pathol Microbiol Scandinavica Section B Microbiol. (1977) 85:262–70. doi: 10.1111/j.1699-0463.1977.tb01972.x
44. Dessein P, Shipton E, Stanwix A, Joffe B, Ramokgadi J. Beneficial effects of weight loss associated with moderate calorie/carbohydrate restriction, and increased proportional intake of protein and unsaturated fat on serum urate and lipoprotein levels in gout: a pilot study. Ann Rheum Dis. (2000) 59:539–43. doi: 10.1136/ard.59.7.539
Keywords: gout, gut microbiota, microbiome, dysbiosis, hyperuricemia
Citation: Shirvani-Rad S, Khatibzade-Nasari N, Ejtahed H-S and Larijani B (2023) Exploring the role of gut microbiota dysbiosis in gout pathogenesis: a systematic review. Front. Med. 10:1163778. doi: 10.3389/fmed.2023.1163778
Received: 11 February 2023; Accepted: 28 April 2023;
Published: 17 May 2023.
Edited by:
Gabriela Angélica Martínez-Nava, National Institute of Rehabilitation Luis Guillermo Ibarra Ibarra, MexicoReviewed by:
Mangesh Vasant Suryavanshi, Lerner Research Institute, United StatesXinwang Duan, Second Affiliated Hospital of Nanchang University, China
Copyright © 2023 Shirvani-Rad, Khatibzade-Nasari, Ejtahed and Larijani. This is an open-access article distributed under the terms of the Creative Commons Attribution License (CC BY). The use, distribution or reproduction in other forums is permitted, provided the original author(s) and the copyright owner(s) are credited and that the original publication in this journal is cited, in accordance with accepted academic practice. No use, distribution or reproduction is permitted which does not comply with these terms.
*Correspondence: Hanieh-Sadat Ejtahed, aGFuaWVqdGFoZWRAeWFob28uY29t