- 1Department of Critical Care Medicine, Xiangyang Central Hospital, Affiliated Hospital of Hubei University of Arts and Science, Xiangyang, Hubei, China
- 2Nursing Department, Xiangyang Central Hospital, Affiliated Hospital of Hubei University of Arts and Science, Xiangyang, Hubei, China
- 3Department of Nursing, School of Nursing, Wuhan University, Wuhan, Hubei, China
Purpose: To evaluate the effects of different mechanical ventilation modes on critical patients.
Methods: PubMed, Embase, Web of science, and Cochrane Library databases were searched from their inception to November 15, 2022 for randomized controlled trials on the application of different mechanical ventilation modes in critical patients. Two researchers independently screened the literature, extracted data, and assessed the risk of bias in the included studies. R4.2.1 was used for this network meta-analysis.
Results: Twenty-eight RCTs involving 3,189 patients were included. The interventions in these RCTs included NAVA (neurally adjusted ventilatory assist), PAV (proportional assist ventilation), ASV (adaptive support ventilation), Smartcare/PS (Smartcare/pressure support), PSV (pressure support ventilation), PSV_ATC (pressure support ventilation_automatic tube compensation), and SIMV (synchronized intermittent mandatory ventilation). The network meta-analysis showed that, compared with the PSV group, there was no significant difference in duration of mechanical ventilation, duration of ICU stay, and hospital stay between NAVA, SIMV, AVS, PAV, Smartcare/PS, and PSV_ATC groups. Compared with PSV, PAV improved the success rate of withdrawal of ventilator [OR = 3.07, 95%CI (1.21, 8.52)]. Compared with PSV and PAV, NAVA reduced mortality in the ICU [OR = 0.63, 95%CI (0.43, 0.93); OR = 0.45, 95%CI (0.21, 0.97)].
Conclusion: NAVA can reduce mortality in ICU, and PAV may increase the risk of withdrawal of the ventilator. There was no significant difference between PSV and other mechanical ventilation modes (NAVA, SIMV, AVS, PAV, Smartcare/PS, and PSV_ATC) in the duration of mechanical ventilation, duration of ICU stay, or hospital stay. Due to the limitations, more high-quality studies are needed to verify these findings.
1. Introduction
Critical patients are characterized by acute onset, rapid change, and a high mortality rate, mainly manifested as acute hypoxic respiratory insufficiency or respiratory failure. General treatment often fails in treating this condition and mechanical ventilation is the main approach (1). As more intensive care units (ICUs) have been built in recent years, ventilators have been widely applied, and mechanical ventilation has showed remarkable effects in the treatment of critical patients (2). Mechanical ventilation is an approach to airway maintenance that uses a ventilator. Generally, mechanical ventilation utilizes mechanical devices to change, control, or replace the patients’ spontaneous breathing movement. Mechanical ventilation can improve ventilation and oxygenation, prevent the accumulation of carbon dioxide, and ameliorate hypoxia, thus preparing critical patients for further treatment (3, 4). The mechanisms of different mechanical ventilation modes and their effects on patients varied greatly. In current clinical practice, ventilation modes for critical patients include NAVA, SIMV, PSV, AVS, PAV, Smartcare/PS, and PSV_ATC (5, 6). Although mechanical ventilation has assured benefits, long-term invasive mechanical ventilation could probably induce lung infection, lung volutrauma, atelectrauma, tracheal injury, biological damage, barotrauma, oxygen poisoning, and diaphragmatic insufficiency (7). Moreover, it is challenging to stop using the ventilator in time. Extending the time to withdrawal of mechanical ventilation can increase the mortality and hospitalization time, result in high treatment costs, and increase the economic burden of patients. Therefore, it is of an urgent need to find the most effective mechanical ventilation mode in clinical practice (8).
At present, there is no definite agreement on which mode to use for mechanical ventilation in critical patients (9). This study aimed to resolve the disputes via network meta-analysis to provide potent evidence for the clinical decision of choosing the most effective treatment.
2. Materials and methods
2.1. Literature retrieval
PubMed, Embase, Cochrane Library, and Web of science databases were searched for RCTs on the use of different mechanical ventilation modes for critical patients. The retrieval was as of November 15, 2022. Subject terms and free words were used, and the search terms included (Interactive Ventilatory Support OR Proportional Assist Ventilation OR Assist Ventilation, Proportional OR adaptive support ventilation OR Smartcare/PS) AND (Critical Illness OR Intensive Care Units). The specific search strategies are shown in Supplementary Table S1.
2.2. Inclusion and exclusion criteria
Inclusion criteria: critical patients (>18 years of age) admitted to the ICU due to a critical illness; treatment with mechanical ventilation (including NAVA, SIMV, PSV, AVS, PAV, Smartcare/PS, PSV_ATC); the primary outcome measures were duration of mechanical ventilation, duration of ICU stay, hospital stay, and ICU mortality; and the secondary outcome measure was withdrawal of the ventilator.
Exclusion criteria: conference abstract, protocol, letter, duplication, system review, full text unavailable, data unavailable, and animal experiments.
2.3. Data extraction
Two independent reviewers screened the literature to extract data. The titles and abstracts of the literature were reviewed. Professionals of the related area were consulted if the reviewers had disagreements on the articles. The full texts of the articles were downloaded for screening. The inclusion and exclusion criteria were strictly followed in the literature screening. The outcome measures of the two groups were extracted and cross-checked to ensure the consistency of the extracted data. The extracted data mainly included the first author’s name, year of publication, country, sample size, gender, age, patient characteristics, and outcome measures.
2.4. Quality evaluation
The quality evaluation of the included studies was completed independently by 2 researchers. The quality of the included studies was evaluated using the bias evaluation tool of Cochrane Handbook for Systemic Reviews of Interactions 5.1.0. The evaluation included 7 items: random sequence generation (selective bias), allocation concealment (selective bias), blinding to the implementer and participant (implementation bias), blinding to the outcome evaluator (observation bias), result integrity (follow-up bias), selective reporting of study results (reporting bias), and other sources of bias. Each included study was evaluated according to the items above. The studies were rated as “low risk” if all the criteria above were met, indicating that the overall risk of bias was low and the study quality was high. The studies were rated as “unclear risk” if part of the criteria above were met, indicating that there was a moderate risk of bias. The studies were rated as “high risk” if none of the criteria above were met, indicating that there was a high risk of bias and the study quality was low.
2.5. Statistical analysis
The gemtc software package in R 4.2.1 software and the JAGS software were used to conduct this network meta-data analysis and plot a network evidence map as well as a probability ranking map. The point and interval estimations were used as indicators for effect size. The continuous variables (hospitalization duration, ICU duration, and mechanical ventilation duration) were presented as standard mean difference (SMD) with 95% confidence interval (CI). SMD < 0 indicated that one ventilation mode might be inferior to the other, and SMD > 0 indicated that one ventilation mode might be more effective than the other. The inclusion of 0 in the 95%CI indicated no significant difference. The discrete variables (ICU mortality and withdrawal of the ventilator) was presented as odds ratio (OR) with 95% CI. OR < 1 indicated that one ventilation mode might be inferior to the other, and OR > 1 that one ventilation mode might be more effective than the other. The inclusion of 1 in the 95% CI indicated no significant difference. In this study, Bayesian Markov–Monte Carlo random-effects model was used to quantitatively synthesize the results. Five chains were used for simulation, with 5,000 pre-iterations and 20,000 iterations. When there was a closed loop in the evidence network, the node splitting method was used for the inconsistency test to verify whether the direct comparison was consistent with the indirect comparison. p > 0.05 indicated that there was no significant inconsistency between the direct and indirect comparisons; otherwise, there was statistically significant inconsistency. The probability ranking map was drawn and the priorities of different interventions were ranked according to the SUCRA values.
3. Results
3.1. Literature screening and results
PubMed, Embase, Cochrane Library, and Web of Science databases were searched and a total of 1,530 articles were initially retrieved. 1,082 articles were obtained after removing the duplication. Fifty-two articles were excluded by reading the titles and abstracts, and 28 articles were finally obtained by reading the full text. The detailed screening process is shown in Figure 1.
3.2. The table for the basic characteristics of the included articles
A total of 28 (10–37) RCTs were included, involving 3,189 patients and interventions such as NAVA, SIMV, PSV, AVS, PAV, Smartcare/PS, and PSV_ATC. The patients were admitted to the ICU mainly due to ARDS, COPD, etc. The table for baseline characteristics is shown in Table 1.
3.3. The evaluation of risk of bias of the included articles
The specific methods for generation of random sequences were explicitly reported in the 28 included studies, which were rated as low-risk. The main methods included a random number table and a computer-generated random number table. The basic characteristics of the included articles are shown in Figures 2, 3.
3.4. Network diagram
The network diagram involving 5 interventions is shown in Figure 4. The spot represented the specific intervention, and the size of the spot indicated the number of patients receiving the intervention. Straight lines indicated that there was evidence of a direct comparison between the 2 interventions, and the thickness of the straight lines indicated the number of studies directly comparing the 2 interventions.
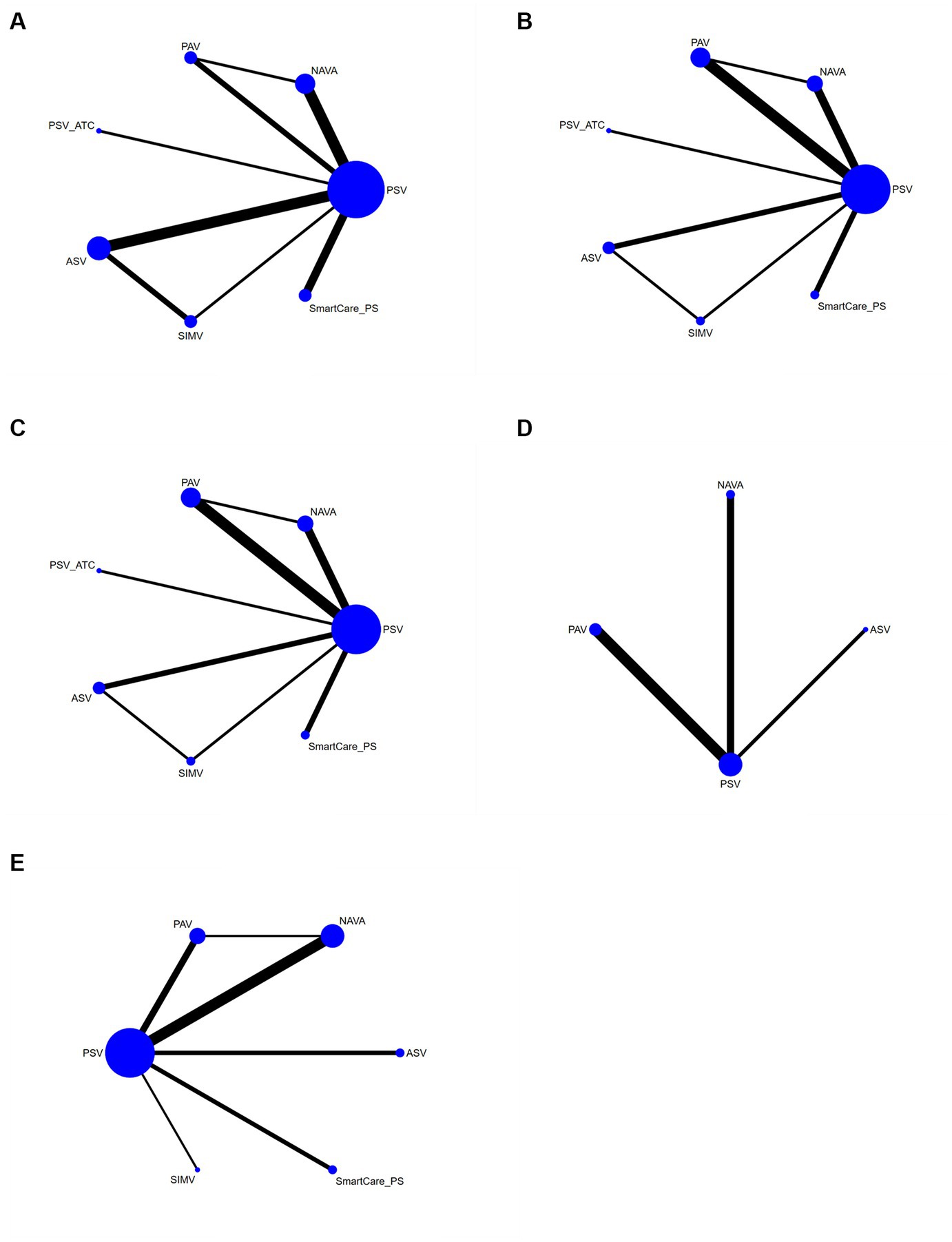
Figure 4. Network diagram (A) duration of mechanical ventilation, (B) duration of ICU stay, (C) hospital stay, (D) successfully weaned from the ventilator, and (E) ICU mortality.
3.5. Network meta-analysis results
3.5.1. Duration of mechanical ventilation
A total of 18 studies involving a total of 1,745 patients mentioned the duration of mechanical ventilation (the patients did not switch from invasive ventilation to non-invasive ventilation after extubating). The network meta-analysis generated 21 direct or indirect comparisons without statistical significance. This indicated that there were no significant differences in the duration of mechanical ventilation between PSV and the other interventions (NAVA, SIMV, AVS, PAV, Smartcare/PS, and PSV_ATC) (Supplementary Table S2). The interventions were ranked according to SUCRA values as follows: SIMV (83%) > ASV (79%) > NAVA (52%) > Smartcare/PS (51%) > PAV (40%) > PSV (24%) > PSV_ATC (21%) (Supplementary Table S3).
3.5.2. Duration of ICU stay
The duration of ICU stay was reported in 19 articles involving a total of 1,927 patients. The network meta-analysis generated 21 direct or indirect comparisons without statistical significance. This indicated that there was no significant differences in the duration of ICU stay between PSV and the other interventions (NAVA, SIMV, AVS, PAV, Smartcare/PS, and PSV_ATC) (Supplementary Table S2). The interventions were ranked according to SUCRA values as follows: Smartcare/PS (68%) > NAVA (65%) > ASV (60%) > PSV_ATC (46%) > PAV (41%) > PSV (37%) > SIMV (33%) (Supplementary Table S3).
3.5.3. Hospital stay
The hospital stay was reported in 15 studies involving a total of 1,625 patients. The network meta-analysis generated 21 direct or indirect comparisons without statistical significance. This indicated that there were no significant differences in the duration of ICU stay between PSV and the other interventions (NAVA, SIMV, AVS, PAV, Smartcare/PS, and PSV_ATC) (Supplementary Table S2). The interventions were ranked according to SUCRA values as follows: Smartcare/PS (67%) > NAVA (65%) > ASV (60%) > PAV_ATC (45%) > PAV (41%) > PSV (37%) > SIMV (33%) (Supplementary Table S3).
3.5.4. Withdrawal of the ventilator
The withdrawal of the ventilator was reported in 6 studies involving a total of 584 patients and interventions such as ASV, PAV, PSV, NAVA, etc. The network meta-analysis generated 6 direct or indirect comparisons. Compared with PSV, PAV increased the rate of withdrawal of the ventilator [OR = 3.07, 95%CI (1.21, 8.52)] (Supplementary Table S2). The interventions were ranked according to SUCRA values as follows: PAV (89%) > NAVA (58%) > ASV (40%) > PSV (13%) (Figure 5).
3.5.5. ICU mortality
ICU mortality was reported in 14 articles involving 1,587 subjects and interventions such as NAVA, SIMV, PSV, AVS, PAV, and Smartcare/PS. A meta meta-analysis generated 15 direct and indirect comparisons. Compared with PSV and PAV, NAVA reduced ICU mortality (0.63, 95%CI (0.43, 0.93); 0.45, 95%CI (0.21, 0.97)). The interventions were ranked according to SUCRA values as follows: NAVA (94%) > SIMV (54%) > PSV (52%) > ASV (40%) > SmartCare/PS (36%) > PAV (24%) (Figure 6).
3.6. Publication bias
The correction-comparison funnel plot was created to evaluate the publication bias of duration of mechanical ventilation, duration of ICU stay, hospital stay, ICU mortality, and withdrawal of the ventilator. The result showed that the plot was not symmetric, indicating that there might be publication bias. Results are presented in Supplementary Table S4.
4. Discussion
To our knowledge, this is the first network meta-analysis comparing different types of ventilation modes. Mechanical ventilation is a life-support system used to ensure blood gas exchange and to assist the respiratory muscles in ventilating the lungs during the acute phase of lung disease or after surgery. Positive pressure mechanical ventilation is very different from normal physiological breathing (12). This can lead to several negative physiological consequences for the lungs and peripheral organs. Firstly, hemodynamic changes affect cardiovascular function, cerebral perfusion pressure (CPP) and renal venous drainage. Second, the negative effects of mechanical ventilation (compressive stress) on alveolar-capillary membranes and extracellular matrix may cause local and systemic inflammation and promote lung and peripheral organ damage. Third, thoracic hypertension may further impair lung and peripheral organs functioning during controlled and assisted ventilation (14, 35).
We found that there was no significant difference between PSV and other mechanical ventilation modes (NAVA, SIMV, AVS, PAV, Smartcare/PS, and PSV_ATC) in the duration of mechanical ventilation, duration of ICU stay, or hospital stay. SIMV mode could assist the patients in spontaneous breathing under the preset respiratory parameters every minute. ASV is a safe and effective mode based on an adaptive ventilation technique and intelligent synchronization technique, which can be applied in the whole treatment process from intubation to withdrawal of the ventilator (38, 39). Smartcare/PS mode is a new computer-controlled pressure-supported closed-loop ventilation mode, which is commonly used in the stage of withdrawal of the ventilator (40). These conclusions were consistent with a previous meta-analysis (41), which conducted a combined analysis of 20 studies and revealed that there was no significant difference in the duration of mechanical ventilation between NAVA, Smart care/PS, and ASV. In clinical practice, the difficulty in withdrawal of the ventilator could prolong the mechanical ventilation time and increase the risk of mechanical ventilation-related complications, thus prolonging the patients’ stay in the ICU (42). Studies have found that diaphragmatic dysfunction might result in prolonged hospitalization in ICU, failure in withdrawal of the ventilator and increased mortality, while long-term mechanical ventilation could in turn aggravate diaphragmatic dysfunction. These two factors promote and influence each other, which leads to the prolonged hospitalization of patients. In this study, we also found that, compared with PSV, PAV significantly improved the success rate of withdrawal of the ventilator [OR = 3.07, 95% CI (1.21, 8.52)]. PAV mode effectively reduced respiratory work, relieved respiratory muscle fatigue, and improved man–machine coordination and the quality of mechanical ventilation (43, 44). Baudin et al. (45) also proposed that increased respiratory load resulting from man–machine coordination was the main reason for patients’ dependence on the ventilator and failure in withdrawal of the ventilator. Our study also found that the success rate of withdrawal of the ventilator in the PAV group was higher than that in the PSV group, indicating that the PAV mode could reduce respiratory work, relieve respiratory muscle fatigue, improve man–machine coordination and the quality of mechanical ventilation, and consequently assist the patients in the withdrawal of the ventilator. Compared with PSV and PAV, NAVA could reduce ICU mortality (0.63, 95%CI (0.43, 0.93); 0.45, 95%CI (0.21, 0.97)). NAVA is a new assistive ventilation mode directly coupling mechanical ventilation and nerve impulses, which can control the switch of inspiratory and expiratory functions of the ventilator via patients’ own feedback mechanism, thereby reducing the dependence on the ventilator of the ICU patients (46, 47). Some study (48) demonstrated that NAVA could reduce respiratory work and prevent respiratory muscle weakness caused by traditional mechanical ventilation. This new mechanical ventilation mode has significant advantages as follows: (1) since the nerve impulses are monitored, NAVA can effectively shorten the trigger delay, significantly improve human-computer synchronization and avoid false triggering. (2) The breathing mode of NAVA is the most similar to the physiological status. It also adopted the most intelligent tidal volume to maximize man–machine coordination. Firestone et al. (49) also confirmed that respiratory regulation required changes at any time due to the changing condition in patients with respiratory distress syndrome. NAVA mode uses the brain as the most intelligent regulation center to effectively regulate various physiological needs such as respiration, we also need to pay attention to the effects of prone ventilation on the heart, such as reducing heart rate, reducing cardiac load, and increasing cardiac output.
The limitations must be considered when interpreting the conclusions above. First, most studies did not explicitly describe allocation concealment, which might result in selection, implementation, and measurement biases. Second, there was publication bias, which required careful interpretation of the results of this study. Finally, due to the nature of the studied interventions, the blinding of the outcome evaluators was not clearly reported in most enrolled RCTs, thus introducing bias into our analysis. Although in some studies the application of PSV relied on routine care or local guidelines and most assessments were well defined, the risk of deviations in using PSV cannot be completely reduced.
Conclusion
NAVA can reduce mortality in ICU, and PAV may increase the risk of withdrawal of the ventilator. There was no significant difference between PSV and other mechanical ventilation modes (NAVA, SIMV, AVS, PAV, Smartcare/PS, and PSV_ATC) in the duration of mechanical ventilation, duration of ICU stay, or hospital stay. Due to the limitations, more high-quality studies are needed to verify these findings.
Data availability statement
The original contributions presented in the study are included in the article/Supplementary material, further inquiries can be directed to the corresponding author.
Author contributions
MW, XZ, YJ, and YY: concept and design. MW, YG, WZ, HH, and YY: acquisition of data. MW, XZ, YJ, YG, WZ, and HH: statistical analysis. MW, XZ, and YY: interpretation of data and writing original draft. MW, XZ, YJ, YG, WZ, HH, and YY: writing review and editing. All authors contributed to the article and approved the submitted version.
Conflict of interest
The authors declare that the research was conducted in the absence of any commercial or financial relationships that could be construed as a potential conflict of interest.
Publisher’s note
All claims expressed in this article are solely those of the authors and do not necessarily represent those of their affiliated organizations, or those of the publisher, the editors and the reviewers. Any product that may be evaluated in this article, or claim that may be made by its manufacturer, is not guaranteed or endorsed by the publisher.
Supplementary material
The Supplementary material for this article can be found online at: https://www.frontiersin.org/articles/10.3389/fmed.2023.1159567/full#supplementary-material
References
1. Juern, JS . Removing the critically ill patient from mechanical ventilation. Surg Clin North Am. (2012) 92:1475–83. doi: 10.1016/j.suc.2012.08.008
2. Nakajima, Y . Controversies in the temperature management of critically ill patients. J Anesth. (2016) 30:873–83. doi: 10.1007/s00540-016-2200-7
3. Rackley, CR . Monitoring during mechanical ventilation. Respir Care. (2020) 65:832–46. doi: 10.4187/respcare.07812
4. Rialp, G . Mechanical ventilation: past and present. Med Intensiva (Engl Ed). (2021) 45:1–2. doi: 10.1016/j.medin.2020.08.012
5. Modrykamien, A, Chatburn, RL, and Ashton, RW. Airway pressure release ventilation: an alternative mode of mechanical ventilation in acute respiratory distress syndrome. Cleve Clin J Med. (2011) 78:101–10. doi: 10.3949/ccjm.78a.10032
6. Orso, D, Vetrugno, L, Federici, N, Borselli, M, Spadaro, S, Cammarota, G, et al. Mechanical ventilation management during mechanical chest compressions. Respir Care. (2021) 66:334–46. doi: 10.4187/respcare.07775
7. Vetrugno, L, Castaldo, N, Fantin, A, et al. Ventilatory associated barotrauma in COVID-19 patients: a multicenter observational case control study (COVI-MIX-study). Pulmonology. (2022) 24:S2531–0437. doi: 10.1016/j.pulmoe.2022.11.002
8. Alessandri, F, Pugliese, F, and Ranieri, VM. Mechanical ventilation: we have come a long way but still have a long road ahead. Lancet Respir Med. (2017) 5:922–4. doi: 10.1016/S2213-2600(17)30431-9
9. Goligher, EC, Ferguson, ND, and Brochard, LJ. Clinical challenges in mechanical ventilation. Lancet. (2016) 387:1856–66. doi: 10.1016/S0140-6736(16)30176-3
10. Zhang, J, Yang, Z, Chen, K, Zhang, X, Zhao, T, and Zhang, X. Efficacy of adaptive ventilation support combined with lung recruitment maneuvering for acute respiratory distress syndrome. Am J Transl Res. (2022) 14:2109–16.
11. Liu, L, Xu, X, Sun, Q, Yu, Y, Xia, F, Xie, J, et al. Neurally adjusted ventilatory assist versus pressure support ventilation in difficult weaning: a randomized trial. Anesthesiology. (2020) 132:1482–93. doi: 10.1097/ALN.0000000000003207
12. Kacmarek, RM, Villar, J, Parrilla, D, Alba, F, Solano, R, Liu, S, et al. Neurally adjusted ventilatory assist in acute respiratory failure: a randomized controlled trial. Intensive Care Med. (2020) 46:2327–37. doi: 10.1007/s00134-020-06181-5
13. Hadfield, DJ, Rose, L, Reid, F, Cornelius, V, Hart, N, Finney, C, et al. Neurally adjusted ventilatory assist versus pressure support ventilation: a randomized controlled feasibility trial performed in patients at risk of prolonged mechanical ventilation. Crit Care. (2020) 24:220. doi: 10.1186/s13054-020-02923-5
14. Chittawatanarat, K, Orrapin, S, Jitkaroon, K, Mueakwan, S, and Sroison, U. An open label randomized controlled trial to compare low level pressure support and T-piece as strategies for discontinuation of mechanical ventilation in a general surgical intensive care unit. Med Arch. (2018) 72:51–7. doi: 10.5455/medarh.2018.72.51-57
15. Botha, J, Green, C, Carney, I, Haji, K, Gupta, S, and Tiruvoipati, R. Proportional assist ventilation versus pressure support ventilation in weaning ventilation: a pilot randomised controlled trial. Crit Care Resusc. (2018) 20:33–40.
16. Arnal, JM, Garnero, A, Novotni, D, Corno, G, Donati, SY, Demory, D, et al. Closed loop ventilation mode in intensive care unit: a randomized controlled clinical trial comparing the numbers of manual ventilator setting changes. Minerva Anestesiol. (2018) 84:58–67. doi: 10.23736/S0375-9393.17.11963-2
17. Yazdannik, A, Zarei, H, and Massoumi, G. Comparing the effects of adaptive support ventilation and synchronized intermittent mandatory ventilation on intubation duration and hospital stay after coronary artery bypass graft surgery. Iran J Nurs Midwifery Res. (2016) 21:207–12. doi: 10.4103/1735-9066.178250
18. Kuo, NY, Tu, ML, Hung, TY, Liu, SF, Chung, YH, Lin, MC, et al. A randomized clinical trial of neurally adjusted ventilatory assist versus conventional weaning mode in patients with COPD and prolonged mechanical ventilation. Int J Chron Obstruct Pulmon Dis. (2016) 11:945–51. doi: 10.2147/COPD.S103213
19. Di Mussi, R, Spadaro, S, Mirabella, L, Volta, CA, Serio, G, Staffieri, F, et al. Impact of prolonged assisted ventilation on diaphragmatic efficiency: NAVA versus PSV. Crit Care. (2016) 20:1. doi: 10.1186/s13054-015-1178-0
20. Demoule, A, Clavel, M, Rolland-Debord, C, Perbet, S, Terzi, N, Kouatchet, A, et al. Neurally adjusted ventilatory assist as an alternative to pressure support ventilation in adults: a French multicentre randomized trial. Intensive Care Med. (2016) 42:1723–32. doi: 10.1007/s00134-016-4447-8
21. Bosma, KJ, Read, BA, Bahrgard Nikoo, MJ, Jones, PM, Priestap, FA, and Lewis, JF. A pilot randomized trial comparing weaning from mechanical ventilation on pressure support versus proportional assist ventilation. Crit Care Med. (2016) 44:1098–108. doi: 10.1097/CCM.0000000000001600
22. Teixeira, SN, Osaku, EF, Costa, CR, Toccolini, BF, Costa, NL, Cândia, MF, et al. Comparison of proportional assist ventilation plus, T-tube ventilation, and pressure support ventilation as spontaneous breathing trials for Extubation: a randomized study. Respir Care. (2015) 60:1527–35. doi: 10.4187/respcare.03915
23. Taniguchi, C, Victor, ES, Pieri, T, Henn, R, Santana, C, Giovanetti, E, et al. Smart care™ versus respiratory physiotherapy-driven manual weaning for critically ill adult patients: a randomized controlled trial. Crit Care. (2015) 19:246. doi: 10.1186/s13054-015-0978-6
24. Mohamed, KAE, and El Maraghi, SK. Role of adaptive support ventilation in weaning of COPD patients. Egyptian journal of chest diseases and tuberculosis. (2014) 63:449–54. doi: 10.1016/j.ejcdt.2013.12.017
25. Elganady, AA, Beshey, BN, and Abdelaziz, AAH. Proportional assist ventilation versus pressure support ventilation in the weaning of patients with acute exacerbation of chronic obstructive pulmonary disease. Egyptian J Chest Diseases Tuberculosis. (2014) 63:643–50. doi: 10.1016/j.ejcdt.2014.04.001
26. Sasikumar, S, Shanbhag, V, Shenoy, A, and Unnikrishnan, R. Comparison of pressure support and proportional assist ventilation with load-adjustable gain factors for weaning from mechanical ventilation in critically ill patients. European respiratory society annual congress, 2013 September, 7–11, Barcelona, Spain. (2013); 42:1045s [P4926]
27. Agarwal, R, Srinivasan, A, Aggarwal, AN, and Gupta, D. Adaptive support ventilation for complete ventilatory support in acute respiratory distress syndrome: a pilot, randomized controlled trial. Respirology. (2013) 18:1108–15. doi: 10.1111/resp.12126
28. Schädler, D, Engel, C, Elke, G, Pulletz, S, Haake, N, Frerichs, I, et al. Automatic control of pressure support for ventilator weaning in surgical intensive care patients. Am J Respir Crit Care Med. (2012) 185:637–44. doi: 10.1164/rccm.201106-1127OC
29. Aghadavoudi, O, Kamran, M, and Masoudifar, M. Comparison of two modes of ventilation after fast-track cardiac surgery: adaptive support ventilation versus synchronized intermittent mandatory ventilation. Pakistan J Med Sci. (2012) 28:303–8.
30. Kirakli, C, Ozdemir, I, Ucar, ZZ, Cimen, P, Kepil, S, and Ozkan, SA. Adaptive support ventilation for faster weaning in COPD: a randomised controlled trial. Eur Respir J. (2011) 38:774–80. doi: 10.1183/09031936.00081510
31. Stahl, C, Dahmen, G, Ziegler, A, and Muhl, E. Comparison of automated protocol-based versus non-protocol-based physician-directed weaning from mechanical ventilation. Intensivmedizin Notfallmedizin. (2009) 46:441–6. doi: 10.1007/s00390-009-0061-0
32. Dongelmans, DA, Veelo, DP, Paulus, F, de Mol, BA, Korevaar, JC, Kudoga, A, et al. Weaning automation with adaptive support ventilation: a randomized controlled trial in cardiothoracic surgery patients. Anesth Analg. (2009) 108:565–71. doi: 10.1213/ane.0b013e318190c49f
33. Aggarwal, AN, Agarwal, R, and Gupta, D. Automatic tube compensation as an adjunct for weaning in patients with severe neuroparalytic snake envenomation requiring mechanical ventilation: a pilot randomized study. Respir Care. (2009) 54:1697–702.
34. Xirouchaki, N, Kondili, E, Vaporidi, K, Xirouchakis, G, Klimathianaki, M, Gavriilidis, G, et al. Proportional assist ventilation with load-adjustable gain factors in critically ill patients: comparison with pressure support. Intensive Care Med. (2008) 34:2026–34. doi: 10.1007/s00134-008-1209-2
35. Rose, L, Presneill, JJ, Johnston, L, and Cade, JF. A randomised, controlled trial of conventional versus automated weaning from mechanical ventilation using SmartCare/PS. Intensive Care Med. (2008) 34:1788–95. doi: 10.1007/s00134-008-1179-4
36. Fernández-Vivas, M, Caturla-Such, J, González de la Rosa, J, Acosta-Escribano, J, Alvarez-Sánchez, B, and Cánovas-Robles, J. Noninvasive pressure support versus proportional assist ventilation in acute respiratory failure. Intensive Care Med. (2003) 29:1126–33. doi: 10.1007/s00134-003-1768-1
37. Bernstein, G, Mannino, FL, Heldt, GP, Callahan, JD, Bull, DH, Sola, A, et al. Randomized multicenter trial comparing synchronized and conventional intermittent mandatory ventilation in neonates. J Pediatrics. (1996) 4:453–63. doi: 10.1016/s0022-3476(96)70354-2
38. Casali, D . Influence of ventilatory strategies on outcomes and length of hospital stay: assist control and synchronized intermittent mandatory ventilation modes. Intern Emerg Med. (2021) 16:263–5. doi: 10.1007/s11739-020-02527-5
39. Cekmen, B, Bildik, B, Bozan, O, Atis, SE, Dogan, S, and Kocak, AO. Utility of non-invasive synchronized intermittent mandatory ventilation in acute cardiogenic pulmonary edema. Am J Emerg Med. (2022) 56:71–6. doi: 10.1016/j.ajem.2022.03.044
40. Mersmann, S . SmartCare: automatizing clinical guidelines. Biomed Tech. (2009) 54:283–8. doi: 10.1515/BMT.2009.034
41. Kampolis, CF, Mermiri, M, Mavrovounis, G, Koutsoukou, A, Loukeri, AA, and Pantazopoulos, I. Comparison of advanced closed-loop ventilation modes with pressure support ventilation for weaning from mechanical ventilation in adults: a systematic review and meta-analysis. J Crit Care. (2022) 68:1–9. doi: 10.1016/j.jcrc.2021.11.010
42. Cohen, IL, Lambrinos, J, and Fein, IA. Mechanical ventilation for the elderly patient in intensive care. Incremental changes and benefits. JAMA. (1993) 269:1025–9.
43. Delgado, M, Subirá, C, Hermosa, C, Gordo, F, Riera, J, and Fernández, R. Proportional assist ventilation feasibility in the early stage of respiratory failure: a prospective randomized multicenter trial. Minerva Anestesiol. (2019) 85:862–70. doi: 10.23736/S0375-9393.19.12618-1
44. Wysocki, M, Richard, JC, and Meshaka, P. Noninvasive proportional assist ventilation compared with noninvasive pressure support ventilation in hypercapnic acute respiratory failure. Crit Care Med. (2002) 30:323–9. doi: 10.1097/00003246-200202000-00010
45. Baudin, F, Emeriaud, G, Essouri, S, Beck, J, Javouhey, E, and Guerin, C. Neurally adjusted ventilatory assist decreases work of breathing during non-invasive ventilation in infants with severe bronchiolitis. Crit Care. (2019) 23:120. doi: 10.1186/s13054-019-2379-8
46. Cammarota, G, Sguazzotti, I, Della Corte, F, and Vaschetto, R. Cheyne-stokes breathing pattern and neurally adjusted ventilatory assist in a neuro-critical patient. Intensive Care Med. (2020) 46:540–1. doi: 10.1007/s00134-019-05774-z
47. Gama de Abreu, M, and Belda, FJ. Neurally adjusted ventilatory assist: letting the respiratory center take over control of ventilation. Intensive Care Med. (2013) 39:1481–3. doi: 10.1007/s00134-013-2953-5
48. Saddy, F, Sutherasan, Y, Rocco, PR, and Pelosi, P. Ventilator-associated lung injury during assisted mechanical ventilation. Semin Respir Crit Care Med. (2014) 35:409–17. doi: 10.1055/s-0034-1382153
Keywords: critical illness, mechanical ventilation, network meta-analysis, systematic review, mortality
Citation: Wu M, Zhang X, Jiang Y, Guo Y, Zhang W, He H and Yin Y (2023) Comparison of clinical outcomes in critical patients undergoing different mechanical ventilation modes: a systematic review and network meta-analysis. Front. Med. 10:1159567. doi: 10.3389/fmed.2023.1159567
Edited by:
Dawei Yang, Fudan University, ChinaReviewed by:
Frédéric Lofaso, Hôpital Raymond-Poincaré, FranceLuigi Vetrugno, University of Studies G. d’Annunzio Chieti and Pescara, Italy
Copyright © 2023 Wu, Zhang, Jiang, Guo, Zhang, He and Yin. This is an open-access article distributed under the terms of the Creative Commons Attribution License (CC BY). The use, distribution or reproduction in other forums is permitted, provided the original author(s) and the copyright owner(s) are credited and that the original publication in this journal is cited, in accordance with accepted academic practice. No use, distribution or reproduction is permitted which does not comply with these terms.
*Correspondence: Yanhua Yin, MTkwNTgyMDExNkBxcS5jb20=
†These authors have contributed equally to this work and share first authorship