- 1Department of Endocrinology and Metabolism, Dushu Lake Hospital Affiliated to Soochow University, Medical Center of Soochow University, Suzhou, Jiangsu, China
- 2The First Clinical Medical College, Soochow University, Suzhou, Jiangsu, China
- 3Department of Ophthalmology, The First Affiliated Hospital of Soochow University, Suzhou, Jiangsu, China
- 4Department of Ophthalmology, Changshu No. 1 People’s Hospital, Suzhou, Jiangsu, China
Thioredoxin-interacting protein (TXNIP) was first isolated from Vitamin D3-exposed HL60 cells. TXNIP is the main redox-regulating factor in various organs and tissues. We begin with an overview of the TXNIP gene and protein information, followed by a summary of studies that have shown its expression in human kidneys. Then, we highlight our current understanding of the effect of TXNIP on diabetic kidney disease (DKD) to improve our understanding of the biological roles and signal transduction of TXNIP in DKD. Based on the recent review, the modulation of TXNIP may be considered as a new target in the management of DKD.
Introduction
Diabetic kidney disease (DKD) is the main diabetes-related complication and a major factor resulting in end-stage renal disease (ESRD). It imposes a substantial financial burden and greatly affects the lives of the patients and their families. Hyperglycemia is the primary factor but not the only etiological factor inducing DKD. The initiation and maintenance of intrarenal pathogenetic pathways due to an increase in glucose concentrations are aided by a variety of processes such as dyslipidemia, oxidative stress, inflammation, vascular perfusion changes, and excessive activation of the renin-angiotensin-aldosterone system (RAAS) (1). Medical studies have shown that DKD has the main clinical symptoms of hypertension and proteinuria, along with gradually declining kidney function (2). Typical morphological alterations in DKD involve mesangial cell proliferation, podocyte loss, glomerular hypertrophy, glomerular sclerosis, tubular injury, interstitial fibrosis, and thickening of the basement membrane. Overall, DKD shows high heterogeneity in the clinical symptoms, histopathological changes, and progression rate; thus, making it difficult to formulate efficient treatment methods.
The thioredoxin-interacting protein (TXNIP) has received a lot of attention in recent years because it has a wide range of activities and influences many metabolic processes. Many studies have suggested that TXNIP plays an important role in the onset and progression of diabetes, and TXNIP epigenetic regulation is linked to diabetes prevalence (3, 4). Studies on mice have suggested that TXNIP deficiency can enhance glucose homeostasis while ameliorating insulin resistance (5). Furthermore, anti-diabetic medications, such as glucagon-like peptide-1 (GLP-1) and metformin, can enhance glucose homeostasis by regulating TXNIP (6, 7). Moreover, several experts have elucidated the mechanism of TXNIP in the progression of diabetes and its role in the treatment of diabetes (8). Additionally, TXNIP expression increases in the kidneys of diabetic patients and model rodents and is closely related to changes in kidney morphology and function (9–14). However, the effect of TXNIP on the pathogenic mechanism of DKD is rarely generalized. In this study, we summarized the information regarding TXNIP biology and emphasized its effect on DKD to facilitate further studies. The studies on TXNIP and DKD have been summarized in Table 1.
General information on TXNIP
TXNIP, also referred to as Vitamin D3-upregulated protein 1 (VDUP-1), was first cloned and characterized in 1994 in HELA cells stimulated with Vitamin D3 (25). Subsequently, TXNIP was identified as a thioredoxin (TRX)-binding protein using the yeast two-hybrid system, which can suppress the expression and activity of TRX, and thus, it was called thioredoxin-binding protein-2 (TBP-2) (26). Studies on the TXNIP promoter could not identify the consensus vitamin-D3 response element. Moreover, vitamin-D3-mediated TXNIP transcription was not verified in other cells (27). A nonsense mutation in the TBP-2 gene was discovered in another study on HcB-19 mice, causing the mice to develop familial combined hyperlipidemia; thus, VDUP1/TBP-2 was called TXNIP (28). The human TXNIP gene is located on chromosome 1q21.1 and has eight introns and eight exons that cover 4,174 bp. Its nucleotide sequences are highly homologous to those of mouse (89%) and zebrafish (77%), suggesting that the functions of TXNIP are essential (25). The human TXNIP protein is a member of the α-arrestin protein family and contains 391 amino acids with a molecular weight (MW) of 46 kDa. TXNIP has an arrestin-like C-terminus (175–298 aa) as well as an arrestin-like N-terminus (10–152 aa) (29). There are two intramolecular disulfide bonds between Cys-247 and Cys-63 in TXNIP, which may help TXNIP to efficiently interact with TRX and inhibit its activity (26).
TXNIP expression in the kidney of diabetic patients
In 2009, Andrew Advani first reported TXNIP in normal humans via in situ hybridization. TXNIP was expressed in the renal arteriole endothelium, glomeruli, collecting ducts, and distal convoluted tubules. Additionally, the researchers conducted qRT-PCR using renal biopsy samples. They extracted the mRNA from DKD cases, as well as from healthy renal tissues collected from the contralateral side of tumor cases receiving nephrectomy. Their results suggested that TXNIP was upregulated in patients with DKD (9). In 2018, Yachun Han found that TXNIP levels were significantly higher in the renal tissues of DKD patients compared to those of healthy controls by performing immunohistochemistry (IHC) and semi-quantification (19). In another study, IHC analysis showed that TXNIP expression was significantly higher in the glomeruli of patients with DKD relative to its expression in the glomeruli of normal controls (21). Despite the fact that these studies consistently showed high renal expression of TXNIP in DKD patients, the number of cases in the studies was small. Therefore, a large number of kidney samples from DKD patients must be collected for further research. Furthermore, serum TXNIP levels in diabetics and DKD patients were significantly higher than in healthy volunteers (30). Although some studies on serum TXNIP levels in patients with diabetes have yielded consistent results (31, 32), there is insufficient evidence on whether it can be used as a serum marker in patients with diabetes or DKD. Furthermore, another study has revealed that TXNIP expression was higher in the urinary sediment in type 1 diabetics with DKD, which was related to a reduction in the estimated glomerular filtration rate (eGFR) levels (33). These findings suggest that TXNIP expression in serum, urine, and kidney is significantly higher in DKD patients and that TXNIP may play an important role in the progression of DKD. Therefore, more research into the signal transduction role of TXNIP in DKD progression is critical.
TXNIP signaling in DKD
ROS-TRX-TXNIP-NLRP3 pathway
Reactive oxygen species (ROS) are generated in mammalian cells to mediate different physiological responses, such as cell growth, invasion, and differentiation. Oxidative stress (OS) is a major pathophysiological mechanism linked to a variety of diseases, including diabetes and DKD. TXNIP is a key mediator of the high glucose (HG)-induced OS. TXNIP and TRX active site thiols can form mixed disulfide bonds, which inhibit TRX activity while increasing ROS production (34). Mitochondria is the main (90%) ROS source in cells (35). Additionally, an increase in the production of mitochondrial ROS (mtROS) in the renal tissues of DKD cases and diabetic model rodents had a strong effect on the occurrence of DKD (19). mtROS is the main factor that activates the NLRP3 inflammasome (36). Additionally, TXNIP is related to activating the NLRP3 inflammasome in DKD (19, 22, 24). When TXNIP is released from TRX, it can bind to NLRP3 via a leucine-rich repeat domain, activating the NLRP3 inflammasome in response to mtROS stimulation. The activated NLRP3 can then cleave interleukin-1β (IL-1β) and interleukin-18 (IL-18) precursors, inducing an immunoinflammatory response in the kidneys of diabetics. MitoQ (a mitochondria-targeted antioxidant)-induced reduction in mtROS expression in the renal tissues of db/db mice and HK-2 cells can mitigate the activation of the TXNIP-NLRP3 inflammasome pathway, thereby alleviating renal cell inflammation, fibrosis, and apoptosis (19). Besides being produced in the mitochondria, cellular ROS can also be produced via the NADPH oxidase (Nox) system in the diabetic state. There are three Nox isoforms identified within renal tissues, including Nox1, Nox2, and Nox4; the isoform(s) that can induce ROS during diabetes is undetermined (37). However, Nox4 downregulation was shown to suppress the dissociation of TXNIP from TRX and inhibit the NLRP3 inflammasome from being activated in a DKD mouse model constructed by administering a high-fat diet (HFD), as well as injecting streptozotocin (STZ) intraperitoneally (22). Therefore, mtROS and Nox-mediated ROS generation significantly affect the TXNIP-NLRP3 inflammasome axis. TXNIP can modulate HG-mediated TRX activity impairment, whereas silencing TXNIP can abolish HG-mediated collagen generation and OS in diabetic kidneys (9). In a recent study, Shah et al. demonstrated DKD resistance in diabetic TXNIP−/−mice, a reduction in ROS production, Nox4 level, inflammation, and tubulointerstitial fibrosis. The renal function was preserved compared to the levels of these factors/activities in diabetic TXNIP+/+ mice (16). Wang et al. obtained similar results in TXNIP knockout diabetic mice (38). These results indicated the important effect of TXNIP on intracellular ROS production and NLRP3 inflammasome activation, which play key roles in the process of DKD.
FOXO1-TXNIP
Forkhead transcription factor O1 (FOXO1) belongs to the forkhead box-containing transcription factor O family. FOXO1 can mediate OS within endothelial cells, renal proximal tubular cells (RPTCs), and pancreatic β-cells along with TRX and TXNIP (20, 39). Linlin et al. discovered that by binding to the promoters of TRX and TXNIP, FOXO1 reduced albuminuria, ROS generation, interstitial fibrosis, and RPTC apoptosis in HFD and STZ-induced diabetic mice and HG-treated RPTCs. Furthermore, it modulated ROS levels by increasing TRX expression while decreasing TXNIP expression (20). Our previous studies found that the FOXO1-TXNIP-NLRP3 inflammasome pathway was activated in RPTCs through ATP stimulation, resulting in the secretion of IL-1β (40). Although in-depth studies of this intracellular signaling pathway in the DKD state are lacking, it has been demonstrated that FOXO1 can suppress NLRP3 inflammasome activation by reducing the TXNIP level in patients with diabetic atherosclerosis and diabetic liver (41, 42). There is increasing evidence that FOXO1 plays an important role in the pathogenesis of DKD through the regulation of autophagy, apoptosis, and other cellular processes, which is independent of TXNIP (43). Therefore, FOXO1 may be another potential target for preventing and treating DKD.
TXNIP and autophagy
Autophagy is responsible for transporting cytoplasmic contents into lysosomes to maintain homeostasis and cell functions. Apoptosis changes can cause cell injury and even cell death. In the case of diabetes-related metabolic changes or hyperglycemia, injured organelles and proteins accumulate, which is linked to the development of DKD. Autophagy changes in RPTCs and podocytes during diabetes (44). Microtubule-associated protein 1A/1B light chain 3B (LC3) is an autophagy marker that shows specific localization in autophagic structures during autophagy between phagophore and lysosomal degeneration. The LC3-I/LC3-II ratio (LC3 conversion) can be easily determined because LC3-II content is tightly associated with the quantity of autophagosome (45). The degradation of p62 is another important marker for monitoring autophagic activity since p62 can directly bind to LC3 and is selectively degraded through autophagy (46). TXNIP is an autophagy regulator in diabetes. Downregulation of TXNIP restores LC3-II and p62 protein levels and reduces autophagic flux in the HG-exposed rat retinal Müller cells (rMC-1). Thus, TXNIP downregulation can improve the visual function of diabetic rats (47). Chunling et al. were the first to report the upregulation of p62, TXNIP, and LC3/LC3-II in DKD mouse RPTCs and the formation of autophagic vacuoles in human RPTCs treated with HG. TXNIP silencing reduced p62 and LC3-II levels, as well as autophagic vacuoles, in HG-treated human RPTCs (15). Later, Chunling et al. also showed the dysfunction of tubular autophagy in renal tissues of DKD rats and patients. Their results suggested that the abnormal p62 and LC3 levels were normalized in the renal tissues of the TXNIP DNAzyme-treated diabetic rats. Additionally, HG dysregulated tubular mitophagy in RPTCs in vitro, and the effect was reversed by TXNIP siRNA, which inhibited mammalian target of rapamycin (mTOR) (17), an important factor related to the key regulatory mechanisms of autophagy. Based on the above studies, we hypothesized that balance autophagy by regulating TXNIP might be a prospective treatment for DKD in clinical.
TXNIP and lipid
Lipometabolic disorder can cause ectopic renal lipid deposition in individuals with diabetes. Renal lipid concentrations, measured by MRI, are considerably higher in diabetics compared to that non-diabetic subjects (48). Furthermore, based on tissue staining results, the aberrant lipid droplets deposited in the interstitium, mesangial cells, and renal glomeruli suggest impairment of renal morphology and function, particularly in the renal tubules of diabetics (49, 50). Recent studies have suggested the key effect of TXNIP on lipid metabolism during diabetes (51). Chunyang et al. reported that TXNIP knockdown suppressed fatty acid (FA) production and promoted FA oxidation to mitigate lipid accumulation in HG-treated RPTCs and renal tissues of STZ-induced diabetic mice. This was partially achieved due to the activation of the mTOR pathway (18). Additionally, we found TXNIP knockdown can suppress cholesterol synthesis and uptake to mitigate lipid accumulation in the RPTCs of db/db mice, thus, improving the morphology and function of renal tubules (23). Though there have been few studies on the effects of TXNIP on diabetic renal lipid metabolism, it is critical to further investigate the lipid regulatory function of TXNIP. In the future, TXNIP could be used to treat renal lipid disorders.
Conclusion and future perspective
Over the years, significant advances have been made in TXNIP biology and its role in metabolic regulation. As mentioned above, TXNIP plays a role in renal oxidative stress, inflammation, autophagy, and lipid metabolism during DKD and may be a key regulatory factor for the pathogenic mechanism and development of DKD (Figure 1). However, more research is needed to fully understand the mechanism underlying TXNIP’s physiological activities in DKD, and targeting TXNIP may offer a unique treatment strategy to combat the global DKD problem.
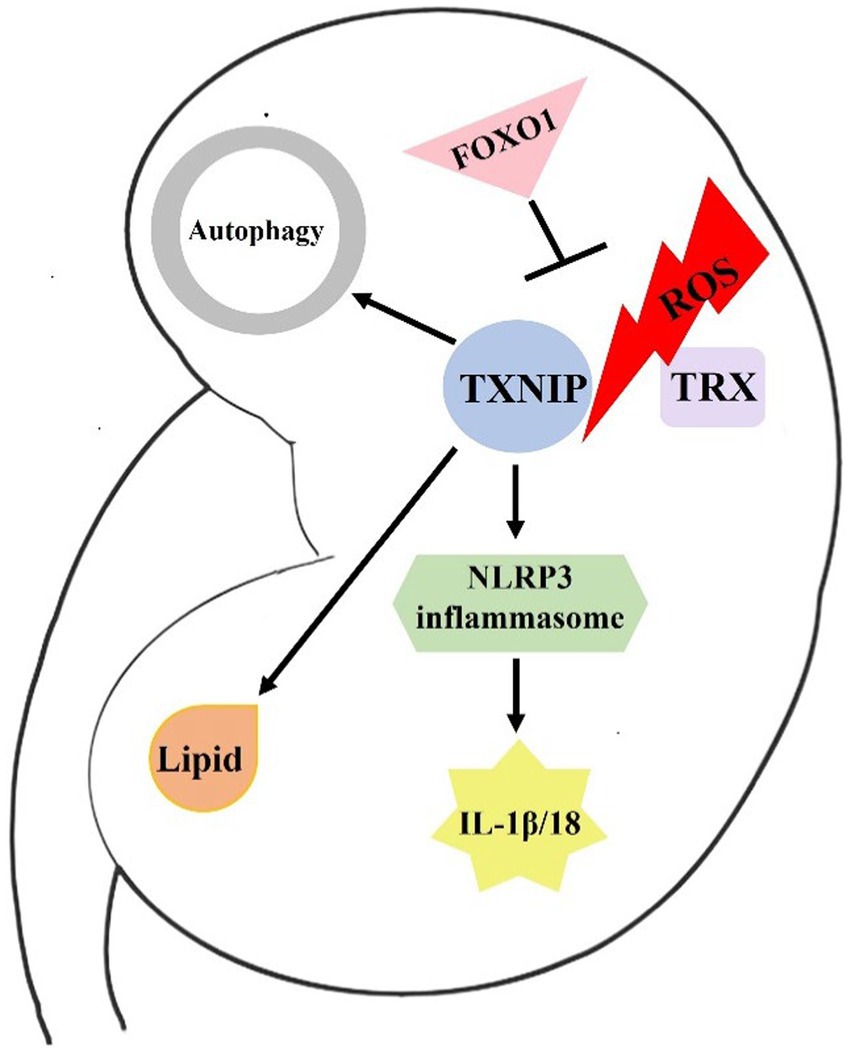
Figure 1. Brief mechanism of TXNIP in DKD. In response to ROS stimulation, TXNIP is released from TRX. Then, it binds to NLRP3 and activates the NLRP3 inflammasome. The activated NLRP3 can then cleave IL-1β and IL-18 precursors, inducing an immunoinflammatory response in the kidneys of diabetics. FOXO1 may ameliorate DKD by affecting ROS generation and TXNIP-TRX pathway. In addition, TXNIP also damages renal cell autophagy and lipid metabolism, resulting in the development of DKD.
Author contributions
HS designed the article and wrote the first draft. RS and YH searched the literature. XS and QL revised the manuscript. All authors read and approved the final manuscript.
Funding
This work was supported by the National Natural Science Foundation of China (grant no. 82270755 to HS), Suzhou Science and Technology Project (grant no. SYS2020104, SZM2022013 to HS and SZM2021013 to XS).
Acknowledgments
The authors would like to thank the Key Talents Stratified by Gusu Health Talent Program.
Conflict of interest
The authors declare that the research was conducted in the absence of any commercial or financial relationships that could be construed as a potential conflict of interest.
Publisher’s note
All claims expressed in this article are solely those of the authors and do not necessarily represent those of their affiliated organizations, or those of the publisher, the editors and the reviewers. Any product that may be evaluated in this article, or claim that may be made by its manufacturer, is not guaranteed or endorsed by the publisher.
References
1. Thomas, MC, Brownlee, M, Susztak, K, Sharma, K, Jandeleit-Dahm, KA, Zoungas, S, et al. Diabetic kidney disease. Nat Rev Dis Primers. (2015) 1:15018. doi: 10.1038/nrdp.2015.18
2. Sugahara, M, Pak, WLW, Tanaka, T, Tang, SCW, and Nangaku, M. Update on diagnosis, pathophysiology, and management of diabetic kidney disease. Nephrology (Carlton). (2021) 26:491–500. doi: 10.1111/nep.13860
3. Zhang, D, Cheng, C, Cao, M, Wang, T, Chen, X, Zhao, Y, et al. TXNIP hypomethylation and its interaction with obesity and hypertriglyceridemia increase type 2 diabetes mellitus risk: a nested case-control study. J Diabetes. (2020) 12:512–20. doi: 10.1111/1753-0407.13021
4. Liang, T, Wu, Z, Du, S, and Hu, L. TXNIP gene single nucleotide polymorphisms associated with the risk of type 2 diabetes mellitus in a Chinese Han population. DNA Cell Biol. (2020) 39:1513–20. doi: 10.1089/dna.2020.5592
5. Thielen, LA, Chen, J, Jing, G, Moukha-Chafiq, O, Xu, G, Jo, S, et al. Identification of an anti-diabetic, orally available small molecule that regulates TXNIP expression and glucagon action. Cell Metab. (2020) 32:353–365.e8 e8. Epub 2020/07/30. doi: 10.1016/j.cmet.2020.07.002
6. Chai, TF, Hong, SY, He, H, Zheng, L, Hagen, T, Luo, Y, et al. A potential mechanism of metformin-mediated regulation of glucose homeostasis: inhibition of Thioredoxin-interacting protein (Txnip) gene expression. Cell Signal. (2012) 24:1700–5. doi: 10.1016/j.cellsig.2012.04.017
7. Zhou, W, Shao, W, Zhang, Y, Liu, D, Liu, M, and Jin, T. Glucagon-like peptide-1 receptor mediates the beneficial effect of liraglutide in an acute lung injury mouse model involving the thioredoxin-interacting protein. Am J Physiol Endocrinol Metab. (2020) 319:E568–78. doi: 10.1152/ajpendo.00292.2020
8. Thielen, L, and Shalev, A. Diabetes pathogenic mechanisms and potential new therapies based upon a novel target called TXNIP. Curr Opin Endocrinol Diabetes Obes. (2018) 25:75–80. doi: 10.1097/MED.0000000000000391
9. Advani, A, Gilbert, RE, Thai, K, Gow, RM, Langham, RG, Cox, AJ, et al. Expression, localization, and function of the thioredoxin system in diabetic nephropathy. J Am Soc Nephrol. (2009) 20:730–41. doi: 10.1681/ASN.2008020142
10. Almomen, SM, Guan, Q, Liang, P, Yang, K, Sidiqi, AM, Levin, A, et al. Daily intake of grape powder prevents the progression of kidney disease in obese type 2 diabetic ZSF1 rats. Nutrients. (2017) 9:345. doi: 10.3390/nu9040345
11. Tan, SM, Zhang, Y, Cox, AJ, Kelly, DJ, and Qi, W. Tranilast attenuates the up-regulation of thioredoxin-interacting protein and oxidative stress in an experimental model of diabetic nephropathy. Nephrol Dial Transplant. (2011) 26:100–10. doi: 10.1093/ndt/gfq355
12. Siddiqi, FS, Majumder, S, Thai, K, Abdalla, M, Hu, P, Advani, SL, et al. The histone methyltransferase enzyme enhancer of Zeste homolog 2 protects against podocyte oxidative stress and renal injury in diabetes. J Am Soc Nephrol. (2016) 27:2021–34. doi: 10.1681/ASN.2014090898
13. De Marinis, Y, Cai, M, Bompada, P, Atac, D, Kotova, O, Johansson, ME, et al. Epigenetic regulation of the thioredoxin-interacting protein (TXNIP) gene by hyperglycemia in kidney. Kidney Int. (2016) 89:342–53. doi: 10.1016/j.kint.2015.12.018
14. Samra, YA, Said, HS, Elsherbiny, NM, Liou, GI, El-Shishtawy, MM, and Eissa, LA. Cepharanthine and Piperine ameliorate diabetic nephropathy in rats: role of NF-kappa B and NLRP3 inflammasome. Life Sci. (2016) 157:187–99. doi: 10.1016/j.lfs.2016.06.002
15. Huang, C, Lin, MZ, Cheng, D, Braet, F, Pollock, CA, and Chen, XM. Thioredoxin-interacting protein mediates dysfunction of tubular autophagy in diabetic kidneys through inhibiting autophagic flux. Lab Investig. (2014) 94:309–20. doi: 10.1038/labinvest.2014.2
16. Shah, A, Xia, L, Masson, EA, Gui, C, Momen, A, Shikatani, EA, et al. Thioredoxin-interacting protein deficiency protects against diabetic nephropathy. J Am Soc Nephrol. (2015) 26:2963–77. doi: 10.1681/ASN.2014050528
17. Huang, C, Zhang, Y, Kelly, DJ, Tan, CY, Gill, A, Cheng, D, et al. Thioredoxin interacting protein (TXNIP) regulates tubular autophagy and mitophagy in diabetic nephropathy through the mTOR signaling pathway. Sci Rep. (2016) 6:29196. Epub 2016/07/07. doi: 10.1038/srep29196
18. Du, C, Wu, M, Liu, H, Ren, Y, Du, Y, Wu, H, et al. Thioredoxin-interacting protein regulates lipid metabolism via Akt/mTOR pathway in diabetic kidney disease. Int J Biochem Cell Biol. (2016) 79:1–13. doi: 10.1016/j.biocel.2016.08.006
19. Han, Y, Xu, X, Tang, C, Gao, P, Chen, X, Xiong, X, et al. Reactive oxygen species promote tubular injury in diabetic nephropathy: the role of the mitochondrial ros-txnip-nlrp 3 biological axis. Redox Biol. (2018) 16:32–46. doi: 10.1016/j.redox.2018.02.013
20. Ji, L, Wang, Q, Huang, F, An, T, Guo, F, Zhao, Y, et al. FOXO1 overexpression attenuates Tubulointerstitial fibrosis and apoptosis in diabetic kidneys by ameliorating oxidative injury via TXNIP-TRX. Oxidative Med Cell Longev. (2019) 2019:1–14. doi: 10.1155/2019/3286928
21. Song, S, Qiu, D, Shi, Y, Wang, S, Zhou, X, Chen, N, et al. Thioredoxin-interacting protein deficiency alleviates phenotypic alterations of podocytes via inhibition of mTOR activation in diabetic nephropathy. J Cell Physiol. (2019) 234:16485–502. doi: 10.1002/jcp.28317
22. An, X, Zhang, Y, Cao, Y, Chen, J, Qin, H, and Yang, L. Punicalagin protects diabetic nephropathy by inhibiting Pyroptosis based on TXNIP/NLRP3 pathway. Nutrients. (2020) 12:1516. doi: 10.3390/nu12051516
23. Sun, H, Chen, J, Sun, L, Shi, B, and Li, J. Role of Thioredoxin-interacting protein in diabetic fatty kidney induced by advanced glycation end-products. J Agric Food Chem. (2021) 69:11982–91. doi: 10.1021/acs.jafc.1c03559
24. Eisa, NH, Khodir, AE, El-Sherbiny, M, Elsherbiny, NM, and Said, E. Phenethyl isothiocyanate attenuates diabetic nephropathy via modulation of glycative/oxidative/inflammatory signaling in diabetic rats. Biomed Pharmacother. (2021) 142:111666. doi: 10.1016/j.biopha.2021.111666
25. Chen, KS, and DeLuca, HF. Isolation and characterization of a novel cDNA from HL-60 cells treated with 1, 25-dihydroxyvitamin D-3. Biochim Biophys Acta. (1994) 1219:26–32. doi: 10.1016/0167-4781(94)90242-9
26. Nishiyama, A, Matsui, M, Iwata, S, Hirota, K, Masutani, H, Nakamura, H, et al. Identification of thioredoxin-binding protein-2/vitamin D (3) up-regulated protein 1 as a negative regulator of thioredoxin function and expression. J Biol Chem. (1999) 274:21645–50. doi: 10.1074/jbc.274.31.21645
27. Ludwig, DL, Kotanides, H, Le, T, Chavkin, D, Bohlen, P, and Witte, L. Cloning, genetic characterization, and chromosomal mapping of the mouse VDUP1 gene. Gene. (2001) 269:103–12. doi: 10.1016/s0378-1119(01)00455-3
28. Bodnar, JS, Chatterjee, A, Castellani, LW, Ross, DA, Ohmen, J, Cavalcoli, J, et al. Positional cloning of the combined hyperlipidemia gene Hyplip 1. Nat Genet. (2002) 30:110–6. doi: 10.1038/ng811
29. Patwari, P, Higgins, LJ, Chutkow, WA, Yoshioka, J, and Lee, RT. The interaction of thioredoxin with Txnip. Evidence for formation of a mixed disulfide by disulfide exchange. J Biol Chem. (2006) 281:21884–91. doi: 10.1074/jbc.M600427200
30. Xu, LL, Gao, W, Chen, ZM, Shao, KK, Wang, YG, Cui, L-L, et al. Relationships between diabetic nephropathy and insulin resistance, inflammation, Trx, Txnip, Cys C and serum complement levels. Eur Rev Med Pharmacol Sci. (2020) 24:11700–6. doi: 10.26355/eurrev_202011_23815
31. Chu, Y, Widjaja, J, Hong, J, Dolo, PR, Zhu, X, and Yao, L. Effect of sleeve gastrectomy on plasma Thioredoxin-interacting protein (TXNIP). Obes Surg. (2021) 31:4829–35. doi: 10.1007/s11695-021-05649-7
32. Guo, Y, Chen, J, Liu, N, Liu, Z, Shi, B, and Sun, H. Association of Circulating TXNIP levels with fatty liver in newly diagnosed type 2 diabetes mellitus. Diabetes Metab Syndr Obes. (2022) 15:225–33. doi: 10.2147/DMSO.S349153
33. Monteiro, MB, Santos-Bezerra, DP, Thieme, K, Admoni, SN, Perez, RV, Machado, CG, et al. Thioredoxin interacting protein expression in the urinary sediment associates with renal function decline in type 1 diabetes. Free Radic Res. (2016) 50:101–10. doi: 10.3109/10715762.2015.1109083
34. Lee, S, Kim, SM, and Lee, RT. Thioredoxin and thioredoxin target proteins: from molecular mechanisms to functional significance. Antioxid Redox Signal. (2013) 18:1165–207. doi: 10.1089/ars.2011.4322
35. Kowaltowski, AJ, de Souza-Pinto, NC, Castilho, RF, and Vercesi, AE. Mitochondria and reactive oxygen species. Free Radic Biol Med. (2009) 47:333–43. doi: 10.1016/j.freeradbiomed.2009.05.004
36. Kelley, N, Jeltema, D, Duan, Y, and He, Y. The NLRP3 Inflammasome: An overview of mechanisms of activation and regulation. Int J Mol Sci. (2019) 20:3328. doi: 10.3390/ijms20133328
37. Gorin, Y, and Block, K. Nox as a target for diabetic complications. Clin Sci (Lond). (2013) 125:361–82. doi: 10.1042/CS20130065
38. Wang, W, Wu, QH, Sui, Y, Wang, Y, and Qiu, X. Rutin protects endothelial dysfunction by disturbing Nox 4 and ROS-sensitive NLRP3 inflammasome. Biomed Pharmacother. (2017) 86:32–40. doi: 10.1016/j.biopha.2016.11.134
39. Kibbe, C, Chen, J, Xu, G, Jing, G, and Shalev, A. FOXO1 competes with carbohydrate response element-binding protein (ChREBP) and inhibits thioredoxin-interacting protein (TXNIP) transcription in pancreatic beta cells. J Biol Chem. (2013) 288:23194–202. doi: 10.1074/jbc.M113.473082
40. Sun, H, Sun, Z, Varghese, Z, Guo, Y, Moorhead, JF, Unwin, RJ, et al. Nonesterified free fatty acids enhance the inflammatory response in renal tubules by inducing extracellular ATP release. Am J Physiol Renal Physiol. (2020) 319:F292–303. doi: 10.1152/ajprenal.00098.2020
41. Kim, DH, Kim, SM, Lee, B, Lee, EK, Chung, KW, Moon, KM, et al. Effect of betaine on hepatic insulin resistance through FOXO1-induced NLRP3 inflammasome. J Nutr Biochem. (2017) 45:104–14. doi: 10.1016/j.jnutbio.2017.04.014
42. Nyandwi, JB, Ko, YS, Jin, H, Yun, SP, Park, SW, and Kim, HJ. Rosmarinic acid inhibits ox LDL-induced inflammasome activation under high-glucose conditions through downregulating the p 38-FOXO1-TXNIP pathway. Biochem Pharmacol. (2020) 182:114246. doi: 10.1016/j.bcp.2020.114246
43. Wang, Y, and He, W. Improving the dysregulation of fox O1 activity is a potential therapy for alleviating diabetic kidney disease. Front Pharmacol. (2021) 12:630617. doi: 10.3389/fphar.2021.630617
44. Gonzalez, CD, Carro Negueruela, MP, Nicora Santamarina, C, Resnik, R, and Vaccaro, MI. Autophagy dysregulation in diabetic kidney disease: from pathophysiology to pharmacological interventions. Cells. (2021) 10:2497. doi: 10.3390/cells10092497
45. Mizushima, N, and Yoshimori, T. How to interpret LC3 immunoblotting. Autophagy. (2007) 3:542–5. doi: 10.4161/auto.4600
46. Yoshii, SR, and Mizushima, N. Monitoring and measuring autophagy. Int J Mol Sci. (2017) 18:1865. doi: 10.3390/ijms18091865
47. Ao, H, Li, H, Zhao, X, Liu, B, and Lu, L. TXNIP positively regulates the autophagy and apoptosis in the rat muller cell of diabetic retinopathy. Life Sci. (2021) 267:118988. doi: 10.1016/j.lfs.2020.118988
48. Yokoo, T, Clark, HR, Pedrosa, I, Yuan, Q, Dimitrov, I, Zhang, Y, et al. Quantification of renal steatosis in type II diabetes mellitus using Dixon-based MRI. J Magn Reson Imaging. (2016) 44:1312–9. doi: 10.1002/jmri.25252
49. de Vries, AP, Ruggenenti, P, Ruan, XZ, Praga, M, Cruzado, JM, Bajema, IM, et al. Fatty kidney: emerging role of ectopic lipid in obesity-related renal disease [research support, non-U.S. Gov't review]. Lancet Diabet Endocrinol. (2014) 2:417–26. doi: 10.1016/S2213-8587(14)70065-8
50. Herman-Edelstein, M, Scherzer, P, Tobar, A, Levi, M, and Gafter, U. Altered renal lipid metabolism and renal lipid accumulation in human diabetic nephropathy. J Lipid Res. (2014) 55:561–72. doi: 10.1194/jlr.P040501
Keywords: diabetic kidney disease, thioredoxin-interacting protein, reactive oxygen species, autophagy, lipid
Citation: Sun H, Sun R, Hua Y, Lu Q and Shao X (2023) An update on the role of thioredoxin-interacting protein in diabetic kidney disease: A mini review. Front. Med. 10:1153805. doi: 10.3389/fmed.2023.1153805
Edited by:
Yimn Niu, Southeast University, ChinaReviewed by:
Xueli Zhang, Southeast University, ChinaRanjan Das, Oregon Health and Science University, United States
Copyright © 2023 Sun, Sun, Hua, Lu and Shao. This is an open-access article distributed under the terms of the Creative Commons Attribution License (CC BY). The use, distribution or reproduction in other forums is permitted, provided the original author(s) and the copyright owner(s) are credited and that the original publication in this journal is cited, in accordance with accepted academic practice. No use, distribution or reproduction is permitted which does not comply with these terms.
*Correspondence: Xinyu Shao, YnJlbnRvQDEyNi5jb20=; Qianyi Lu, bHVxeTg1QDE2My5jb20=