- 1School of Heath Preservation and Rehabilitation, Chengdu University of Traditional Chinese Medicine, Chengdu, China
- 2School of Preclinical Medicine, Chengdu University, Chengdu, China
Background: Chronic rhinosinusitis (CRS) is a clinical syndrome primarily characterized by long-term mucosal inflammation of the nasal cavity and sinuses. The pathogenesis of CRS is still unclear due to its high heterogeneity. A number of studies have recently focused on the sinonasal epithelium. Thus, there has been a quantum leap in awareness of the role of the sinonasal epithelium, which is now understood as an active functional organ rather than simply an inert mechanical barrier. Undoubtedly, epithelial dysfunction plays a vital role in the onset and development of CRS.
Objective: In this article, we discuss the potential contribution of sinonasal epithelium dysfunction to CRS pathogenesis and explore a few current and developing therapeutic options targeting the sinonasal epithelium.
Results: Impaired mucociliary clearance (MCC) and an abnormal sinonasal epithelial barrier are usually considered to be the main causative factors in CRS. Epithelial-derived bioactive substances, such as cytokines, exosomes, and complements, play a vital role in the regulation of innate and adaptive immunity and contribute to the pathophysiological alterations of CRS. The phenomena of epithelial–mesenchymal transition (EMT), mucosal remodeling, and autophagy observed in CRS offer some novel insights into the pathogenesis of this disease. In addition, existing treatment options targeting disorder of sinonasal epithelium can help to relieve the main symptoms associated with CRS to some extent.
Conclusion: The presence of a normal epithelium is fundamental for maintaining homeostasis in the nasal and paranasal sinuses. Here, we describe various aspects of the sinonasal epithelium and highlight the contributions of epithelial dysfunction to CRS pathogenesis. Our review provides sound evidence of the need for in-depth study of the pathophysiological alterations of this disease and for the development of novel epithelium-targeting alternative treatments.
Introduction
Chronic rhinosinusitis (CRS), which causes local inflammation of the nasal mucosa and paranasal sinuses, is mainly characterized by symptoms of congestion, stuffiness, facial pressure, and hyposmia, with a duration of at least 3 months. This disease affects ~10% of the global population and can significantly impair their quality of life (1, 2). Based on the presence or absence of nasal polyps (NPs), CRS has been classically divided into two major subgroups, namely, CRS with nasal polyps (CRSwNP) and CRS without nasal polyps (CRSnNP) (3). Moreover, based on the cytokine production patterns of canonical T-cells, recent studies have described three distinct endotypes of patients, as follows: type 1, regulated by Th1 cytokine IFN-γ; type 2, mediated by Th2 cytokines IL-4, IL-5, IL-13, and IL-9; and type 3, controlled by Th17 cytokines IL-17 and IL-22 (4, 5). Thus, given the significant variation found in the presence and extent of eosinophilia in the sinuses, CRS has been classified into two subsets, namely, idiopathic eosinophilic CRS (E-CRS) and non-eosinophilic CRS (NE-CRS). It has been shown that CRS patients with severe tissue eosinophilia usually display severe symptoms and experience poorer treatment outcomes (6).
As CRS is a highly heterogeneous disease, its pathogenesis has not yet been well-elucidated. A number of factors have been found to be associated with the onset and development of CRS, including geographical and racial factors, environmental exposures, sinus microbiota dysbiosis, mucociliary clearance (MCC) dysfunction, epithelial barrier impairment, and disrupted immune response (1, 2, 7, 8). The epithelium is the first line of defense for the nasal mucosa. It has been established that the MCC system and cell–cell contact form the fundamental mechanical barrier. When the epithelial barrier of the sinuses is damaged, its appearance changes substantially, from that of an inert physical barrier to that of an active functional organ (9) that can secrete a wide variety of bioactive substances, such as cytokines and exosomes, as well as complement proteins, and thus facilitates the recruitment and regulation of different immune cells (10–12). Essentially, the sinonasal epithelium plays a key role in both innate and adaptive immunity. However, abnormal capability of the epithelium can have a significant impact on the onset and development of CRS. Epithelial-to-mesenchymal transition (EMT), mucosal remodeling, and autophagy have been commonly observed in CRS, presenting new insight into sinonasal epithelium malfunction; these phenomena might have some connection with the pathogenesis of this disease.
The optimal treatment of CRS has undoubtedly been found to be complicated due to its multifactorial pathogenesis. However, administration of topical or systemic corticosteroids plays a fundamental role in medical treatment of CRS (13). Nasal saline irrigation is an effective adjunct to treatment for relieving the various symptoms of CRS (14). Failure of medical management can often lead patients to undergo surgery. However, this option does not provide effective prevention of relapse of the disease (15). Recently, the effectiveness of biological treatment has been demonstrated, and biologics have emerged as good options for CRS therapy (16). Despite the presence of a wide variety of therapies for this disease, there is still a sizable proportion of patients with CRS who display poor treatment outcomes, which can be mainly attributed to its intricate pathogenesis. In this article, we provide an elegant summary of the contributions of altered epithelial functions to the pathogenesis of CRS and discuss the possible influence of the current treatment regime on the sinonasal epithelium.
Roles of epithelial dysfunction in the development of CRS
Barrier dysfunction
The physical-mechanical barrier consists of tight junctions (TJs), adherens junctions, gap junctions, desmosomes, and hemidesmosomes; these components facilitate strong cell–cell contact, form a tight barrier, establish cell polarity, and can effectively regulate the mobility of different ions and molecules (17). TJs consist of zonula occludens-1 (ZO-1), occludin, and claudins, as well as junctional adhesion molecule 1 (JAM-1) proteins (18). Adherens junctions contain the transmembrane proteins E-cadherin and nectin and the intracellular proteins α- and β-catenin (18). Desmosomal attachments consist of the heterotypic binding of desmoglein (DSG1, DSG2) (19).
Interestingly, some studies have observed significantly decreased expression of ZO-1, occludin, claudin-1, JAM-1, DSG1, and DSG2 in the primary epithelial cells of CRS patients as compared with those of healthy controls (20–22). Transepithelial electrical resistance (TER) is one of the indicators reflecting the function of the epithelial barrier (23). Patients with CRSwNP exhibit reduced TER, which could be associated with epithelial barrier damage (21). In patients with E-CRSwNP, E-cadherin expression is markedly downregulated (24). In addition, changes in the sinus microenvironment can also cause barrier damage. CRSwNP is often characterized by type 2 inflammation with high levels of Th2 cytokines, including IL-4 and IL-13 (25). CRSnNP is associated with high levels of Th1 cytokines such as IFN-γ; however, it is noteworthy that IFN-γ has been observed to be highly expressed in NE-CRSwNP (26). Elevation of IL-4, IL-13, and IFN-γ in CRSwNP can cause the downregulation of TJ proteins and reduction of TER, thus leading to epithelial disruption (21, 27, 28).
The interaction of the airway epithelial cells with airborne environmental pollution and bacterial toxins is critical in the impairment of epithelial barrier function. A study on house dust mites (HDMs) has indicated that the compromised epithelial barrier function caused by HDMs is associated with lower expression of both occludin and ZO-1 proteins (29). Moreover, another study has reported that the cigarette smoke extract (CSE) stimulation causes global disruption of the epithelial junctional proteins ZO-1 and JAM-A, along with an associated decrease in TER levels (22). Particulate matter (PM) generated by human activities could be very harmful to the health of the respiratory mucosa. According to in vitro studies, exposure of human nasal epithelial cells to PM with a diameter of <2.5 μm can lead to significantly decreased expression of various TJ proteins (claudin-1, occludin, and ZO-1), increased paracellular permeability, and decreased TER, and to an increase in the production of pro-inflammatory cytokines IL-8 and thymic stromal lymphopoietin (TSLP), which can significantly damage the integrity and function of the epithelial barrier (30, 31). Moreover, exposure to PM2.5 can increase levels of intracellular reactive oxygen species (ROS) and the nuclear translocation of NF-E2-related factor-2 (Nrf2), induce oxidative stress and inflammatory responses, and aggravate existing damage to the nasal epithelial barrier (32). In addition, bacterial infections caused by Staphylococcus aureus have been detected in airways with compromised barrier function. For instance, one study found that S. aureus enterotoxin B (SEB) can activate toll-like receptor 2 (TLR2) and stimulate the subsequent release of the pro-inflammatory cytokines IL-6 and IL-8, which can cause epithelial cells to reduce expression of both occludin and ZO-1 and increase mucosal permeability (33).
When the integrity of the epithelium is destroyed, epithelial permeability can effectively increase. Invading exogenous irritants can thus activate the immune cells and thereby initiate an immune response (34). Selected studies highlighting the destruction of CRS epithelial integrity are summarized in Table 1; selected studies highlighting the various factors affecting epithelial barrier function are summarized in Table 2.
Mucociliary dysfunction
The mucociliary clearance (MCC) system consists of three major functional components, namely, cilia activity, the airway surface liquid (ASL) layer, and mucin secretion. Ciliated epithelial cells mainly function to clear away the various pathogens and inhaled irritants trapped by the airway surface liquid and mucus. A number of prior studies have reported that CRS is related to impairment of MCC, the degree of this impairment being correlated with the severity of CRS (11, 35). Patients with CRS often have a local infection in the nasal and paranasal cavities. In previous studies, bacteria such as Haemophilus influenzae, Streptococcus pneumoniae, S. aureus, Aspergillus fumagatus, and P. aeruginosa have been demonstrated to release different toxins that can effectively destroy ciliated epithelial cells (36). Interestingly, two distinct parameters, namely, ciliary beating frequency (CBF) and ciliary bend distance (CBD), can potentially be used to evaluate cilia transport capacity. ASL combined with coordinated ciliary beating is a critical component of the mucociliary clearance apparatus. Moreover, studies have found that CBD, CBF, and ASL are regulated by CI−. Cl− secretion of the nasal epithelial cells has been found to be significantly reduced in patients with CRS, thus damaging the function of the cilia as well as the ASL and increasing the viscosity of mucus (37–39). Mucin 5AC (MUC5AC), produced by the goblet cells of the airway epithelium, is a major constituent of airway mucus. Epidermal growth factor (EGF) has been found to upregulate MUC5AC expression in mucus secreted by patients with CRS through transmembrane protein 16A (TMEM16A) (40, 41). Similarly, major basic protein (MBP) in the airway mucus can effectively disrupt exogenous pathogens and infected cells. Moreover, researchers have found that the mucus of patients with CRS may exhibit higher expression of MBP in comparison to that of healthy people. Notably, excessive expression of MBP harms the normal host cells and intensifies inflammation of the nasal mucosa (42). Mucociliary dysfunction can weaken protection of the nasal mucosa and has been found to be conducive to bacterial colonization, biofilm formation, and mucositis, which is a non-negligible factor in CRS pathogenesis.
Roles of epithelial-derived bioactive substances
Cytokines
Considering the elevation of canonical T-cell cytokines in this condition, recent studies have described three endotypes of CRS: type 1 is mainly associated with an increase in Th1 cytokines IFN-γ and TNF-α; type 2 is associated with an increase in Th2 cytokines IL-4, IL-5, and IL-13; and type 3 is related to the Th17 cytokines IL-17 and IL-22 (4, 5).
When exposed to various foreign irritants, nasal epithelial cells can secrete a variety of inflammatory cytokines, such as TNF-α, TSLP, IL-25, IL-33, and IL-6 (43). TNF-α is a Th1 cytokine and can promote the release of IL-6, IL-10, and IFN-γ. It is generally believed that CRSsNP mainly involves Th1-type inflammation with high expression of IFN-γ (44). In addition, pro-inflammatory cytokines such as TNF-α and IFN-γ have been reported to induce necroptosis in CRSwNP, which in turn can stimulate the production and release of pro-inflammatory cytokines and the recruitment of neutrophils, thus leading to exacerbation of the inflammation. TNF-α and IFN-γ may act in conjunction, which can cause a vicious cycle of necroptosis and inflammation (45).
Epithelial-derived cytokines TSLP, IL-25, and IL-33 can promote the production of different Th2 cytokines, such as IL-4, IL-5, and IL-13, by activating Th2 cells as well as group 2 innate lymphoid cells (ILC2s), thus further inducing type 2 inflammatory responses (46). A number of studies have shown that the nasal polyp tissues of patients with CRSwNP accumulate more dendritic cells (DCs) expressing IL-17Rb, ST2, and TSLPR than the tissues of control individuals. DCs are activated in response to IL-25, IL-33, and TSLP and can then initiate Th2 reactions (47). Moreover, some recent studies have provided evidence that increased expression of IL-25, IL-33, and TSLP could be correlated with severe tissue eosinophilia, especially in the nasal polyp tissues of patients with CRSwNP (48, 49).
Interleukin-6 is a crucial regulator of inflammation, plays a role in activating B-cells, and can promote the recruitment of neutrophils (50). In addition, IL-6 and TGF-β have been found to induce juvenile T-cells to differentiate effectively into Th17 cells to produce the type 3 cytokine IL-17 (51). IL-6 is highly expressed in CRSwNP tissues (50). After epithelial damage, IL-6 can promote sinonasal epithelial cell proliferation. It has been demonstrated in vitro that IL-6 can facilitate repair of the nasal epithelial cells of patients with CRSwNP. However, IL-6 may also cause the epithelium to overreact to external stimuli and contribute to the formation of nasal polyps (52) (Figure 1).
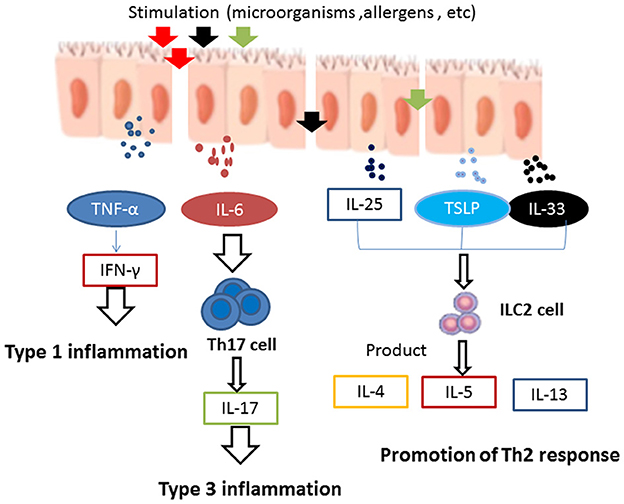
Figure 1. When exposed to foreign irritants, nasal epithelial cells secrete a wide variety of inflammatory cytokines, such as TNF-α, TSLP, IL-25, IL-33, and IL-6. TNF-α can promote the release of IFN-γ. It is generally believed that CRSsNP mainly involves Th1-type inflammation and high expression of IFN-γ. Epithelial-derived cytokines, such as TSLP, IL-25, and IL-33, promote the Th2 response in the development of innate lymphoid cells (ILCs) and induce the production of Th2 cytokines, such as IL-4, IL-5, and IL-13, which can further enhance type 2 inflammation. IL-6 induces the differentiation of naive T-cells into Th17 cells and produces the type 3 cytokine IL-17, which is involved in type 3 inflammation.
Exosomes
Exosomes are spherical to cup-shaped vesicles, 30–150 nm in size, that contain proteins as well as genetic materials (such as miRNA, mRNA, and DNA); they can be secreted by all types of cells (53, 54). Nasal exosomes can be sampled from the nasal mucus or nasal lavage fluid (55). It has been reported that exosomes might be involved in the local pathophysiological process and immune response in CRS. Nitric oxide (NO) is well-known to play a critical role in the immune protection of airway epithelial cells. Inhaled pathogens can activate toll-like receptor 4 (TLR4) on the nasal epithelial cells, thus resulting in the doubling of basal exosome secretion rates. These exosomes carry nearly twice the level of nitric oxide synthase 2 (NOS2) found in unstimulated exosomes and can further enhance the antibacterial ability of the nasal epithelium via an increase in local NO content (56). Nasal mucus-derived exosomes have been found to contain various defensive proteins that can aid in strengthening the immune defensive barrier function of the sinonasal mucosa. In addition, studies have found that mucus-derived exosomes rich in P-glycoprotein (P-gp) can transfer P-gp between epithelial cells, causing type 2 helper T-cell inflammation (57). Nasal exosome proteomic analysis has revealed that exosomes display a strong association with the immune function of the nasal mucosa. A variety of immune cells, such as monocytes, NK cells, and neutrophils, can be attracted to the nasal cavity by exosomes (58). Ganglioside GD3, found on the surface of nasal mucus-derived exosomes, has the capacity to prevent T-cell receptor (TCR)-mediated T-cell activation and plays a key role in immunosuppression (59). Interestingly, a study involving proteomic analysis of the nasal mucus exosome in patients with CRSwNP has indicated that the unique bioimprints of cystatin-SN, peroxidase-5, and glycoprotein VI in the exosomes of CRSwNP nasal mucus could facilitate accurate prediction of the occurrence of this disease (60). In addition, cystatin levels in exosomes may be predictive of CRS phenotype, CRS severity, and early recurrences (61, 62). Exosomes have also been found to be associated with nasal polyp formation. A study of proteins in tissues and mucus-derived exosomes has indicated that coagulation-related tissue proteins, including fibronectin and fibrinogen gamma chains, are the most overexpressed, and fibrinolytic pathway-related proteins, including plasminase and tissue plasminogen activator, are generally downregulated in CRSwNP tissues. These observations suggest that the coagulation pathway is significantly disordered within the nasal polyp tissue: specifically, the coagulation cascade is upregulated and the fibrinolysis cascade is downregulated, thus leading to retention of plasma proteins, intense edema, excessive fibrin deposition in nasal polyps, and subsequent tissue remodeling. In addition, the expression of plasmin and coagulin in exosomes is significantly negatively correlated with their expression in the tissues, possibly due to the depletion of the cellular proteins after packaging and release into exosomes (63).
Other mediators
Complement expression and activity are tightly regulated to avoid immune dysregulation and host tissue damage. Both inefficient activation and overstimulation of complement have been found to be associated with increased susceptibility to infection and inflammation (64). In addition, previous studies have found that patients with CRS exhibit various complement defects (65). Patients with CRSwNP have a higher level of serum complement component 3 (C3) than patients with CRSnNP, suggesting that serum C3 may be involved in the pathogenesis of NPs. The level of serum C3 in post-operative recurrent CRSwNP patients can increase to the pre-operative level, supporting the notion that a high level of serum C3 might be considered an independent factor by which patients at high risk of recurrence can be pinpointed. Post-operative change in C3 levels might have the potential to function as a predictor of nasal polyp progression (66). The cleavage products C3a and C3b of C3 derived from the nasal epithelial cells can act to dilate the blood vessels, increase vascular permeability, promote histamine release, function as chemoattractants, activate eosinophils, regulate Th2 inflammation, and are closely related to the onset of CRSwNP (67).
Vitamin D3 (VD3) is a steroid hormone with significant antiproliferative properties (68). The dysregulation of 1α-hydroxylase and vitamin D in the sinuses in CRS has been reported previously (69). Moreover, 1α-hydroxylase can convert the inactive form of 25(OH)D3 into an active form, 1,25(OH)2D3. Thus, the reduction of sinonasal 1α-hydroxylase and 1,25(OH)2D3 in patients with CRSwNP has been found to be associated with poorer SNOT22 scores and with disease severity (70). In addition, in preclinical experiments in mice, AD3 deficiency has been found to cause changes in sinonasal immunity, resulting in increased infiltration of total inflammatory cells in the nasal cavity, including upregulation of eosinophils, neutrophils, and lymphocytes and selective exacerbation of inflammation (71). Although VD3 deficiency has been found to be associated with more severe disease, a clinical trial has demonstrated that supplementation of VD3 in patients with CRS does not significantly improve patient outcomes (72). In addition, recent studies have identified a link between complement activation and VD3. Under various stimuli, C3 and C3a might be released, which can inhibit 1α-hydroxylase expression and suppress the conversion of 25(OH)D3 to 1,25(OH)2D3, thus explaining the limited benefits of oral VD3 treatment (73).
Epithelial-to-mesenchymal transition
The process by which adherent epithelial cells are converted to migratory cells invading the extracellular matrix is known collectively as EMT (74). It can occur in both physiological and pathological conditions, participating in several biological processes such as embryogenesis, wound healing, inflammation, fibrosis, and tumor metastasis (75). The phenomenon of EMT has been observed in patients with CRS and there is also evidence that it affects patients with CRSwNP. For instance, a recent study has indicated that the macrophage-derived mucin domain 4 (TIM-4) can facilitate nasal polyp formation by promoting transforming growth factor-β (TGF-β1)-mediated EMT in the nasal epithelial cells (76). It has also been discovered that TGF-β1 can induce the process of EMT via modulation of various microRNAs, such as microRNA-182 and microRNA-21, in CRSwNP, with attendant downregulation of the epithelial marker E-cadherin and upregulation of the mesenchymal marker vimentin and of N-cadherin (77, 78).
Interestingly, further findings have revealed that abnormal expression of EMT-related markers can be observed to a greater extent in the epithelial tissues of patients with E-CRSwNP in comparison to those obtained from patients with NE-CRSwNP, suggesting that the process of EMT might be related to the acceleration of nasal polyp formation in patients with E-CRSwNP (24). Canonical Wnt signaling has been demonstrated to be activated in nasal polyps, triggering epithelial disorders such as compromised adherens junctions and reduced ciliogenesis, which can in turn stimulate cytokine release (79). Furthermore, some studies have found that elevated expression of the various Wnt-related proteins, including nuclear β-catenin, WNT3A, and cyclin D1, in nasal polyp tissues could be accompanied by the overexpression of mesenchymal markers, thus suggesting that Wnt signaling might be involved in the pathogenesis of nasal polyp formation via stimulation of the activation of EMT (80).
Mucosal remodeling
Remodeling of the nasal mucosa in CRS primarily takes the form of epithelial injury, basement membrane thickening (BMT), epithelial goblet cell metaplasia, mucinous gland hyperplasia, extracellular matrix (ECM) collagen deposition, and so on. Mucosal remodeling is found not only in CRSwNP but also in CRSsNP (81, 82), and E-CRS has been found to be associated with strong nasal mucosal eosinophilic infiltration (83). Evidence suggests that both eosinophilia and eosinophil activation could be closely associated with CRS remodeling and mucosal damage (84). In addition, an in vitro study has found that eosinophil-derived neurotoxin (EDN) degranulation from eosinophils activated by IL-5 can effectively promote the secretion of matrix metalloproteinase 9 (MMP-9) from the nasal epithelium, thereby affecting epithelial regeneration and breakdown of ECM, and ultimately leading to nasal remodeling (85). ECM proteins are also involved in the maintenance of physical scaffolding, homeostasis, and regulation of inflammation of the airway mucosa (86). Periostin, an ECM protein, is induced by IL-4 as well as IL-13 and is secreted by airway epithelial cells (87). Interestingly, studies involving nasal mucosal biopsies of CRS have shown that periosteal proteins can cause eosinophilic infiltration and mediate fibrosis, thereby participating in mucosal remodeling (88). TGF-β is a pleiotropic and multifunctional growth factor that can induce fibroblast proliferation, differentiation, and fibrotic characteristics. Moreover, it can also stimulate the production of tissue inhibitors of metalloproteinase 1 (TIMP-1), thereby preventing the enzymatic breakdown of ECM (89). It has been reported that there is a significant difference between CRSsNP and CRSwNP in terms of TGF-β levels, with higher levels detected in CRSsNP along with thicker collagen fibers in the extracellular matrix, leading to excessive tissue repair as well as the formation of fibrosis. In contrast, TGF-β is absent in CRSwNP and tissue repair is compromised, which is reflected by the presence of loose connective tissue and edema formation in the severely inflamed tissues (90).
Autophagy
Autophagy is a lysosomal degradation pathway that is essential for cell survival, differentiation, and homeostasis. Intracellular cargo is delivered to the lysosomes and further degraded through the formation of autophagosomes (91–93). It has been established that, for protection against airborne PM2.5, smoke, and other harmful stimuli, epithelial cells may endocytose small amounts of harmful substances and trigger autophagy in airway epithelial cells, thereby increasing mucus secretion (94). However, if the induction of autophagy is insufficient to reset the cellular state to normal levels, it will further develop or even aggravate CRS (91). In the case of human neutrophil elastase (HNE)-induced CRS, studies have found that human neutrophil elastase can promote autophagy through TNF receptor-associated factor 6 (TRAF6), thus resulting in hyperexpression of MUC5AC in CRSwNP (95). Autophagy has been found to increase the secretion of MUC5AC by promoting the phosphorylation of c-Jun N-terminal kinase (JNK) and c-Jun (96). Another study of eosinophilic and non-eosinophilic nasal polyps has found that the reduction of autophagy is negatively correlated with the severity of eosinophilic inflammation and the extent of tissue remodeling (97). Other evidence suggests that autophagy in the myeloid cells, particularly macrophages, could be disrupted by the deletion of autophagy-related gene 7 (Atg7) and could further induce eosinophilic inflammation and the Th2 response, resulting in eosinophilia, epithelial hyperplasia, and mucosal thickening in E-CRS mice (98).
Impact of therapeutic approaches on the sinonasal epithelium
It has been established above that the pathogenesis of CRS is intricate and multifactorial. For this reason, a large proportion of patients with CRS exhibit limited therapeutic benefits. As the defensive barrier of the nasal mucosa, dysfunction of the epithelium has vital significance for CRS pathophysiology. The impact of traditional and developing therapeutic approaches on the sinonasal epithelium is discussed in this section.
Corticosteroids
Corticosteroids have well-known anti-inflammatory effects and generally suppress adaptive responses and enhance innate responses (99). The medical treatment of CRS mainly involves the application of topical, intranasal, and oral corticosteroids (100). Corticosteroids predominantly act on the glucocorticoid receptors (GCRs) distributed in sinonasal epithelial cells and exert powerful anti-inflammatory effects (101). To date, four distinct isoforms of GCR, namely, GCRα, GCRβ, GCRδ, and GCRγ, have been identified. The active isoform GCRα, after combining with corticosteroids, can bind with DNA and affect the transcription of various genes associated with inflammation, thus inhibiting the inflammatory process (102). However, variable expression of all the GCR isoforms can compromise the efficacy of corticosteroid treatment. A number of prior studies have confirmed that corticosteroid treatment can promote epithelial repair, reduce eosinophil infiltration, and affect collagen deposition, thus playing an important role in modulating mucosal remodeling (103, 104). Corticosteroids can also suppress eosinophils and Th2 cells but are relatively less effective on neutrophils and the Th17 subset (105). Intranasal corticosteroids are considered the first-line medical treatment for CRS patients after surgery. In contrast to a simple nasal spray, the use of corticosteroids delivered via nasal irrigation for the management of CRS is supported by a higher level of scientific evidence. For instance, an RCT in patients after sinus surgery showed that corticosteroids delivered via nasal irrigation can significantly improve inflammatory symptoms, such as nasal congestion and nasal discharge, and Lund-Mackay score (101). In addition, another retrospective study has demonstrated that long-term, daily intranasal corticosteroid treatment does not cause intraocular pressure, subcapsular cataracts, or other adverse effects (106). A meta-analysis has shown that, compared to placebo treatment or no treatment, the administration of a short course of oral steroids as an adjunct therapy to intranasal corticosteroids can effectively alleviate CRS symptoms, improve the microenvironment of the sinuses, and reduce the size of nasal polyps (107). The exhalation delivery system with fluticasone (EDS-FLU), a new tool for treating CRS, employs an “exhalation delivery” mechanism, exploiting balanced closure of the soft palate to deliver corticosteroids widely in order to reach key anatomical sites in the superior and posterior aspects of the nasal cavity (108). Interestingly, an RCT comparing EDS-FLU with EDS-placebo in patients with CRSwNP showed that EDS-FLU significantly improves nasal obstruction, facial pain, rhinorrhea, and hyposmia; reduces polyp size; and improves sinonasal symptoms and quality of life, while adverse events are similar to those occurring with other intranasal steroids, with the most common being epistaxis (109).
Nasal irrigation
Nasal irrigation is regarded as an effective treatment adjunct and primarily functions to dilute the mucus, remove infective pathogens and inflammatory mediators, reduce edema of the sinonasal mucosa as well as antigen load, and improve mucociliary clearance capacity (110). The various drugs used in current nasal irrigation treatments include corticosteroids, saline, and traditional Chinese medicine (111, 112). For post-operative patients with CRS, topical steroid irrigation is especially recommended for its effectiveness in relieving various inflammatory symptoms, especially nasal congestion (106). For instance, one study has shown that isotonic saline irrigation can eliminate the antimicrobial peptides secreted by sinonasal epithelium, thus weakening the innate defensive capability of the nasal mucosa. However, low-salt-solution rinses might contribute to the secretion of various antimicrobial components (113). A meta-analysis on saline irrigation indicates that hypertonic saline, in contrast to isotonic saline, can enhance the TER levels of sinonasal epithelial cells to a remarkable degree and increase ciliary movement, thereby indicating that hypertonic saline rinses should be able to bolster the barrier function of the sinonasal mucosa (14). However, an in vitro study has reported that hypertonic solution rinses do substantial harm to sinus epithelial cells (114); nevertheless, detrimental effects of hypertonic solution rinses in patients have not been reported. Nasal irrigation with traditional Chinese medicine has gained widespread attention in recent years; this has unique advantages in the prevention and treatment of CRS. A number of studies have found that local nasal irrigation with traditional Chinese medicine can effectively reduce mucosal edema and promote the recovery of the structure and motor function of cilia with high levels of safety and no obvious adverse reactions (112). Honey has antibacterial and anti-inflammatory properties; thyme and honey nasal spray has been demonstrated to reduce inflammation and polyp formation as well as promoting mucosal healing in patients with CRS after surgery (115).
Sinus surgery
Surgery is often recommended for patients experiencing failure of medical treatment. Endoscopic sinus surgery (ESS) has emerged as an optimal choice among current surgical interventions (105). A study involving face-to-face interviews with patients with CRSwNP who had undergone at least one ESS found that these patients tend to focus on the symptoms that caused their quality of life (QOL) to decline. Headaches and nasal congestion appear to impact the QoL of these patients. The most common rhinological symptom is decreased sense of smell/taste, which is a major source of distress, suggesting that clinicians should discuss CRS patients' goals and expectations for ESS with them before surgery (116). In addition, patients with CRS also suffer from poor sleep quality and reduced QOL. A meta-analysis has found that patients with CRS who undergo ESS exhibit significantly improved Pittsburgh Sleep Quality Index (PSQI) scores and QOL, although there is no significant improvement on several objective indices, including total sleep time, sleep latency, and awakenings after sleep onset (117, 118). Moreover, studies have found that the functions of the cilia and epithelial cells of patients with CRS exhibit some improvement 6 months after ESS, whereas MCC function shows some improvement 3 months after ESS (119, 120). ESS could also relieve obstruction of the nasal airway and sinus ostium, debride inflamed tissue, reestablish MCC, and provide suitable access for topical medication (105).
Failure of surgery for CRS is typically defined as the persistence or recurrence of symptoms, which often necessitates revision surgery. According to a study of 29,934 patients followed for 9.7 years, the average time between the first and second surgeries is 4.39 years, and the average time between the fourth and fifth surgeries decreases to 2.18 years (121). The average time between operations decreases as the number of revision surgeries increases. Risk factors for revision sinus surgery include phenotype, comorbidities, and surgical approach (120). For example, DeConde et al. found that polyp recurrence is common after ESS with control of polyps for up to 18 months, occurring in ~60–70% of patients with CRSwNP (122). A retrospective study of 424 adult patients with CRS receiving ESS revealed that, in an analysis by CRS subtype, revision ESS rates were 5.1% and 3.5% for CRSwNP and CRSsNP, respectively (123). Patients with CRSwNP are more likely to undergo multiple revision surgeries. Cytokine profile analysis has shown that the levels of Th17-associated mediators (IL-8, IL-17A, and IL-23), B-cell activating factor (BAFF), and Th1 cytokine (INF-γ) are significantly upregulated in the NPs of recurrence patients with CRSwNP in comparison to controls and a primary NP group (124). A meta-analysis has also shown that patients with CRSwNP who have aspirin-aggravated respiratory disease (AERD) exhibit a higher revision rate of 27.2% in comparison to various other types of patients with CRSwNP (125). In another study, Gill et al. followed 33,090 patients (7,693 with CRS with asthma (CRS-A) and 25,397 with CRS only) for 9.8 years and found that the rate of revision ESS in CRS-A was double that of patients with CRS alone; patients with CRS-A and nasal polyposis are 6 times more likely to require revision than those with CRS alone (126).
Monoclonal biological therapies
Chronic rhinosinusitis with nasal polyps (CRSwNP) frequently remains uncontrolled despite the administration of available therapies and sinonasal surgery. Biological therapies targeting specific antagonists of pro-inflammatory mechanisms appear to be promising for personalized treatment of the inflammatory disease CRS (127). For instance, IL-5 is a potent stimulator of eosinophilic growth as well as proliferation, and the concentration of IL-5 is significantly higher in polyp tissues. A phase 3 trial of mepolizumab, an anti-IL-5 biologic, in the treatment of CRSwNP found that, after administration of mepolizumab subcutaneously at a dose of 100 mg every 4 weeks for 52 weeks (in addition to primary care), total endoscopic nasal polyp scores and nasal obstruction VAS scores significantly improved, indicating marked improvement in nasal polyp size and a reduction in polyp recurrence (128). The epithelial-derived cytokine TSLP plays a key role in inflammatory signaling and the response of congenital lymphoid cells. It can activate the Th2 response and is associated with the pathogenesis of asthma (129). Tezepelumab, a monoclonal antibody targeting TSLP, received approval in 2021 for the treatment of asthma, chronic obstructive pulmonary disease (COPD), and CRSwNP (130). In a phase 2 clinical trial, tezepelumab administered subcutaneously at 210 mg every 4 weeks for a planned 28 weeks was found to significantly reduce eosinophil counts and decrease the frequency of asthma exacerbations; it therefore represents an effective add-on treatment option for patients with CRS-A (131). Dupilumab is a monoclonal antibody that can inhibit signaling of IL-4 and IL-13, two cytokines central to Th2-mediated inflammation. In a prospective study of patients with CRSwNP who had AERD, dupilumab (at a dose of 300 mg administered subcutaneously every 2 weeks for 24 weeks, in addition to standardized treatment) was found to significantly improve scores on the SNOT-22, the asthma control test (ACT), and the Mini Asthma Quality of Life Questionnaire (AQLQ), as well as improving sinus opacification and markers of T2 inflammation. It can therefore be used as an effective therapy for these patients (132). Moreover, another RCT has demonstrated a significantly reduced polyp burden after 16 weeks of weekly subcutaneous injection of dupilumab 300 mg compared with the use of mometasone nasal spray alone (133). When epithelial cells are re-exposed to an allergen, IgE can bind to the surface receptors of both the mast cells and basophils, thus triggering degranulation of inflammatory mediators (histamine, prostate, leukotrienes, etc.) and producing various nasal symptoms (134). Omalizumab, an anti-IgE biologic agent, can produce broad therapeutic effects in patients with CRSwNP, regardless of whether they have undergone surgery, suffer from asthma, or have aspirin sensitivity (135). It can significantly improve the symptoms of nasal obstruction, runny nose, and so on in patients with CRSwNP who have an insufficient intranasal steroid response (136). The efficacy and safety of omalizumab could be extended to 1 year (137). IL-33 is involved in promoting the process of TH2 differentiation and is a key inflammatory cytokine that can mediate eosinophilic infiltration (138). Etokimab, a monoclonal antibody targeting IL-33, will be evaluated for use in patients with CRSwNP in an upcoming phase 2 controlled trial (139). These drugs offer a novel opportunity to directly target the inflammatory pathway of patients with CRS once clear biomarkers have been identified. Thus, it is possible that, once the endotypes of CRS are more thoroughly understood, personalized treatment can be further refined for the benefit of patients.
Conclusion
Chronic rhinosinusitis is a multifactorial disease with limited treatment options. Epithelial disorder is pivotal in the etiology or pathogenesis of CRS; epithelial barrier disruption and MCC dysfunction constitute the fundamental pathogenesis of CRS. EMT, mucosal remodeling, and autophagy can affect the sinonasal epithelium and thus can also participate in the genesis of CRS. Epithelium-derived bioactive substances such as cytokines, exosomes, and complements can play an important role in the development and progression of this disease by modulating both innate and adaptive immune responses. VD3 deficiency has been found to be associated with more severe disease, but supplementation does not significantly improve patient outcomes. In terms of treatment, glucocorticoids can effectively alleviate nasal symptoms through anti-inflammatory activities, delay the onset of remodeling, and alter the course of the disease. Nasal irrigation can reduce edema by diluting the mucus and removing inflammatory mediators. Biological agents antagonize specific targets of pro-inflammatory mechanisms, thus relieving nasal symptoms and reducing polyp size. Post-operative sinus symptoms can be improved significantly in this way, and polyp burden and edema can both be relieved. In practice, however, no treatment modalities have yet been found to be successful in restoring the mucosa to its prior physiological state. Thus, novel strategies should be developed to restore the homeostasis of the nasal mucosal epithelium for treatment of CRS. Overall, considering the importance of sinonasal epithelium-mediated immunity disorders for CRS pathogenesis, vigorous efforts should be applied to the development of novel biological agents that can antagonize the actions of inflammatory bioactive components produced by the epithelium, maintain the immune balance of the nasal mucosa, alleviate local inflammation, and reduce nasal polyp formation.
Author contributions
YH and YF were participated in drafting. YW to supplement. TZ and HL read the manuscript and gave some opinions. All authors read and approved the final manuscript.
Funding
This research was supported by the Natural Science Foundation of China (81674037) and the Sichuan Science and Technology Program (2022YFS0421).
Conflict of interest
The authors declare that the research was conducted in the absence of any commercial or financial relationships that could be construed as a potential conflict of interest.
Publisher's note
All claims expressed in this article are solely those of the authors and do not necessarily represent those of their affiliated organizations, or those of the publisher, the editors and the reviewers. Any product that may be evaluated in this article, or claim that may be made by its manufacturer, is not guaranteed or endorsed by the publisher.
References
1. Jiao J, Wang CS, Zhang L. Epithelial physical barrier defects in chronic rhinosinusitis. Expert Rev Clin Immu. (2019) 15:679–88. doi: 10.1080/1744666X.2019.1601556
2. Schleimer RP. Immunopathogenesis of chronic rhinosinusitis and nasal polyposis. Annu Rev Pathol. (2017) 24:331–57. doi: 10.1146/annurev-pathol-052016-100401
3. Lam K, Schleimer R, Kern RC. The etiology and pathogenesis of chronic rhinosinusitis: a review of current hypotheses. Curr Allergy Asthma Rep. (2015) 15:41. doi: 10.1007/s11882-015-0540-2
4. Han XY, Wu DW, Sun ZF, Sun HL, Lv QW, Zhang LC, et al. Type 1/type 2 inflammatory cytokines correlate with olfactory function in patients with chronic rhinosinusitis. Am J Otolaryng. (2020) 41:102587. doi: 10.1016/j.amjoto.2020.102587
5. Wang M, Zhang N, Zheng M, Li Y, Meng L, Ruan Y, et al. Cross-talk between T(H)2 and T(H)17 pathways in patients with chronic rhinosinusitis with nasal polyps. J Allergy Clin Immunol. (2019) 144:1254–64. doi: 10.1016/j.jaci.2019.06.023
6. Fujieda S, Imoto Y, Kato Y, Ninomiya T, Tokunaga T, Tsutsumiuchi T, et al. Eosinophilic chronic rhinosinusitis. Allergol Int. (2019) 68:403–12. doi: 10.1016/j.alit.2019.07.002
7. Gohy ST, Hupin C, Pilette C, Ladjemi MZ. Chronic inflammatory airway diseases: the central role of the epithelium revisited. Clin Exp Allergy. (2016) 46:529–42. doi: 10.1111/cea.12712
8. Carlier FM, de Fays C, Pilette C. Epithelial barrier dysfunction in chronic respiratory diseases. Front Physiol. (2021) 12:691227. doi: 10.3389/fphys.2021.691227
9. Gohy S, Hupin C, Ladjemi MZ, Hox V, Pilette C. Key role of the epithelium in chronic upper airways diseases. Clin Exp Allergy. (2020) 50:135–46. doi: 10.1111/cea.13539
10. Tsukita K, Yano T, Tamura A, Tsukita S. Reciprocal association between the apical junctional complex and ampk: a promising therapeutic target for epithelial/endothelial barrier function? Int J Mol Sci. (2019) 20:6012. doi: 10.3390/ijms20236012
11. Mall MA. Role of cilia, mucus, and airway surface liquid in mucociliary dysfunction: lessons from mouse models. J Aerosol Med Pulmon Drug Delivery. (2008) 21:13–24. doi: 10.1089/jamp.2007.0659
12. Nur Husna SM, Tan HT, Md Shukri N, Mohd Ashari NS, Wong KK. Nasal epithelial barrier integrity and tight junctions disruption in allergic rhinitis: overview and pathogenic insights. Front Immunol. (2021) 12:663626. doi: 10.3389/fimmu.2021.663626
13. Zhang YY, Lou HF, Wang Y, Li Y, Zhang L, Wang CS. Comparison of corticosteroids by 3 approaches to the treatment of chronic rhinosinusitis with nasal polyps. Allergy Asthma Immun. (2019) 11:482–97. doi: 10.4168/aair.2019.11.4.482
14. Liu L, Pan M, Li Y, Tan G, Yang Y. Efficacy of nasal irrigation with hypertonic saline on chronic rhinosinusitis: systematic review and meta-analysis. Braz J Otorhinolaryngol. (2020) 86:639–46. doi: 10.1016/j.bjorl.2020.03.008
15. Zhang L, Zhang Y, Gao YB, Wang KJ, Lou HF, Meng YF, et al. Long-term outcomes of different endoscopic sinus surgery in recurrent chronic rhinosinusitis with nasal polyps and asthma. Rhinology. (2020) 58:126–35. doi: 10.4193/Rhin19.184
16. Kim C, Han J, Wu T, Bachert C, Fokkens W, Hellings P, et al. Role of biologics in chronic rhinosinusitis with nasal polyposis: state of the art review. Otolaryngol Head Neck Surg. (2021) 164:57–66. doi: 10.1177/0194599820939964
17. Angulo-Urarte A, van der Wal T, Huveneers S. Cell-cell junctions as sensors and transducers of mechanical forces. Biochim Biophys Acta Biomembranes. (2020) 1862:183316. doi: 10.1016/j.bbamem.2020.183316
18. Hartsock A, Nelson WJ. Adherens and tight junctions: structure, function and connections to the actin cytoskeleton. Biochim Biophys Acta. (2008) 1778:660–9. doi: 10.1016/j.bbamem.2007.07.012
19. Kasperkiewicz M, Ellebrecht CT, Takahashi H, Yamagami J, Zillikens D, Payne AS, et al. Pemphigus. Nat Rev Dis Primers. (2017) 3:17026. doi: 10.1038/nrdp.2017.26
20. Li Y, Wang X, Wang R, Bo M, Fan E, Duan S, et al. The expression of epithelial intercellular junctional proteins in the sinonasal tissue of subjects with chronic rhinosinusitis: a histopathologic study. ORL. (2014) 76:110–9. doi: 10.1159/000362246
21. Soyka MB, Wawrzyniak P, Eiwegger T, Holzmann D, Treis A, Wanke K, et al. Defective epithelial barrier in chronic rhinosinusitis: the regulation of tight junctions by IFN-γ and IL-4. J Allergy Clin Immunol. (2012) 130:1087–96.e10. doi: 10.1016/j.jaci.2012.05.052
22. Tharakan A, Halderman AA, Lane AP, Biswal S, Ramanathan M, Jr. Reversal of cigarette smoke extract-induced sinonasal epithelial cell barrier dysfunction through Nrf2 activation. Int Forum Allergy Rhinol. (2016) 6:1145–50. doi: 10.1002/alr.21827
23. Sun X, Yang Q, Rogers CJ, Du M, Zhu MJ. AMPK improves gut epithelial differentiation and barrier function via regulating Cdx2 expression. Cell Death Differ. (2017) 24:819–31. doi: 10.1038/cdd.2017.14
24. Wang M, Sun Y, Li C, Qu J, Zhou B. Eosinophils correlate with epithelial-mesenchymal transition in chronic rhinosinusitis with nasal polyps. ORL. (2022) 84:70–80. doi: 10.1159/000516847
25. Poposki JA, Klingler AI, Tan BK, Soroosh P, Banie H, Lewis G, et al. Group 2 innate lymphoid cells are elevated and activated in chronic rhinosinusitis with nasal polyps. Immunity Inflamm Dis. (2017) 5:233–43. doi: 10.1002/iid3.161
26. Kim DK, Jin HR, Eun KM, Mutusamy S, Cho SH, Oh S, et al. Non-eosinophilic nasal polyps shows increased epithelial proliferation and localized disease pattern in the early stage. PLoS ONE. (2015) 10:e0139945. doi: 10.1371/journal.pone.0139945
27. Jiao J, Duan S, Meng N, Li Y, Fan E, Zhang L. Role of IFN-γ, IL-13, and IL-17 on mucociliary differentiation of nasal epithelial cells in chronic rhinosinusitis with nasal polyps. Clin Exp Allergy. (2016) 46:449–60. doi: 10.1111/cea.12644
28. Dickinson JD, Alevy Y, Malvin NP, Patel KK, Gunsten SP, Holtzman MJ, et al. IL13 activates autophagy to regulate secretion in airway epithelial cells. Autophagy. (2016) 12:397–409. doi: 10.1080/15548627.2015.1056967
29. Steelant B, Farré R, Wawrzyniak P, Belmans J, Dekimpe E, Vanheel H, et al. Impaired barrier function in patients with house dust mite-induced allergic rhinitis is accompanied by decreased occludin and zonula occludens-1 expression. J Allergy Clin Immunol. (2016) 137:1043–53.e5. doi: 10.1016/j.jaci.2015.10.050
30. Xian M, Ma S, Wang K, Lou H, Wang Y, Zhang L, et al. Particulate matter 2.5 causes deficiency in barrier integrity in human nasal epithelial cells. Allergy Asthma Immunol Res. (2020) 12:56–71. doi: 10.4168/aair.2020.12.1.56
31. Zhao R, Guo Z, Zhang R, Deng C, Xu J, Dong W, et al. Nasal epithelial barrier disruption by particulate matter ≤ 2.5 μm via tight junction protein degradation. J Appl Toxicol. (2018) 38:678–87. doi: 10.1002/jat.3573
32. Hong Z, Guo Z, Zhang R, Xu J, Dong W, Zhuang G, et al. Airborne fine particulate matter induces oxidative stress and inflammation in human nasal epithelial cells. Tohoku J Exp Med. (2016) 239:117–25. doi: 10.1620/tjem.239.117
33. Martens K, Seys SF, Alpizar YA, Schrijvers R, Bullens DMA, Breynaert C, et al. Staphylococcus aureus enterotoxin B disrupts nasal epithelial barrier integrity. Clin Exp Allergy. (2021) 51:87–98. doi: 10.1111/cea.13760
34. Gon Y, Hashimoto S. Role of airway epithelial barrier dysfunction in pathogenesis of asthma. Allergol Int. (2018) 67:12–7. doi: 10.1016/j.alit.2017.08.011
35. Whitsett JA. Airway epithelial differentiation and mucociliary clearance. Ann Am Thorac Soc. (2018) 15(Suppl. 3):S143–8. doi: 10.1513/AnnalsATS.201802-128AW
36. Kuek LE, Lee RJ. First contact: the role of respiratory cilia in host-pathogen interactions in the airways. Am J Physiol Lung Cell Mol Physiol. (2020) 319:L603–19. doi: 10.1152/ajplung.00283.2020
37. Inui TA, Murakami K, Yasuda M, Hirano S, Ikeuchi Y, Kogiso H, et al. Ciliary beating amplitude controlled by intracellular Cl(-) and a high rate of CO(2) production in ciliated human nasal epithelial cells. Pflugers Arch Eur J Physiol. (2019) 471:1127–42. doi: 10.1007/s00424-019-02280-5
38. Inui TA, Yasuda M, Hirano S, Ikeuchi Y, Kogiso H, Inui T, et al. Daidzein-stimulated increase in the ciliary beating amplitude via an [Cl(-)](i) decrease in ciliated human nasal epithelial cells. Int J Mol Sci. (2018) 19:3754. doi: 10.3390/ijms19123754
39. McCormick J, Hoffman K, Thompson H, Skinner D, Zhang S, Grayson J, et al. Differential chloride secretory capacity in transepithelial ion transport properties in chronic rhinosinusitis. Am J Rhinol Allergy. (2020) 34:830–7. doi: 10.1177/1945892420930975
40. Kao SS, Bassiouni A, Ramezanpour M, Finnie J, Chegeni N, Colella AD, et al. Proteomic analysis of nasal mucus samples of healthy patients and patients with chronic rhinosinusitis. J Allergy Clin Immunol. (2021) 147:168–78. doi: 10.1016/j.jaci.2020.06.037
41. Jiao J, Zhang T, Zhang Y, Li JY, Wang M, Wang M, et al. Epidermal growth factor upregulates expression of MUC5AC via TMEM16A, in chronic rhinosinusitis with nasal polyps. Allergy Asthma Clin Immul. (2020) 16:40. doi: 10.1186/s13223-020-00440-2
42. Hou J, Zhou B, Zhang L, Li Y, Liu M, Wei Y, et al. Expression and significance of eosinophil basic proteins in nasal mucus of chronic sinusitis. J Clin Otorhinolaryngol Head Neck Surg. (2008) 10:454–455.
43. Chen Z, Liu JY, Chen J, Ma YY, Liao M, Li S, et al. The role of epithelial cells in the formation and development of nasal polyps. J Clin Otorhinolaryngol Head Neck Surg. (2020) 34:1053–6. doi: 10.13201/j.issn.2096-7993.2020.11.024
44. Cao PP, Li HB, Wang BF, Wang SB, You XJ, Cui YH, et al. Distinct immunopathologic characteristics of various types of chronic rhinosinusitis in adult Chinese. J Allergy Clin Immunol. (2009) 124:478–84. doi: 10.1016/j.jaci.2009.05.017
45. Xie Y, Li M, Chen K, Zhu H, Tang M, Zhou C, et al. Necroptosis underlies neutrophilic inflammation associated with the chronic rhinosinusitis with nasal polyps (CRSwNP). J Inflamm Res. (2021) 14:3969–83. doi: 10.2147/JIR.S322875
46. Ryu G, Kim DW. Th2 inflammatory responses in the development of nasal polyps and chronic rhinosinusitis. Curr Opin Allergy Clin Immunol. (2020) 20:1–8. doi: 10.1097/ACI.0000000000000588
47. Zheng R, Wang D, Wang K, Gao WX, Yang QT, Jiang LJ, et al. Elevated expression of IL-17RB and ST2 on myeloid dendritic cells is associated with a Th2-skewed eosinophilic inflammation in nasal polyps. Clin Transl Allergy. (2018) 8:50. doi: 10.1186/s13601-018-0237-4
48. Patel NN, Kohanski MA, Maina IW, Workman AD, Herbert DR, Cohen NA. Sentinels at the wall: epithelial-derived cytokines serve as triggers of upper airway type 2 inflammation. Int Forum Allergy Rhinol. (2019) 9:93–9. doi: 10.1002/alr.22206
49. Liao B, Cao PP, Zeng M, Zhen Z, Wang H, Zhang YN, et al. Interaction of thymic stromal lymphopoietin, IL-33, and their receptors in epithelial cells in eosinophilic chronic rhinosinusitis with nasal polyps. Allergy. (2015) 70:1169–80. doi: 10.1111/all.12667
50. Peters AT, Kato A, Zhang N, Conley DB, Suh L, Tancowny B, et al. Evidence for altered activity of the IL-6 pathway in chronic rhinosinusitis with nasal polyps. J Allergy Clin Immunol. (2010) 125:397–403. doi: 10.1016/j.jaci.2009.10.072
51. Kimura A, Kishimoto T. IL-6: regulator of Treg/Th17 balance. Eur J Immunol. (2010) 40:1830–5. doi: 10.1002/eji.201040391
52. Bequignon E, Mangin D, Bécaud J, Pasquier J, Angely C, Bottier M, et al. Pathogenesis of chronic rhinosinusitis with nasal polyps: role of IL-6 in airway epithelial cell dysfunction. J Transl Med. (2020) 18:136. doi: 10.1186/s12967-020-02309-9
53. Yang D, Zhang W, Zhang H, Zhang F, Chen L, Ma L, et al. Progress, opportunity, and perspective on exosome isolation - efforts for efficient exosome-based theranostics. Theranostics. (2020) 10:3684–707. doi: 10.7150/thno.41580
54. Mueller SK. The role of exosomes in the pathophysiology of chronic rhinosinusitis. Front Cell Infect Microbiol. (2021) 11:812920. doi: 10.3389/fcimb.2021.812920
55. Wang LF, Lee CH, Liang SS, Hung CC, Wu YR, Chien CY, et al. Mucin 5AC is significantly upregulated in exosomes from the nasal lavage fluid and may promote the expression of COX-2, VEGF and MMP-9: an implication in nasal polyp pathogenesis. Rhinology. (2021) 59:328–36. doi: 10.4193/Rhin20.564
56. Nocera AL, Mueller SK, Stephan JR, Hing L, Seifert P, Han X, et al. Exosome swarms eliminate airway pathogens and provide passive epithelial immunoprotection through nitric oxide. J Allergy Clin Immunol. (2019) 143:1525–35. doi: 10.1016/j.jaci.2018.08.046
57. Nocera AL, Miyake MM, Seifert P, Han X, Bleier BS. Exosomes mediate interepithelial transfer of functional P-glycoprotein in chronic rhinosinusitis with nasal polyps. Laryngoscope. (2017) 127:E295–300. doi: 10.1002/lary.26614
58. Lässer C, O'Neil SE, Shelke GV, Sihlbom C, Hansson SF, Gho YS, et al. Exosomes in the nose induce immune cell trafficking and harbour an altered protein cargo in chronic airway inflammation. J Transl Med. (2016) 14:181. doi: 10.1186/s12967-016-0927-4
59. Shenoy GN, Bhatta M, Loyall JL, Kelleher RJ Jr, Bernstein JM, Bankert RB. Exosomes represent an immune suppressive T cell checkpoint in human chronic inflammatory microenvironments. Immunol Investig. (2020) 49:726–43. doi: 10.1080/08820139.2020.1748047
60. Mueller SK, Nocera AL, Dillon ST, Gu X, Wendler O, Otu HH, et al. Noninvasive exosomal proteomic biosignatures, including cystatin SN, peroxiredoxin-5, and glycoprotein VI, accurately predict chronic rhinosinusitis with nasal polyps. Int Forum Allergy Rhinol. (2019) 9:177–86. doi: 10.1002/alr.22226
61. Miyake MM, Workman AD, Nocera AL, Wu D, Mueller SK, Finn K, et al. Discriminant analysis followed by unsupervised cluster analysis including exosomal cystatins predict presence of chronic rhinosinusitis, phenotype, and disease severity. Int Forum Allergy Rhinol. (2019) 9:1069–76. doi: 10.1002/alr.22380
62. Mueller SK, Wendler O, Nocera A, Grundtner P, Schlegel P, Agaimy A, et al. Escalation in mucus cystatin 2, pappalysin-A, and periostin levels over time predict need for recurrent surgery in chronic rhinosinusitis with nasal polyps. Int Forum Allergy Rhinol. (2019) 9:1212–9. doi: 10.1002/alr.22407
63. Mueller SK, Nocera AL, Dillon ST, Wu D, Libermann TA, Bleier BS. Highly multiplexed proteomic analysis reveals significant tissue and exosomal coagulation pathway derangement in chronic rhinosinusitis with nasal polyps. Int Forum Allergy Rhinol. (2018) 8:1438–44. doi: 10.1002/alr.22189
64. Holers VM. Complement and its receptors: new insights into human disease. Annu Rev Immunol. (2014) 32:433–59. doi: 10.1146/annurev-immunol-032713-120154
65. Gaunsbaek MQ, Lange B, Kjeldsen AD, Svane-Knudsen V, Skjoedt K, Henriksen ML, et al. Complement defects in patients with chronic rhinosinusitis. PLoS ONE. (2012) 7:e47383. doi: 10.1371/journal.pone.0047383
66. Wang F, Yang Y, You Q, Chen H. Prognostic significance of serum complement component 3 in chronic rhinosinusitis with nasal polyps. ORL. (2020) 82:67–73. doi: 10.1159/000504195
67. DiScipio RG, Schraufstatter IU. The role of the complement anaphylatoxins in the recruitment of eosinophils. Int Immunopharmacol. (2007) 7:1909–23. doi: 10.1016/j.intimp.2007.07.006
68. Carroll WW, Schlosser RJ, O'Connell BP, Soler ZM, Mulligan JK. Vitamin D deficiency is associated with increased human sinonasal fibroblast proliferation in chronic rhinosinusitis with nasal polyps. Int Forum Allergy Rhinol. (2016) 6:605–10. doi: 10.1002/alr.21704
69. Christensen JM, Cheng J, Earls P, Gunton J, Sewell W, Sacks R, et al. Vitamin D pathway regulatory genes encoding 1α-hydroxylase and 24-hydroxylase are dysregulated in sinonasal tissue during chronic rhinosinusitis. Int Forum Allergy Rhinol. (2017) 7:169–76. doi: 10.1002/alr.21852
70. Schlosser RJ, Carroll WW, Soler ZM, Pasquini WN, Mulligan JK. Reduced sinonasal levels of 1α-hydroxylase are associated with worse quality of life in chronic rhinosinusitis with nasal polyps. Int Forum Allergy Rhinol. (2016) 6:58–65. doi: 10.1002/alr.21576
71. Mulligan JK, Pasquini WN, Carroll WW, Williamson T, Reaves N, Patel KJ, et al. Dietary vitamin D3 deficiency exacerbates sinonasal inflammation and alters local 25(OH)D3 metabolism. PLoS ONE. (2017) 12:e0186374. doi: 10.1371/journal.pone.0186374
72. Jiao J, King TS, McKenzie M, Bacharier LB, Dixon AE, Codispoti CD, et al. Vitamin D3 therapy in patients with asthma complicated by sinonasal disease: Secondary analysis of the Vitamin D Add-on Therapy Enhances Corticosteroid Responsiveness in Asthma trial. J Allergy Clin Immunol. (2016) 138:589–92. doi: 10.1016/j.jaci.2015.12.1329
73. Mulligan JK, Nord D, Villanueva MV, Justice J, Lobo B, Schlosser RJ, et al. Role of C3a as a novel regulator of 25(OH)D(3) to 1α,25-dihydroxyvitamin D(3) metabolism in upper airway epithelial cells. J Immunol. (2022) 209:262–9. doi: 10.4049/jimmunol.2000726
74. Jayachandran J, Srinivasan H, Mani KP. Molecular mechanism involved in epithelial to mesenchymal transition. Arch Biochem Biophys. (2021) 710:108984. doi: 10.1016/j.abb.2021.108984
75. Acloque H, Adams MS, Fishwick K, Bronner-Fraser M, Nieto MA. Epithelial-mesenchymal transitions: the importance of changing cell state in development and disease. J Clin Invest. (2009) 119:1438–49. doi: 10.1172/JCI38019
76. Qin D, Liu P, Zhou H, Jin J, Gong W, Liu K, et al. TIM-4 in macrophages contributes to nasal polyp formation through the TGF-β1-mediated epithelial to mesenchymal transition in nasal epithelial cells. Front Immunol. (2022) 13:941608. doi: 10.3389/fimmu.2022.941608
77. Jiang W, Zhou C, Ma C, Cao Y, Hu G, Li H. TGF-β1 induces epithelial-to-mesenchymal transition in chronic rhinosinusitis with nasal polyps through microRNA-182. Asian Pac J Allergy Immunol. (2021). doi: 10.12932/ap-040921-1224
78. Li X, Li C, Zhu G, Yuan W, Xiao ZA. TGF-β1 induces epithelial-mesenchymal transition of chronic sinusitis with nasal polyps through microRNA-21. Int Arch Allergy Immunol. (2019) 179:304–19. doi: 10.1159/000497829
79. Böscke R, Vladar EK, Könnecke M, Hüsing B, Linke R, Pries R, et al. Wnt signaling in chronic rhinosinusitis with nasal polyps. Am J Respir Cell Mol Biol. (2017) 56:575–84. doi: 10.1165/rcmb.2016-0024OC
80. Bae JS, Ryu G, Kim JH, Kim EH, Rhee YH, Chung YJ, et al. Effects of Wnt signaling on epithelial to mesenchymal transition in chronic rhinosinusitis with nasal polyp. Thorax. (2020) 75:982–93. doi: 10.1136/thoraxjnl-2019-213916
81. Bassiouni A, Chen PG, Wormald PJ. Mucosal remodeling and reversibility in chronic rhinosinusitis. Curr Opin Allergy Clin Immunol. (2013) 13:4–12. doi: 10.1097/ACI.0b013e32835ad09e
82. Lee K, Tai J, Lee SH, Kim TH. Advances in the knowledge of the underlying airway remodeling mechanisms in chronic rhinosinusitis based on the endotypes: a review. Int J Mol Sci. (2021) 22:910. doi: 10.3390/ijms22020910
83. Do TQ, Barham HP, Earls P, Sacks R, Christensen JM, Rimmer J, et al. Clinical implications of mucosal remodeling from chronic rhinosinusitis. Int Forum Allergy Rhinol. (2016) 6:835–40. doi: 10.1002/alr.21754
84. Barham HP, Osborn JL, Snidvongs K, Mrad N, Sacks R, Harvey RJ. Remodeling changes of the upper airway with chronic rhinosinusitis. Int Forum Allergy Rhinol. (2015) 5:565–72. doi: 10.1002/alr.21546
85. Tsuda T, Maeda Y, Nishide M, Koyama S, Hayama Y, Nojima S, et al. Eosinophil-derived neurotoxin enhances airway remodeling in eosinophilic chronic rhinosinusitis and correlates with disease severity. Int Immunol. (2019) 31:33–40. doi: 10.1093/intimm/dxy061
86. Frantz C, Stewart KM, Weaver VM. The extracellular matrix at a glance. J Cell Sci. (2010) 123(Pt 24):4195–200. doi: 10.1242/jcs.023820
87. Takayama G, Arima K, Kanaji T, Toda S, Tanaka H, Shoji S, et al. Periostin: a novel component of subepithelial fibrosis of bronchial asthma downstream of IL-4 and IL-13 signals. J Allergy Clin Immunol. (2006) 118:98–104. doi: 10.1016/j.jaci.2006.02.046
88. Ebenezer JA, Christensen JM, Oliver BG, Oliver RA, Tjin G, Ho J, et al. Periostin as a marker of mucosal remodelling in chronic rhinosinusitis. Rhinology. (2017) 55:234–41. doi: 10.4193/Rhino16.215
89. Watelet JB, Bachert C, Claeys C, Van Cauwenberge P. Matrix metalloproteinases MMP-7, MMP-9 and their tissue inhibitor TIMP-1: expression in chronic sinusitis vs nasal polyposis. Allergy. (2004) 59:54–60. doi: 10.1046/j.1398-9995.2003.00364.x
90. Van Bruaene N, Derycke L, Perez-Novo CA, Gevaert P, Holtappels G, De Ruyck N, et al. TGF-beta signaling and collagen deposition in chronic rhinosinusitis. J Allergy Clin Immunol. (2009) 124:253–9. doi: 10.1016/j.jaci.2009.04.013
91. Painter JD, Galle-Treger L, Akbari O. Role of autophagy in lung inflammation. Front Immunol. (2020) 11:1337. doi: 10.3389/fimmu.2020.01337
92. Levine B, Kroemer G. Autophagy in the pathogenesis of disease. Cell. (2008) 132:27–42. doi: 10.1016/j.cell.2007.12.018
93. Li K, Li M, Li W, Yu H, Sun X, Zhang Q, et al. Airway epithelial regeneration requires autophagy and glucose metabolism. Cell Death Dis. (2019) 10:875. doi: 10.1038/s41419-019-2111-2
94. Chen ZH, Wu YF, Wang PL, Wu YP, Li ZY, Zhao Y, et al. Autophagy is essential for ultrafine particle-induced inflammation and mucus hyperproduction in airway epithelium. Autophagy. (2016) 12:297–311. doi: 10.1080/15548627.2015.1124224
95. Zhang Y, Qi J, Yan D, Deng Y, Zhang J, Luo Q. HNE induces the hyperexpression of MUC5AC in chronic rhinosinusitis with nasal polyps by activating the TRAF6/autophagy regulatory axis. Am J Rhinol Allergy. (2022) 36:816–26. doi: 10.1177/19458924221116939
96. Ye Y, Zhao J, Ye J, Jiang X, Liu H, Xie Y, et al. The role of autophagy in the overexpression of MUC5AC in patients with chronic rhinosinusitis. Int Immunopharmacol. (2019) 71:169–80. doi: 10.1016/j.intimp.2019.03.028
97. Wang C, Zhou ML, Liu YC, Cheng KJ. The roles of autophagy, mitophagy, and the Akt/mTOR pathway in the pathogenesis of chronic rhinosinusitis with nasal polyps. J Immunol Res. (2022) 2022:2273121. doi: 10.1155/2022/2273121
98. Choi GE, Yoon SY, Kim JY, Kang DY, Jang YJ, Kim HS. Autophagy deficiency in myeloid cells exacerbates eosinophilic inflammation in chronic rhinosinusitis. J Allergy Clin Immunol. (2018) 141:938–50. doi: 10.1016/j.jaci.2017.10.038
99. Regan P, Elkhalifa S, Barratt P. The systemic immunosuppressive effects of peripheral corticosteroid injections: a narrative review of the evidence in the context of COVID-19. Musculoskeletal Care. (2022) 20:431–41. doi: 10.1002/msc.1603
100. Mullol J, Obando A, Pujols L, Alobid I. Corticosteroid treatment in chronic rhinosinusitis: the possibilities and the limits. Immunol Allergy Clinics North Am. (2009) 29:657–68. doi: 10.1016/j.iac.2009.07.001
101. Harvey RJ, Snidvongs K, Kalish LH, Oakley GM, Sacks R. Corticosteroid nasal irrigations are more effective than simple sprays in a randomized double-blinded placebo-controlled trial for chronic rhinosinusitis after sinus surgery. Int Forum Allergy Rhinol. (2018) 8:461–70. doi: 10.1002/alr.22093
102. Guan YC, Jiang L, Ma LL, Sun XN, Yu DD, Liu J, et al. Expression of glucocorticoid receptor isoforms and associations with serine/arginine-rich protein 30c and 40 in patients with systemic lupus erythematosus. Clin Exp Rheumatol. (2015) 33:225–33.
103. de Borja Callejas F, Martínez-Antón A, Picado C, Alobid I, Pujols L, Valero A, et al. Corticosteroid treatment regulates mucosal remodeling in chronic rhinosinusitis with nasal polyps. Laryngoscope. (2015) 125:E158–67. doi: 10.1002/lary.25147
104. Radajewski K, Kalińczak-Górna P, Zdrenka M, Antosik P, Wierzchowska M, Grzanka D, et al. Short term pre-operative oral corticosteroids-tissue remodeling in chronic rhinosinusitis with nasal polyps. J Clin Med. (2021) 10:3346. doi: 10.3390/jcm10153346
105. Ghogomu N, Kern R. Chronic rhinosinusitis: the rationale for current treatments. Expert Rev Clin Immunol. (2017) 13:259–70. doi: 10.1080/1744666X.2016.1220833
106. Grayson JW, Harvey RJ. Topical corticosteroid irrigations in chronic rhinosinusitis. Int Forum Allergy Rhinol. (2019) 9:S9–15. doi: 10.1002/alr.22331
107. Head K, Chong LY, Hopkins C, Philpott C, Schilder AG, Burton MJ. Short-course oral steroids as an adjunct therapy for chronic rhinosinusitis. Cochrane Database Syst Rev. (2016) 4:Cd011992. doi: 10.1002/14651858.CD011992.pub2
108. Ow RA, McGinnis JP II, Sacks HJ, Mehle ME. The effect of EDS-FLU on objective and patient-reported subjective outcomes for patients with chronic rhinosinusitis with nasal polyps. Ear Nose Throat J. (2022) 1455613221088698. doi: 10.1177/01455613221088698
109. Sindwani R, Han JK, Soteres DF, Messina JC, Carothers JL, Mahmoud RA, et al. NAVIGATE I: randomized, placebo-controlled, double-blind trial of the exhalation delivery system with fluticasone for chronic rhinosinusitis with nasal polyps. Am J Rhinol Allergy. (2019) 33:69–82. doi: 10.1177/1945892418810281
110. Succar EF, Turner JH, Chandra RK. Nasal saline irrigation: a clinical update. Int Forum Allergy Rhinol. (2019) 9:S4–8. doi: 10.1002/alr.22330
111. Lee VS. Topical irrigations for chronic rhinosinusitis. Immunol Allergy Clin North Am. (2020) 40:317–28. doi: 10.1016/j.iac.2019.12.014
112. Yang Y, Sun Y, Xiang F, Zhang M, Fu L, Zhang Q. Efficacy and safety of traditional Chinese medicine nasal irrigation on chronic rhinosinusitis recovery after endoscopic sinus surgery: a protocol for a systematic review and meta-analysis. PLoS ONE. (2022) 17:e0272371. doi: 10.1371/journal.pone.0272371
113. Woods CM, Tan S, Ullah S, Frauenfelder C, Ooi EH, Carney AS. The effect of nasal irrigation formulation on the antimicrobial activity of nasal secretions. Int Forum Allergy Rhinol. (2015) 5:1104–10. doi: 10.1002/alr.21604
114. Jiao J, Yang J, Li J, Li Y, Zhang L. Hypertonic saline and seawater solutions damage sinonasal epithelial cell air-liquid interface cultures. Int Forum Allergy Rhinol. (2020) 10:59–68. doi: 10.1002/alr.22459
115. Hashemian F, Baghbanian N, Majd Z, Rouini MR, Jahanshahi J, Hashemian F. The effect of thyme honey nasal spray on chronic rhinosinusitis: a double-blind randomized controlled clinical trial. Eur Arch Oto-Rhino-Laryngol. (2015) 272:1429–35. doi: 10.1007/s00405-014-3233-x
116. Saydy N, Moubayed SP, Desrosiers M. Patient perspectives on endoscopic sinus surgery for chronic rhinosinusitis. J Otolaryngol Head Neck Surg. (2021) 50:34. doi: 10.1186/s40463-021-00515-z
117. Sukato DC, Abramowitz JM, Boruk M, Goldstein NA, Rosenfeld RM. Endoscopic sinus surgery improves sleep quality in chronic rhinosinusitis: a systematic review and meta-analysis. Otolaryngol Head Neck Surg. (2018) 158:249–56. doi: 10.1177/0194599817737977
118. Little RE, Alt JA, Ramakrishnan VR, Platt MP, Schlosser RJ, Storck KA, et al. Objective sleep measures after endoscopic sinus surgery in patients with chronic rhinosinusitis. Int Forum Allergy Rhinol. (2021) 11:1056–63. doi: 10.1002/alr.22738
119. Naraghi M, Baghbanian N, Moharari M, Saghazadeh A. Improvement of sinonasal mucociliary function by endoscopic sinus surgery in patients with chronic rhinosinusitis. Am J Otolaryngol. (2018) 39:707–10. doi: 10.1016/j.amjoto.2018.07.019
120. Alanin MC, Hopkins C. Effect of functional endoscopic sinus surgery on outcomes in chronic rhinosinusitis. Curr Allergy Asthma Rep. (2020) 20:27. doi: 10.1007/s11882-020-00932-6
121. Smith KA, Orlandi RR, Oakley G, Meeks H, Curtin K, Alt JA. Long-term revision rates for endoscopic sinus surgery. Int Forum Allergy Rhinol. (2019) 9:402–8. doi: 10.1002/alr.22264
122. DeConde AS, Mace JC, Levy JM, Rudmik L, Alt JA, Smith TL. Prevalence of polyp recurrence after endoscopic sinus surgery for chronic rhinosinusitis with nasal polyposis. Laryngoscope. (2017) 127:550–5. doi: 10.1002/lary.26391
123. Miglani A, Divekar RD, Azar A, Rank MA, Lal D. Revision endoscopic sinus surgery rates by chronic rhinosinusitis subtype. Int Forum Allergy Rhinol. (2018) 8:1047–51. doi: 10.1002/alr.22146
124. Ryu G, Kim DK, Dhong HJ, Eun KM, Lee KE, Kong IG, et al. Immunological characteristics in refractory chronic rhinosinusitis with nasal polyps undergoing revision surgeries. Allergy Asthma Immunol Res. (2019) 11:664–76. doi: 10.4168/aair.2019.11.5.664
125. Loftus CA, Soler ZM, Koochakzadeh S, Desiato VM, Yoo F, Nguyen SA, et al. Revision surgery rates in chronic rhinosinusitis with nasal polyps: meta-analysis of risk factors. Int Forum Allergy Rhinol. (2020) 10:199–207. doi: 10.1002/alr.22487
126. Gill AS, Smith KA, Meeks H, Oakley GM, Curtin K, LeClair L, et al. Asthma increases long-term revision rates of endoscopic sinus surgery in chronic rhinosinusitis with and without nasal polyposis. Int Forum Allergy Rhinol. (2021) 11:1197–206. doi: 10.1002/alr.22779
127. Bartier S, Coste A, Béquignon E. Biotherapy and treatment of adult primary chronic rhinosinusitis with nasal polyps: cellular and molecular bases. Eur Ann Otorhinolaryngol Head Neck Dis. (2021) 138:355–62. doi: 10.1016/j.anorl.2020.12.002
128. Han JK, Bachert C, Fokkens W, Desrosiers M, Wagenmann M, Lee SE, et al. Mepolizumab for chronic rhinosinusitis with nasal polyps (SYNAPSE): a randomised, double-blind, placebo-controlled, phase 3 trial. Lancet Respir Med. (2021) 9:1141–53. doi: 10.1016/s2213-2600(21)00097-7
129. Scadding G. Cytokine profiles in allergic rhinitis. Curr Allergy Asthma Rep. (2014) 14:435. doi: 10.1007/s11882-014-0435-7
131. Diver S, Khalfaoui L, Emson C, Wenzel SE, Menzies-Gow A, Wechsler ME, et al. Effect of tezepelumab on airway inflammatory cells, remodelling, and hyperresponsiveness in patients with moderate-to-severe uncontrolled asthma (CASCADE): a double-blind, randomised, placebo-controlled, phase 2 trial. Lancet Respir Med. (2021) 9:1299–312. doi: 10.1016/S2213-2600(21)00226-5
132. Mustafa SS, Vadamalai K, Scott B, Ramsey A. Dupilumab as add-on therapy for chronic rhinosinusitis with nasal polyposis in aspirin exacerbated respiratory disease. Am J Rhinol Allergy. (2021) 35:399–407. doi: 10.1177/1945892420961969
133. Bachert C, Mannent L, Naclerio RM, Mullol J, Ferguson BJ, Gevaert P, et al. Effect of subcutaneous dupilumab on nasal polyp burden in patients with chronic sinusitis and nasal polyposis: a randomized clinical trial. JAMA. (2016) 315:469–79. doi: 10.1001/jama.2015.19330
134. Wheatley LM, Togias A. Clinical practice. Allergic rhinitis. N Engl J Med. (2015) 372:456–63. doi: 10.1056/NEJMcp1412282
135. Damask C, Chen M, Holweg CTJ, Yoo B, Millette LA, Franzese C. Defining the efficacy of omalizumab in nasal polyposis: a POLYP 1 and POLYP 2 subgroup analysis. Am J Rhinol Allergy. (2022) 36:135–41. doi: 10.1177/19458924211030486
136. Gevaert P, Omachi TA, Corren J, Mullol J, Han J, Lee SE, et al. Efficacy and safety of omalizumab in nasal polyposis: 2 randomized phase 3 trials. J Allergy Clin Immunol. (2020) 146:595–605. doi: 10.1016/j.jaci.2020.05.032
137. Gevaert P, Saenz R, Corren J, Han JK, Mullol J, Lee SE, et al. Long-term efficacy and safety of omalizumab for nasal polyposis in an open-label extension study. J Allergy Clin Immuno. (2022) 149:957–65. doi: 10.1016/j.jaci.2021.07.045
138. Kim YH, Yang TY, Park CS, Ahn SH, Son BK, Kim JH, et al. Anti-IL-33 antibody has a therapeutic effect in a murine model of allergic rhinitis. Allergy. (2012) 67:183–90. doi: 10.1111/j.1398-9995.2011.02735.x
Keywords: epithelium, dysfunction, pathogenesis, treatment, chronic rhinosinusitis
Citation: He Y, Fu Y, Wu Y, Zhu T and Li H (2023) Pathogenesis and treatment of chronic rhinosinusitis from the perspective of sinonasal epithelial dysfunction. Front. Med. 10:1139240. doi: 10.3389/fmed.2023.1139240
Received: 06 January 2023; Accepted: 13 March 2023;
Published: 17 April 2023.
Edited by:
Ismail Ogulur, University of Zurich, SwitzerlandReviewed by:
Luca Malvezzi, Humanitas San Pio X Hospital, ItalyAyca Kiykim, Istanbul University-Cerrahpasa, Türkiye
Copyright © 2023 He, Fu, Wu, Zhu and Li. This is an open-access article distributed under the terms of the Creative Commons Attribution License (CC BY). The use, distribution or reproduction in other forums is permitted, provided the original author(s) and the copyright owner(s) are credited and that the original publication in this journal is cited, in accordance with accepted academic practice. No use, distribution or reproduction is permitted which does not comply with these terms.
*Correspondence: Hui Li, dHRsaWh1aSYjeDAwMDQwOzE2My5jb20=
†These authors have contributed equally to this work