- 1Department of Ophthalmology, Hopitaux Universitaires de Genève (HUG), Genève, Switzerland
- 2Department of Ophthalmology, CHU Bordeaux, Bordeaux, France
- 3INSERM, Bordeaux Population Health Research Center, Team LEHA, UMR 1219, Univ. Bordeaux, Bordeaux, France
Cataract surgery is among the most frequently performed surgical procedures worldwide and has a tremendous impact on patients' quality of life. Phacoemulsification (PCS) is accepted as a standard of care; its technique has continuously evolved and already achieved good anatomical, visual, and refractive outcomes. Lasers in ophthalmology are widely used in clinical practice, femtosecond lasers (FSLs) for corneal surgery in particular. It was natural to assess the usefulness of FSL in cataract surgery as this technology was within reach. Indeed, precise and reproducible cuttings provided by FSL platforms could improve standardization of care and limit the risk associated with the human element in surgery and provide a step toward robot-assisted surgery. After docking and planning the procedure, femtosecond lasers are used to perform corneal incisions, capsulorhexis, lens fragmentation, and arcuate incisions in an automated manner. A well-constructed corneal incision is primordial as it offers safety during the procedure, self-seals afterward, and influences the refractive outcome. Capsulorhexis size, centration, and resistance to shearing influence the surgery, intraocular lens (IOL) centration and stability, and posterior capsular opacification formation. Lens fragmentation is where most of the energy is delivered into the eye, and its amount influences endothelial cell damage and potential damage to other ocular structures. The arcuate incisions offer an additional opportunity to influence postoperative astigmatism. Femtosecond laser-assisted cataract surgery (FLACS) has been a topic of research in many studies and clinical trials that attempted to assess its potential benefits and cost-effectiveness over PCS and is the subject of this mini-review.
Introduction
Cataract remains the leading cause of blindness and the second cause of moderate and severe visual impairment in 2020, affecting 15.2 and 78.8 million people, respectively, worldwide. Cataract surgery is the most routinely performed surgical procedure, with 7 million surgeries performed per year in Europe, 3.7 million in the United States, and 20 million worldwide (1). Since its first introduction in 1967 by Kelman and continuous development of machines and intraocular lenses, phacoemulsification cataract surgery (PCS) has become the standard of care (2–6).
The recent development of near-infrared lasers with ultrashort pulse duration of the femtosecond domain (10−15 s) has opened new insights in ophthalmic surgery. Femtosecond lasers (FSLs) had their first clinical application in ophthalmology in corneal treatment in the late 1990s; they were then evaluated for their role in cataract surgery (7), with first treated patients in 2009 (8) and received Food and Drug Administration approval in 2010. During FLACS, after the docking procedure and owing to non-invasive and precise tissue treatment (9), FSL can be used to perform corneal incisions, capsulotomy, lens fragmentation, and arcuate incisions. Eliminating the human element from the first steps of the surgery has been suggested to improve the safety and reproducibility of surgical outcomes (10, 11). However, well-conducted and sufficiently powered randomized clinical trials comparing both techniques were needed to provide robust evidence and recommendations (12).
In this article, we report on the principles of FLACS and review current scientific evidence on PCS and its potential perspectives.
Technical performances and principles of FLACS
Femtosecond laser is a near-infrared (1,053 nm) solid-state laser achieving photodisruption in ocular tissues; it is capable of delivering ultrashort femtosecond pulses 200–800 fs (1 fs = 10−15 s) of low overall energy and high peak power. It leads to more precise impact, less energy per pulse, and, in turn, less damage in collateral tissue than longer pulse lasers, e.g., nanosecond lasers (13). To achieve photodisruption, a certain degree of energy threshold must be surpassed, depending on the nature of treated tissue and different laser features: numerical aperture (NA) and wavelength. A larger NA decreases the volume of the focal spot and, together with a shorter wavelength (e.g., in the UV range for new prototype femtosecond lasers), lowers the energy threshold. A shorter pulse laser enables a decrease in the total energy needed to achieve this threshold. Photodisruption consists of three consecutive events: plasma formation, shock wave generation, and cavitation. Rapidly expanding plasma leads to the creation of a focal shock wave, which is atraumatic for the surrounding tissue and leaves behind only a small residual gas bubble (14). The mechanism of tissue separation achieved by FSL is twofold: ablation and cleaving. Gas bubbles represent directly ablated tissue by plasma formation within the laser focus, while rapid expansion and cavitation achieve further tissue separation by cleaving. Laser parameters determine which of those two mechanisms plays a chief role in tissue separation. At higher pulse energies, the radius of disrupted tissue is greater than the spot size, and tissue is separated by mechanical forces of expanding gas bubbles. Lower energies generate less cavitation and ablate a smaller volume of tissue; they allow for less traumatic tissue separation but require much tighter spot placement and call for higher pulse frequencies.
The cataract laser faces challenges different from the corneal platforms. Treatment depths in the former are usually between 100 and 130 μm, as with LASIK, while cataract surgery demands flexibility up to 7,500 μm. Such a difference generates the need for increased spot size and higher pulse energy with the cataract lasers. In addition, 5–10 times greater total energy and spot placements are required for lens surgery, in comparison to corneal surgery (8).
In FLACS, complicated and flexible laser patterns are needed, as each step of cataract surgery requires its proprietary treatment and energy profile. Laser capsulotomy requires an adjustable circular laser incision (achieved with postage stamp perforations) at a considerable depth with good accuracy. Lens fragmentation enables the surgeon to choose between different patterns of lens softening: cubes, spheres, pies, etc. So far, no ideal pattern enabling a low cumulative dissipated energy (CDE) has been described, but some lens softening patterns may be better than others in different grades or types of cataract (15).
The patient interface (PI) is essential to connect the laser to the eye and therefore must provide adequate stability without distortion of eye structures, be well tolerated by the patient, and not interfere with laser treatment. In FLACS, either a curved lens applanation or a fluid interface is used. Studies comparing docking with a curved vs. fluid PI showed no significant differences in capsulotomy regularity (16), but corneal folds, sometimes occurring with applanating PI, may induce incomplete capsulotomies with subsequent anterior capsular tears (17). The non-applanating designs, using a suction ring attached to the sclera and a fluid immersion chamber, do require more corneal exposure while docking but offer a larger treatment area, better compensation for lens tilt, and a much lower induced IOP rise during the treatment (17). Speculation exists whether the anterior movement of the lens, anterior hyaloid, and vitreous base during suction can predispose patients to develop vitreoretinal pathology, but no evidence from corneal-only FSL exists (18). The docking procedure is paramount in achieving good laser treatment but is an extra step compared to PCS and can be time-consuming (19, 20). A potential complication is the loss of suction leading to incomplete laser treatment. In an early study by Bali et al. (20), the authors experienced suction loss requiring abandoning the procedure in 2.5% of cases, but the study demonstrated a learning curve for the first 100 cases. Manning et al. (21) indicated that the laser procedure had to be abandoned in 0.1% (3 eyes). Zhang et al. (22) found the completion of capsulotomy, lens fragmentation, and corneal incisions to be at 98.6, 98.5, and 97.6%, respectively. In the first studies, the most frequently reported complication related to docking was transient conjunctival redness or hemorrhage in up to 34% of cases (23).
Finally, unlike FSL corneal procedures, precise and reliable imaging is needed for FLACS procedures. OCT is the most frequent imaging technology, but three-dimensional confocal structured illumination imaging is also used by one platform. Indeed, by enabling a three-dimensional reconstruction of anterior eye structures, it helps define capsulotomy margins, corneal incision placement, and safety zones within the lens itself.
Clinical results
Corneal incisions
Incisions are a crucial step in completing cataract surgery with clear corneal incisions being the standard for PCS and FLACS. Laser-created incisions were found to be reproducible and stable and did not significantly change the high-order aberrations over time (24–27). Users can also skip this step and elect to perform them with the microkeratome; this seemed to be the common procedure among surgeons as indicated in the 2016 study from the European Registry of Quality Outcomes for Cataract and Refractive Surgery, since in only 34.7% of FLACS cases, the incisions were performed by laser (21). The reason might be due to the fact that laser incisions take significantly longer to perform. The laser-created incisions show a saw-tooth pattern, increased cell apoptosis, and are less smooth than manual incisions (28). They may be less stable in the early postoperative period as well (29).
Diakonis et al. (30) did not show any significant difference in surgically induced astigmatism between FLACS and PCS using a multivariate vectorial analysis.
Capsulotomy
The main advantage of FLACS is the ability to create a perfectly circular, centered capsulotomy with an accurate and reproducible diameter (31–33). Anterior capsulotomy conditions both the remainder of cataract surgery and the refractive outcome achieved by patients. Inaccurate prediction of effective lens position (ELP), which is, in turn, affected by capsulotomy size and morphology, is one of the biggest factors leading to residual refractive error contributing to 35% of total residual error (34). Studies have confirmed femtosecond laser capsulotomies to be rounder, more precise, better centered, and more stable over time as compared to manual capsulorhexis. However, this advantage has not been translated into better visual acuity or effective lens position (35).
There are, however, disadvantages to a laser capsulotomy, mainly its strength. Electron microscopy images demonstrate that the laser impacts do not produce a smooth capsulotomy rim as in a manual rhexis but instead produces one with numerous notches that could be more prone to tearing (36), resulting in an overall lower tensile strength (37) and, in turn, a higher frequency of anterior capsular tears (up to 4% in early published studies) (23, 38). Completeness of anterior capsulotomy can also be impeded by eye tremor during laser delivery, laser misfires, or corneal opacities such as scars or radial keratotomies (38, 39), but the latter might be remedied by adjusting laser settings (39, 40). Laser settings seem to be key even in routine FLACS, as different lasers produce microscopically different capsulotomy edges which might explain the variability of capsulotomy completeness and anterior radial tears in literature (0.1–4%) (23, 31, 41).
Intraoperative miosis following docking and laser procedure has also been reported in one-third of cases (23). This is mainly related to prostaglandin release during laser treatment of the anterior capsule (42, 43). Factors associated with intraoperative miosis are older patient age, longer duration of laser treatment, smaller laser capsulotomy–pupil margin distance, shallower anterior chamber, and smaller preoperative pupil size (44, 45). Some preoperative treatment regimens have been proposed to reduce intraoperative miosis onset or severity: mainly topical non-steroidal anti-inflammatory drugs 1–4 days before surgery and the day of the surgery (46–48).
Femtosecond laser-assisted arcuate keratotomy (FSAK)
Femtosecond laser-assisted cataract surgery has an advantage of an optional step of performing FSAK to correct low-to-moderate astigmatism during cataract surgery. FSAK has been found to be effective, safe, and stable over time (49–51). They also offer some flexibility as the surgeon can elect to open them at the slit-lamp during the postoperative period for a greater corrective effect.
Clinical studies have found FSAK to be comparable to toric IOLs in mild-to-moderate, central, and with-the-rule astigmatism correction but less predictable in moderate-to-high, against-the-rule, or limbus-to-limbus astigmatism (52, 53). A recent study by Hernandez et al. (54) found the toric IOL to be superior over FSAK in the correction of moderate (1.25–3.0 diopter) astigmatism. Nevertheless, FSAK is more predictable in comparison to manual limbal relaxing incisions, and the development of new nomograms in the future may improve their efficacy (49).
Endothelial cell loss, ultrasound energy delivered in the eye, and difficult cases
A potential advantage of FLACS over PCS could be that the lens softening allows for the reduction in effective phacoemulsification time (EPT) and cumulative dissipated energy (CDE) and thus may result in less damage to ocular tissues, particularly the corneal endothelium. An early study by Abell et al. (55) demonstrated a 96.2% reduction in EPT in the optimized FLACS group associated with a 36.1% reduction in endothelial cell loss; the laser pretreatment enabled an ultrasound-free lens aspiration in 30% of patients.
On the contrary, the FEMCAT trial did not observe any difference in central corneal thickness or corneal endothelial cell count between FLACS and PCS (56), and the FACT trial even showed more cell loss in the FLACS group (57). Other clinical trials seem to agree with this observation (58, 59), and the 2022 report by the American Academy of Ophthalmology concluded that the adequately powered RTCs did not demonstrate a significant difference in endothelial cell loss between FLACS and PCS (60).
There might, however, exist groups of patients that could benefit from the decreased EPT more than those with a routine cataract. Patients with hard nuclear cataracts or with shallow anterior chambers were shown to achieve a faster visual recovery after FLACS (61, 62). Yong et al. (63) found a significant difference in endothelial cell loss among Fuchs' patients (mean of 15.3 ± 17.5% for PCS and 4.4 ± 25.0% for FLACS) that was even more significant in the mild cataract subgroup.
Pediatric cataracts
Cataract surgery in infants differs from age-related phacosclerosis mainly because of the elasticity of the anterior capsule, the softness of the lens nucleus, and the necessity to perform posterior capsulotomy (64). FLACS has already been used in congenital cataract surgery with success (65–67), including challenging cases like Peters anomaly type 2 and persistent hyperplastic primary vitreous (68, 69). Femtosecond laser use is off-label in children and requires some adaptations in the surgical procedure. It creates capsulotomies that are larger than expected because of the tissue elasticity, and corrective factors must be used in treatment planning (66, 67). A laser-assisted posterior capsulotomy is feasible but requires redocking after lens aspiration (65).
Cystoid macular edema and mediators of inflammation release
Cystoid macular edema (CME) is a relatively infrequent complication of any intraocular surgery with an incidence of 0.95% after cataract surgery (70). FLACS was seen as a potential way of limiting this complication by decreasing EPT. However, a release of prostaglandins into the aqueous humor has been demonstrated during FLACS, especially after capsulotomy (42, 43). This can result in intraoperative miosis increasing risks and surgical time during cataract removal and impacting CME formation through inflammation cascade and disruption of the blood–retina barrier (71, 72). In clinical studies, this relationship was not as straightforward, as Koo et al. (73) found FLACS to be associated with a lesser likelihood of the usage of a pupil expansion device in comparison with PCS (7.0 vs. 24.2%) and a faster CME resolution in FLACS in cases of pseudoexfoliation.
Posterior capsule opacification (PCO) and phimosis
FLACS produces reliable capsulotomies contributing to bag stability and elimination of lens epithelial cells (LEC) and has been shown to induce LEC apoptosis in LEC close to the capsulotomy edge, which is linearly correlated with laser energy parameters and duration (74–76). This effect could theoretically decrease PCO rates in FLACS. Few results are reported, and some studies have even found higher PCO rates in FLACS compared to PCS and reported early optic axis opacities (21, 77), possibly in relation to the mesenchymal transformation of LEC when exposed to adjacent laser treatment.
Results of randomized controlled trials: FLACS vs. PCS
Several well-designed and rigorously conducted randomized controlled trials (RCTs) comparing FLACS to PCS have been published in the literature (56–59, 62, 78–84); their details are shown in Table 1. FEMCAT, FACT, and St. Thomas (56, 57, 82–84) trials evaluated corrected, uncorrected distance vision acuities, and refractive outcomes and found no statistically significant differences between both groups. Since complications in cataract surgery occur rarely, no RCT has been powered sufficiently for a meaningful analysis, and the published trials have not found differences in complications of FLACS compared to PCS, with the exception of St. Thomas trial, which found a statistically higher rate of posterior capsule rupture in PCS (3 vs. 0%) (83). The endothelial cell loss was similar between FLACS and PCS in published RCTs.
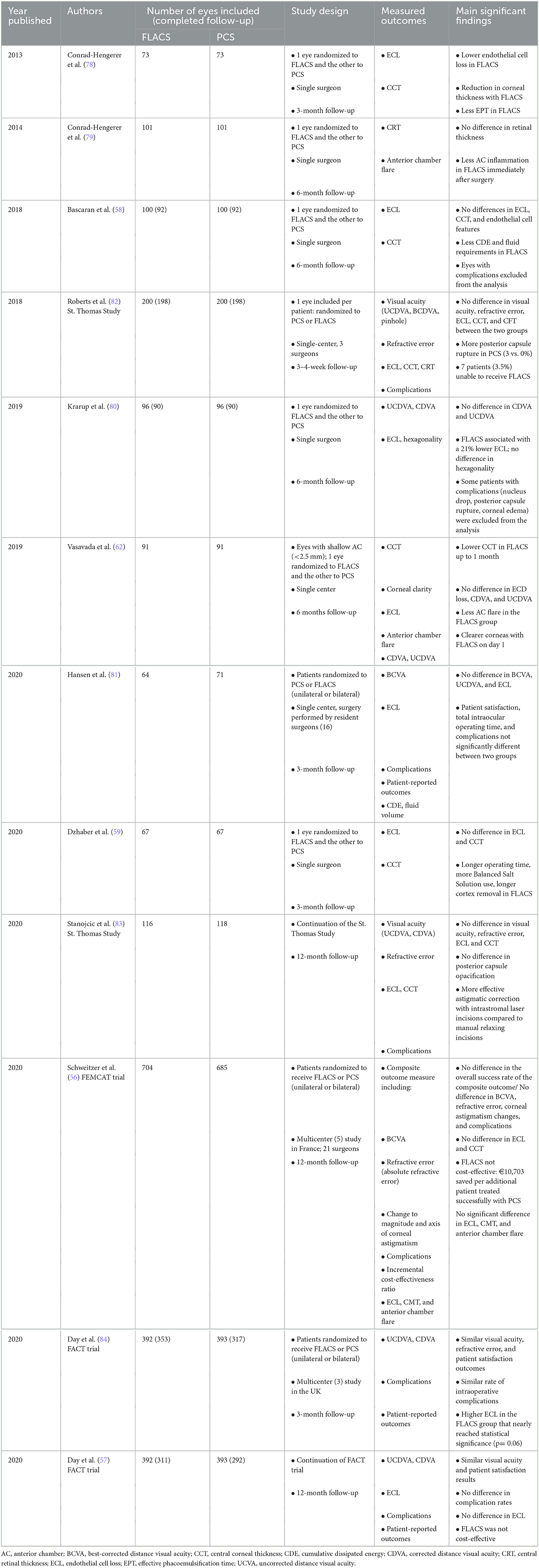
Table 1. Summary of the main randomized clinical trials comparing phacoemulsification cataract surgery (PCS) and femtosecond laser-assisted cataract surgery (FLACS).
Cost-effectiveness
The FLACS technology is expensive both for the hospital in relation to the initial cost of the laser, space, and personnel and for the patient due to the cost of consumables (85). In addition, FLACS still requires the use of a phacoemulsification-aspiration machine to remove lens pieces or viscoelastic devices. Cost-effectiveness is a key aspect of integrating a surgical procedure in the medical landscape; only a few studies examined this in regard to FLACS in published literature. The FEMCAT trial (56) confirmed this hypothesis and concluded that FLACS was more expensive and less effective as compared to PCS, with an incremental cost-effectiveness ratio (ICER) of € 10,703.2 saved per patient successfully treated with PCS. While the FACT trial found FLACS to be non-inferior to PCS, FLACS was also not cost-effective (86).
Roberts et al. (87) designed a model that could make FLACS cost-effective only if efficiency increased by 100% or if the patient interface cost was reduced by 70%.
Learning curve for FLACS
FLACS, similar to any new surgical technique, has a learning curve even for experienced surgeons. Studies have found that, after ~100 cases, the complication rate drops significantly (20, 88). Surgeons familiar with refractive femtosecond lasers may learn FLACS faster (88). Improvements in training techniques and laser software can also contribute to significantly flattening the learning curve (89). However, a survey showed that 82.4% of surgeons felt that 10 cases or less require FLACS to be performed with the same safety as PCS (90).
Although FLACS should also be performed by surgeons who have already mastered PCS, some studies showed that senior residents had similar surgical outcomes between PCS and FLACS (81, 91, 92).
Discussion and perspectives
Femtosecond laser use does not have to be confined to biological tissue interaction only; they can theoretically alter properties of an already implanted IOL changing its refractive index and, in turn, overall power, toricity, inducing/erasing multifocality, or generating pinhole apertures. Such concepts have only been tested in vitro so far (93–95). These techniques might decrease rates of IOL exchange, as those are related to multifocal intolerance and lens power error by 15 and 16%, respectively (96).
Capsulorhexis-fixated IOLs can theoretically benefit from the perfectly round, well-centered, and adequately sized laser capsulotomy (97). Future studies are needed to evaluate whether this will improve ELP and refractive outcomes.
Femtosecond laser technology is likely to evolve as well. Shorter pulse durations could enable higher peak power with less energy per pulse, and a multispot laser treatment could shorten the treatment time. Robotization of the steps of lens aspiration is in its infancy in cataract surgery (98, 99), but this technology could be integrated with FLACS to create fully automated cataract extraction platforms in the future.
Conclusion
Although FLACS offers precise and reproducible technical performances, in its current state of development, these advantages do not significantly translate into clinical practice. To date, FLACS has not been able to tackle most frequent and burdensome complications of cataract surgery such as residual refractive error [24.9 vs. 22% of eyes with an absolute refractive error >0.5D in FLACS and PCS groups, respectively, in FEMCAT (56)], CME, endothelial cell loss, or vitreous loss. Furthermore, FLACS is a technological adaptation from corneal laser surgery aiming to replace steps of PCS, but the use of phacoemulsification machines is still needed to remove lens pieces and viscoelastic devices. However, although FLACS does not seem to benefit the most typical patients with cataract, it may assist in more challenging cases such as traumatic cataracts, Fuchs's dystrophy, low endothelial cell count, and some pediatric cases. In addition, despite the precise and reproducible technical performances of FSL platforms, FLACS remains in its infancy of development. Indeed, the refinement of lasers, the development of new technologies to replace phacoemulsification machines, or new IOL technologies may help improve clinical outcomes and cost-effectiveness over the conventional PCS.
Author contributions
MK and CS have conducted literature research and have written the manuscript. Both authors contributed to the article and approved the submitted version.
Conflict of interest
The authors declare that the research was conducted in the absence of any commercial or financial relationships that could be construed as a potential conflict of interest.
Publisher's note
All claims expressed in this article are solely those of the authors and do not necessarily represent those of their affiliated organizations, or those of the publisher, the editors and the reviewers. Any product that may be evaluated in this article, or claim that may be made by its manufacturer, is not guaranteed or endorsed by the publisher.
References
1. Rossi T, Romano MR, Iannetta D, Romano V, Gualdi L, D'Agostino I, et al. Cataract surgery practice patterns worldwide: a survey. BMJ Open Ophthalmol. (2021) 6:e000464. doi: 10.1136/bmjophth-2020-000464
2. Day AC, Donachie PH, Sparrow JM, Johnston RL, Royal College of Ophthalmologists' National Ophthalmology D. The royal college of ophthalmologists' national ophthalmology database study of cataract surgery: report 1, visual outcomes and complications. Eye. (2015) 29:552–60. doi: 10.1038/eye.2015.3
3. Zaidi FH, Corbett MC, Burton BJ, Bloom PA. Raising the benchmark for the 21st century: the 1,000 cataract operations audit and survey—outcomes, consultant-supervised training and sourcing NHS choice. Br J Ophthalmol. (2007) 91:731–6. doi: 10.1136/bjo.2006.104216
4. Gale RP, Saldana M, Johnston RL, Zuberbuhler B, McKibbin M. Benchmark standards for refractive outcomes after NHS cataract surgery. Eye. (2009) 23:149–52. doi: 10.1038/sj.eye.6702954
5. Kugelberg M, Lundstrom M. Factors related to the degree of success in achieving target refraction in cataract surgery: Swedish national cataract register study. J Cataract Refract Surg. (2008) 34:1935–9. doi: 10.1016/j.jcrs.2008.06.036
6. Lundstrom M, Dickman M, Henry Y, Manning S, Rosen P, Tassignon MJ, et al. Risk factors for refractive error after cataract surgery: analysis of 282,811 cataract extractions reported to the European registry of quality outcomes for cataract and refractive surgery. J Cataract Refract Surg. (2018) 44:447–52. doi: 10.1016/j.jcrs.2018.01.031
7. Krueger RR, Kuszak J, Lubatschowski H, Myers RI, Ripken T, Heisterkamp A. First safety study of femtosecond laser photodisruption in animal lenses: tissue morphology and cataractogenesis. J Cataract Refract Surg. (2005) 31:2386–94. doi: 10.1016/j.jcrs.2005.05.034
8. Nagy Z, Takacs A, Filkorn T, Sarayba M. Initial clinical evaluation of an intraocular femtosecond laser in cataract surgery. J Refract Surg. (2009) 25:1053–60. doi: 10.3928/1081597X-20091117-04
9. Chung SH, Mazur E. Surgical applications of femtosecond lasers. J Biophotonics. (2009) 2:557–72. doi: 10.1002/jbio.200910053
10. Chee SP, Yang Y, Ti SE. Clinical outcomes in the first 2 years of femtosecond laser-assisted cataract surgery. Am J Ophthalmol. (2015) 159:714–9. doi: 10.1016/j.ajo.2015.01.016
11. Scott WJ, Tauber S, Gessler JA, Ohly JG, Owsiak RR, Eck CD. Comparison of vitreous loss rates between manual phacoemulsification and femtosecond laser-assisted cataract surgery. J Cataract Refract Surg. (2016) 42:1003–8. doi: 10.1016/j.jcrs.2016.04.027
12. Day AC, Gore DM, Bunce C, Evans JR. Laser-assisted cataract surgery vs. standard ultrasound phacoemulsification cataract surgery. Cochrane Database Syst Rev. (2016) 7:CD010735. doi: 10.1002/14651858.CD010735.pub2
13. Vogel A, Busch S, Jungnickel K, Birngruber R. Mechanisms of intraocular photodisruption with picosecond and nanosecond laser pulses. Lasers Surg Med. (1994) 15:32–43. doi: 10.1002/lsm.1900150106
14. Heisterkamp A, Ripken T, Mamom T, Drommer W, Welling H, Ertmer W, et al. Nonlinear side effects of fs pulses inside corneal tissue during photodisruption. Appl Phys B. (2002) 74:419–25. doi: 10.1007/s003400200825
15. Kaur M, Titiyal JS, Surve A, Falera R, Verma M. Effect of lens fragmentation patterns on phacoemulsification parameters and postoperative inflammation in femtosecond laser-assisted cataract surgery. Curr Eye Res. (2018) 43:1228–32. doi: 10.1080/02713683.2018.1485951
16. Al Harthi K, Al Shahwan S, Al Towerki A, Banerjee PP, Behrens A, Edward DP. Comparison of the anterior capsulotomy edge created by manual capsulorhexis and 2 femtosecond laser platforms: scanning electron microscopy study. J Cataract Refract Surg. (2014) 40:2106–12. doi: 10.1016/j.jcrs.2014.10.001
17. Talamo JH, Gooding P, Angeley D, Culbertson WW, Schuele G, Andersen D, et al. Optical patient interface in femtosecond laser-assisted cataract surgery: contact corneal applanation vs. liquid immersion. J Cataract Refract Surg. (2013) 39:501–10. doi: 10.1016/j.jcrs.2013.01.021
18. Kanclerz P, Grzybowski A. Does corneal refractive surgery increase the risk of retinal detachment? A literature review and statistical analysis. J Refract Surg. (2019) 35:517–24. doi: 10.3928/1081597X-20190710-02
19. Feldman BH. Femtosecond laser will not be a standard method for cataract extraction 10 years from now. Surv Ophthalmol. (2015) 60:360–5. doi: 10.1016/j.survophthal.2015.02.002
20. Bali SJ, Hodge C, Lawless M, Roberts TV, Sutton G. Early experience with the femtosecond laser for cataract surgery. Ophthalmology. (2012) 119:891–9. doi: 10.1016/j.ophtha.2011.12.025
21. Manning S, Barry P, Henry Y, Rosen P, Stenevi U, Young D, et al. Femtosecond laser-assisted cataract surgery vs. standard phacoemulsification cataract surgery: study from the European registry of quality outcomes for cataract and refractive surgery. J Cataract Refract Surg. (2016) 42:1779–90. doi: 10.1016/j.jcrs.2016.10.013
22. Zhang X, Yu Y, Zhang G, Zhou Y, Zhao G, Chen M, et al. Performance of femtosecond laser-assisted cataract surgery in Chinese patients with cataract: a prospective, multicenter, registry study. BMC Ophthalmol. (2019) 19:77. doi: 10.1186/s12886-019-1079-0
23. Nagy ZZ, Takacs AI, Filkorn T, Kránitz K, Gyenes A, Juhász É, et al. Complications of femtosecond laser-assisted cataract surgery. J Cataract Refract Surg. (2014) 40:20–8. doi: 10.1016/j.jcrs.2013.08.046
24. Alio JL, Abdou AA, Soria F, Javaloy J, Fernández-Buenaga R, Nagy ZZ, et al. Femtosecond laser cataract incision morphology and corneal higher-order aberration analysis. J Refract Surg. (2013) 29:590–5. doi: 10.3928/1081597X-20130819-01
25. Masket S, Sarayba M, Ignacio T, Fram N. Femtosecond laser-assisted cataract incisions: architectural stability and reproducibility. J Cataract Refract Surg. (2010) 36:1048–9. doi: 10.1016/j.jcrs.2010.03.027
26. Chaves M, de Medeiros AL, Vilar CMC, Magalhães KR, Gonçalves MR, de Moraes Tzelikis PF, et al. Architecture evaluation of the main clear corneal incisions in femtosecond laser-assisted cataract surgery by optical coherence tomography imaging. Clin Ophthalmol. (2019) 13:365–72. doi: 10.2147/OPTH.S184024
27. Mastropasqua L, Toto L, Mastropasqua A, Vecchiarino L, Mastropasqua R, Pedrotti E, et al. Femtosecond laser vs. manual clear corneal incision in cataract surgery. J Refract Surg. (2014) 30:27–33. doi: 10.3928/1081597X-20131217-03
28. Mayer WJ, Klaproth OK, Hengerer FH, Kook D, Dirisamer M, Priglinger S, et al. In vitro immunohistochemical and morphological observations of penetrating corneal incisions created by a femtosecond laser used for assisted intraocular lens surgery. J Cataract Refract Surg. (2014) 40:632–8. doi: 10.1016/j.jcrs.2014.02.015
29. Rodrigues R, Santos MSD, Silver RE, Campos M, Gomes RL. Corneal incision architecture: VICTUS femtosecond laser vs. manual keratome. Clin Ophthalmol. (2019) 13:147–52. doi: 10.2147/OPTH.S181144
30. Diakonis VF, Yesilirmak N, Cabot F, Kankariya VP, Kounis GA, Warren D, et al. Comparison of surgically induced astigmatism between femtosecond laser and manual clear corneal incisions for cataract surgery. J Cataract Refract Surg. (2015) 41:2075–80. doi: 10.1016/j.jcrs.2015.11.004
31. Mastropasqua L, Toto L, Calienno R, Mattei PA, Mastropasqua A, Vecchiarino L, et al. Scanning electron microscopy evaluation of capsulorhexis in femtosecond laser-assisted cataract surgery. J Cataract Refract Surg. (2013) 39:1581–6. doi: 10.1016/j.jcrs.2013.06.016
32. Friedman NJ, Palanker DV, Schuele G, Andersen D, Marcellino G, Seibel BS, et al. Femtosecond laser capsulotomy. J Cataract Refract Surg. (2011) 37:1189–98. doi: 10.1016/j.jcrs.2011.04.022
33. Pantanelli SM, Diakonis VF, Al-Mohtaseb Z, Cabot F, Yesilirmak N, Kounis GA, et al. Anterior capsulotomy outcomes: a comparison between two femtosecond laser cataract surgery platforms. J Refract Surg. (2015) 31:821–5. doi: 10.3928/1081597X-20151118-01
34. Norrby S. Sources of error in intraocular lens power calculation. J Cataract Refract Surg. (2008) 34:368–76. doi: 10.1016/j.jcrs.2007.10.031
35. Panthier C, Costantini F, Rigal-Sastourné JC, Brézin A, Mehanna C, Guedj M, et al. Change of capsulotomy over 1 year in femtosecond laser-assisted cataract surgery and its impact on visual quality. J Refract Surg. (2017) 33:44–9. doi: 10.3928/1081597X-20161028-01
36. Reyes Lua M, Oertle P, Camenzind L, Goz A, Meyer CH, Konieczka K, et al. Superior rim stability of the lens capsule following manual over femtosecond laser capsulotomy. Invest Ophthalmol Vis Sci. (2016) 57:2839–49. doi: 10.1167/iovs.15-18355
37. Daya S, Chee SP Ti SE, Packard R, Mordaunt DH. Comparison of anterior capsulotomy techniques: continuous curvilinear capsulorhexis, femtosecond laser-assisted capsulotomy and selective laser capsulotomy. Br J Ophthalmol. (2020) 104:437–42. doi: 10.1136/bjophthalmol-2018-313421
38. Abell RG, Davies PE, Phelan D, Goemann K, McPherson ZE, Vote BJ. Anterior capsulotomy integrity after femtosecond laser-assisted cataract surgery. Ophthalmology. (2014) 121:17–24. doi: 10.1016/j.ophtha.2013.08.013
39. Trinh T, Solomon B, Mimouni M, Cohen E, Gouvea L, Santaella G, et al. Outcomes of femtosecond laser-assisted cataract and refractive lens surgery in patients with prior radial keratotomy. J Cataract Refract Surg. (2022) 48:449–55. doi: 10.1097/j.jcrs.0000000000000784
40. Dick HB, Schultz T. Comment on: Outcomes of femtosecond laser-assisted cataract and refractive lens surgery in patients with prior radial keratotomy. J Cataract Refract Surg. (2022) 48:1223–4. doi: 10.1097/j.jcrs.0000000000001029
41. Day AC, Gartry DS, Maurino V, Allan BD, Stevens JD. Efficacy of anterior capsulotomy creation in femtosecond laser-assisted cataract surgery. J Cataract Refract Surg. (2014) 40:2031–4. doi: 10.1016/j.jcrs.2014.07.027
42. Schultz T, Joachim SC, Kuehn M, Dick HB. Changes in prostaglandin levels in patients undergoing femtosecond laser-assisted cataract surgery. J Refract Surg. (2013) 29:742–7. doi: 10.3928/1081597X-20131021-03
43. Schultz T, Joachim SC, Stellbogen M, Dick HB. Prostaglandin release during femtosecond laser-assisted cataract surgery: main inducer. J Refract Surg. (2015) 31:78–81. doi: 10.3928/1081597X-20150122-01
44. Popiela MZ, Young-Zvandasara T, Nidamanuri P, Moore T, Leccisotti A, Kumar V. Factors influencing pupil behaviour during femtosecond laser assisted cataract surgery. Cont Lens Anterior Eye. (2019) 42:295–8. doi: 10.1016/j.clae.2018.10.010
45. Jun JH, Hwang KY, Chang SD, Joo CK. Pupil-size alterations induced by photodisruption during femtosecond laser-assisted cataract surgery. J Cataract Refract Surg. (2015) 41:278–85. doi: 10.1016/j.jcrs.2014.10.027
46. Schultz T, Joachim SC, Szuler M, Stellbogen M, Dick HB. NSAID pretreatment inhibits prostaglandin release in femtosecond laser-assisted cataract surgery. J Refract Surg. (2015) 31:791–4. doi: 10.3928/1081597X-20151111-01
47. Anisimova NS, Arbisser LB, Petrovski G, Petrichuk SV, Sobolev NP, Petrovski B, et al. Effect of NSAIDs on pupil diameter and expression of aqueous humor cytokines in FLACS vs. conventional phacoemulsification. J Refract Surg. (2018) 34:646–52. doi: 10.3928/1081597X-20180814-02
48. Kiss HJ, Takacs AI, Kranitz K, Sandor GL, Toth G, Gilanyi B, et al. One-day use of preoperative topical nonsteroidal anti-inflammatory drug prevents intraoperative prostaglandin level elevation during femtosecond laser-assisted cataract surgery. Curr Eye Res. (2015) 41:1–4. doi: 10.3109/02713683.2015.1092556
49. Roberts HW, Wagh VK, Sullivan DL, Archer TJ, O'Brart DPS. Refractive outcomes after limbal relaxing incisions or femtosecond laser arcuate keratotomy to manage corneal astigmatism at the time of cataract surgery. J Cataract Refract Surg. (2018) 44:955–63. doi: 10.1016/j.jcrs.2018.05.027
50. Vickers LA, Gupta PK. Femtosecond laser-assisted keratotomy. Curr Opin Ophthalmol. (2016) 27:277–84. doi: 10.1097/ICU.0000000000000267
51. Visco DM, Bedi R, Packer M. Femtosecond laser-assisted arcuate keratotomy at the time of cataract surgery for the management of preexisting astigmatism. J Cataract Refract Surg. (2019) 45:1762–9. doi: 10.1016/j.jcrs.2019.08.002
52. Yoo A, Yun S, Kim JY, Kim MJ, Tchah H. Femtosecond laser-assisted arcuate keratotomy vs. toric IOL implantation for correcting astigmatism. J Refract Surg. (2015) 31:574–8. doi: 10.3928/1081597X-20150820-01
53. Noh H, Yoo YS, Shin KY, Lim DH, Chung TY. Comparison of penetrating femtosecond laser-assisted astigmatic keratotomy and toric intraocular lens implantation for correction of astigmatism in cataract surgery. Sci Rep. (2021) 11:7340. doi: 10.1038/s41598-021-86763-5
54. Hernandez R, Almenara C, Soriano D, Idoipe M, Larrosa JM, Pablo LE, et al. Toric intraocular lens implantation vs. femtosecond laser-assisted arcuate keratotomy for correction of moderate astigmatism in cataract surgery. J Cataract Refract Surg. (2022) 48:887–93. doi: 10.1097/j.jcrs.0000000000000879
55. Abell RG, Kerr NM, Vote BJ. Toward zero effective phacoemulsification time using femtosecond laser pretreatment. Ophthalmology. (2013) 120:942–8. doi: 10.1016/j.ophtha.2012.11.045
56. Schweitzer C, Brezin A, Cochener B, Monnet D, Germain C, Roseng S, et al. Femtosecond laser-assisted vs. phacoemulsification cataract surgery (FEMCAT): a multicentre participant-masked randomised superiority and cost-effectiveness trial. Lancet. (2020) 395:212–24. doi: 10.1016/S0140-6736(19)32481-X
57. Day AC, Burr JM, Bennett K, Doré CJ, Bunce C, Hunter R, et al. Femtosecond laser-assisted cataract surgery compared with phacoemulsification cataract surgery: randomized noninferiority trial with 1-year outcomes. J Cataract Refract Surg. (2020) 46:1360–7. doi: 10.1097/j.jcrs.0000000000000257
58. Bascaran L, Alberdi T, Martinez-Soroa I, Sarasqueta C, Mendicute J. Differences in energy and corneal endothelium between femtosecond laser-assisted and conventional cataract surgeries: prospective, intraindividual, randomized controlled trial. Int J Ophthalmol. (2018) 11:1308–16. doi: 10.18240/ijo.2018.08.10
59. Dzhaber D, Mustafa O, Alsaleh F, Mihailovic A, Daoud YJ. Comparison of changes in corneal endothelial cell density and central corneal thickness between conventional and femtosecond laser-assisted cataract surgery: a randomised, controlled clinical trial. Br J Ophthalmol. (2020) 104:225–9. doi: 10.1136/bjophthalmol-2018-313723
60. Lin CC, Rose-Nussbaumer JR, Al-Mohtaseb ZN, Pantanelli SM, Steigleman WA, Hatch KM, et al. Femtosecond laser-assisted cataract surgery: a report by the American academy of ophthalmology. Ophthalmology. (2022) 129:946–54. doi: 10.1016/j.ophtha.2022.04.003
61. Chen X, Yu Y, Song X, Zhu Y, Wang W, Yao K. Clinical outcomes of femtosecond laser-assisted cataract surgery vs. conventional phacoemulsification surgery for hard nuclear cataracts. J Cataract Refract Surg. (2017) 43:486–91. doi: 10.1016/j.jcrs.2017.01.010
62. Vasavada VA, Vasavada S, Vasavada AR, Vasavada V, Srivastava S. Comparative evaluation of femtosecond laser-assisted cataract surgery and conventional phacoemulsification in eyes with a shallow anterior chamber. J Cataract Refract Surg. (2019) 45:547–52. doi: 10.1016/j.jcrs.2018.11.037
63. Yong WWD, Chai HC, Shen L, Manotosh R, Anna Tan WT. Comparing outcomes of phacoemulsification with femtosecond laser-assisted cataract surgery in patients with fuchs endothelial dystrophy. Am J Ophthalmol. (2018) 196:173–80. doi: 10.1016/j.ajo.2018.08.006
64. BenEzra D, Cohen E. Posterior capsulectomy in pediatric cataract surgery: the necessity of a choice. Ophthalmology. (1997) 104:2168–74. doi: 10.1016/S0161-6420(97)30045-1
65. Dick HB, Schultz T. Femtosecond laser-assisted cataract surgery in infants. J Cataract Refract Surg. (2013) 39:665–8. doi: 10.1016/j.jcrs.2013.02.032
66. Dick HB, Schelenz D, Schultz T. Femtosecond laser-assisted pediatric cataract surgery: Bochum formula. J Cataract Refract Surg. (2015) 41:821–6. doi: 10.1016/j.jcrs.2014.08.032
67. Liao M, Guo D, Liao S, Zhang W, Lin D, Tang Q. Study on the enlargement index of femtosecond laser-assisted capsulorhexis in 2–6-year-old patients with congenital cataract. BMC Ophthalmol. (2021) 21:441. doi: 10.1186/s12886-021-02184-y
68. Hou JH, Crispim J, Cortina MS, Cruz JL. Image-guided femtosecond laser-assisted cataract surgery in Peters anomaly type 2. J Cataract Refract Surg. (2015) 41:2353–7. doi: 10.1016/j.jcrs.2015.10.045
69. Tereshchenko AV, Trifanenkova IG, Vladimirovich VM. Femtosecond laser-assisted anterior and posterior capsulotomies in children with persistent hyperplastic primary vitreous. J Cataract Refract Surg. (2020) 46:497–502. doi: 10.1097/j.jcrs.0000000000000139
70. Daien V, Korobelnik JF, Delcourt C, Cougnard-Gregoire A, Delyfer MN, Bron AM, et al. French medical-administrative database for epidemiology and safety in ophthalmology (EPISAFE): the EPISAFE collaboration program in cataract surgery. Ophthalmic Res. (2017) 58:67–73. doi: 10.1159/000456721
71. Henderson BA, Kim JY, Ament CS, Ferrufino-Ponce ZK, Grabowska A, Cremers SL. Clinical pseudophakic cystoid macular edema. Risk factors for development and duration after treatment. J Cataract Refract Surg. (2007) 33:1550–8. doi: 10.1016/j.jcrs.2007.05.013
72. Lobo C. Pseudophakic cystoid macular edema. Ophthalmologica. (2012) 227:61–7. doi: 10.1159/000331277
73. Koo EH, Bolton EM, Vanner EA, Eghrari AO, Donaldson KE. Outcomes of femtosecond laser-assisted cataract surgery compared to conventional phacoemulsification in eyes with pseudoexfoliation syndrome. Semin Ophthalmol. (2022) 37:631–6. doi: 10.1080/08820538.2022.2051054
74. Toto L, Calienno R, Curcio C, Mattei PA, Mastropasqua A, Lanzini M, et al. Induced inflammation and apoptosis in femtosecond laser-assisted capsulotomies and manual capsulorhexes: an immunohistochemical study. J Refract Surg. (2015) 31:290–4. doi: 10.3928/1081597X-20150423-01
75. Pisciotta A, De Maria M, Verdina T, Fornasari E, de Pol A, Cavallini GM. Anterior capsule of the lens: comparison of morphological properties and apoptosis induction following FLACS and standard phacoemulsification surgery. Biomed Res Int. (2018) 2018:7242837. doi: 10.1155/2018/7242837
76. Sun W, Liu J, Li J, Wu D, Wang J, Wang MW, et al. Human lens epithelial cell apoptosis and epithelial to mesenchymal transition in femtosecond laser-assisted cataract surgery. Int J Ophthalmol. (2018) 11:401–7. doi: 10.18240/ijo.2018.03.09
77. Lundstrom M, Dickman M, Henry Y, Stenevi U, Henry Y, Rosen P. Femtosecond laser-assisted cataract surgeries reported to the European Registry of quality outcomes for cataract and refractive surgery: baseline characteristics, surgical procedure, and outcomes. J Cataract Refract Surg. (2017) 43:1549–56. doi: 10.1016/j.jcrs.2017.09.029
78. Conrad-Hengerer I, Al Juburi M, Schultz T, Hengerer FH, Dick HB. Corneal endothelial cell loss and corneal thickness in conventional compared with femtosecond laser-assisted cataract surgery: 3-month follow-up. J Cataract Refract Surg. (2013) 39:1307–13. doi: 10.1016/j.jcrs.2013.05.033
79. Conrad-Hengerer I, Hengerer FH, Al Juburi M, Schultz T, Dick HB. Femtosecond laser-induced macular changes and anterior segment inflammation in cataract surgery. J Refract Surg. (2014) 30:222–6. doi: 10.3928/1081597X-20140321-01
80. Krarup T, Ejstrup R, Mortensen A, la Cour M, Holm LM. Comparison of refractive predictability and endothelial cell loss in femtosecond laser-assisted cataract surgery and conventional phaco surgery: prospective randomised trial with 6 months of follow-up. BMJ Open Ophthalmol. (2019) 4:e000233. doi: 10.1136/bmjophth-2018-000233
81. Hansen B, Blomquist PH, Ririe P, Pouly S, Nguyen C, Petroll WM, et al. Outcomes of resident-performed laser-assisted vs. traditional phacoemulsification. J Cataract Refract Surg. (2020) 46:1273–7. doi: 10.1097/j.jcrs.0000000000000253
82. Roberts HW, Wagh VK, Sullivan DL, Hidzheva P, Detesan DI, Heemraz BS, et al. A randomized controlled trial comparing femtosecond laser-assisted cataract surgery vs. conventional phacoemulsification surgery. J Cataract Refract Surg. (2019) 45:11–20. doi: 10.1016/j.jcrs.2018.08.033
83. Stanojcic N, Roberts HW, Wagh VK Li JO, Naderi K, O'Brart DP, A. randomised controlled trial comparing femtosecond laser-assisted cataract surgery vs. conventional phacoemulsification surgery: 12-month results. Br J Ophthalmol. (2021) 105:631–8. doi: 10.1136/bjophthalmol-2020-316311
84. Day AC, Burr JM, Bennett K, Bunce C, Doré CJ, Rubin GS, et al. Femtosecond laser-assisted cataract surgery vs. phacoemulsification cataract surgery (FACT): a randomized noninferiority trial. Ophthalmology. (2020) 127:1012–9. doi: 10.1016/j.ophtha.2020.02.028
85. Bartlett JD, Miller KM. The economics of femtosecond laser-assisted cataract surgery. Curr Opin Ophthalmol. (2016) 27:76–81. doi: 10.1097/ICU.0000000000000219
86. Day AC, Burr JM, Bennett K, Hunter R, Bunce C, Doré CJ, et al. Femtosecond laser-assisted cataract surgery compared with phacoemulsification: the FACT non-inferiority RCT. Health Technol Assess. (2021) 25:1–68. doi: 10.3310/hta25060
87. Roberts HW, Ni MZ, O'Brart DPS. Financial modelling of femtosecond laser-assisted cataract surgery within the National Health Service using a ‘hub and spoke' model for the delivery of high-volume cataract surgery. BMJ Open. (2017) 7:e013616. doi: 10.1136/bmjopen-2016-013616
88. Roberts TV, Lawless M, Bali SJ, Hodge C, Sutton G. Surgical outcomes and safety of femtosecond laser cataract surgery: a prospective study of 1,500 consecutive cases. Ophthalmology. (2013) 120:227–33. doi: 10.1016/j.ophtha.2012.10.026
89. Sutton G, Bali SJ, Hodge C. Femtosecond cataract surgery: transitioning to laser cataract. Curr Opin Ophthalmol. (2013) 24:3–8. doi: 10.1097/ICU.0b013e32835a936b
90. Song C, Baharozian CJ, Hatch KM, Talamo JH. Assessment of surgeon experience with femtosecond laser-assisted cataract surgery. Clin Ophthalmol. (2018) 12:1373–7. doi: 10.2147/OPTH.S171743
91. Pittner AC, Sullivan BR. Resident surgeon efficiency in femtosecond laser-assisted cataract surgery. Clin Ophthalmol. (2017) 11:291–7. doi: 10.2147/OPTH.S128626
92. Brunin G, Khan K, Biggerstaff KS, Wang L, Koch DD, Khandelwal SS. Outcomes of femtosecond laser-assisted cataract surgery performed by surgeons-in-training. Graefes Arch Clin Exp Ophthalmol. (2017) 255:805–9. doi: 10.1007/s00417-016-3581-x
93. Kermani O, Dick HB, Lubatschowski H. Femto-masking: laser-generated apertures to extend depth of focus and reduce optical aberrations in intraocular lenses. J Cataract Refract Surg. (2022) 48:1095–6. doi: 10.1097/j.jcrs.0000000000000955
94. Ford J, Werner L, Mamalis N. Adjustable intraocular lens power technology. J Cataract Refract Surg. (2014) 40:1205–23. doi: 10.1016/j.jcrs.2014.05.005
95. Nguyen J, Werner L, Ludlow J, Aliancy J, Ha L, Masino B, et al. Intraocular lens power adjustment by a femtosecond laser: in vitro evaluation of power change, modulation transfer function, light transmission, and light scattering in a blue light-filtering lens. J Cataract Refract Surg. (2018) 44:226–30. doi: 10.1016/j.jcrs.2017.09.036
96. Goemaere J, Trigaux C, Denissen L, Dragnea D, Hua MT, Tassignon MJ, et al. Fifteen years of IOL exchange: indications, outcomes, and complications. J Cataract Refract Surg. (2020) 46:1596–603. doi: 10.1097/j.jcrs.0000000000000349
97. Darian-Smith E, Versace P. Visual performance and positional stability of a capsulorhexis-fixated extended depth-of-focus intraocular lens. J Cataract Refract Surg. (2020) 46:179–87. doi: 10.1097/j.jcrs.0000000000000044
98. Chen CW, Lee YH, Gerber MJ, Cheng H, Yang YC, Govetto A, et al. Intraocular robotic interventional surgical system (IRISS): semi-automated OCT-guided cataract removal. Int J Med Robot. (2018) 14:e1949. doi: 10.1002/rcs.1949
Keywords: cataract, cataract surgery, femtosecond laser (fs), femtosecond laser assisted cataract surgery (FLACS), phacoemulsification
Citation: Kecik M and Schweitzer C (2023) Femtosecond laser-assisted cataract surgery: Update and perspectives. Front. Med. 10:1131314. doi: 10.3389/fmed.2023.1131314
Received: 24 December 2022; Accepted: 07 February 2023;
Published: 02 March 2023.
Edited by:
Rachid Tahiri, Avranches Granville Hospital, FranceReviewed by:
Lijun Shen, Eye Hospital and School of Ophthalmology and Optometry, ChinaBoris E. Malyugin, S. Fyodorov Eye Microsurgery Federal State Institution, Russia
Wajdene Ghouali, National Hospital Center of Ophthalmology Quinze-Vingts, France
Copyright © 2023 Kecik and Schweitzer. This is an open-access article distributed under the terms of the Creative Commons Attribution License (CC BY). The use, distribution or reproduction in other forums is permitted, provided the original author(s) and the copyright owner(s) are credited and that the original publication in this journal is cited, in accordance with accepted academic practice. No use, distribution or reproduction is permitted which does not comply with these terms.
*Correspondence: Cedric Schweitzer, cedric.schweitzer@chu-bordeaux.fr