- Department of Pharmacology, Faculty of Medicine, Kagawa University, Takamatsu, Japan
Treatment with a sodium glucose cotransporter 2 (SGLT2) inhibitor in patients with chronic kidney disease reduces the renal risk independent of changes in blood glucose concentrations and blood pressure. However, the precise mechanism responsible for this SGLT2 inhibitor-induced renoprotective effect is unclear. We have previously shown that SGLT2 inhibitors induce antihypertensive effects with decreased sympathetic nerve activity, which is associated with transient natriuresis. Furthermore, treatment with an SGLT2 inhibitor improves renal ischemia by producing vascular endothelial growth factor-a in the renal tubules. Other studies have suggested that ketone body production, changes in glomerular hemodynamics, and intrarenal metabolic changes and a reduction in oxidative stress due to decreased tubulointerstitial glucose levels may also be involved in the renoprotective effects of SGLT2 inhibitors. In this review, we summarize the mechanism responsible for the SGLT2 inhibitor-induced renoprotective effects, including our recent hypothesis regarding an “aestivation-like response,” which is a biological defense response to starvation.
1. Introduction
Sodium glucose cotransporter 2 (SGLT2) is primary expressed in the S1 and S2 segments of the proximal tubules. Therefore, SGLT2 inhibitors suppress glucose reabsorption at the proximal tubules and induce glucosuria. As a result, a reduction in blood sugar concentrations is accompanied by caloric loss (1, 2). However, recent large-scale, clinical trials have shown that treatment with SGLT2 inhibitors have improved renal outcome not only in patients with diabetic kidney disease (DKD), but also in patients with chronic kidney disease (CKD) without diabetes (3–5). Although complex factors may be involved in the SGLT2 inhibitor-induced renoprotective effects, the precise mechanism involved is unclear.
SGLT2 is a glucose transporter that reabsorbs glucose and sodium ions together in a 1:1 ratio in the S1 and S2 segments of the proximal tubule (1). Therefore, SGLT2 inhibitors should block the reabsorption of glucose and sodium ions and increase their concentration in the tubular lumen at this site. However, other glucose transporters and sodium channels are located at distal sites, which may buffer the effects of an SGLT2 inhibitor to some extent. Approximately 90% of the glucose filtered by the glomerulus is thought to be reabsorbed by SGLT2 expressed at the S1 and S2 segments of the proximal tubule, whereas the remaining 10% is reabsorbed by SGLT1 expressed at the distal S3 segment of the proximal tubule (1). Therefore, even when SGLT2 is completely inhibited by SGLT2 inhibitors, a certain amount of increased glucose in the tubular lumen due to inhibited reabsorption is reabsorbed by SGLT1 expressed in the more distal segment. SGLT1 has a high reserve capacity for glucose reabsorption and can reabsorb one glucose and two sodium ions together (1). Therefore, SGLT1 is assumed to reabsorb large amounts of sodium because of its high glucose reabsorption reserve capacity. Consequently, although an SGLT2 inhibitor is a simple agent that inhibits SGLT2 in the proximal tubular S1 and S2 segments, it may considerably alter the environment within the kidney. In this review article, we briefly discuss our hypotheses regarding the possible mechanism of SGLT2 inhibitor-induced renoprotective effects.
2. SGLT2 inhibitor-induced systemic changes and their renoprotective effects
2.1. Hypoglycemic effect
SGLT2 inhibitors were developed as hypoglycemic agents to control blood glucose concentrations in patients with diabetes. In patients with DKD, lowering blood glucose concentrations elicits a beneficial effect on renal injury. Several studies have shown that high glucose concentrations cause oxidative stress in the kidney, which induces renal tissue injury (6). Obesity also causes metabolic abnormalities in the kidney, which may be ameliorated by caloric loss with an SGLT2 inhibitor. Tanaka et al. (7) showed that the administration of an SGLT2 inhibitor or dietary restriction improves kidney tissue metabolism and oxidative stress in obese type 2 diabetic mice.
Ketone bodies are produced in the body as energy when blood glucose concentrations are lowered owing to caloric loss (8). Tomita et al. (9) showed that ketone bodies produced by SGLT2 inhibitor administration led to a protective effect on glomerular and tubular injury in obese diabetic mice. However, caloric loss promotes gluconeogenesis in the kidney. To the best of our knowledge, a specific effect of gluconeogenesis on renal injury has not yet been clarified.
2.2. Blood pressure reduction
In hypertensive patients with CKD, adequate blood pressure control is important to attenuate the progression of renal dysfunction (10). SGLT2 inhibitors have been clinically shown to have an antihypertensive effect (11). We have also reported that, in the Otsuka Long Evans Tokushima Fatty rat (animal model of obesity) and in the SHR/NDmcr-cp animal model of metabolic syndrome, treatment of an SGLT2 inhibitor normalized the dipping pattern of blood pressure in addition to its antihypertensive effects (12, 13). The pharmacological mechanism for the antihypertensive effect of SGLT2 inhibitors may be through weight loss and hypoglycemia. However, most of the antihypertensive effects of SGLT2 inhibitors do not correlate with changes in body weight or blood glucose concentrations (11). Another possible mechanism is the improvement of insulin resistance by SGLT2 inhibitors. Clinical studies have reported that hyperinsulinemia may contribute to high blood pressure by sodium increasing sodium reabsorption in the kidney (14). However, in the above-mentioned animal studies with Otsuka Long Evans Tokushima Fatty rats and the SHR/NDmcr-cp model, an improvement in diurnal blood pressure variability by SGLT2 inhibitors was accompanied by increased urinary sodium excretion (12, 13, 15). These data suggest that the natriuretic effect of SGLT2 inhibitors is also involved in lowering blood pressure. In fact, our clearance studies in rats showed that acute administration of an SGLT2 inhibitor induced a transient increase in urinary sodium ion excretion, which was accompanied by an increase in urinary glucose concentrations (16).
2.3. Aestivation-like response
SGLT2 inhibitors suppress the reabsorption of glucose and sodium at the proximal tubule, and urinary glucose becomes an osmolyte in the urine and induces osmotic diuresis (1). These effects of SGLT2 inhibitors should be associated with glucose loss and fluid loss. With regard to glucose loss due to SGLT2 inhibitors, the hypoglycemic effect is likely to continue because of the sustained increase in urinary glucose concentrations during treatment. However, in response to such caloric loss, hunger is experienced, and energy is achieved through internal ketone body production and glucogenesis (8). Therefore, many clinical reports have shown that weight loss does not continue for a long period of time and is limited to a loss of 2–3 kg in a few months (3–5). However, a reduction in body fluid induced by an SGLT2 inhibitor is accompanied by osmotic diuresis, which lasts only a few days (17). Immediately after SGLT2 inhibitor administration, diuresis (i.e., fluid loss) with increased urine volume and urinary sodium excretion occurs for several days, but this diuretic effect usually diminishes soon thereafter owing to the adaptive activation of the renin–angiotensin and vasopressin systems (18). Therefore, although SGLT2 inhibitors cause caloric and fluid loss, various compensatory mechanisms in the body are triggered to counteract these losses. As a result, the organism is maintained in a constant state, which is thought to minimize the appearance of serious side effects of an SGLT2 inhibitor.
Among the various compensatory mechanisms that occur in vivo upon SGLT2 inhibitor administration, we have proposed the possibility of an “aestivation-like response” as an adaptive response (19, 20). Aestivation is observed in lungfish and amphibians, and it retains body fluids by producing osmotic substances such as urea (21). We recently found that part of this process is also maintained in humans, which we named the “aestivation-like response” (21). Interestingly, metabolomic analysis of metabolic changes in patients with type 2 diabetes has shown that treatment with an SGLT2 inhibitor promotes a catabolic state, which is involved in the urea cycle and branched differentiated amino acids (22). Our data have also shown that, during an aestivation-like response, energy shifts to the urea cycle and other processes occur, and sympathetic nerves are inhibited accordingly (23). Similarly, several studies have shown that SGLT2 inhibitor administration suppresses sympathetic nerve activity (24, 25). These findings suggest that caloric and body fluid losses due to SGLT2 inhibitor administration may induce metabolic energy and water conservation. This conservation is associated with an aestivation-like response, such as protein catabolism and the suppression of sympathetic nerve activity (19, 20, 23). We speculate that the body’s latent biological defense capacity is activated by an SGLT2 inhibitor, which may be partly responsible for organ protection. Obviously, our hypothesis is still speculative and requires further validation. The differences between “aestivation” and “aestivation-like response” are briefly summarized in Table 1.
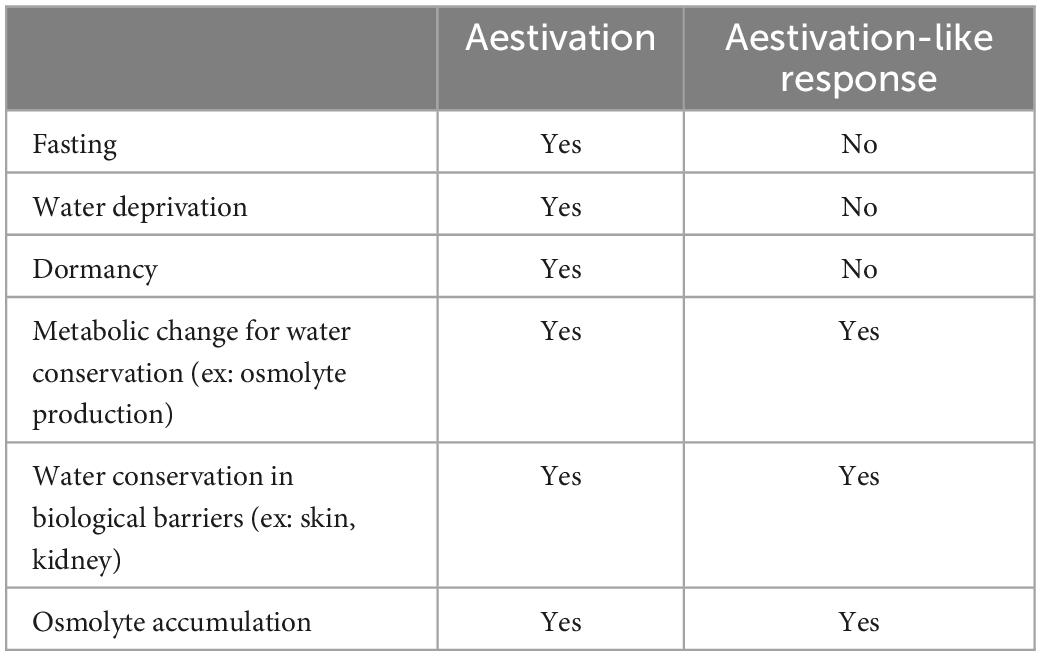
Table 1. Similarities and differences between aestivation and aestivation-like response for water conservation.
3. SGLT2 inhibitor-induced changes in renal hemodynamics and their renoprotective effects
3.1. Changes in glomerular hemodynamics
Large clinical trials have indicated that an improvement in renal outcome with SGLT2 inhibitors is accompanied by a reduction in the glomerular filtration rate (GFR) and albuminuria (3–5). These data suggest that a reduction in intraglomerular pressure plays an important role in the renoprotective effect of SGLT2 inhibitors. Several investigators have suggested that a reduction in intraglomerular pressure by SGLT2 inhibitors is induced by the activation of a tubuloglomerular glomerular feedback (TGF) mechanism (2, 26). When SGLT2 inhibitors are administered, a large amount of solutes, such as glucose and sodium ions, reach the macula densa at the distal tubules where TGF mechanism is activated (27). Macula densa cells sense an increase in solutes in the tubular lumen and release ATP and adenosine extracellularly. This release causes the nearby glomerular afferent arterioles to constrict, leading to a reduction in intraglomerular pressure (28). Therefore, SGLT2 inhibitors may reduce intraglomerular pressure (simultaneously lowering GFR), thereby improving glomerular hyperfiltration and protecting the kidney. However, no reports have shown a positive relationship between the incidence of renal events and a reduction in the GFR or albuminuria. In addition, animal studies in both mice and rats have shown inconsistent data on the effects of SGLT2 inhibitors on the GFR and albuminuria (15, 16). Another study reported that SGLT2 inhibitors affect mesangial cellular contraction, which is possibly involved in the regulation of the GFR by the TGF mechanism (29).
Recently, Hare et al. (30) showed that, in a rat model of type 1 diabetes, SGLT2 inhibitor treatment decreased tissue oxygen saturation in the deep renal cortex and outer medulla. This decrease was associated with a decreased GFR and increased blood erythropoietin expression and erythrocyte reticulocyte count. In contrast, no such changes were observed in other studies (31, 32). Therefore, these data suggest that the effects of SGLT2 inhibitors in promoting erythropoietin production in the kidney differ depending on the pathogenesis of CKD.
3.2. Other hemodynamic changes
SGLT2 inhibitors increase urinary excretion of glucose and cause osmotic diuresis because glucose is an osmolyte. Water is thought to move from the renal interstitium into the urine, which is associated with a reduction in the amount of renal interstitial water. These effects of SGLT2 inhibitors have been suggested to improve renal congestion (33, 34). Preliminary studies by Hirose et al. (34) showed that treatment with SGLT2 inhibitors improved renal congestion in a model of renal venous coarctation. We also showed that, in a mouse model of renal fibrosis after ischemia–reperfusion, an SGLT2 inhibitor markedly improved renal congestion via increased tubular vascular endothelial growth factor-a expression (35). Therefore, SGLT2 inhibitors may improve hemodynamics in perivascular vessels and in the glomeruli. More detailed studies on this possibility are expected in the future.
4. SGLT2 inhibitor-induced reduction in renal tubulointerstitial glucose concentrations and its renoprotective effects
As mentioned above, SGLT2 is expressed primarily in the S1 and S2 segments of the proximal tubules, which reabsorb glucose and sodium in a 1:1 ratio. Therefore, SGLT2 inhibitors may reduce glucose concentrations in the tubular cells and interstitial space in this area. However, a detailed study showed that, in normal mice, even when glucose reabsorption was inhibited by an SGLT2 inhibitor, glucose concentrations in the S1 segment proximal tubular cells remained relatively constant because glucose flowed in from the interstitial side via glucose transporter type 2 (GLUT2) (35, 36). Interestingly, this compensatory response does not work well in damaged kidneys because GLUT2 expression and function are impaired. As a result, glucose concentrations in proximal tubular cells at the S1 and S2 segments are considerably reduced by treatment with an SGLT2 inhibitor (35). Therefore, in the pathogenesis of CKD, an SGLT2 inhibitor may reduce glucose concentrations in proximal tubular cells and the interstitial space, at least near the S1 and S2 segments, which elicit various effects on tubulointerstitial function. A growing body of experimental evidence has shown that, in the proximal tubular S1 and S2 segments, SGLT2 inhibitors suppress glucose-induced tubular cell epithelial-mesenchymal transition (37), oxidative stress (6, 7), and aging (38). On the other hand, in the distal proximal tubular S3 segment, where SGLT2 is not expressed but SGLT1 is abundant instead (1), SGLT2 inhibitors may increase intracellular glucose concentrations. However, these effects of SGLT2 inhibitors have not been studied in detail.
The effects of an SGLT2 inhibitor have been studied in animal models of polycystic kidney disease (PKD) in which lesions occur further downstream in the collecting ducts (39, 40). In PKD Han: SPRD rats, the administration of an SGLT2 inhibitor did not change cyst size, while the GFR was increased and albuminuria was attenuated (40). However, in PKD PCK rats, treatment with an SGLT2 inhibitor worsened albuminuria and the cyst size (39). These experimental data suggest that SGLT2 inhibitor administration is not recommended in patients with PKD. Thus, SGLT2 inhibitor administration may greatly alter the intrarenal environment not only at the proximal tubular S1 and S2 segments, but also at the distal nephron, and careful observation is required in the future.
5. Discussion
SGLT2 inhibitors have attracted attention as agents that improve the prognosis of CKD. This review outlines the currently proposed mechanisms by which SGLT2 inhibitors elicit renoprotective effects. Further determination of the mechanism responsible for SGLT2 inhibitor-induced renoprotective effects will likely lead to the development of new drugs for patients with CKD. Several SGLT2 inhibitors are currently available, but only a limited number of them have proven renoprotective effects. However, based on their respective drug profiles, it is possible that all SGLT2 inhibitors to have similar renoprotective effects.
Author contributions
AN: manuscript draft preparation and writing—review and editing. KK: review and editing. Both authors contributed to the article and approved the submitted version.
Funding
This study was supported by the Grants-in-Aid for Scientific Research from the Ministry of Education, Science, and Culture of Japan (22H03514 and 22K19712 to AN).
Acknowledgments
We thank Ellen Knapp Ph.D. from Edanz (https://jp.edanz.com/ac) for editing the draft of this manuscript.
Conflict of interest
AN has received speaking honoraria from Taisho, Daiichi-Sankyo, Mochida, Bayer, AstraZeneca, and Tanabe-Mitsubishi and has received research funds from Daiichi-Sankyo, Boehringer Ingelheim, Bayer, and Taisho. The funders had no role in the study design, data collection and analysis, decision to publish, or preparation of the manuscript.
The remaining author declares that the research was conducted in the absence of any commercial or financial relationships that could be construed as a potential conflict of interest.
Publisher’s note
All claims expressed in this article are solely those of the authors and do not necessarily represent those of their affiliated organizations, or those of the publisher, the editors and the reviewers. Any product that may be evaluated in this article, or claim that may be made by its manufacturer, is not guaranteed or endorsed by the publisher.
References
1. Ghezzi C, Loo DDF, Wright EM. Physiology of renal glucose handling via SGLT1, SGLT2 and GLUT2. Diabetologia. (2018) 61:2087–97. doi: 10.1007/s00125-018-4656-5
2. Vallon V, Thomson SC. The tubular hypothesis of nephron filtration and diabetic kidney disease. Nat Rev Nephrol. (2020) 16:317–36. doi: 10.1038/s41581-020-0256-y
3. EMPA-KIDNEY Collaborative Group, Herrington WG, Staplin N, Wanner C, Green JB, Hauske SJ, et al. Empagliflozin in patients with chronic kidney disease. N Engl J Med. (2022) 388:117–27. doi: 10.1056/NEJMoa2204233
4. Heerspink HJL, Stefansson BV, Correa-Rotter R, Chertow GM, Greene T, Hou FF, et al. Dapagliflozin in patients with chronic kidney disease. N Engl J Med. (2020) 383:1436–46. doi: 10.1056/NEJMoa2024816
5. Perkovic V, Jardine MJ, Neal B, Bompoint S, Heerspink HJL, Charytan DM, et al. Canagliflozin and renal outcomes in type 2 diabetes and nephropathy. N Engl J Med. (2019) 380:2295–306. doi: 10.1056/NEJMoa1811744
6. Hatanaka T, Ogawa D, Tachibana H, Eguchi J, Inoue T, Yamada H, et al. Inhibition of SGLT2 alleviates diabetic nephropathy by suppressing high glucose-induced oxidative stress in type 1 diabetic mice. Pharmacol Res Perspect. (2016) 4:e00239. doi: 10.1002/prp2.239
7. Tanaka S, Sugiura Y, Saito H, Sugahara M, Higashijima Y, Yamaguchi J, et al. Sodium-glucose cotransporter 2 inhibition normalizes glucose metabolism and suppresses oxidative stress in the kidneys of diabetic mice. Kidney Int. (2018) 94:912–25. doi: 10.1016/j.kint.2018.04.025
8. Lupsa BC, Kibbey RG, Inzucchi SE. Ketones: the double-edged sword of SGLT2 inhibitors? Diabetologia. (2022) 66:23–32. doi: 10.1007/s00125-022-05815-1
9. Tomita I, Kume S, Sugahara S, Osawa N, Yamahara K, Yasuda-Yamahara M, et al. SGLT2 inhibition mediates protection from diabetic kidney disease by promoting ketone body-induced mTORC1 inhibition. Cell Metab. (2020) 32:404–19.e406. doi: 10.1016/j.cmet.2020.06.020
10. Tsuboi N, Sasaki T, Okabayashi Y, Haruhara K, Kanzaki G, Yokoo T. Assessment of nephron number and single-nephron glomerular filtration rate in a clinical setting. Hypertens Res. (2021) 44:605–17. doi: 10.1038/s41440-020-00612-y
11. Rahman A, Hitomi H, Nishiyama A. Cardioprotective effects of SGLT2 inhibitors are possibly associated with normalization of the circadian rhythm of blood pressure. Hypertens Res. (2017) 40:535–40. doi: 10.1038/hr.2016.193
12. Rahman A, Fujisawa Y, Nakano D, Hitomi H, Nishiyama A. Effect of a selective SGLT2 inhibitor, luseogliflozin, on circadian rhythm of sympathetic nervous function and locomotor activities in metabolic syndrome rats. Clin Exp Pharmacol Physiol. (2017) 44:522–5. doi: 10.1111/1440-1681.12725
13. Takeshige Y, Fujisawa Y, Rahman A, Kittikulsuth W, Nakano D, Mori H, et al. A sodium-glucose co-transporter 2 inhibitor empagliflozin prevents abnormality of circadian rhythm of blood pressure in salt-treated obese rats. Hypertens Res. (2016) 39:415–22. doi: 10.1038/hr.2016.2
14. Kario K, Okada K, Kato M, Nishizawa M, Yoshida T, Asano T, et al. 24-hour blood pressure-lowering effect of an SGLT-2 inhibitor in patients with diabetes and uncontrolled nocturnal hypertension: results from the randomized, placebo-controlled SACRA study. Circulation. (2018) 139:2089–97. doi: 10.1161/CIRCULATIONAHA.118.037076
15. Rahman A, Kittikulsuth W, Fujisawa Y, Sufiun A, Rafiq K, Hitomi H, et al. Effects of diuretics on sodium-dependent glucose cotransporter 2 inhibitor-induced changes in blood pressure in obese rats suffering from the metabolic syndrome. J Hypertens. (2016) 34:893–906. doi: 10.1097/HJH.0000000000000871
16. Ansary TM, Fujisawa Y, Rahman A, Nakano D, Hitomi H, Kobara H, et al. Responses of renal hemodynamics and tubular functions to acute sodium-glucose cotransporter 2 inhibitor administration in non-diabetic anesthetized rats. Sci Rep. (2017) 7:9555. doi: 10.1038/s41598-017-09352-5
17. Yasui A, Lee G, Hirase T, Kaneko T, Kaspers S, von Eynatten M, et al. Empagliflozin induces transient diuresis without changing long-term overall fluid balance in japanese patients with type 2 diabetes. Diabetes Ther. (2018) 9:863–71. doi: 10.1007/s13300-018-0385-5
18. Ansary TM, Nakano D, Nishiyama A. Diuretic effects of sodium glucose cotransporter 2 inhibitors and their influence on the renin-angiotensin system. Int J Mol Sci. (2019) 20:629. doi: 10.3390/ijms20030629
19. Kitada K, Kidoguchi S, Nakano D, Nishiyama A. Sodium/glucose cotransporter 2 and renoprotection: from the perspective of energy regulation and water conservation. J Pharmacol Sci. (2021) 147:245–50. doi: 10.1016/j.jphs.2021.07.006
20. Marton A, Kaneko T, Kovalik JP, Yasui A, Nishiyama A, Kitada K, et al. Organ protection by SGLT2 inhibitors: role of metabolic energy and water conservation. Nat Rev Nephrol. (2021) 17:65–77. doi: 10.1038/s41581-020-00350-x
21. Kitada K, Daub S, Zhang Y, Klein JD, Nakano D, Pedchenko T, et al. High salt intake reprioritizes osmolyte and energy metabolism for body fluid conservation. J Clin Invest. (2017) 127:1944–59. doi: 10.1172/JCI88532
22. Kappel BA, Lehrke M, Schutt K, Artati A, Adamski J, Lebherz C, et al. Effect of empagliflozin on the metabolic signature of patients with type 2 diabetes mellitus and cardiovascular disease. Circulation. (2017) 136:969–72. doi: 10.1161/CIRCULATIONAHA.117.029166
23. Morisawa N, Kitada K, Fujisawa Y, Nakano D, Yamazaki D, Kobuchi S, et al. Renal sympathetic nerve activity regulates cardiovascular energy expenditure in rats fed high salt. Hypertens Res. (2020) 43:482–91. doi: 10.1038/s41440-019-0389-1
24. Shimizu W, Kubota Y, Hoshika Y, Mozawa K, Tara S, Tokita Y, et al. Effects of empagliflozin versus placebo on cardiac sympathetic activity in acute myocardial infarction patients with type 2 diabetes mellitus: the EMBODY trial. Cardiovasc Diabetol. (2020) 19:148. doi: 10.1186/s12933-020-01127-z
25. Wan N, Fujisawa Y, Kobara H, Masaki T, Nakano D, Rahman A, et al. Effects of an SGLT2 inhibitor on the salt sensitivity of blood pressure and sympathetic nerve activity in a nondiabetic rat model of chronic kidney disease. Hypertens Res. (2020) 43:492–9. doi: 10.1038/s41440-020-0410-8
26. Kashihara N, Kidokoro K, Kanda E. Renoprotective effects of sodium-glucose cotransporter-2 inhibitors and underlying mechanisms. Curr Opin Nephrol Hypertens. (2020) 29:112–8. doi: 10.1097/MNH.0000000000000561
27. Nishiyama A, Rahman M, Inscho EW. Role of interstitial ATP and adenosine in the regulation of renal hemodynamics and microvascular function. Hypertens Res. (2004) 27:791–804. doi: 10.1291/hypres.27.791
28. Kidokoro K, Cherney DZI, Bozovic A, Nagasu H, Satoh M, Kanda E, et al. Evaluation of glomerular hemodynamic function by empagliflozin in diabetic mice using in vivo imaging. Circulation. (2019) 140:303–15. doi: 10.1161/CIRCULATIONAHA.118.037418
29. Wakisaka M, Nagao T, Yoshinari M. Sodium glucose cotransporter 2 (SGLT2) plays as a physiological glucose sensor and regulates cellular contractility in rat mesangial cells. PLoS One. (2016) 11:e0151585. doi: 10.1371/journal.pone.0151585
30. Hare GMT, Zhang Y, Chin K, Thai K, Jacobs E, Cazorla-Bak MP, et al. Impact of sodium glucose linked cotransporter-2 inhibition on renal microvascular oxygen tension in a rodent model of diabetes mellitus. Physiol Rep. (2021) 9:e14890. doi: 10.14814/phy2.14890
31. Masuda T, Watanabe Y, Fukuda K, Watanabe M, Onishi A, Ohara K, et al. Unmasking a sustained negative effect of SGLT2 inhibition on body fluid volume in the rat. Am J Physiol Renal Physiol. (2018) 315:F653–64. doi: 10.1152/ajprenal.00143.2018
32. Yamazaki D, Konishi Y, Morikawa T, Kobara H, Masaki T, Hitomi H, et al. Failure to confirm a sodium-glucose cotransporter 2 inhibitor-induced hematopoietic effect in non-diabetic rats with renal anemia. J Diabetes Investig. (2020) 11:834–43. doi: 10.1111/jdi.13205
33. Kuriyama S. A potential mechanism of cardio-renal protection with sodium-glucose cotransporter 2 inhibitors: amelioration of renal congestion. Kidney Blood Press Res. (2019) 44:449–56. doi: 10.1159/000501081
34. Hirose T, Mstuki T, Takahashi C, Yamakoshi S, Oba-Yabana I, Endo A, et al. Renoprotective effect of SGLT1/2 inhibitor in rat renal congestion model. Hypertension. (2020) 76:A038. doi: 10.1161/hyp.76.suppl_1.P038
35. Zhang Y, Nakano D, Guan Y, Hitomi H, Uemura A, Masaki T, et al. A sodium-glucose cotransporter 2 inhibitor attenuates renal capillary injury and fibrosis by a vascular endothelial growth factor-dependent pathway after renal injury in mice. Kidney Int. (2018) 94:524–35. doi: 10.1016/j.kint.2018.05.002
36. Zhang A, Nakano D, Kittikulsuth W, Yamashita Y, Nishiyama A. Luseogliflozin, a SGLT2 inhibitor, does not affect glucose uptake kinetics in renal proximal tubules of live mice. Int J Mol Sci. (2021) 22:8169. doi: 10.3390/ijms22158169
37. Li J, Liu H, Takagi S, Nitta K, Kitada M, Srivastava SP, et al. Renal protective effects of empagliflozin via inhibition of EMT and aberrant glycolysis in proximal tubules. JCI Insight. (2020) 5:e129034. doi: 10.1172/jci.insight.129034
38. Umino H, Hasegawa K, Minakuchi H, Muraoka H, Kawaguchi T, Kanda T, et al. High basolateral glucose increases sodium-glucose cotransporter 2 and reduces sirtuin-1 in renal tubules through glucose transporter-2 detection. Sci Rep. (2018) 8:6791. doi: 10.1038/s41598-018-25054-y
39. Kapoor S, Rodriguez D, Riwanto M, Edenhofer I, Segerer S, Mitchell K, et al. Effect of sodium-glucose cotransport inhibition on polycystic kidney disease progression in PCK rats. PLoS One. (2015) 10:e0125603. doi: 10.1371/journal.pone.0125603
Keywords: SGLT2 inhibitor, chronic kidney disease (CKD), diabetic kidney disease (DKD), renal function, metabolism, aestivation-like response
Citation: Nishiyama A and Kitada K (2023) Possible renoprotective mechanisms of SGLT2 inhibitors. Front. Med. 10:1115413. doi: 10.3389/fmed.2023.1115413
Received: 03 December 2022; Accepted: 31 January 2023;
Published: 09 March 2023.
Edited by:
Masaaki Nakayama, St. Luke’s International University, JapanReviewed by:
Masashi Mizuno, Nagoya University, JapanCopyright © 2023 Nishiyama and Kitada. This is an open-access article distributed under the terms of the Creative Commons Attribution License (CC BY). The use, distribution or reproduction in other forums is permitted, provided the original author(s) and the copyright owner(s) are credited and that the original publication in this journal is cited, in accordance with accepted academic practice. No use, distribution or reproduction is permitted which does not comply with these terms.
*Correspondence: Akira Nishiyama, bmlzaGl5YW1hLmFraXJhQGthZ2F3YS11LmFjLmpw