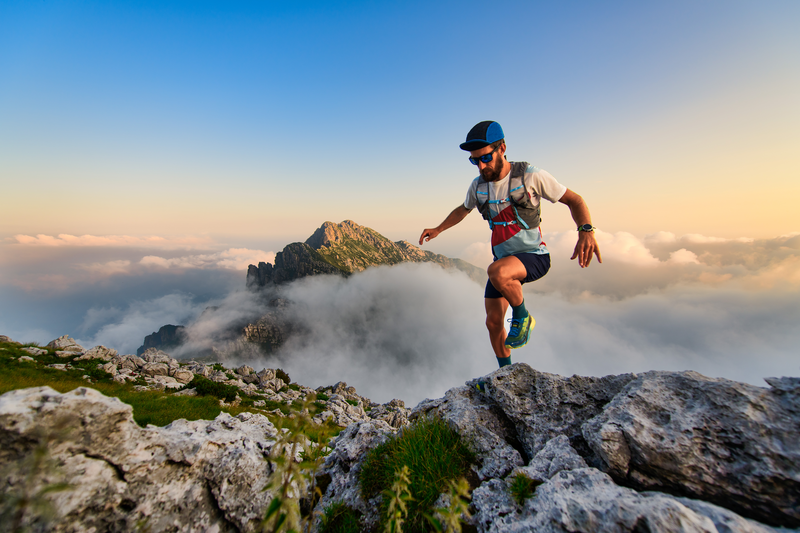
95% of researchers rate our articles as excellent or good
Learn more about the work of our research integrity team to safeguard the quality of each article we publish.
Find out more
REVIEW article
Front. Med. , 26 October 2022
Sec. Ophthalmology
Volume 9 - 2022 | https://doi.org/10.3389/fmed.2022.999251
This article is part of the Research Topic Reviews in: Ophthalmology 2022 View all 22 articles
A correction has been applied to this article in:
Corrigendum: Ocular effects caused by viral infections and corresponding vaccines: An overview of varicella zoster virus, measles virus, influenza viruses, hepatitis B virus, and SARS-CoV-2
Many viral infections can affect vision and the visual system. Vaccination to prevent diseases is commonplace today, acting by stimulating an immune response without developing the pathology. It involves the production of persisting antibodies against the pathogen and the activation of T cells. Certain diseases have already been eradicated by rigorous vaccination campaigns, while others are hoped to be eliminated soon. Vaccines currently available on the market are largely safe, even if they can rarely cause some adverse effects, such as ocular complications. Analyzing existing literature, we aimed to compare the pathological effects on the eye due to the most common viral infections [in particular varicella zoster virus (VZV), measles virus, influenza viruses, hepatitis B virus, and SARS-CoV-2] with the possible ocular adverse effects of their relative vaccines, in order to establish a risk-benefit relationship from an ophthalmological point of view.
Vaccine safety represents a very topical theme, especially in the most developed Countries. Some people consider vaccination as unnecessary and potentially harmful, whereas the infectious risk is perceived as irrelevant, often because they ignore the potential harmful effects of diseases. Focusing on the ocular system, we decided to analyze current literature aiming to compare the possible ocular effects caused by different pathogens with those potentially caused by the administration of their related vaccines, to highlight the benefit-risk ratio of vaccination.
Currently, the main pathogens against which effective vaccinations are available are: Varicella Zoster Virus (VZV), Corynebacterium Diphtheriae, Poliovirus, Hepatitis B Virus (HBV), Hepatitis A Virus, Haemophilus Influenzae type B (Hib), Pneumococcus, Meningococcus, Measles Virus (MeV), Rubella Virus, Human Papilloma Virus (HPV), Rotavirus, several Influenza Viruses (IVs), and recently also SARS-CoV-2. In developed Countries, vaccination against these pathogens is largely mandatory or strongly recommended by the competent authorities. In our analysis we will especially focus on pathogens which have been shown to have more frequently serious impact on ocular structures: VZV, MeV, IVs, HBV, and SARS-CoV-2.
VZV is a double-stranded DNA virus of the Herpesviridae family (1). Primary VZV infection is responsible for chickenpox, a disease characterized by fever and a typical vesicular rash; it is commonly contracted in childhood, but it can also affect adults, both immunodeficient and healthy subjects (2). The reactivation of the virus, which remains in latent form inside sensory nerve ganglia, causes Herpes Zoster (HZ) (1).
Primary VZV infection in children can sometimes be associated with ocular complications: in 12–25% of varicella cases an acute anterior uveitis, usually mild, can develop, which causes discomfort, lid swelling, irritation, perilimbar injection, photophobia, and decreased visual acuity (3). Generally, this condition is self-limiting and does not cause long-term ocular damage. However, there are rare cases where chickenpox can determine serious forms of ocular involvement. Even in adults, primary varicella infection can become complicated with ocular manifestations, which (3) are usually recurrent granulomatous or non-granulomatous anterior uveitis and keratitis (4). In addition, cases have been reported of primary VZV infection becoming complicated with acute retinal necrosis (ARN) (4).
- Reactivation, causing Herpes Zoster, is a fairly common event (5, 6), but it is more typical in subjects over 50 years and in immunocompromised subjects (7–10) and with immunosenescence due to the aging (11). Herpes Zoster Ophthalmicus (HZO) is the involvement of ophthalmic branch of the fifth cranial nerve, and it represents 10–20% of HZ cases (5).
All ocular structures can be affected by the disease: the virus can affect the eyelids causing hyperemia, edema, skin rash, ptosis, decreased palpebral motility, or even lagophthalmos (the inability to fully close the eyelids) as a result of the paralysis of orbicular muscle (12–14).
At conjunctival level the virus can determine a follicular reaction, with the potential formation of membranes or pseudomembranes; moreover, vesicles can develop on bulbar or eyelid conjunctiva (12–15). It is possible a worsening of the clinical situation with superimposed infections, ulceration, scar development, and eventually symblepharon (a cicatricial fusion between the globe and the inner surface of the eyelid) or mechanical entropion (the inversion or turning inwards of the border of the eyelid against the eyeball, causing the eyelashes to rub against the ocular surface) (12).
The cornea is the ocular structure more frequently involved (about 65% of cases) (12, 15–17): HZO can produce corneal surface epithelial keratitis (12–14), pseudodendrites (10, 13, 14), anterior stromal infiltrates (12, 14), late corneal mucous plaques keratitis (MPK) (18, 19), disciform keratitis (12, 14), endothelitis with potential subsequent loss of endothelial cells (20), neurotrophic keratitis due to involvement of the sensory nerve with corneal hypoesthesia or anesthesia (10, 16, 21), or exposure keratitis, if associated with an eyelid defect (12). In the most severe forms, corneal ulceration and even perforation could appear, generally at perilimbar level (12, 15, 22). Following the acute phase, corneal scars are common and they can manifest as either a stromal haze or opaque areas, eventually associated with corneal thinning (12–15). Finally, in case of extensive corneal involvement, neovascularization can be observed (14).
Other manifestations of HZO are scleritis and episcleritis (13, 15, 23), anterior uveitis (13, 14, 16, 24), cataracts (10) and iris damage with sectoral atrophy (13, 14). As a complication of the uveal involvement there may be iris adhesion to the angle or lens structures, called synechiae (14): in fact it is not uncommon to detect a high intraocular pressure during HZO (10, 13, 15), potentially linked to trabeculitis (14), and secondary glaucoma (10, 13, 25).
Moreover, rare complication of HZO is optic neuritis, which can occur during the acute phase of the infection or as a post-herpetic complication; symptoms include reduced vision and central visual field defects, which are typically associated with edema and hyperemia of the optic papilla in the active phase, and with atrophy of the optic disc thereafter (16, 26–29). Although the actual cause is unknown, it is assumed that optic neuritis can be caused either by direct nerve infection by the virus through the cavernous sinus or by an immune-mediated reaction causing edema of the optic disc and inflammatory demyelination of the nerve (26).
In less frequent cases, HZO can be associated with paralysis of the cranial nerves, especially oculomotor nerve, abducent nerve and trochlear nerve (16, 30–32), and even with retinal detachment (14, 33). As already mentioned, VZV is also the most frequent cause of ARN, a severe occurrence characterized by 1 or more foci of retinal necrosis with defined edges and localized to the retinal periphery, which progress rapidly without antiviral therapy, generally circumferentially (13–16, 33); this pathological condition is a result of occlusive vasculopathy with arteriolar involvement, and is accompanied by reaction in the anterior and vitreous chamber (34, 35). The visual outcome is usually very poor (33), in about half of the cases often less than 20/200 (36), and can be followed by retinal detachment, chronic vitretitis, epiretinal membrane, macular ischemia, macular edema or optic neuropathy (37, 38).
Because of the potential ocular complications, HZO is reported to cause a loss of visual acuity in 6.6–10% of patients (13, 15, 39).
In many developed countries vaccination against primary VZV infection is recommended in children over 1 year of age, teenagers and adults without a previous history of chickenpox (13, 40); the vaccine name is Varivax (or Varilix, depending on producer and country), consisting of live-attenuated virus. The incidence of varicella, as well as varicella-related hospitalizations, has decreased significantly since implementation of the varicella vaccination program in 1995. Overall, varicella incidence declined an average of 97% from prevaccine years (from 1993–1995 to 2013–2014) based on data from four states that have been continuously reporting varicella to the National Notifiable Diseases Surveillance System (NNDSS) since before the varicella vaccination program (41).
In very rare cases, anterior uveitis or keratitis have been observed in the days following vaccination. However, in most of these cases, although the temporal correlation, it is impossible to define whether the ocular complications were due to the pre-existing latency of the wild-type virus or to the live attenuated virus injected (40, 42).
There are two vaccinations available for immunization against Herpes Zoster: Zostavax, a live attenuated vaccine available since 2006, and Shingrix, a recombinant subunit vaccine available since 2017.
Both Varivax and Zostavax contain the same live attenuated virus, however Zostavax contains a higher dose than Varivax. Several studies showed that this vaccine significantly reduces (more than 60%) the incidence of herpes zoster and post-herpetic neuralgia (43) and therefore it is assumed that it also reduces HZO rate (14, 43). In the USA it was initially recommended for the population over 60 years old, and later also for the population aged between 50 and 59 (13, 39, 43).
However, some studies revealed that some patients with history of HZO could develop ophthalmic, dermatological or disseminated recurrence following vaccination with Zostavax (13, 23, 44).
It was pointed out how Zostavax, in order to prevent latent VZV reactivation, increases the activity of cell-mediated immunity, which in some cases can determine a reaction against persistent viral DNA in the eye tissues, triggering keratitis (45–47), sometimes complicated by perforation risk (48), and anterior uveitis (47, 49, 50). In rarer, but more serious cases, ARN following vaccination with Zostavax was observed (51–53). Usually, these manifestations were reported to follow vaccine administration for about 2–4 weeks (45, 46, 48, 50, 54). Other studies identify in previous history of HZO a risk factor for a possible recurrence following vaccination (45, 46, 50). On the other hand, reassuringly, a study based on the Health-Claim Database (47) showed that there is not an increased risk of anterior segment complications in patients who received Zostavax compared to those who had a first diagnosis of HZ; it was also observed (40, 55–57) that the reactivation of the virus and HZ following vaccination with Zostavax is very rare. So, despite these potential risks, the vaccine is considered safe (54), complications are rare (58) and therefore a past history of HZO is not actually a contraindication to vaccination (5, 23, 46, 50, 59, 60). However, particular attention is recommended for these patients, with eye checks in the 4–6 weeks following vaccination (47).
Currently, Zostavax has largely been superseded by recombinant zoster vaccine Shingrix in many countries. Shingrix is a subunit vaccine that contains a VZV glycoprotein E antigen and the AS01B adjuvant system. Shingrix represents a novel, highly effective and well-tolerated vaccine option for reducing incidence of HZ (more than 90% reduction of risk of HZ) and postherpetic neuralgia in adults aged ≥ 50 years. It is not contraindicated in immunocompromised subjects, and it is preferred over a live attenuated HZ vaccine in immunocompetent individuals, according to the US and Canadian guidelines (61).
Post-licensure surveillance of Shingrix found a reporting rate for inflammatory eye diseases of 0.6/100,000 with limited reports related to uveitis (62). It is unknown whether any of these patients had pre-existing inflammatory ocular disease, and the possibility that these cases actually occurred from a lack of vaccine efficiency cannot be excluded.
Results from two large randomized placebo-controlled phase 3 trials of the Shingrix found potential immune-mediated diseases occurred at a similar rate between those receiving vaccine and controls at all-time points (63). Similarly, subjects with pre-existing possible immune-mediated diseases did not demonstrate an increased risk for a new possible immune mediated process or exacerbation of their prior disease after vaccination compared with controls. Ocular autoimmune diseases were a pre-defined reportable adverse event in both trials; uveitis was only recorded in 1 of 14,645 subjects receiving Shingrix.
A single case of ARN and disseminated zoster after receiving the recombinant subunit vaccine has also been described in a 65-year-old woman with past medical history of multiple myeloma (64). Though post-vaccination VZV infection or reactivation appears to be rare, clinicians should be aware of this potential complication to the recombinant subunit vaccine. Nevertheless, as Shingrix does not contain infectious virus, this most likely represented a failure of efficacy in boosting immunity to VZV.
Uveitis recurrence is an infrequent but serious potential ocular side effect of recombinant zoster vaccination. Three cases of uveitis reactivation following Shingrix have been reported: one patient developed a reactivation of a previously controlled multifocal choroiditis within 1 week of receiving vaccine, and two patients with a previously controlled anterior uveitis developed new anterior segment inflammation (65).
The development of HZO following Shingrix is extremely rare, with only two reported cases of HZ keratitis reactivation shortly following the vaccine: an 89-year-old man (66) and a 75-year-old woman (67) with a history of a well-controlled HZO keratitis had developed a recurrent keratitis a few weeks after their first dose of Shingrix. Fortunately, they made a complete recovery following treatment.
The mechanism behind the activation of HZO following the subunit vaccine is unknown. For live-attenuated vaccines activation could possibly come from direct inoculation of the attenuated, but still active, virus strain. For inactivated vaccines such as Shingrix, the mechanism is more puzzling; one possible explanation is a general upregulated immune response to the vaccine, causing ocular inflammation. Another hypothesis is that adjuvants contained in the vaccines could create an autoinflammatory response. This phenomena is known as Shoenfeld syndrome (68, 69). Shingrix vaccine does not contain aluminum salts (the most common cause of Shoenfelt syndrome), even though it contains a lipid formulation which could represent a possible responsible factor (67).
In conclusion, despite the possibility of ocular effects following Shingrix, they are extremely rare and vaccination is recommended (61, 62). Rather, this report highlights the importance of ensuring primary care providers are aware of a patient’s history of immune-mediated eye disease.
Measles is an important cause of child morbidity and mortality worldwide; the World Health Organisation (WHO) estimated that approximately 114,900 people, mostly children under 5 years of age, died of measles and resulting sequelae in 2014. The virus causing measles is MeV, an RNA virus belonging to Paramyxoviridae family; it is an extremely contagious virus, which is transmitted by respiratory route (70). Typical prodromal symptoms of measles are fever, generalized malaise, cough, coryza, and conjunctivitis; in this phase the diagnosis can be suggested by the presence of the pathognomonic Koplik spots on buccal mucosa. In following phases the classic maculopapular rash appears, which initially involves the face and then extends to trunk and extremities (71–73). In the most cases, measles is a self-limiting disease and once resolved leaves a permanent immunity. However, exceptionally it can be complicated by severe neurological sequelae such as acute disseminated encephalomyelitis (ADEM), measles inclusion body encephalitis (MIBE), or subacute sclerosing panencephalitis (SSPE) (74).
Moreover, measles can be accompanied by ocular complications: in its prodromal phase, MeV can cause conjunctivitis, characterized by bulbar and tarsal hyperemia with papillary reaction and eventual mucous secretions; corneal involvement, from mild forms such as punctate superficial keratitis to more severe ones such as subconjunctival bleeding, corneal ulceration, corneal perforation, leukoma and, in very rare cases, chorioretinitis and central vein occlusions (75). More rarely, it has been reported optic neuritis, optic atrophy, retinal vasculitis and macular and chorioretinal alterations, such as macular epithelial pigment anomalies, macular edema, macular hemorrhage, internal limiting membrane contracture and serous macular detachment (76–81).
Notably, ocular complications occur in about half of patients affected by SSPE (76, 77) and they may precede, follow or appear simultaneously with other neurological symptoms and signs (78–81). They are often serious and involve the posterior segment, the optic nerve and more generally all CNS structures associated with vision function: symptoms include gradual visual acuity reduction, episodes of transient blindness, homonymous visual field defects, abnormal spatial perception, visual hallucinations, nystagmus, cortical blindness (76–82). The most typical ocular manifestation in the course of SSPE is the appearance of white, focal or multifocal retinal lesions, which rapidly evolve in areas of atrophy of the retinal pigment epithelium and gliotic scars (76–78).
Measles is best prevented through vaccination: vaccine consists of an attenuated live form of the virus, proved as safe and effective (83). The delivery of the two doses of vaccine needed to achieve a > 90% immunity is accomplished by routine immunization of infants at 9–15 months followed by a second dose delivered before school entry or by periodical mass vaccination campaigns (84). There are only a few case reports in literature about possible appearance of ocular complications related to the administration of this vaccine (85, 86) involving oculomotor palsy and acute bilateral photoreceptor degeneration. Since these are single and sporadic cases, it is not possible to establish a real correlation between the appearance of the ocular manifestation and the administration of the vaccine (86).
Influenza type A, B, and C viruses (IAVs, IBVs, and ICVs) are RNA viruses belonging to the Orthomyxoviridae family (87). Among the three, influenza A viruses are clinically the most important, being responsible for severe epidemics in humans and domestic animals. Aerosol droplets transmit the virus, which causes a respiratory disease that can lead to severe pneumonia and even death (88). The main characteristic of IVs is the high variability caused by antigenic shift, which is the result of recombination with other strains. It allows a lack of recognition of the new variant by the immune system. So, IAVs arising from different host species can combine, producing pandemic strains that are antigenically novel but otherwise well adapted to humans (87).
Several case reports shown a correlation between some types of IVs, especially IAVs, and ocular manifestations. Eye complications mainly involve the posterior segment and the uvea. Different kinds of retinitis was correlated with IAVs infection, characterized by submacular hemorrhage without neovascularization (89), macular edema with exudates (90), perifoveal edema with star-like pattern (91) or by alterations visible by fluorangiography, as hyperfluorescent spots associated with multiple dark circular lesions at the posterior pole (92). In some cases (90) angiopathy was observed, sometimes associated with frosted branch angiitis-like fundus (93). In addition, it is occasionally possible to observe generalized uveal involvement, known as uveal effusion syndrome, characterized by conjunctival congestion, tenderness, pain exacerbated by eye movement, choroidal, and subretinal exudation (94). In the majority of cases symptoms resolves completely over time (90, 92–94) even though, in exceptionally severe cases, they can become permanent: an example is reported by Breker et al. (95), describing the case of a 13 years old girl affected by IAV H1N1, who developed encephalitis associated with severe permanent visual impairment, caused by a confluent ischemic retinopathy and infarction of the lateral geniculate body.
The high mutation rate combined with a high replication speed and possible antigenic shift allow the virus to rapidly change its structural features and so to escape the immune system or become resistant to drugs: this causes annual epidemics (also Influenza type B arising) and demands for compositions of new vaccine (88). The influenza vaccine can be an inactivated virus vaccine or a split-virus vaccine; usually adults receive a trivalent whole virus vaccine, containing three different viral strains anticipated to be prevalent in the upcoming season (96): subjects receiving the influenza vaccine have a reported lower risk of influenza compared with those who do not receive a vaccination over the course of a single influenza season (97).
Some studies have reported cases of optic neuritis following IVs vaccination, with consequent visual field deficits, in some cases recovering after steroid therapy (98–101), but becoming permanent in other ones (96, 102, 103). It has been hypothesized that the involvement of the optic nerve could be triggered by the similarity between some IVs antigens and proteins located in the CNS: the immune response caused by the viral antigen presence would stimulate an inflammatory reaction in the CNS and against the optic nerve, with consequent demyelination (96, 101). Other ocular manifestations following influenza vaccination was observed affecting the retina: these include multiple evanescent withe dot syndrome (MEWDS) (104) and acute posterior multifocal placoid pigment epitheliopathy (APMPPE) (105, 106): also for these manifestations it was assumed that a molecular mimicry between the viral antigens contained in the vaccine and the retinal pigment epithelium (the specialized epithelium lying in the interface between the neural retina and the underlying choroid) would trigger an inflammatory reaction (105). Finally, in rare cases corneal transplant rejection was observed following vaccination, both in case of perforating keratoplasty (107, 108) and in case of deep anterior lamellar keratoplasty (109): however, in these cases the correlation between vaccination and the rejection was vitiated by other factors, which did not make it possible to establish a certain cause-effect relationship.
HBV is a DNA virus (110) representing the most common cause of liver cancer in the world (111). HBV mainly infects hepatocytes and during the acute phase causes nausea, vomiting, diarrhea, abdominal discomfort, decreased appetite, fatigue, fever, myalgia, dark urine and jaundice. Interestingly, HBV can also induce ocular manifestations as retinal vasculitis (112), optic neuritis (113–115), paralysis of the third cranial nerve with pupil-sparing (116) and uveitis (117–119). All these manifestations seem to be caused in part by the indirect action of the virus, due to the accumulation of inflammatory debris in different eye structures, and in part by the immune reaction triggered to eradicate the infection (112, 120).
HBV vaccination significantly reduced the prevalence of the virus worldwide (121). The vaccine is produced through recombinant DNA technique, allowing the administration of the purified HBsAg antigen, usually in three doses generally starting from the third month of life (122).
Reported ocular complications occurring after the administration of the vaccine included uveitis (112–114), which is the most common manifestation and typically appears 3 days or more following the first dose of the vaccine but rarely occurs again after the second and third dose (123, 124). The posterior segment and the optic nerve resulted also potentially involved, with MEWDS (125), APMPPE (126, 127), central retinal vein occlusion (113, 128), papilledema (129) and optic neuritis (113, 130, 131).
COronaVIrus Disease 19 (COVID-19), due to SARS-CoV-2, was first reported in Wuhan, China in December 2019 and has rapidly become pandemic all over the world, with an exponential increase in the number of cases. The most common symptoms are fever, cough and fatigue, and sometimes diarrhea. SARS-CoV-2 can be transmitted from person to person mainly through respiratory droplets or close contact (132, 133).
Being the ocular surface exposed to the outside environment, it can be a potential gateway for virus to invade the body. In addition, ACE 2 is a cellular receptor for SARS-CoV2 (134), that can also been detected in human retina, choroid, cornea, and conjunctiva (135–137). For these reasons, the eye is one of the possible targets of the virus, causing a wide variety of ocular diseases. A retrospective study of three hospitals in Wuhan during the very first phase of the pandemic (January 16-February 19, 2020) reported that 1.4% of patients had visual impairment (138). Wu et al. studied the prevalence of ocular manifestations in patients with COVID-19 and reported that chemosis, epiphora, and conjunctival hyperemia were present in one-third of the patients, most commonly in patients with a severe systemic involvement (139).
Moreover, the virus can be present in tear and conjunctival secretions, suggesting that SARS-CoV-2 could potentially be transmitted also through the eyes (140).
Conjunctivitis and keratoconjunctivitis can be the first symptom in infected patients (141). Dinkin et al. also reported two cases of ophthalmoparesis with abducens nerve palsies that developed within a few days of mild respiratory symptoms (142). The abducens nerve controls the lateral rectus muscle, which abducts the eye. Abducens nerve palsy causes an esotropia due to the unopposed action of the antagonistic medial rectus muscle, so the affected eye turns medially and is unable to abduct properly. Chen et al. suggested that ocular manifestations secondary to COVID-19 can also develop in the middle phase of the disease. They reported a young COVID-19-positive patient who developed a bilateral acute follicular conjunctivitis 13 days after illness onset. Viral RNA was detected in conjunctival swabs and RT-PCR was negative at resolution of symptoms (143). Another patient with severe COVID-19 developed a pseudomembranous and hemorrhagic conjunctivitis 19 days after the beginning of symptoms (144).
There is a report about acute corneal endothelial graft rejection with coinciding COVID-19 infection (145). Regarding corneal graft rejection, any systemic immune dysregulation may alterate corneal ocular immune privilege and increase the patient’s susceptibility for rejection (146). Cells of the innate immune system can invade the cornea and result in the up regulation of cytokines and other pro-inflammatory molecules, including tumor necrosis factor–α (TNF–α), and interleukin-6 (IL-6), normally higher during COVID-19 inflammation (147), which can result in rejection of the corneal transplants.
Furthermore, based on murine models of other CoVs, viral-induced retinitis and optic neuritis secondary to autoantibody production against neuroretina should also be possible, so positive patients should be monitored for signs of neuroretinal degeneration in the long term. Hyper-reflective lesions at the level of the inner plexiform and ganglion cell layers have been also described in infected patients (148–150).
The European Medicines Agency (EMA) and the US Food and Drug Administration have approved emergency use authorization for several COVID-19 vaccines. BNT162b2 (Pfizer/BioNTech, Mainz, Germany) (151) and mRNA-1273 (Moderna, Cambridge, MA, USA) (152) belong to the category of lipid nanoparticle (LNP)-formulated mRNA COVID-19 vaccines, while ChAdO × 1 (University of Oxford/AstraZeneca, Oxford, UK) (153) and Ad26.COV2.S (Johnson & Johnson/Janssen, New Brunswick, NJ, USA) (154) belong to the category of adenovirus vector COVID-19 vaccines.
Among the adverse events after receiving COVID-19 vaccines, ocular manifestations have been reported, occurring up to 42 days after vaccination and affecting eyelids, cornea and ocular surface, retina, uvea, nerve, and vessels. Vaccine-induced immunologic responses may be responsible (155, 156).
The Pfizer-BioNTech (157) and Moderna (158) vaccine trials suggest an imbalance in the incidence of Facial Nerve palsy following vaccination (1:5,272) compared with the placebo arm of each trial (1:36,938). Nevertheless, based on the odds ratio (OR) from different studies (159), and after adjustment for pre-existing immune- or inflammatory-related disorders, diabetes, and a previous episode of peripheral nerve palsy, it is highly unlikely that Bell’s palsy is associated with COVID-19 vaccination. Reyes-Capo et al. reported that a patient was diagnosed with right abducens nerve palsy (160), while Helmchen et al. reported female with a history of relapsing-remittent multiple sclerosis (MS) who was diagnosed with Optic neuritis with AQP4-antibody negative neuromyelitis optica spectrum disorders-like syndrome 2 weeks after the first dose of the ChAdO × 1 COVID-19 vaccine (161). Maleki et al. reported an old female who had a sudden bilateral loss of vision 2 days after the second dose of BNT162b2 COVID-19 vaccine, and a bilateral Arteritic anterior ischemic optic neuropathy (AAION) was diagnosed (162).
Literature reported six patients with eyelid manifestations after COVID-19 vaccination: eyelid swelling, eyelid purpuric lesions, Herpes Zoster Ophthalmicus (HZO) (163–165). HZO is a result of VZV reactivation, so vaccine may have induced VZV to reactivate.
Six patients developed corneal manifestations after vaccination. The mean age of these patients was 68.5 (range 56–83) years old. The mean duration between COVID-19 vaccination and onset of ocular symptoms was 14.0 (range 7–21) days. The reported ocular manifestations were corneal graft rejection after penetrating keratoplasty (PKP) (166) and corneal graft rejection after Descemet membrane endothelial keratoplasty (DMEK) (167, 168). The activation of the immune system post-vaccination could be a possible involved mechanisms related to vaccine-related corneal graft rejection (146, 147).
Also uvea is reported to be among the ocular structures involved in post-vaccination manifestations, with many new onset uveitis (169–172). Renisi et al. reported an acute anterior uveitis affecting a 23-year-old male after receiving the BNT162b2 vaccine (172). Mudie et al. reported a panuveitis affecting a 43-year-old female who developed decreased vision 3 days after the second dose of the BNT162b2 COVID-19 vaccine, and she was also diagnosed with asymptomatic COVID-19 shortly after the onset of ocular symptoms (171). Goyal et al. reported a 34-year-old man had vision loss 1 week after receiving the second dose of the COVID-19 vaccine, and a bilateral multifocal choroiditis was diagnosed (170). Also one case of acute zonal occult outer retinopathy (AZOOR) (162) and one patient with a reactivation of Vogt-Koyanagi-Harada (VKH) Disease were described: a woman with a pre-existing diagnosis of VKH well controlled for the past 6 years, who manifested a severe reactivation of VKH 6 weeks after receiving the second dose of the BNT162b2 vaccine (173). Furer et al. focused on the immunogenicity of the BNT162b2 vaccine in 686 patients with autoimmune inflammatory rheumatic diseases, reporting one case of uveitis several weeks after the first dose of the vaccine and two cases after the second dose (174).
Similarly retina and its vascularization could be a possible target in post-vaccination ocular manifestations. Four studies (175–178) reported cases of Acute Macular Neuroretinopathy (AMN), a rare retinal disease causing loss of vision, in which a microvascular abnormality in the deep capillary plexus of the retina is hypothesized (179). All patients were female on oral contraceptive pills (OCP), identified as a risk factor for AMN (180), and received the ChAdOx1 nCoV-19 vaccine. Ocular symptoms occurred 2 days after the first dose. The rarity of disease and the temporal association between the vaccination and the onset of manifestations should be taken into consideration, supposing an additional role in AMN pathogenesis of the thrombogenic role of COVID-19 vaccination.
Central Serous Chorioretinopathy has been reported among the possible retinal manifestations following vaccine administration. Fowler et al. reported a 33-year-old male who developed blurred vision and metamorphopsia in his right eye 69 h after receiving the BNT162b2 COVID-19 vaccine (181).
In literature, we found one case of bilateral retinal detachment. Subramony et al. reported a 22-year-old female with myopia who developed vision loss in her right eye 15 days after the second dose of the mRNA-1273 COVID-19 vaccine. Fundoscopy revealed bilateral retinal detachment without any trauma (182).
In addition, 8 cases of Vascular Thrombosis after vaccination are reported, with a mean age of the patients of 42.9 (range 18–60) years old. The mean duration between vaccine administration and onset of ocular manifestations was 8.1 (range 2–13) days. Regarding post-vaccination thrombosis, rare cases of post vaccination immune thrombotic thrombocytopenia and cerebral venous sinus thrombosis (CVST) after administration of the adenovirus vector vaccines ChAdOx1 nCoV-19 and Ad26.COV2 have been well described (183–189). Anatomically, CVST post-COVID-19 vaccination has been reported to occur in virtually all the dural venous sinuses, and a majority of patients are females.
In conclusion, based on the several reported ocular manifestations after COVID-19 vaccination, physicians should be aware of the possible associations between COVID-19 vaccines and ocular symptoms in order to increase early diagnosis and treatment. Considering that COVID-19 vaccines are very recent and that most of the literature includes case reports and series, there may be evolving data on adverse ocular effects of vaccination, and at the moment no certain causality should be established. On the basis that several vaccines (as the ones described in this review) can induce well demonstrated ocular adverse effects, realistically also COVID-19 vaccines can determine ocular complications, both in case of mRNA vaccines and adenoviral vector vaccines. Vaccine-induced immunologic responses could be responsible for the pathogenesis of the ocular symptoms after COVID-19 vaccination (156).
Nevertheless, the overall benefits of the COVID-19 vaccine in preventing COVID-19 are well established, and the incidence rate of ocular symptoms after receiving the vaccine, considering the massive rollout campaign across the world, is considerably lower than the prevalence rate of ocular involvement in infected patients, so people are encouraged to get vaccinated since the benefits outweigh the risks (155). To date, there is no evidence to suggest that individuals should avoid getting vaccinated for ophthalmic-related reasons (156).
The reported literature shows how ocular involvement may occur as a result of both infection and vaccination (Table 1). Vaccine-associated adverse events such as disease reactivation are uncommon and difficult to prove. In most cases it is not clear if the ocular manifestations (usually described as case reports of few subjects) following vaccination are due to the vaccine itself, and so if they are directly associated with the vaccination, or if they are simply coincidental. It is not possible to exclude that some adverse events would have occurred also in the unvaccinated populations. However, it is clear how the risk of ocular complications following vaccine administration is lower than the potential rate of complications due to wild virus infection.
A future effort should be the development of effective vaccines with less adverse effects, stimulating a protective antibody response and a T cell response without risk of cross-reaction, using as an example purified viral antigens instead of the whole pathogen; however, the main problem in this approach is that the stimulated immune response could not be able to guarantee a lasting immunity comparable to that produced by live-attenuated virus. The key, as already obtained with other pathogens, could be the development of carriers to enhance the immune response triggered by the antigen, without the need to expose the patient to the whole pathogen.
In addition, it would be helpful to develop vaccine to prevent viral diseases with very frequent ocular involvement, such as Herpes Simplex Virus (HSV) infection. HSV is responsible for several distinct medical disorders, such as orolabial herpes, HSV folliculitis, herpes gladiatorum, herpetic whitlow, herpes encephalitis, and eczema herpeticum. Ocular involvement can present as a primary infection or recurrence from latent disease. Almost every ocular structure can be involved, with blepharitis, conjunctivitis, epithelial or stromal keratitis, endotheliitis, iritis, trabeculitis, and retinitis. Ocular HSV infection is usually due to HSV-1, which establishes latency in the trigeminal ganglion, but HSV-2 can also be a cause of HSV keratitis (190). Ocular complications cause significant visual burden, being the most common cause of corneal blindness in developed countries. They also impair quality of life, with need for long-term maintenance medications for recurrent or chronic cases as a basis for effective management (191). Despite this large public health burden, there is still active debate about the optimal management of ocular HSV (192), and the HSV disease remains challenging to prevent, also because the immune-mediated response to HSV plays an important role in physiopathology of herpetic keratitis. Development of an HSV vaccine represents a promising preventing strategy. One exciting area focuses on a corneal dendritic cell based DNA vaccine that has shown encouraging results in murine models (193, 194). In a recent study of mice previously exposed to a live-attenuated HSV candidate vaccine, when challenged with HSV-1, they did not develop any corneal pathology and had complete preservation of visual acuity, with no additional ocular effects (195). If successfully translated into humans, an HSV vaccine would essentially change the management of ocular HSV infection.
In conclusion, the possible, although rare, risk of ocular involvement should not therefore discourage vaccination, which should be promoted and carried out whenever it is deemed useful or necessary. In fact the benefits of vaccines for the patient and for the population far outweigh the risks of the infections, such as possible systemic manifestations and even severe ocular complications.
All authors gave their substantial contribution to conception and design of the manuscript and to the acquisition and interpretation of data and materials, contribution in drafting the manuscript and in its critical revision for important intellectual content, approved the manuscript in its present form for publication, and agreed to be accountable for all aspects of the work in ensuring that questions related to the accuracy or integrity of any part of the work are appropriately investigated and resolved.
The authors declare that the research was conducted in the absence of any commercial or financial relationships that could be construed as a potential conflict of interest.
All claims expressed in this article are solely those of the authors and do not necessarily represent those of their affiliated organizations, or those of the publisher, the editors and the reviewers. Any product that may be evaluated in this article, or claim that may be made by its manufacturer, is not guaranteed or endorsed by the publisher.
2. Matsuo T, Koyama M, Matsuo N. Acute retinal necrosis as a novel complication of chickenpox in adults. Br J Ophthalmol. (1990) 74:443–4.
3. Johnston NR. Red eye in chickenpox: varicella-related acute anterior uveitis in a child. BMJ Case Rep. (2010) 2010:bcr0120102678. doi: 10.1136/bcr.01.2010.2678
4. Gargouri S, Khochtali S, Zina S, Khairallah M, Zone-Abid I, Kaibi I, et al. Ocular involvement associate with varicella in adults. J Ophthalmic Inflamm Infect. (2016) 6:47. doi: 10.1186/s12348-016-0117-9
5. Liesegang TJ. Herpes zoster ophtamicus natural history, risk factors, clinical presentation, and morbidity. Ophthalmology. (2008) 115:S3–12.
6. Harpaz R, Ortega-Sanchez IR, Seward JF, Advisory Committee on Immunization Practices [ACIP] Centers for Disease Control and Prevention [CDC]. Prevention of Herpes Zoster: reccomendations of the advisory committee on immunization practices (ACIP). MMWR Recomm Rep. (2008) 57: CE32–4.
7. Donahue JG, Choo PW, Manson JE, Platt R. The incidence of herpes zoster. Arch Intern Med. (1995) 155:1605–9.
8. Yawn BP, Saddier P, Wollan PC, St Sauver JL, Kurland MJ, Sy LS. A popultion-based study of the incidence and complication rates of herpes zoster before zoster vaccine introduction. Mayo Clinic Proc. (2007) 82:1341–9. doi: 10.4065/82.11.1341
9. Weinberg J. Herpes zoster: epidemiology, natural history, and common complications. J Am Acas Dermatol. (2007) 57:S130–5.
10. Ghaznawi N, Virdi A, Dayan A, Hammersmith KM, Rapuano CJ, Laibson PR, et al. Herpes zoster ophthalmicus: comparison of disease in patients 60 years and older versus younger than 60 years. Ophthalmology. (2011) 118:2242–50. doi: 10.1016/j.ophtha.2011.04.002
11. Gilden D. What does epidemiology tell us about the risk factors for HZ? Lancet Neurol. (2009) 8:705–6.
12. Kaufman SC. Anterior segment complication of herpes zoster opthalmicus. Ophthalmology. (2008) 115:S24–32. doi: 10.1016/j.ophtha.2007.10.010
13. Tugal-Tutkun I, Cimino L, Akova YA. Review for disease of the year: varicella zoster virus-induced anterior uveitis. Ocul Immunol Inflamm. (2018) 26:171–7. doi: 10.1080/09273948.2017.1383447
14. Vrcek I, Choudhury E, Durairaj V. Herpes zoster ophthalmicus: a review for the internist. Am J Med. (2017) 130:21–6. doi: 10.1016/j.amjmed.2016.08.039
15. Yawn BP, Wollan PC, St Sauver JL, Butterfield LC. Herpes zoster eye complications: rates and trends. Mayo Clin Proc. (2013) 88:562–70. doi: 10.1016/j.mayocp.2013.03.014
16. Kahloun R, Attia S, Jelliti B, Attia AZ, Khochtali S, Yahia SB, et al. Ocular involvement and visual outcome of herpes zoster ophthalmicus: review of 45 patient from Tunisia, North Africa. J Ophthalmic Inflamm Infect. (2014) 4:25. doi: 10.1186/s12348-014-0025-9
17. Puti LR, Shrestha GB, Shah DN, Chaudhary M, Thakur A. Ocular manifestation in herpes zoster ophthalmicus. Nepal J Ophthalmol. (2011) 3:165–71.
19. Piebenga LW, Laibson PR. Dendritic lesion in herpes zoster ophthalmicus. Arch Ophthalmol. (1973) 90:268–70.
20. Rejo A, Antti V, Jukka M. Endothelial cell loss in herpes zoster keratouveitis. Br J Ophthalmol. (1983) 67:751–4. doi: 10.1136/bjo.67.11.751
21. Cobo M, Foulks GN, Liesegang T, Lass J, Sutphin J, Wilhelmus K, et al. Observations on the natural history of herpes zoster ophthalmicus. Curr Eye Res. (1987) 6:195–9. doi: 10.3109/02713688709020090
22. Mondino BJ, Brown SI, Mondzelewski JP. Peripheral corneal ulcers with herpes zoster ophthalmicus. Am J Ophthalmol. (1978) 86:611–4.
23. Tran KD, Falcone MM, Choi DS, Goldhardt R, Karp CL, Davis JL, et al. Epidemiology od herpes zoster ophthalmicus: recurrence and chronicity. Ophthalmology. (2016) 123:1469–75. doi: 10.1016/j.ophtha.2016.03.005
24. Kido S, Sugita S, Horie S, Miyanaga M, Miyata K, Shimizu N, et al. Association of varicella zoster virus load in the aqueous humor with clinical manifestation of anterior uveitis in herpes zoster ophthalmicus and zoster sine herpete. Br J Ophthalmol. (2008) 92:505–8. doi: 10.1136/bjo.2007.125773
25. Thean JH, Hall AJ, Stanwell RJ. Uveitis in herpes zoster ophthalmicus. Clin Exp Ophthalmol. (2001) 29:406–10.
26. de Mello Vitor B, Foureaux EC, Porto FB. Herpes zoster optic neuritis. Int Ophthalmol. (2011) 31:233–6.
28. Lexa FJ, Galetta SL, Yousem DM, Faber M, Oberholtzer JC, Atlas SW. Herpes ophthalmicus with orbital pseudotumor complicated by optic nerve infarction and cerebral granulomatous angiitis:ME-pathologic correlation. Am J Neuroradiol. (1993) 14:185–90.
29. Gunduz K, Ozdemir O. bilateral retrobulbar neuritis following unilateral herpes zoster ophthalmicus. Ophthalmologica. (1994) 208:61–4. doi: 10.1159/000310454
30. Park KC, Yoon SS, Yoon JE, Rhee HY. A case of herpes zoster ophthalmicus with isolated trochlear nerve involvement. J Clin Neurol. (2011) 7:47–9.
31. Chhabra MS, Golnik KC. Recovery of ocular motor cranial nerve palsy after gerpes zoster ophthalmicus. J Neuroophthalmol. (2014) 34:20–2. doi: 10.1097/WNO.0b013e3182a59c69
32. Tsuda H, Ito T, Yoshioka M, Ishihara N, Sekine Y. Isolated trochlear nerve palsy in herpes zoster ophthalmicus. Intern Med. (2007) 46:535–6.
33. Schoenberger SD, Kim SJ, Thorne JE, Mruthyunjaya P, Yeh S, Bakri SJ, et al. Diagnosis and treatment of acute retinal necrosis: a report by the American academy of ophthalmology. Ophthalmology. (2017) 124:382–92. doi: 10.1016/j.ophtha.2016.11.007
34. Muthiah MN, Michaelides M, Child CS, Mithcell SM. Acute retinal necrosis: a nation population-based study to assess the incidence, methods of diagnonis, tretament strategies and outcomes in the UK. Br J Ophthalmol. (2007) 91:1452–5. doi: 10.1136/bjo.2007.114884
35. Lau CH, Missotten T, Salzmann J, Lightman SL. Acute retinal necrosis features, management and outocomes. Ophthalmology. (2007) 114:756–62. doi: 10.1016/j.ophtha.2006.08.037
36. Cochrane TF, Silvestri G, McDowell C, Foot B, McAvoy CE. Acute retinal necrosis in the United Kingdom: results of a prospective surveillance study. Eye (London). (2012) 26:370–7. doi: 10.1038/eye.2011.338
37. Hillenkamp J, Nolle B, Bruns C, Rautenberg P, Fickenscher H, Roider J. Acute retinal necrosis: clinical features, early vitrectomy, and outocomes. Ophthalmology. (2009) 116:1971–5. doi: 10.1016/j.ophtha.2009.03.029
38. Wong RW, Jumper JM, McDonald HR, Johnson RN, Fu A, Lujan BJ, et al. Emerging concepts in the management of acute retinal necrosis. Br J Ophthalmol. (2013) 97:545–52.
39. Szeto SKH, Chan TCY, Wong RLM, Ng ALK, Li EYM, Jhanji V. Prevalence of ocular manifestations and visual outcomes in patients with herpes zoster ophthalmicus. Cornea. (2017) 36:338–42. doi: 10.1097/ICO.0000000000001046
40. Centers for Disease Control and Prevention. Prevention of varicella: reccomendations of tha advisory committee on immunization practices (ACIP). MMWR Morb Mort Wkly Rep. (1996) 45:1–36.
41. Lopez A, Zhang J, Marin M. Epidemiology of varicella during the 2-dose varicella vaccination program – United States, 2005-2014. MMWR Morb Mortal Wkly Rep. (2016) 65:902–5. doi: 10.15585/mmwr.mm6534a4
42. Krall P, Kubal A. Herpes zoster stromal keratitis after varicella vaccine booster in a pediatric patient. Cornea. (2014) 33:988–9. doi: 10.1097/ICO.0000000000000199
43. Hales CM, Harpaz R, Ortega-Sanchez I, Bialek SR, Centers for Disease Control and Prevention [CDC]. Update on recommendations for use of herpes zoster vaccine. MMWR Morb Mortal Wkly Rep. (2014) 63:729–31.
44. Galea SA, Sweet A, Beninger P, Steinberg SP, Larussa PS, Gershon AA, et al. The safety profile of varicella vaccine: a 10-years review. J Infect Dis. (2008) 197:S165–9.
45. Hwang CW, Steigleman WA, Saucedo-Sanchez E, Tuli SS. Reactivation of herpes zoster keratitis in an adult after varicella zoster vaccination. Cornea. (2013) 32:508–9. doi: 10.1097/ICO.0b013e318277acae
46. Khalifa YM, Jacoby RM, Margolis TP. Exacerbation of zoster interstitial keratitis after zoster vaccination in an adult. Arch Ophthalmol. (2010) 128:1079–80. doi: 10.1001/archophthalmol.2010.142
47. Liu RT, Yeung SN, Carleton B, Etminan M. Risk of anterior segment complications associated with the live herpes zoster vaccine: evidence from health-claim database. Cornea. (2018) 37:952–6. doi: 10.1097/ICO.0000000000001595
48. Jastrzebski A, Brownstein S, Ziai S, Saleh S, Lam K, Jackson WB. Reactivation of herpes zoster keratiris with corneal perforation after zoster vaccination. Cornea. (2017) 36:740–2. doi: 10.1097/ICO.0000000000001203
49. Murray AV, Reisinger KS, Kerzner B, Stek JE, Sausser TA, Xu J, et al. Safety and tolerability of zoster vaccine in adults >60 years old. Hum Vaccin. (2011) 7:1130–6.
50. Sham CW, Levinson RD. Uveitis exaceration after varicella-zoster vaccination in an adult. Arch Ophthalmol. (2012) 130:793–4. doi: 10.1001/archophthalmol.2011.1881
51. Heath G, Depledge DP, Brown JR, Hale AD, Tutil H, Williams E, et al. Acute retinl necrosis caused by the zoster vaccine virus. Clin Infect Dis. (2017) 65:2122–5. doi: 10.1093/cid/cix683
52. Charkoudian LD, Kaiser GM, Steinmetz RL, Srivastava SK. Acute retinal necrosis after herpes zoster vaccination. Arch Ophthalmol. (2011) 129:1495–7. doi: 10.1001/archophthalmol.2011.320
53. Ali A, Kirschenbaum MD, Sharma S, Wandel TL. Acute retinal necrosis and controlateral cutaneous eruption after the shingles vaccine. Retin Cases Brief Rep. (2018) 15:43–4. doi: 10.1097/ICB.0000000000000729
54. Willis ED, Woodward M, Brown E, Popmihajlov Z, Saddier P, Annunziato PW, et al. Herpes zoster vaccine live: a 10 years review of post-marketing safety experience. Vaccine. (2017) 35:7231–9. doi: 10.1016/j.vaccine.2017.11.013
55. Mills R, Tyring SK, Levin MJ, Parrino J, Li X, Coll KE, et al. Safety, tolerability and immunogenicity of zoster vaccine in subjects with a history of herpes zoster. Vaccine. (2010) 28:4204–9. doi: 10.1016/j.vaccine.2010.04.003
56. Tseng HF, Chi M, Smith N, Marcy SM, Sy LS, Jacobsen SJ. Herpes zoster vaccine and the incidence of recurrent herpes zoster in immunocompetent eldery population. J Infect Dis. (2012) 206:190–6.
57. Tseng HF, Schmid DS, Harpaz R, LaRussa P, Jensen NJ, Rivailler P, et al. Herpes zoster caused by vaccine-strain varicella zoster virus in an immunocompetent recipient of zoster vaccine. Clin Infect Dis. (2014) 58:1125–8. doi: 10.1093/cid/ciu058
58. Simberkoff MS, Arbeit RD, Johnson GR, Oxman MN, Boardman KD, Williams HM, et al. Safety of herpes zoster vaccine in the shingles prevention study: a randomized trial. Ann Inter Med. (2010) 152:545–54.
59. Cheetham TC, Marcy SM, Tseng HF, Sy LS, Liu IL, Bixler F, et al. Risk of herpes zoster and disseminated varicella zoster in patients taking immunosuppressant drugs at the time of zoster vaccination. Mayo Clin Proc. (2015) 90:865–73. doi: 10.1016/j.mayocp.2015.04.021
60. Tseng HF, Lewin B, Hales CM, Sy LS, Harpaz R, Bialek S, et al. Zoster vaccine and risk of postherpetic neuralgia in pationts who developed herpes zoster despite having received the zoster vaccine. J Infect Dis. (2015) 212:1222–31. doi: 10.1093/infdis/jiv244
61. Syed YY. Recombinant zoster vaccine (Shingrix §): a review in herpes zoster. Drugs Aging. (2018) 35:1031–40. doi: 10.1007/s40266-018-0603-x
62. Tavares-Da-Silva F, Co MM, Dessart C, Hervé C, Lopez-Fauqued M, Mahaux O, et al. Review of the initial post-marketing safety surveillance for the recombinant zoster vaccine. Vaccine. (2020) 38:3489–500.
63. López-Fauqued M, Campora L, Delannois F, El Idrissi M, Oostvogels L, De Looze FJ, et al. Safety profile of the adjuvanted recombinant zoster vaccine: pooled analysis of two large randomised phase 3 trials. Vaccine. (2019) 37:2482–93. doi: 10.1016/j.vaccine.2019.03.043
64. Chen RI, Deaner JD, Srivastava SK, Lowder KY. Acute retinal necrosis following recombinant subunit varicella-zoster virus vaccine. Am J Ophthalmol Case Rep. (2020) 20:100962. doi: 10.1016/j.ajoc.2020.100962
65. Richards PJ, Wingelaar MJ, Armbrust KR, Kopplin LJ. Uveitis reactivation following recombinant zoster vaccination. Am J Ophthalmol Case Rep. (2021) 23:101115. doi: 10.1016/j.ajoc.2021.101115
66. Lehmann A, Matoba A. Reactivation of herpes zoster stromal keratitis after HZ/su adjuvanted herpes zoster subunit vaccine. Ophthalmology. (2018) 125:1682. doi: 10.1016/j.ophtha.2018.08.030
67. Lu TJ, Ta CN. Reactivation of herpes zoster keratitis following shingrix vaccine. Case Rep Ophthalmol. (2022) 13:104–8. doi: 10.1159/000522272
68. Cunningham ET Jr., Moorthy RS, Moorthy RS, Zierhut M. Vaccine-associated uveitis. Ocul Immunol Inflamm. (2019) 27:517–20.
69. Watad A, Quaresma M, Brown S, Cohen Tervaert JW, Rodríguez-Pint I, Cervera R, et al. Autoimmune/inflammatory syndrome induced by adjuvants (shoenfeld’s syndrome) – an update. Lupus. (2017) 7:675–81. doi: 10.1177/0961203316686406
70. De Vries RD, Duprex WP, de Swart RL. Morbillivirus infections: an introduction. Viruses. (2015) 7:699–706.
71. Yanagi Y, Takeda M, Ohno S. Measles virus: cellular receptors, tropism and pathogenesis. J Gen Virol. (2006) 87:2767–79.
72. Griffin DE. Measles Virus. In Fields Virology. 6th ed. Philadelphia, PA: Lippincott Williams & Wilkins (2013).
74. Laksono BM, de Vries RD, McQuaid S, Duprex WP, de Swart RL. Measles virus host invasion and pathogenesis. Viruses. (2016) 8:210. doi: 10.3390/v8080210
75. Kayikçioglu O, Kir E, Söyler M, Güler C, Irkeç M. Ocular findings in a measles epidemic among young adults. Ocul Immunol Inflamm. (2000) 8:59–62. doi: 10.1076/0927-3948(200003)811-sft059
76. Serdaroğlu A, Gücüyener K, Dursun I, Aydin K, Okuyaz C, Subaşi M, et al. Macular retinitis as a first sign of subacute sclerosing panencephalitis: the importance of early diagnosis. Ocul Immun Inflamm. (2005) 13:405–10. doi: 10.1080/09273940490912335
77. Bolivar P, Lebon S, Borruat F-X. Total macular atrophy in subacute sclerosing panencephalitis. Klin Monbl Augenheilkd. (2012) 229:428–30. doi: 10.1055/s-0031-1299167
78. Nguyen NQ, Lee AG, McClure CD, Miller G. Subretinal lesions in subacute sclerosing panencephalitis. J AAPOS. (1999) 3:252–4. doi: 10.1016/s1091-8531(99)70012-1
79. Green SH, Wirtschafter JD. Ophthalmoscopic findings in subacute sclerosing panencephalitis. Br J Ophthalmol. (1973) 57:780–7.
80. Hiatt RL, Grizzard HT, McNeer P, Jabbour JT. Ophthalmoscopic manifestation of subacute sclerosing panencephalitis. Trans Am Acad Ophthalmol Otolarynogol. (1971) 75:344–50. doi: 10.1136/bjo.57.10.780
81. Gravina RF, Nakanishi AS, Faden A. Subacute sclerosing panencephalitis. Am J Ophthalmol. (1978) 86:106–9.
82. Tandon R, Khanna S, Sharma MC, Seshadri S, Menon V. Subacute sclerosing panencephalitis presenting as optic neuritis. Indian J Ophthalmol. (1999) 47: 250–2.
84. Perry RT, Murray JS, Gacic-Dobo M, Dabbagh A, Mulders MN, Strebel PM, et al. Progress towards regional measles elimination, worldwide, 2000–2014. Wkly Epidemiol Rec. (2015) 90:623–31.
85. Chan CC, Sogg RL, Steinman L. Isolated oculomotor palsy after measles immunization. Am J Ophthalmol. (1980) 89:446–8. doi: 10.1016/0002-9394(80)90019-7
86. Kuniyoshi K, Hatsukawa Y, Kimura S, Fujino T, Ohguro H, Nakai R, et al. Acute bilateral photoreceptor degeneration in an infant after vaccination against measles and rubella. JAMA Ophthalmol. (2017) 135:478–82. doi: 10.1001/jamaophthalmol.2017.0380
88. Pleschka S. Overview of influenza viruses. Curr Top Microbiol Immunol. (2013) 370:1–20. doi: 10.1007/82_2012_272
89. Weinberg RJ, Nerney JJ. Bilateral submacular hemorrhages associated with an influenza syndrome. Ann Ophthlmol. (1983) 15:710–2.
90. Rabon RJ, Louis GJ, Zegarra H, Gutman FA. Acute bilateral posterior angiopathy with influenza A viral infection. Am J Ophthalmol. (1987) 103:289–93.
91. Kovacs B. Alteration of blood-retina barriers in cases of viral retinitis. Int Ophthalmol. (1985) 8:159–66. doi: 10.1007/BF00136493
92. Fukami S, Wakakura M, Inouye J. Influenza retinitis: association with influenza encephalitis. Ophthalmology. (2005) 219:119–21. doi: 10.1159/000083273
93. Jo T, Mizota A, Hatano N, Tanaka M. Frosted branch angiitis-like fundus following presumed influenza virus type a infection. JPN J Ophthalmol. (2006) 50:563–4. doi: 10.1007/s10384-006-0358-5
94. Roesel M, Heinz C, Heiligenhaus A. H1N1 and uveal effusion syndrome. Ophthalmology. (2010) 117:1467–67.e1. doi: 10.1016/j.ophtha.2010.03.013
95. Breker DA, Stacey AW, Srinivasan A, Burstztyn LLCD, Trobe JD, Johnson MW. Vision loss caused bu retinal and lateral geniculate nucleus infarction in H1N1 influenza. J Neuroophthalmol. (2015) 35:265–9. doi: 10.1097/WNO.0000000000000247
96. Kawasaki A, Purvin VA, Tang R. Bilateral anterior ischemic optic neuropathy following influenza vaccination. J Neuroophthalmol. (1998) 18:56–9.
97. Demicheli V, Jefferson T, Di Pietrantonj C, Ferroni E, Thorning S, Thomas RE, et al. Vaccines for preventing influenza in the elderly. Cochrane Database Syst Rev. (2018) 2:CD004876. doi: 10.1002/14651858.CD004876.pub4
98. Ray CL, Dreizin IJ. Bilateral optic neuropathy associated with influenza vaccination. J Neuroophthalmol. (1996) 16:182–4.
99. Bienfang DC, Kantrowitz FG, Noble JL, Rayon AM. Ocular abnormalities after influenza immunization. Arch Ohthalmol. (1977) 95:1649.
100. Perry HD, Mallen FJ, Grodin RW, Cossari AJ. Reversible blindness in optic neuritis associated with influenza vaccination. Ann Ophthalmol. (1979) 11:545–50.
101. Rubinov A, Beiran I, Krasnitz I, Miller B. Bilateral optic neuritis after inactivated influenza vaccination. Isr Med Assoc J. (2012) 14:705–7.
102. Cangemi FE, Bergen RL. Optic atrophy following swine flu vaccination. Ann Ophthalmol. (1980) 12:857–63.
103. Macoul KL. Bilateral optic nervea trophy and blindness following swine influenza vaccination. Ann Ophthalmol. (1982) 14:398–9.
104. Goyal S, Nazarian SM, Thayi DR, Hammond F, Petrovic V. Multiple evanescent white dot syndrome following recent influenza vaccination. Can J Ophthalmol. (2013) 48:e115–6. doi: 10.1016/j.jcjo.2013.03.002
105. Mendrinos E, Baglivo E. Acute posterior multifocal placoid pigment epitheliopathy following influenza vaccination. Eye (Lond). (2010) 24:180–1. doi: 10.1038/eye.2009.68
106. Branisteanu D, Bilha A. Acute posterior multifocal placoid pigment epitheliopathy following influenza vaccination. Rom J Ophthalmol. (2015) 59:52–8.
107. Solomon A, Frucht-Pery J. Bilateral simultaneous corneal graft rejection after influenza vaccination. Am J Ophthalmol. (1996) 121:708–9. doi: 10.1016/s0002-9394(14)70638-5
108. Steinemann TL, Koffler BH, Jennings CD. Corneal allograft rejection following immunization. Am J Ophthalmol. (1988) 106:575–8.
109. Hamilton A, Massera R, Maloof A. Stromal rejection in a deep anterior lamellar keratoplasty following influenza vaccination. Clin Exp Ophthalmol. (2015) 43:838–9. doi: 10.1111/ceo.12560
110. Sonneveld MJ, Zoutendijk R, Janssen HLA. Hepatitis B surface antigen onitoring and management of chronic hepatitis B. J Viral Hepat. (2011) 18:449–57.
111. Hadziyannis SJ, Papatheodoris GV. Hepatitis B e antigen-negative chronic hepatitis B: natural history and treatment. Semin Liver Dis. (2006) 26:130–41.
112. Gower RG, Sausker WF, Kohler PF, Thorne GE, McIntosh RM. Small vessel vasculitis caused by hepatitis b virus immune complexes. J Allergy Clin Immunol. (1978) 62:222–8.
114. Farthing CF, Howard RS, Thin RN. Papillitis and hepatitis B. Br Med J (Clin Res Ed). (1986) 292:1712. doi: 10.1136/bmj.292.6537.1712
115. Galli M, Morelli R, Casellato A, Perna MC. Retrobulpbar optic neuritis in a patient with acute type b hepatitis. J Neurol Sci. (1986) 72:195–200. doi: 10.1016/0022-510x(86)90007-9
116. Sood A, Midha V, Sood N, Gupta D. Hepatitis b and pupil-sparing oculomotor nerve palresis. Clin Inf Dis. (1999) 29:1330–1. doi: 10.1086/313449
117. Bloom JN, Rabinowicz M, Schulman ST. Uveitis complicating autoimmune chronic active uveitis. Am J Dis Child. (1983) 137:1175–6.
118. Murra PI, Prasad J, Rahi AH. Status of hepatitis b virus in the aetilogy of uveitis in Great Britain. Br J Ophthalmol. (1983) 67:685–7.
119. Grob PJ, Martenet AC, Witmer R. Non specific immune parameters and hepatitis b antigens in patients with uveitis. Mod Probl Ophthalmol. (1976) 16:254–8.
120. London WT. Hepatitis b virus and antigen-antibody complex disease. N Eng J Med. (1977) 296:1528–9.
121. Ocama P, Opio C, Lee W. Hepatitis B virus infection: current status. Am J Med. (2005) 118:e15–413.
122. Shepard CW, Simard EP, Finelli L, Fiore AE, Bell BP. Hepatitis b virus infection: epidemiology and vaccination. Epidem Rev. (2006) 28:112–25.
123. Fraunfelder FW, Eric BS, Fraunfelder FT. Hepatitis b vaccine and uveitis: an emerging hypotesis suggest by review of 32 case reports. Cutan Ocul Toxic. (2010) 29:26–9. doi: 10.3109/15569520903427717
124. Fried M, Conon D, Conzelmann M, Steinmann E. Uveitis after hepatitis b vaccination. Lancet. (1987) 2:631–2.
125. Baglivo E, Safran AB, Borruat FX. Multiple evanescent withe dot syndrome after hepatitis b vaccine. Brief Rep. (1996) 122:431–2.
126. Brezin A, Massin-Korobelnik P, Boudin M, Gaudric A, LeHoang P. Acute posterior multifocal placoid pigment epitheliopathy after hepatitis B vaccine. Arch Ophthalmol. (1995) 113:297–300.
127. Brezin A, Lautier-Frau M, Hamedani M, Rogeaux O, LeHoang P. Visual loss and eosinophilia after recombinant hepatitis b vaccine. Lancet. (1993) 342:563–4. doi: 10.1016/0140-6736(93)91697-k
128. Devin F, Roques G, Disdier P, Rodor F, Weiller PJ. Occlusion of central retinal vein after heptitis B vaccination. Lancet. (1996) 347:1626. doi: 10.1016/s0140-6736(96)91112-5
129. Fledelius HC. Unilateral papilloedema after hepatitis B vaccination in a migraine patient. A case report including forensic aspects. Acta Ophthalmol Scand. (1999) 77:722–4. doi: 10.1034/j.1600-0420.1999.770626.x
130. Muferet E, Guven S, Akyux U, Bilgic O, Laloglu F. Optic neuritis following hepatitis B vaccination in a 9-years-old girl. J Chin Med Ass. (2009) 72:594–7. doi: 10.1016/S1726-4901(09)70435-6
131. Anonymous. Hepatitis B vaccines: reported reactions. World Health Org Drug Inform. (1990) 4:129.
132. Nuzzi R, Carucci LL, Tripoli F. COVID-19 and ocular implications: an update. J Ophthal Inflamm Infect. (2020) 10:20.
133. Huang C, Wang Y, Li X, Ren L, Zhao J, Hu Y, et al. Clinical features of patients infected with 2019 novel coronavirus in Wuhan, China. Lancet. (2020) 395:497–506.
134. Zhou P, Yang XL, Wang XG, Hu B, Zhang L, Zhang W, et al. A pneumonia outbreak associated with a new coronavirus of probable bat origin. Nature. (2020) 579:270–3.
135. Senanayake Pd, Drazba J, Shadrach K, Milsted A, Rungger-Brandle E, Nishiyama K, et al. Angiotensin II and its receptor subtypes in the human retina. Invest Ophthalmol Vis Sci. (2007) 48:3301–11.
136. Wagner J, Jan Danser AH, Derkx FH, de Jong TV, Paul M, Mullins JJ, et al. Demonstration of renin mRNA, angiotensinogen mRNA, and angiotensin converting enzyme mRNA expression in the human eye: evidence for an intraocular renin-angiotensin system. Br J Ophthalmol. (1996) 80:159–63. doi: 10.1136/bjo.80.2.159
137. Yan Sun LL, Pan X, Jing M. Mechanism of the action between the SARS-CoV S240 protein and the ACE2 receptor in eyes. J Virol. (2006) 6:783–6.
138. Wang D, Hu B, Hu C, Zhu F, Liu X, Zhang J, et al. Clinical characteristics of 138 hospitalized patients with 2019 novel coronavirus-infected pneumonia in Wuhan, China. JAMA. (2020) 323:1061–9. doi: 10.1001/jama.2020.1585
139. Wu P, Duan F, Luo C, Liu Q, Qu X, Liang L, et al. Characteristics of ocular findings of patients with coronavirus disease 2019 (covid-19) in Hubei province, China. JAMA Ophthalmol. (2020) 38:575–8.
140. Douglas KAA, Douglas VP, Moschos MM. Ocular manifestations of COVID-19 (SARS-CoV-2): a critical review of current literature. In Vivo. (2020) 34:1619–28. doi: 10.21873/invivo.1195
141. Cheema M, Aghazadeh H, Nazarali S, Ting A, Hodges J, McFarlane A, et al. Keratoconjunctivitis as the initial medical presentation of the novel coronavirus disease 2019 (covid-19). Can J Ophthalmol. (2020) 55:e125–9. doi: 10.1016/j.jcjo.2020.03.003
142. Dinkin M, Gao V, Kahan J, Bobker S, Simonetto M, Wechsler P, et al. Covid-19 presenting with ophthalmoparesis from cranial nerve palsy. Neurology. (2020) 95:221–3. doi: 10.1212/WNL.0000000000009700
143. Chen L, Liu M, Zhang Z, Qiao K, Huang T, Chen M, et al. Ocular manifestations of a hospitalised patient with confirmed 2019 novel coronavirus disease. Br J Ophthalmol. (2020) 104:748–51. doi: 10.1136/bjophthalmol-2020-316304
144. Navel V, Chiambaretta F, Dutheil F. Haemorrhagic conjunctivitis with pseudomembranous related to sars-cov-2. Am J Ophthalmol Case Rep. (2020) 19:100735. doi: 10.1016/j.ajoc.2020.100735
145. Jin SX, Juthani VV. Acute corneal endothelial graft rejection with coinciding COVID-19 infection. Cornea. (2021) 40:123–4. doi: 10.1097/ico.0000000000002556
147. Hadjadj J, Yatim N, Barnabei L, Corneau A, Boussier J, Smith N, et al. Impaired type I interferon activity and inflammatory responses in severe COVID-19 patients. Science. (2020) 369:718–24. doi: 10.1126/science.abc6027
148. Seah I, Agrawal R. Can the coronavirus disease 2019 (covid19) affect the eyes? A review of coronaviruses and ocular implications in humans and animals. Ocul Immunol Inflamm. (2020) 28:391–5.
149. Marinho PM, Marcos AAA, Romano AC, Nascimento H, Belfort R Jr. Retinal findings in patients with covid-19. Lancet. (2020) 395:1610. doi: 10.1016/S0140-6736(20)31014-X
150. Wang Y, Detrick B, Yu Z-X, Zhang J, Chesky L, Hooks JJ. The role of apoptosis within the retina of coronavirus-infected mice. Invest Ophthalmol Vis Sci. (2000) 41:3011–8.
151. Sahin U, Muik A, Derhovanessian E, Vogler I, Kranz LM, Vormehr M. COVID-19 vaccine BNT162b1 elicits human antibody and TH1 T cell responses. Nature. (2020) 586:594–9.
152. Jackson LA, Anderson EJ, Rouphael NG, Roberts PC, Makhene M, Coler RN, et al. An mRNA vaccine against SARS-CoV-2—preliminary report. N Engl J Med. (2020) 383:1920–31.
153. Mercado NB, Zahn R, Wegmann F, Loos C, Chandrashekar A, Yu J, et al. Single-shot Ad26 vaccine protects against SARS-CoV-2 in rhesus macaques. Nature. (2020) 586:583–8.
154. Arashkia A, Jalilvand S, Mohajel N, Afchangi A, Azadmanesh K, Salehi-Vaziri M, et al. Severe acute respiratory syndrome-coronavirus-2 spike (S) protein based vaccine candidates: state of the art and future prospects. Rev Med Virol. (2021) 31:e2183. doi: 10.1002/rmv.2183
155. Lee YK, Huang YH. Ocular manifestations after receiving COVID-19 vaccine: a systematic review. Vaccines (Basel). (2021) 9:1404. doi: 10.3390/vaccines9121404
156. Ng XL, Betzler BK, Testi I, Ho SL, Tien M, Ngo WK, et al. Ocular adverse events after COVID-19 vaccination. Ocul Immunol Inflamm. (2021) 29:1216–24. doi: 10.1080/09273948.2021.1976221
157. Baden LR, El Sahly HM, Essink B, Kotloff K, Frey S, Novak R, et al. Efficacy and safety of the mRNA-1273 SARS-CoV-2 vaccine. N Engl J Med. (2021) 384:403–16. doi: 10.1056/NEJMoa2035389
158. Heath PT, Galiza EP, Baxter DN, Boffito M, Browne D, Burns F, et al. Safety and efficacy of NVX-CoV2373 Covid-19 vaccine. N Engl J Med. (2021) 385:1172–83. doi: 10.1056/NEJMoa2107659
159. Shemer A, Pras E, Einan-Lifshitz A, Dubinsky-Pertzov B, Hecht I. Association of COVID-19 vaccination and facial nerve palsy: a case-control study. JAMA Otolaryngol Head Neck Surg. (2021) 147:739–43. doi: 10.1001/jamaoto.2021.1259
160. Reyes-Capo DP, Stevens SM, Cavuoto KM. Acute abducens nerve palsy following COVID-19 vaccination. J.AAPOS. (2021) 25:302–3.
161. Helmchen C, Buttler GM, Markewitz R, Hummel K, Wiendl H, Boppel T. Acute bilateral optic/chiasm neuritis with longitudinal extensive transverse myelitis in longstanding stable multiple sclerosis following vector-based vaccination against the SARS-CoV-2. J Neurol. (2021) 15:1–6. doi: 10.1007/s00415-021-10647-x
162. Maleki A, Look-Why S, Manhapra A, Foster CS. COVID-19 recombinant mRNA vaccines and serious ocular inflammatory side effects: real or coincidence? J Ophthalmic Vis Res. (2021) 16:490–501.
163. Al Khames Aga QA, Alkhaffaf WH, Hatem TH, Nassir KF, Batineh Y, Dahham AT, et al. Safety of COVID-19 vaccines. J Med Virol. (2021) 93:6588–94. doi: 10.3389/fmed.2021.691079
164. Mazzatenta C, Piccolo V, Pace G, Romano I, Argenziano G, Bassi A. Purpuric lesions on the eyelids developed after BNT162b2 mRNA COVID-19 vaccine: another piece of SARS-CoV-2 skin puzzle? J Eur Acad Dermatol Venereol. (2021) 35:e543–5. doi: 10.1111/jdv.17340
165. Furer V, Zisman D, Kibari A, Rimar D, Paran Y, Elkayam O. HerpeszosterfollowingBNT162b2mRNACovid-19vaccination in patients with autoimmune inflammatory rheumatic diseases: a case series. Rheumatology. (2021) 12:12.
166. Wasser LM, Roditi E, Zadok D, Berkowitz L, Weill Y. Keratoplasty rejection after the BNT162b2 messenger RNA vaccine. Cornea. (2021) 40:1070–2.
167. Crnej A, Khoueir Z, Cherfan G, Saad A. Acute corneal endothelial graft rejection following COVID-19 vaccination. J Fr Ophtalmol. (2021) 8:8.
168. Phylactou M, Li JPO, Larkin DFP. Characteristics of endothelial corneal transplant rejection following immunisation with SARS-CoV-2 messenger RNA vaccine. Br J Ophthalmol. (2021) 105:893–6. doi: 10.1136/bjophthalmol-2021-319338
169. ElSheikh RH, Haseeb A, Eleiwa TK, Elhusseiny AM. Acute uveitis following COVID-19 vaccination. Ocul Immunol Inflamm. (2021) 29:1207–9. doi: 10.1080/09273948.2021.1962917
170. Goyal M, Murthy SI, Annum S. Bilateral multifocal choroiditis following COVID-19 vaccination. Ocul Immunol Inflamm. (2021) 29:753–7. doi: 10.1080/09273948.2021.1957123
171. Mudie LI, Zick JD, Dacey MS, Palestine AG. Panuveitis following vaccination for COVID-19. Ocul Immunol Inflamm. (2021) 29:741–2. doi: 10.1080/09273948.2021.1949478
172. Renisi G, Lombardi A, Stanzione M, Invernizzi A, Bandera A, Gori A. Anterior uveitis onset after bnt162b2 vaccination: is this just a coincidence? Int J Infect Dis. (2021) 110:95–7. doi: 10.1016/j.ijid.2021.07.035
173. Papasavvas I, Herbort CP. Reactivation of vogt-koyanagi-harada disease under control for more than 6 years, following anti-SARS-CoV-2 vaccination. J OphthalmicInflamm Infect. (2021) 11:21. doi: 10.1186/s12348-021-00251-5
174. Furer V, Eviatar T, Zisman D, Peleg H, Paran D, Levartovsky D, et al. Immunogenicity and safety of the BNT162b2 mRNA COVID-19 vaccine in adult patients with autoimmune inflammatory rheumatic diseases and in the general population: a multicentre study. Ann Rheum Dis. (2021) 80:200–1.
175. Bøhler AD, Strøm ME, Sandvig KU, Moe MC, Jørstad ØK. Acute macular neuroretinopathy following COVID-19 vaccination. Eye (Lond). (2021) 36:644–5. doi: 10.1038/s41433-021-01610-1
176. Book BAJ, Schmidt B, Foerster AMH. Bilateral acute macular neuroretinopathy after vaccination against SARS-CoV-2. JAMA Ophthalmol. (2021) 139:e212471. doi: 10.1001/jamaophthalmol.2021.2471
177. Mambretti M, Huemer J, Torregrossa G, Ullrich M, Findl O, Casalino G. Acute macular neuroretinopathy following coronavirus disease 2019 vaccination. Ocul Immunol Inflamm. (2021) 29:730–3. doi: 10.1080/09273948.2021.1946567
178. Michel T, Stolowy N, Gascon P, Dupessey F, Comet A, Attia R, et al. Acute macular neuroretinopathy after COVID-19 vaccine. J Ophthalmic Inflamm Infect. (2021) 45:e299–302.
179. Hwang CK, Sen HN. Concurrent vascular flow defects at the deep capillary plexus and choriocapillaris layers in acute macular neuroretinopathy on multimodal imaging: a case series. Am J Ophthalmol Case Rep. (2020) 20:100866. doi: 10.1016/j.ajoc.2020.100866
180. Madendag Y, Acmaz G, Atas M, Sahin E, Tayyar AT, Madendag IÇ, et al. The effect of oral contraceptive pills on the macula, the retinal nerve fiber layer, and choroidal thickness. Med Sci Monit. (2017) 23:5657–61. doi: 10.12659/msm.905183
181. Fowler N, Mendez Martinez NR, Pallares BV, Maldonado RS. Acute-onset central serous retinopathy after immunization with COVID-19 mRNA vaccine. Am J Ophthalmol Case Rep. (2021) 23:101136. doi: 10.1016/j.ajoc.2021.101136
182. Subramony R, Lin LC, Knight DK, Aminlari A, Belovarski I. Bilateral retinal detachments in a healthy 22-year-old female following moderna SARS-CoV-2 vaccination. J Emerg Med. (2021) 16:S0736–4679.
183. Sharifian-Dorche M, Bahmanyar M, Sharifian-Dorche A, Mohammadi P, Nomovi M, Mowla A. Vaccine-induced immune thrombotic thrombocytopenia and cerebral venous sinus thrombosis post COVID-19 vaccination; a systematic review. J Neurol Sci. (2021) 428:117607. doi: 10.1016/j.jns.2021.117607
184. Greinacher A, Thiele T, Warkentin TE, Weisser K, Kyrle PA, Eichinger S. Thrombotic thrombocytopenia after ChAdOx1 nCov-19 vaccination. N Engl J Med. (2021) 384:2092–101. doi: 10.1056/NEJMoa2104840
185. Schultz NH, Sørvoll IH, Michelsen AE, Munthe LA, Lund-Johansen F, Ahlen MT, et al. Thrombosis and thrombocytopenia after ChAdOx1 nCoV-19 vaccination. N Engl J Med. (2021) 384:2124–30. doi: 10.1056/NEJMoa2104882
186. Perry RJ, Tamborska A, Singh B, Craven B, Marigold R, Arthur-Farraj P, et al. Cerebral venous thrombosis after vaccination against COVID-19 in the UK: a multicentre cohort study. Lancet. (2021) 398:1147–56. doi: 10.1016/S0140-6736(21)01608-1
187. See I, Su JR, Lale A, Woo EJ, Guh AY, Shimabukuro TT, et al. US case reports of cerebral venous sinus thrombosis with thrombocytopenia after Ad26.COV2.S vaccination, march 2 to April 21, 2021. JAMA. (2021) 325:2448–56. doi: 10.1001/jama.2021.7517
188. Schulz JB, Berlit P, Diener HC, Gerloff C, Greinacher A, Klein C, et al. COVID-19 vaccine-associated cerebral venous thrombosis in Germany. Ann Neurol. (2021) 90:627–39. doi: 10.1002/ana.26172
189. Panovska-Stavridis I, Pivkova-Veljanovska A, Trajkova S, Lazarevska M, Grozdanova A, Filipche V. Case of superior ophthalmic vein thrombosis and thrombocytopenia following ChAdOx1 nCoV-19 vaccine against SARS-CoV-2. Mediterr J Hematol Infect Dis. (2021) 13:e2021048. doi: 10.4084/mjhid.2021.048
190. Valerio GS, Lin CC. Ocular manifestations of herpes simplex virus. Curr Opin Ophthalmol. (2019) 30:525–31. doi: 10.1097/ICU.0000000000000618
191. Reynaud C, Rousseau A, Kaswin G, M’garrech M, Barreau E, Labetoulle M. Persistent impairment of quality of life in patients with herpes simplex keratitis. Ophthalmology. (2017) 124:160–9.
192. Cabrera-Aguas M, Robaei D, McCluskey P, Watson S. Clinical translation of recommendations from randomized trials for management of herpes simplex virus keratitis. Clin Exp Ophthalmol. (2018) 46:1008–16. doi: 10.1111/ceo.13319
193. Dong L-L, Tang R, Zhai Y-J, Malla T, Hu K. DNA vaccine expressing herpes simplex virus 1 glycoprotein C and D protects mice against herpes simplex keratitis. Int J Ophthalmol. (2017) 10:1633–9. doi: 10.18240/ijo.2017.11.01
194. Tang R, Zhai Y, Dong L, Malla T, Hu K. Immunization with dendritic cell-based DNA vaccine pRSC-NLDC145.gD-IL21 protects mice against herpes simplex virus keratitis. Immunotherapy. (2018) 10:189–200. doi: 10.2217/imt-2017-0060
195. Royer DJ, Hendrix JF, Larabee CM, Reagan AM, Sjoelund VH, Robertson DM, et al. Vaccine induced antibodies target sequestered viral antigens to prevent ocular HSV-1 pathogenesis, preserve vision, and preempt productive neuronal infection. Mucosal Immunol. (2019) 12:827–39. doi: 10.1038/s41385-019-0131-y
Keywords: virus infection, vaccine, ocular effects, varicella zoster virus, measles virus, influenza viruses, hepatitis B virus, SARS-CoV-2
Citation: Scalabrin S, Becco A, Vitale A and Nuzzi R (2022) Ocular effects caused by viral infections and corresponding vaccines: An overview of varicella zoster virus, measles virus, influenza viruses, hepatitis B virus, and SARS-CoV-2. Front. Med. 9:999251. doi: 10.3389/fmed.2022.999251
Received: 20 July 2022; Accepted: 30 September 2022;
Published: 26 October 2022.
Edited by:
Georgios Panos, Nottingham University Hospitals NHS Trust, United KingdomReviewed by:
Ravi Mahalingam, University of Colorado Anschutz Medical Campus, United StatesCopyright © 2022 Scalabrin, Becco, Vitale and Nuzzi. This is an open-access article distributed under the terms of the Creative Commons Attribution License (CC BY). The use, distribution or reproduction in other forums is permitted, provided the original author(s) and the copyright owner(s) are credited and that the original publication in this journal is cited, in accordance with accepted academic practice. No use, distribution or reproduction is permitted which does not comply with these terms.
*Correspondence: Raffaele Nuzzi, cHJvZi5udXp6aV9yYWZmYWVsZUBob3RtYWlsLml0
Disclaimer: All claims expressed in this article are solely those of the authors and do not necessarily represent those of their affiliated organizations, or those of the publisher, the editors and the reviewers. Any product that may be evaluated in this article or claim that may be made by its manufacturer is not guaranteed or endorsed by the publisher.
Research integrity at Frontiers
Learn more about the work of our research integrity team to safeguard the quality of each article we publish.