- 1Department of Parasitology and Mycology, School of Medicine, Iran University of Medical Sciences, Tehran, Iran
- 2Laboratory of Mucosal Immunology, Department of Biomedical Sciences, School of Medicine and Health Sciences, University of North Dakota, Grand Forks, ND, United States
Giardia duodenalis is an intestinal protozoan parasite of humans and animal hosts and comprises eight microscopically indistinguishable molecularly-diverse lineages designated as assemblages A–H. Assemblages A and B are the primary sources of infections in humans and a wide range of mammals. Here, we identified assemblages, and inter-/intra-assemblage genetic diversity of human G. duodenalis isolates based on the multilocus sequence typing of the triosephosphate isomerase (tpi), β -giardin (bg), and glutamate dehydrogenase (gdh) loci. Multilocus sequence analysis of 62 microscopically-positive G. duodenalis fecal samples identified 26 (41.9%), 27 (43.5%), and nine (14.5%) isolates belonging to assemblages A, B, and discordant assemblages, respectively. The tpi locus assemblage-specific primers identified dual infections with A and B assemblages (45.2%). The sequence analysis of multiple alignments and phylogenetic analysis showed low genetic polymorphism in assemblage A isolates, classified as sub-assemblage AII at three loci, subtype A2 at tpi and gdh loci, and subtype A2 or A3 at bg locus. High genetic variations were found in assemblage B isolates with 14, 15, and 23 nucleotide patterns at tpi, bg, and gdh loci, respectively. Further concatenated sequence analysis revealed four multilocus genotypes (MLG) in 24 assemblages A isolates, two previously-identified (AII-1 and AII-5), with one novel multilocus genotype. However, the high genetic variations observed in assemblage B isolates among and within the three genetic loci prevented the definitive designation of specific MLGs for these isolates. Multilocus sequence typing may provide new insight into the genetic diversity of G. duodenalis isolates in Tehran, suggesting that humans are likely a potential source of G. duodenalis infection. Further host-specific experimental transmission studies are warranted to elucidate the modes of transmission within multiple host populations.
Introduction
Giardia duodenalis (syn. Giardia lamblia, Giardia intestinalis) is a flagellate protozoan parasite that infects the small intestine of a wide range of mammals, including humans (1, 2). Giardia duodenalis is one of the most prevalent enteric protozoan parasites globally, with prevalence rates varying from 0.4 to 7.5% in developed countries to 8–30% in the developing world (3, 4). The G. duodenalis infections are initiated by ingesting quadrinucleate infective cysts through food or water contaminated with feces from infected humans or animals (3). Asymptomatic G. duodenalis infections are common in humans and, in most cases, are self-limited and cleared within weeks of exposure with no treatments (4, 5). Asymptomatic infections can lead to a malabsorption syndrome, characterized by failure to thrive and/or stunted growth, especially in children in developing countries. However, infected individuals with the symptomatic disease typically exhibit gastrointestinal symptoms, including diarrhea, abdominal discomfort, flatulence, nausea, and bloating (1, 4–6).
The G. duodenalis complex comprises eight morphologically-indistinguishable genotypes with molecularly diverse lineages designated as assemblages A–H (7, 8). Giardia duodenalis assemblages A and B infect humans and a variety of other mammals. Whereas, assemblages C–H are commonly found in dogs and other canids (C, D), hoofed livestock (E), cats (F), rodents (G), and marine mammals (H) (3, 4, 7). However, recent accumulating evidence has demonstrated that those assemblages thought to be only circulating within the livestock (i.e., assemblage E) could also infect humans (9–11). This suggests that host-specificity for at least some assemblages is less strict, and those adapted to non-human mammals might be able to infect humans. An allozyme analysis designated four sub-assemblages within assemblages A and B (AI–AIV and BI–BIV), of which AI, AII, BIII, and BIV have been mainly identified in humans (7, 12). Subsequent nucleotide sequence and phylogenetic analysis have confirmed sub-assemblages AI–AIII within assemblages A, with AI being isolated mainly from animals, whereas AII is predominantly identified in humans. Moreover, AIII is mostly reported in wild mammals (e.g., deer), with only two human cases, which have been recently reported (2, 4, 11, 13). In addition, multilocus sequence typing (MLST) has characterized 9–12 subtypes/genotypes at each of the individual loci within the three major sub-assemblages A (4). However, the phylogenetic analysis of the nucleotide sequences of the main used genetic loci has not identified distinct sub-assemblages within assemblage B, likely reflecting a high sequence diversity within this group not supported by the bootstrap analysis (2, 14). Assemblages A and B of G. duodenalis possess a wide range of mammalian hosts, including humans. Thus, infections with these two assemblages are potentially considered of zoonotic importance (2, 4).
Multiple genetic markers have been routinely employed to discriminate better the genetic diversity and the population dynamics within a given G. duodenalis assemblage (3, 7). To this end, the small subunit ribosomal RNA (SSU-rRNA), glutamate dehydrogenase (gdh), triosephosphate isomerase (tpi), and β-giardin (bg) genes are among the most commonly-used loci to identify multiple variants of G. duodenalis in different host species (3, 7). The SSU-rRNA gene is a multi-copy and highly-conserved locus, making it a feasible genetic surrogate for detecting and differentiating G. duodenalis assemblages. In contrast, this locus is considered less useful in identifying intra-assemblage genetic diversity due to its conserved nature and usually short amplified fragments in most PCR assays based on the SSU-rRNA locus (2). In contrast to the SSU-rRNA locus, the single-copy tpi, bg, and gdh loci are more sensitive to probing the genetic variation and the classification of G. duodenalis populations at the sub-assemblage and genotype levels. However, these loci are not considered feasible candidates for diagnosing G. duodenalis in clinical settings (8, 15). Despite existing consensus over the feasibility of these loci to genetically classify multiple G. duodenalis assemblages, conflicting findings have been reported regarding the usefulness of a given single locus in differentiating G. duodenalis populations into assemblages and sub-assemblages (3, 16). Therefore, to expand accuracy, a numeric multilocus genotyping (MLG) system was introduced using tpi, bg, and gdh genes in analysis simultaneously (17).
Giardia is still considered the most-identified intestinal parasite in Iran, although its overall prevalence rates have dramatically decreased in recent years (18–20). The molecular characterization of the human G. duodenalis isolates in Iran was predominantly conducted using a single locus (21–26). In the current study, however, we employed an MLST approach to fill a gap in our understanding of the population structure, and genetic diversity of G. duodenlais isolates circulating in a major metropolitan area in Iran.
Materials and methods
Study subjects and DNA preparation
From June to November 2015, 41 fecal samples positive for G. duodenalis cysts by microscopy were collected from individuals referred to health centers in Tehran for routine stool screenings. Furthermore, archival DNA specimens from an additional 21 fecal samples positive for G. duodenalis cysts (2009–2014) were also included for further analyses.
In total, 62 G. duodenalis isolates from infected individuals were included in the current study, of which 42 (67.74%) and 18 (29.03%) were males and females, respectively. However, the genders of two participants (3.23%) were not determined. The mean age was 37.1 ± 20.9 years, ranging from 3 to 86 years. Gastrointestinal symptoms were reported by 12 (19.4%) of 62 participants, while 50 (80.6%) were asymptomatic. The most common gastrointestinal symptoms, including diarrhea (n = 10), cramps (n = 9), abdominal pain (n = 8), nausea (n = 5), vomiting (n = 3), flatulence (n = 6), anorexia (n = 6), and constipation (n = 4), were reported. Collected stool samples were immediately transferred to the research laboratory of the Department of Parasitology and Mycology, School of Medicine, Iran University of Medical Sciences at 4°C for further laboratory examination on the same day.
The presence of G. duodenalis cysts in fresh fecal samples was confirmed by light microscopy or a formalin-ether concentration method on a pea-sized piece of fecal samples, followed by further G. duodenalis cysts purification using a sucrose flotation gradient technique on the remaining fresh samples (27) to achieve adequate quantity and quality of G. duodenalis DNA for sequencing (5, 28). Briefly, 10 g of fresh feces was added to 50 mL of PBS (pH 7.4) and thoroughly mixed. The fecal suspension was passed through three layers of clean gauze, followed by centrifugation at 800 × g for 5 min. The sediments were re-suspended in 50 mL of PBS, and 25 mL of the suspension was layered over 20 mL of 1M sucrose solution (specific gravity = 1.13) in a clean 50-mL conical tube and were further centrifuged at 800 × g for 5 min. The interface and the upper layer were carefully transferred to a clean 50-mL conical tube and centrifuged at 800 × g for 5 min. The fecal pellets were washed three times with PBS and re-suspended in 0.4 mL of PBS containing 2% polyvinylpolypyrrolidone (PVPP). The Purified cysts were kept at −80°C for 24 h before DNA extraction. The DNA was extracted from purified cysts using a QIAamp DNA Mini Kit (QIAGEN, Germany) according to the manufacturer's instructions with some modifications (29). The extracted DNA was stored at −20°C for further analysis.
Multilocus genotyping of G. duodenalis isolates
Nested PCR amplification of the tpi and bg Loci
A 530-bp fragment of the tpi locus was specifically amplified using external forward and reverse primers AL3543 and AL3546 and internal forward and reverse primers AL3544 and AL3545, respectively (Table 1) (30). Both primary and secondary PCR reactions were performed in 50 μL volume, containing 25 μL of 2 × Taq DNA Polymerase Master Mix RED (Amplicon III, Copenhagen, Denmark), 0.2 μM of each primer, and 2 μL of template DNA. The amplification scheme consisted of an initial denaturation step at 95°C for 5 min, 35 amplification cycles at 94°C for 45 s, 50°C for 45 s, 72°C for 60 s, with a final extension at 72°C for 10 min. In the second PCR, the annealing temperature was increased to 58°C, whereas other parameters were left unchanged (36).
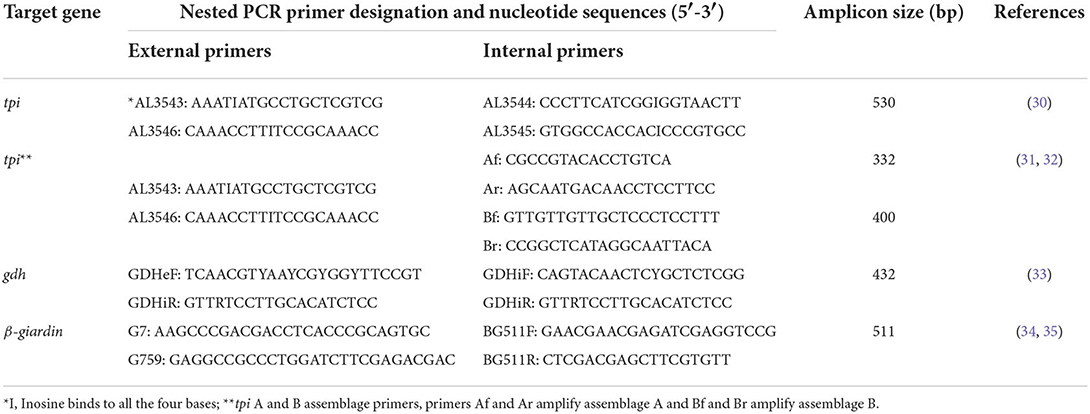
Table 1. Primer sequences and target genes used for molecular identification of Giardia duodenalis assemblages and multilocus sequence genotyping.
Mixed infections with assemblages A and B (A+B) were identified by amplifying the Giardia tpi gene using a nested-PCR protocol described elsewhere (31, 32). The primary PCR reaction was performed as described above, whereas the second PCR reaction was conducted using primers Af and Ar for assemblage A and Bf and Br for assemblage B (Table 1). These primers are designed to amplify a 332-bp and a 400-bp fragment within the tpi locus of assemblages A and B, respectively. The secondary PCR was accomplished in 50 μL volumes with 25 μL of 2 × Taq DNA Polymerase Master Mix RED (Amplicon III, Copenhagen, Denmark), 1–2 μL of the first PCR product as template DNA, and 0.2 μM of each primer (assemblage A) or 0.4 μM (assemblage B). The amplification strategy consisted of an initial denaturation step at 95°C for 5 min, 35 cycles at 94°C for 45 s, 64°C (assemblage A) or 62°C (assemblage B) for 45 s, 72°C for 60 s, followed by a final extension at 72° C for 10 min (36).
A 511-bp fragment within the bg gene was amplified using external and internal forward and reverse primers G7, G759, BG511F, and BG511R (Table 1) (34, 35). The primary and secondary PCR reactions were performed in 50 μL volume, containing 25 μL of 2 × Taq DNA Polymerase Master Mix RED (Amplicon III, Copenhagen, Denmark), 0.2 μM of each primer, and 2 μL of template DNA. The amplification scheme consisted of an initial denaturation step at 95°C for 5 min, 35 cycles at 95°C for 30 s, 65°C for 30 s (55°C for secondary PCR), 72°C for 30 s, and a final extension at 72°C for 7 min (36).
Semi-nested PCR amplification of the gdh locus
A 432-bp fragment of the gdh gene was amplified using external forward and reverse primers GDHeF and GDHiR and internal forward primer GDHiF and reverse primer GDHiR (Table 1) (33). The primary and secondary PCR reactions were performed in 50 μL volume, containing 25 μL of 2 × Taq DNA Polymerase Master Mix RED (Amplicon III, Copenhagen, Denmark), 0.5 μM of each primer, and 2 μL of template DNA. The amplification scheme consisted of an initial step at 94°C for 3 min, 1 cycle at 94°C for 2 min, 61°C for 1 min, and 68°C for 2 min, followed by 30 amplification cycles at 94°C for 30 s, 61°C for 20 s, 68°C for 20 s and a final extension at 68°C for 7 min. The secondary PCR amplification consisted of an initial step at 94°C for 3 min, 1 cycle at 94°C for 2 min, 60°C for 1 min, and 65°C for 2 min, followed by 15 amplification cycles at 94°C for 30 s, 60°C for 20 s, 65°C for 20 s with a final extension at 65° C for 7 min.
All PCR reactions were performed using a Gene Atlas thermocycler (Astec Co., Ltd, Fukuoka, Japan). The DNA obtained from a Giardia reference strain (ATCC® Number, 30888™) and sterile distilled nuclease-free water were included as positive and negative controls, respectively. The PCR products were fractionated on a 1.5% (W/V) agarose gel (SinaClon, Tehran, Iran) in Tris-acetate-EDTA (TAE) buffer, stained with ethidium bromide (0.05 mg/mL), and were visualized under UV illumination (GeneFlash, Syngene Bio-Imaging, Cambridge, UK).
Sequence and phylogenetic analysis
The nested- or semi-nested PCR products for each locus were excised and gel-purified using a MinElute Gel Extraction Kit (Qiagen, Hilden, Germany) and were subjected to sequence analysis in both directions (Macrogen, Korea). The DNA sequences were viewed and read by the CHROMAS software (Technelysium Pty Ltd., Queensland, Australia) and further aligned and assembled with the DNASIS MAX program (v. 3.0; Hitachi, Yokohama, Japan). The DNA sequences were blasted (http://blast.ncbi.nlm.nih.gov) to compare homology against DNA sequences deposited in GenBank. The DNA sequences from the tpi, bg, and gdh loci were combined to achieve concatenated sequence for each G. duodenalis isolate successfully amplified at the three loci (37).
The phylogenetic analysis was performed in MEGA X (www.megasoftware.net) using the maximum likelihood (ML) with evolutionary distances calculated by the best-fitting model to describe a robust estimate of the evolutionary distances. Models with the lowest Bayesian Information Criterion (BIC) scores best describe the substitution pattern. In addition, bootstrap analysis was performed with 1,000 replicates to evaluate the reliability of clusters. The sequences obtained from this study were deposited in the GenBank under the accession numbers LC183913–LC183966, LC184067–LC184028, and LC184423–LC184474 for tpi, bg, and gdh, respectively.
Statistical analysis
The demographics and the association between symptomatic and a given G. duodenalis assemblages were analyzed using SPSS 24.0 software (SPSS Inc., Chicago, IL, USA), and data were presented with 95% confidence intervals.
Results
Giardia duodenalis assemblage identification
The multilocus sequence analysis of 62 G. duodenalis-positive fecal samples using the tpi, bg, and gdh genes identified 26 isolates as assemblage A (41.9%), and 27 isolates (43.5%) as assemblage B, whereas nine G. duodenalis isolates (14.5%) showed inconsistent assemblage classification, also referred to as “discordant assemblages” (Table 2). Using primers targeting the G. duodenalis tpi locus of both assemblages A and B, we found 9 (14.5%) and 23 (37.1%) isolates as assemblages A and B, respectively. However, 28 (45.2%) clinical samples harbored both assemblages A and B (Tables 2, 3).
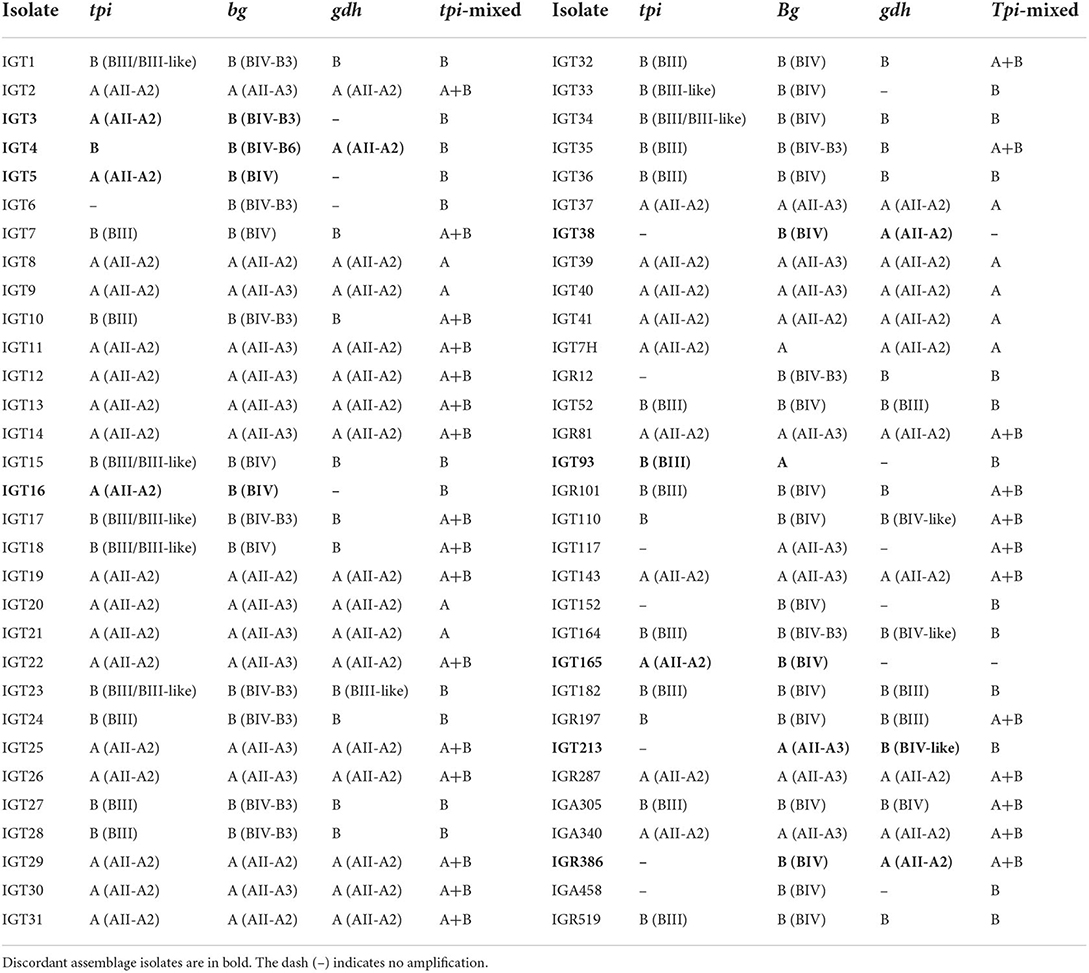
Table 2. Assemblages (subassemblage-subtype) A and B identification based on tpi, bg, and gdh loci and mixed A and B infections according to tpi A and B assemblage-specific primers.
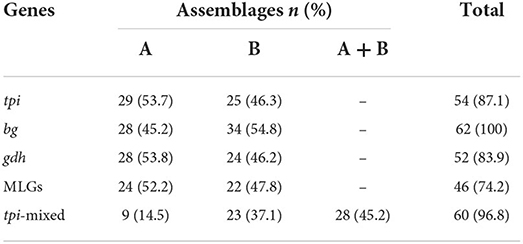
Table 3. Identification of assemblages A and B based on tpi, bg, and gdh loci, multilocus genotypes (MLGs) and mixed infection according to tpi A and B assemblage-specific primers.
The amplification of the bg locus was successful in all 62 isolates (100%), whereas 54 (87.1%) and 52 (83.9%) of the isolates were successfully amplified by targeting tpi, and gdh loci, respectively. Consequently, 48 and 10 isolates were characterized in three and two loci, respectively, and four isolates were only amplified at the bg locus. The sequence analysis of the tpi and bg genes identified 29 (53.7%) and 28 (45.2%) isolates as assemblage A, and 25 (46.3%) and 34 (54.8%) isolates as assemblage B, respectively. Consistently, the amplification of the gdh gene identified 28 (53.8%) and 24 (46.2%) of G. duodenalis isolates as assemblages A and B, respectively (Table 3).
The MLG typing of G. duodenalis isolates from infected individuals without (n = 50) and with (n = 12) clinical symptoms showed 21 (42.0%; 95% CI 29.4–55.8) and 22 (44.0%; 95% CI 31.2–57.7), and 7 (14.0%; 95% CI 7.0–26.2) of the asymptomatic individual were infected with assemblages A, B, and discordant assemblages, respectively. Furthermore, symptomatic individuals were equally infected with assemblages A (5) and B (5) (41.7%; 95% CI 19.3–68.0), while discordant assemblages were detected in 2 (16.7%; 95% CI 4.7–44.8). There was no statistical association between assemblages and symptoms.
Molecular characterization of G. duodenalis isolates within assemblage A: The sole occurrence of AII
Multiple sequence alignments and the phylogenetic tree construction based on the tpi locus classified all 29 assemblage A isolates as sub-assemblage AII, subtype A2 (AII/A2), placing these isolates in a single cluster with AII/A2, as evidenced by a strong bootstrap value (Figure 1). Furthermore, the multiple alignments based on the tpi gene sequences also identified three-nucleotide substitution patterns, where 27 isolates, as represented by IGT2 (LC183914), had a 100% homology with the AII reference sequences (U57897, KJ888993). In comparison, two isolates demonstrated a single nucleotide substitution at positions 536 (T → G) and 445 (G → A) (Table 4).
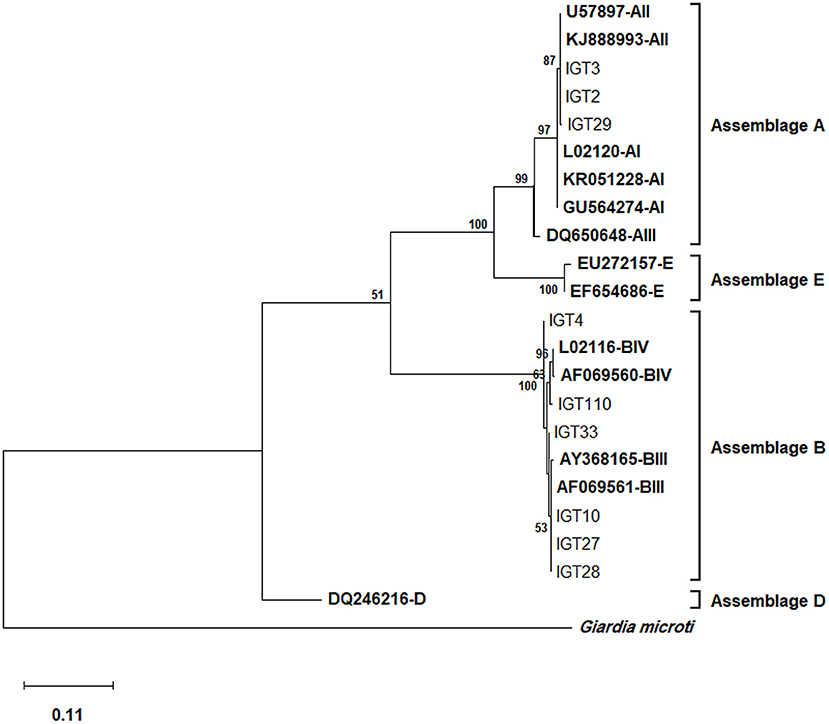
Figure 1. The phylogenetic analysis of the tpi gene nucleotide sequences of Giardia duodenalis using the Maximum Likelihood (ML) method and Tamura 3-parameter model (38) (T92 + G + I). The analysis involved 23 nucleotide sequences: Nine representative nucleotide sequences of tpi retrieved from this study (LC183913–LC183966) compared with 13 reference sequences of known assemblages from Genbank (indicated in bold) with Giardia microti as an outgroup. The percentage of trees in which the associated taxa clustered together (achieved from 1000 replicates) is shown next to the branches, only bootstrap values >50% are demonstrated. A discrete Gamma distribution was used to model evolutionary rate differences among sites [5 categories (+G, parameter = 9.6789)]. The rate variation model allowed for some sites to be evolutionarily invariable [(+I), 27.96% sites]. The scale bar represents substitutions per nucleotide. The final dataset contained 456 positions. Evolutionary analyses were conducted in MEGA X (39).
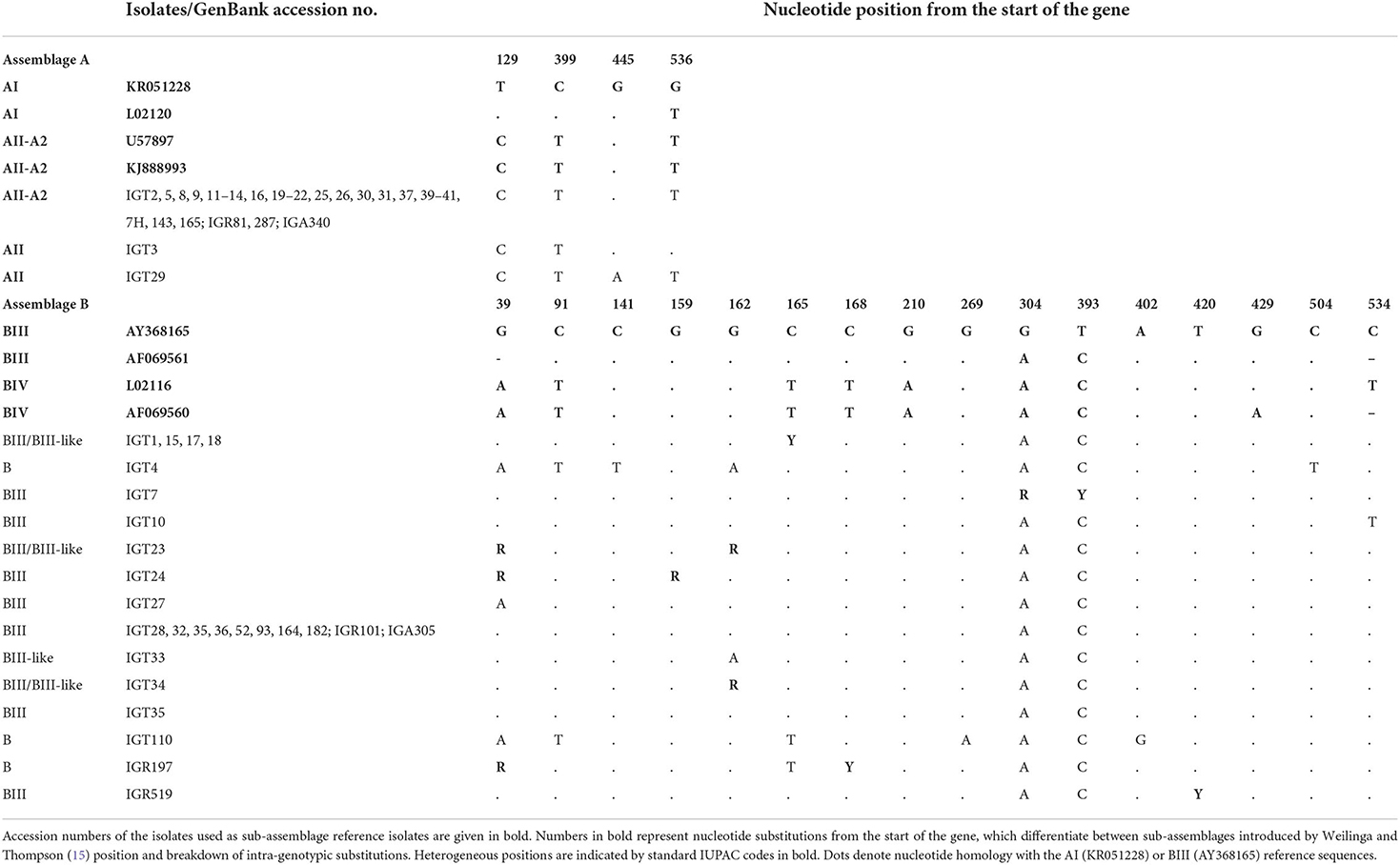
Table 4. Triosephosphate isomerase (tpi) multiple alignment sequence isolates in this study with reference sequences retrieved from GenBank, representing position of intra-genotypic substitutions in assemblages and sub-assemblages of assemblages A and B.
As depicted in Figure 2, the phylogenetic analysis based on the bg locus placed five isolates (representative: IGT8) in a single cluster with sub-assemblage AII, subtype A2 (AII/A2), whereas 18 isolates (representative: IGT2) were classified in a clade together with sub-assemblage AII, subtype A3 (AII/A3). The bg locus sequence analysis found six distinct nucleotide substitution patterns, with two isolates displaying sequence homology to subtype A3 with a single substitution at the nucleotide position 460 (T → C) and one isolate with two nucleotide substitutions at positions 303 (A → G) and 460 (T → C). Furthermore, two G. duodenalis assemblage A isolates had multiple nucleotide substitutions and overlapping nucleotide peaks in fifteen positions, preventing them from being further characterized at the sub-assemblage/subtype level, as shown in Table 5.
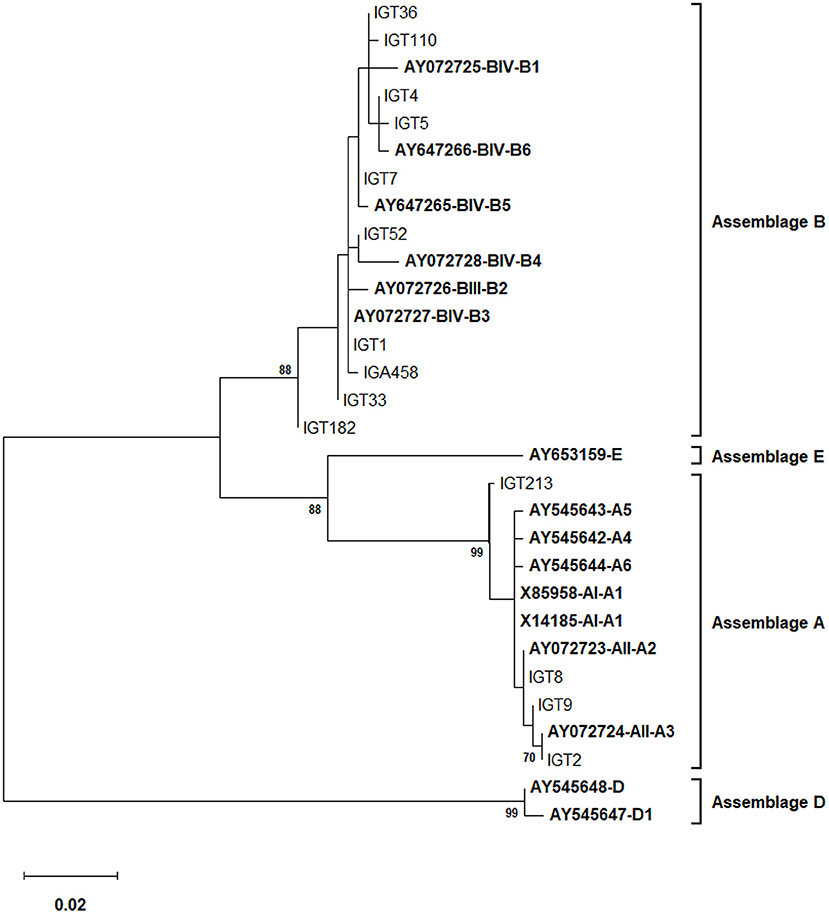
Figure 2. The phylogenetic analysis of the bg gene nucleotide sequences of Giardia duodenalis using the Maximum Likelihood method (ML) and Tamura 3-parameter model (38) (T92 + G + I). The analysis involved 30 nucleotide sequences: 14 representative nucleotide sequences of bg retrieved from this study (LC183967–LC184028) compared with 16 reference sequences of known assemblages from Genbank which are indicated in bold. The percentage of trees in which the associated taxa clustered together (achieved from 1,000 replicates) is shown next to the branches, only bootstraps values >50% are demonstrated. A discrete Gamma distribution was used to model evolutionary rate differences among sites [5 categories (+G, parameter = 0.1294)]. The rate variation model allowed for some sites to be evolutionarily invariable [(+I), 42.59% sites]. The scale bar represents substitutions per nucleotide. The final dataset included 506 positions. Evolutionary analyses were conducted in MEGA X (39).
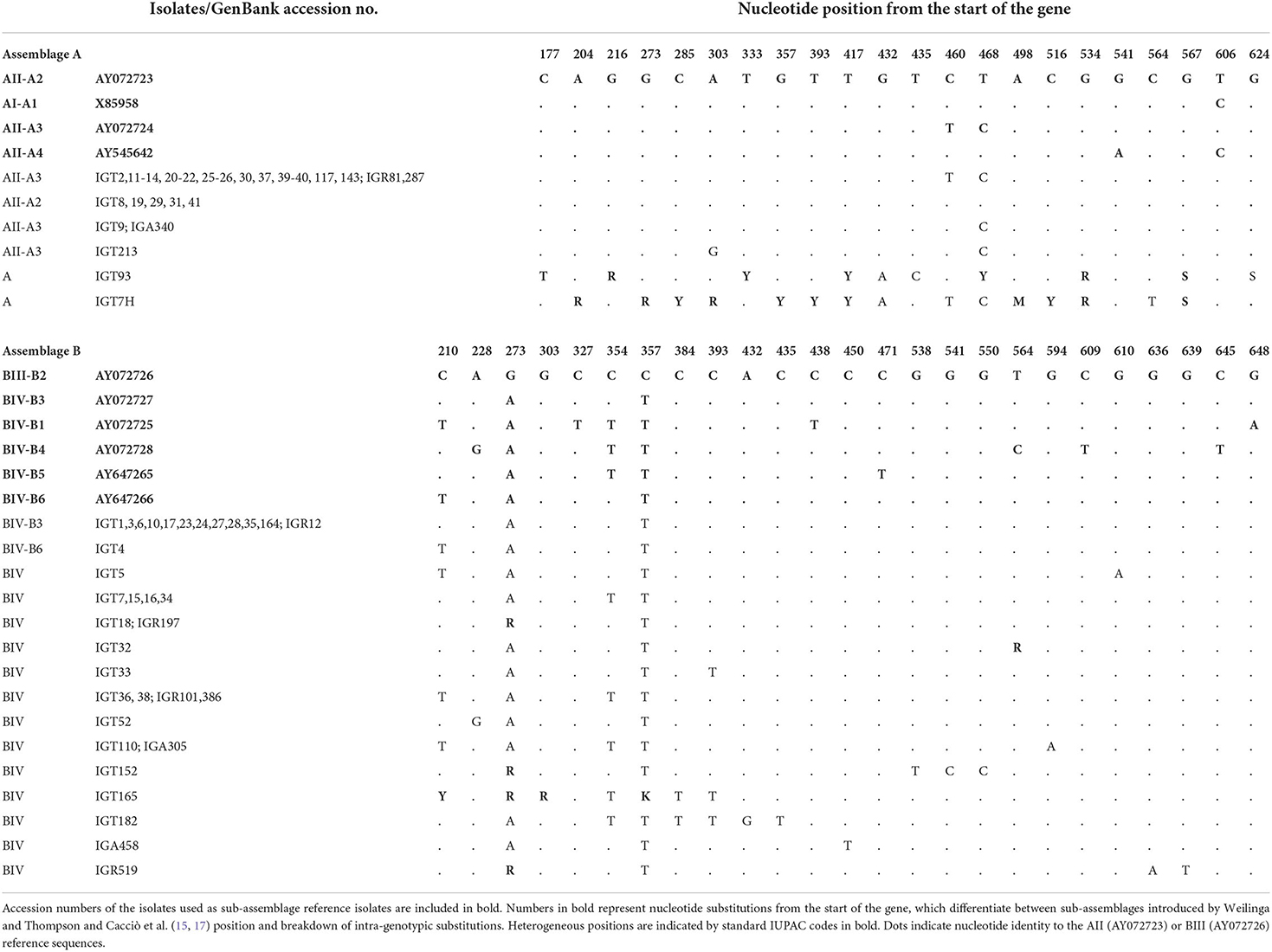
Table 5. β -giardin (bg) multiple alignment sequence isolates in this study with reference sequences retrieved from GenBank, representing position of intra-genotypic substitutions in sub-assemblages of assemblages A and B.
Based on the gdh locus sequence analysis, the phylogenetic tree construction placed all 28 assemblage A isolates in a single cluster with a sub-assemblage/subtype AII/A2 (L40510), with a 99% bootstrap value (Figure 3). Furthermore, the multiple alignments using gdh sequences also demonstrated that 27 isolates possessed a 100% identity with the A2 subtype (L40510) of G. duodenalis, whereas an isolate (i.e., IGT4) showed a single substitution (A → G) at the nucleotide position 562 (Table 6).
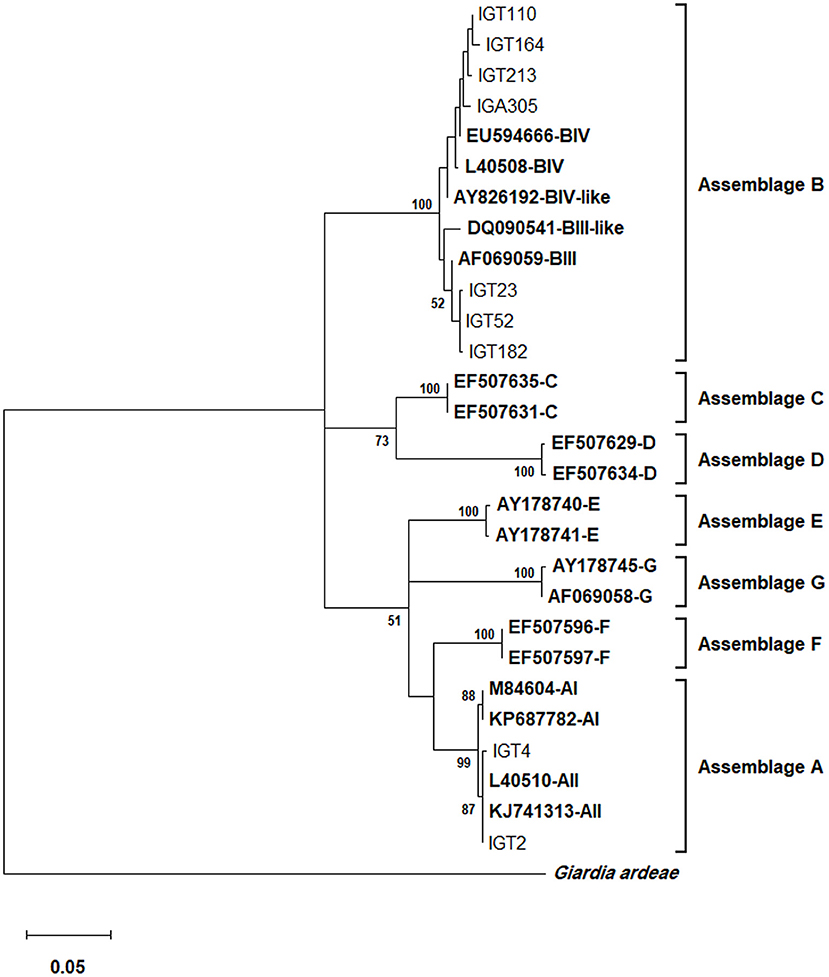
Figure 3. The phylogenetic analysis of the gdh gene nucleotide sequences of Giardia duodenalis using the Maximum Likelihood method and Tamura 3-parameter model (38) (T92 + G + I model). This analysis involved 29 nucleotide sequences: Nine representative nucleotide sequences of gdh retrieved from this study (LC184423–LC184474) compared with 19 reference sequences of known assemblages from Genbank (indicated in bold) with Giardia ardeae as an outgroup. The percentage of trees in which the associated taxa clustered together (achieved from 1,000 replicates) is shown next to the branches, only bootstraps values >50% are demonstrated. A discrete Gamma distribution was used to model evolutionary rate differences among sites [5 categories (+G, parameter = 0.4823)]. The rate variation model allowed for some sites to be evolutionarily invariable [(+I), 36.49% sites]. The scale bar represents substitutions per nucleotide. A total of 433 positions were evaluated in the final dataset. Evolutionary analyses were conducted in MEGA X (39).
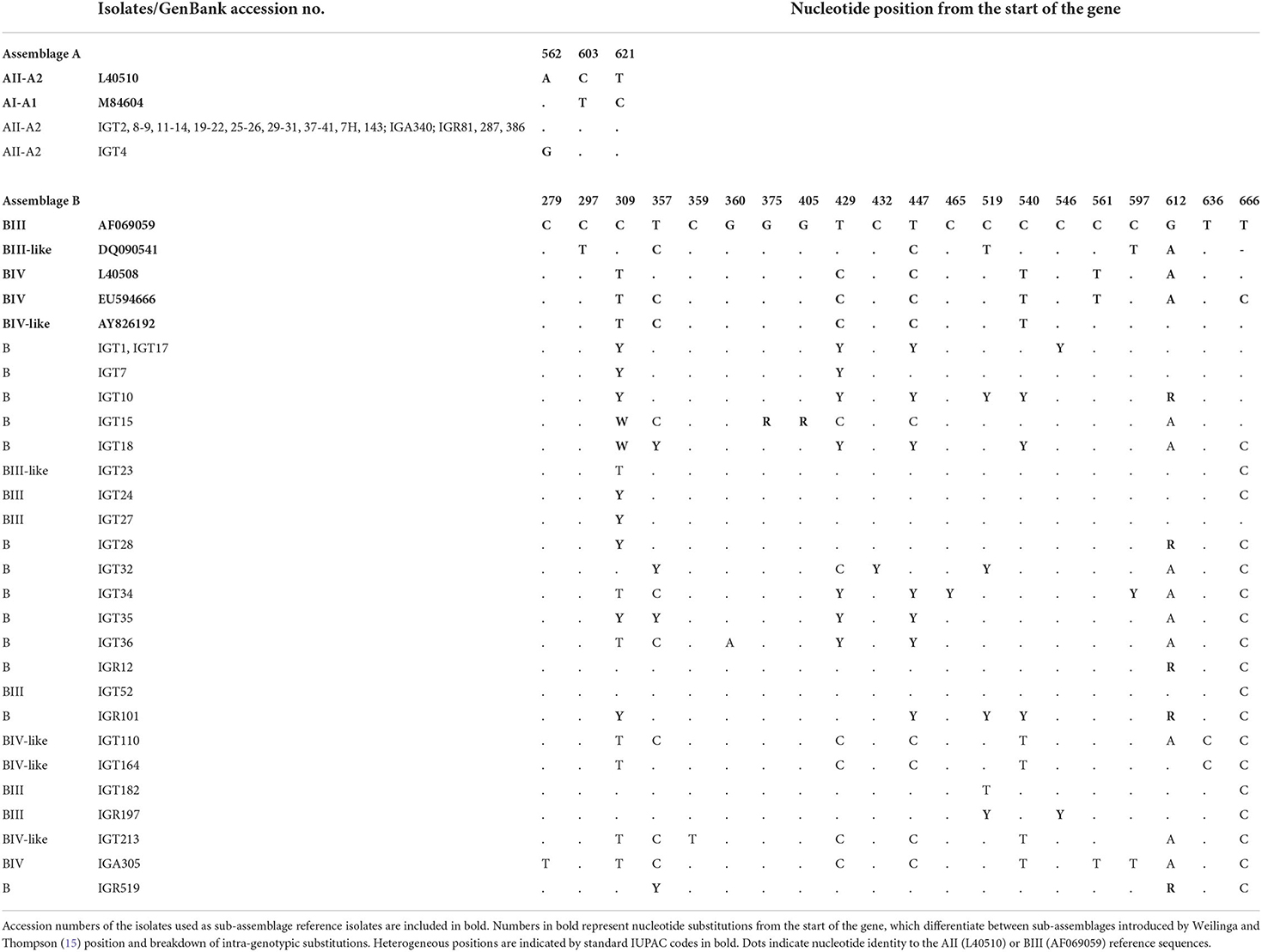
Table 6. Glutamate dehydrogenase (gdh) multiple alignment sequence isolates in this study with reference sequences retrieved from GenBank, representing the position of intra-genotypic substitutions in sub-assemblages of assemblages A and B.
Molecular characterization of G. duodenalis isolates within assemblage B
Multiple sequence alignments based on the tpi locus amplification identified 25 isolates as assemblage B of G. duodenalis, representing 14 distinct nucleotide substitution patterns (Table 4). Fifteen isolates (15/25) were characterized as sub-assemblage BIII, of which 10 isolates had a 100% identity with the reference sequence AF069561. Moreover, two isolates showed a single nucleotide substitution at positions 534 (IGT10) and 39 (IGT27), which were not in the sub-assemblage-defining positions (hotspot sites) (15). Sequences of 10 isolates (10/25, 40%) presented overlapping nucleotide peaks in eight positions. Only two were in hotspot sites, so double peaks did not interfere with the characterization of sub-assemblage BIII in three isolates. The comparative sequence analysis between 14 distinct nucleotide substitution patterns and the reference sequences representing BIII and BIV categorized one isolate as BIII-like, and six isolates showed overlapping nucleotide peaks at one or two positions could classify the isolates as BIII/BIII-like of G. duodenalis. Further characterization of the remaining three isolates initially identified as assemblage B was not attainable at sub-assemblage levels (Table 4). As shown in Figure 1, the phylogenetic analysis showed the monophyletic group of assemblage B with bootstrap support of 100%.
Multiple sequence alignments based on the bg locus confirmed that all 34 isolates initially identified as assemblage B belonged to the BIV sub-assemblage of G. duodenalis, representing a total of 15 nucleotide sequences patterns (Table 5). In addition, two nucleotide substitution patterns representing 12 isolates (i.e., IGT1) and one isolate (IGT4) showed a 100% identity with the B3 (AY072727) and B6 (AY647266) subtype reference sequences of G. duodenalis, respectively. Sequences of six isolates (6/34, 17.6%) presenting overlapping nucleotide peaks in five positions were not in hotspot sites, so double peaks did not interfere characterization of sub-assemblage BIV. The nucleotide heterogeneity and genotype characterization of all 34 isolates are detailed in Table 5. The phylogenetic tree construction based on the bg locus clustered all assemblage B isolates of G. duodenalis in a single clade, with bootstrap support of 88% (Figure 2).
Further sequence alignment based on the gdh locus amplification identified 23 nucleotide substitution patterns, representing 24 assemblage B G. duodenlais isolates (Table 6). Nucleotide sequences of 17 isolates (17/24, 70.8%) presenting double nucleotide peaks in 13 positions mostly were in hotspot sites. Ten isolates were classified at sub-assemblage levels, whereas fourteen isolates exhibited nucleotide substitutions or ambiguous nucleotides at sub-assemblage–defining positions and could not be further characterized at sub-assemblage levels (Table 6). The phylogenetic analysis confirmed that the assemblage B clinical isolates all clustered in a monophyletic clade, supported by a 100% bootstrap value, as shown in Figure 3.
Finally, the sub-assemblage classification of assemblage B isolates by the three markers revealed inconsistent genotyping results at the intra-assemblage level: assemblage B isolates were tentatively classified as sub-assemblages BIII (tpi locus), BIV (bg locus), and BIII or BIV (gdh locus) (Table 2).
Multilocus genotyping of G. duodenalis isolates
Employing an MLST approach, forty-six G. duodenalis isolates were successfully amplified, sequenced, and genotyped using the tpi, bg, and gdh loci. The simultaneous sequence analysis of the three loci (tpi + bg + gdh) were combined for each isolate to obtain the corresponding concatenated sequences (37). Further sequence alignment and phylogenetic analysis on the 29 concatenated sequences with unambiguous (no double peak) positions revealed that 24 G. duodenalis isolates were assemblage A in four distinct haplotypes. Besides, five isolates were assemblage B with five haplotypes (Table 7; Figure 4). The phylogenetic tree construction using obtained concatenated sequences showed that those clinical isolates initially identified as assemblage A or assemblage B of G. duodenalis clustered in two monophyletic branches with robust bootstrap support of 100%, being completely separated from each other and the host-specific assemblages C to G (Figure 4). Moreover, 24 G. duodenalis isolates initially identified as assemblage A were further classified into four MLGs (Table 7; Figure 4). As a result, we identified four isolates as MLG AII-1, profile A2/A2/A2, and 17 isolates as MLG AII-5, profile A2/A3/A2. Furthermore, two isolates were referred to as MLG AII-5, which showed one nucleotide substitution compared with AY072724 (4, 40). These two MLGs have been previously reported (3, 4, 17). Interestingly, we identified one novel MLG for assemblage A isolates, whose MLG could not be classified based on previously-proposed nomenclature (4, 17, 41) (Table 7). More specifically, a single isolate (IGT29) was designated as MLG AII-1N, (Table 7). No further definite classification of assemblage B isolates based on identified MLGs was not possible, since additional information on the nomenclature of assemblage B MLGs is not currently available (Figure 4).
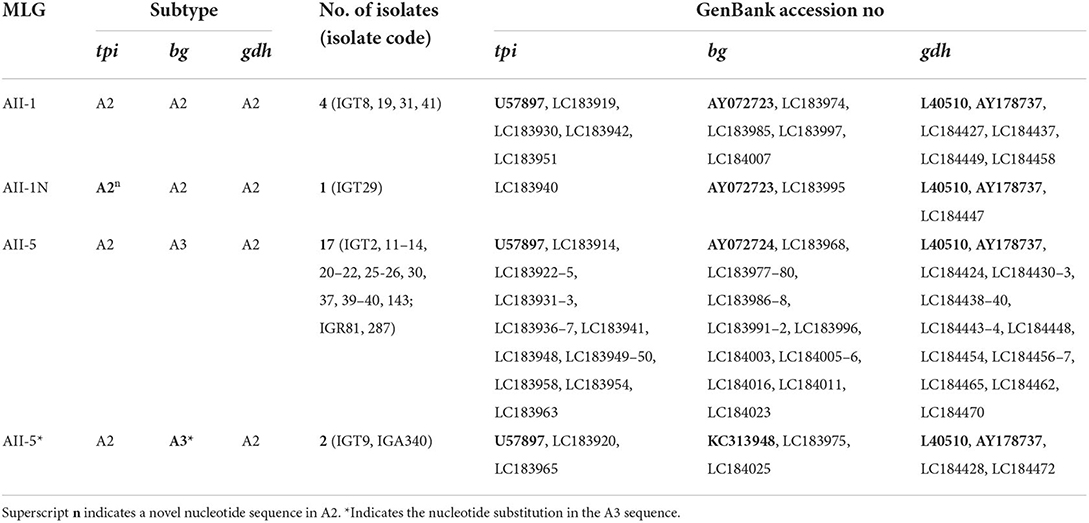
Table 7. Multilocus genotyping (MLG) and subtypes in Giardia duodenalis assemblage A–positive of Iranian isolates according to sequencing data from tpi (triosephosphate isomerase), bg (β -giardin), and gdh (glutamate dehydrogenase) loci.
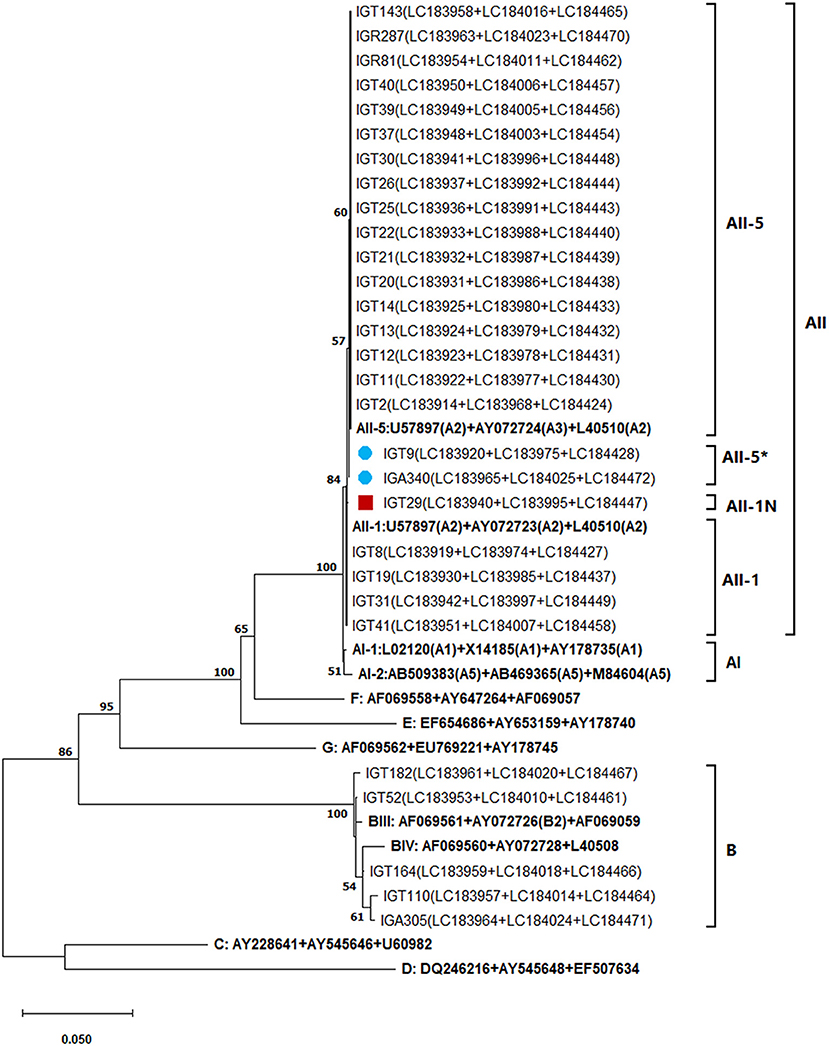
Figure 4. The phylogenetic analysis of the concatenated tpi, bg, and gdh nucleotide sequences of Giardia duodenalis using the maximum likelihood method (ML) and Tamura 3-parameter model (38) (T92 + G + I). The analysis involved 40 nucleotide sequences: 29 concatenated (tpi + bg + gdh) nucleotide sequences retrieved from this study compared with 11 known multilocus genotype reference sequences reported in previous studies (17, 40, 41) are indicated in bold. The red-filled square represents the new MLG of assemblage A reported in this study and the blue-filled circles indicate the one nucleotide substitution in A3 compared with the AII-5 MLG of assemblage A based on the modified numerical MLG reviewed in Cai et al. (4). The final dataset contained 1,395 positions. The percentage of trees in which the associated taxa clustered together (achieved from 1,000 replicates) is shown next to the branches, only bootstraps values >50% are demonstrated. A discrete Gamma distribution was used to model evolutionary rate differences among sites [5 categories (+G, parameter = 0.3229)]. The rate variation model allowed for some sites to be evolutionarily invariable [(+I), 37.78% sites]. The scale bar represents substitutions per nucleotide. Evolutionary analyses were conducted in MEGA X (39).
Discussion
The multilocus sequence typing (MLST) of cyst-positive Giardia isolates from infected individuals in Tehran was performed to classify assemblage and inter-and intra-assemblage genetic diversity of human G. duodenalis in Tehran, Iran, based on tpi, bg, and gdh genes. We identified one new MLG of assemblage A.
To the best of our knowledge, this is the first study conducted on G. duodenalis-infected individuals in Tehran, Iran, using MLST. However, the overwhelming majority of studies in Iran have reported the molecular characterization of G. duodenalis isolates based on the analysis of one locus (21–26, 42, 43) or two loci of gdh and tpi (44–46) or gdh and bg (47). The MLG data was reported for two Giardia isolates in the only multilocus analysis in southwestern Iran (48).
Multilocus sequencing results of tpi, bg, and gdh genes showed G. duodenalis-infected individuals in Tehran to be infected with assemblages A and B, agreeing with reports of human infection worldwide [reviewed in Feng and Xiao (3)]. Furthermore, assemblages A and B occurred at equal rates, similar to a Giardia MLG study performed in Malaysia (36). Although Ryan and Cacciò (7) reported that assemblage B is more prevalent than A in humans worldwide, the predominance of assemblage A was reported in previous studies in Iran (21, 22, 25, 47), Turkey (49), Iraq (50), Syria (51), Saudi-Arabia (52), Egypt (53), Thailand (54), Italy (34), the Czech Republic (55), and Ethiopia (56). The disparities might reflect geographical distribution, study populations and differing molecular tools and genes used, as the effect of loci was evident in the results of assemblage B in bg (~55%, 34/62) compared with the tpi (~46%, 25/54) and gdh (~46%, 24/52) genes in our study (Table 3).
Moreover, the amplification rate of these genetic loci differs, as about 60% of bg and tpi genes and 40–60% of gdh genes can be detected by most primers (3), possibly explaining the different rates of amplification of bg (100%), tpi (87.1%), and gdh (83.9%) observed in this study. These findings are in agreement with previous reports (3, 36). Although the majority of our isolates (77.4%) were consistent assemblage classification in three loci, 14.5% showed discordant assemblage typing, which was in agreement with previous studies on human and animal isolates (3, 36, 57, 58). As Cacciò and Ryan (16) suggested, this phenomenon might result from mixed infections in fecal samples or gene exchange between assemblages, also known as allelic sequence heterozygosity (ASH). A high level of ASH is reported in Giardia parasites, which have a tetraploid genome resulting from being a binucleated organism. Furthermore, the different levels of ASH have evaluated among G. duodenalis assemblages in which the ASH degree in assemblage B is about 10-fold higher than assemblage A isolates. On the other hand, it is usually challenging to distinguish between a high level of ASH or mixed infection when the DNA of cysts retrieved from fecal samples is typing (37).
Mixed assemblage infections have been commonly reported in humans and animals (17, 32, 58), and assemblage-specific PCR assays provide evidence that the prevalence of mixed assemblage infections is high in clinical specimens (32, 36). In this study, tpi assemblage-specific PCR assays showed a high prevalence (45.2%) of mixed infection of A and B assemblage in Tehran isolates, but less than reported in Malaysia (64%) (36), although greater than in Belgium (32.4%) (32) using the same primers. Co-infection by assemblage A and B has been previously reported in Iran based on PCR-RFLP of the gdh locus (21, 25, 43, 45).
Consistent with previously global reports (2, 4), we found that genotyping of assemblage A revealed low genetic polymorphism. Furthermore, AII was the only sub-assemblage identified with the greatest variation at the bg locus, followed by tpi and gdh, which could be reflecting the presence of double peak nucleotide positions in two assemblage A isolates at the bg locus. This finding contrasts with previous studies reporting the greatest variability in the tpi gene and lowest in the bg gene (15, 17). However, it agrees with Wegayehu et al. (59), who observed variation at the bg locus. AII, with the predominant subtype A2, is considered the most prevalent sub-assemblage in humans, whereas AI and AIII sub-assemblages are rarely reported [reviewed in (2, 3, 7)]. Identifying sub-assemblage AII, subtype A2 in the examined assemblage A isolates at the three loci, which is rarely found in other animals, suggests the potential of human-to-human transmission in the population studied. These results support previous findings of AII in the gdh locus that indicate potential anthroponotic transmission of Giardia in Tehran (21), Shiraz (22, 43), and Kashan (25). However, validating this hypothesis requires extensive molecular studies of Giardia isolates in animals and the environment, as well as considering the infection risk factors.
Higher genetic polymorphism of G. duodenalis in assemblage B than in A observed at the bg, tpi, and gdh loci in this study are consistent with previous reports (37, 41, 59–61). Furthermore, the higher genetic heterogeneity in those isolates representing the assemblage B of G. duodenalis was predominantly noticeable at the gdh locus (70.8%), followed by the tpi (40.0%) and bg (17.4%) loci which are in agreement with prior studies (17, 59). However, the finding contrasts with earlier observations (15), showing tpi to be the most polymorphic locus, with fewer polymorphisms observed in bg and gdh loci. Geurden et al. (32) reported high diversity at the bg locus and less at gdh and lowest at tpi, while Lecová et al. (55) reported the highest gdh followed by bg and tpi. These seemingly contradictory results can be potentially further explained by the differential selective pressures that ultimately determine the extent to which a given gene exhibits the genetic polymorphism. In contrast to assemblage A, subgrouping of assemblage B is not supported by phylogenetic analyses of nucleotide sequences of current genotyping loci (2, 3, 14). Sub-assemblage determination was not possible among all assemblage B isolates due to high nucleotide polymorphism with the heterogeneous nucleotide in the sequence, as has been reported (14, 41, 60). Inconsistency among the three markers in sub-assemblage B isolates was observed in this study as well as in earlier reports (32, 41, 55, 59, 62). This finding may reflect differences among the loci (59) or mixed infections with different sub-assemblages (37) or ASH (4, 7, 37).
To resolve the discrepancies among genetic markers, MLST of Giardia-positive samples was performed by combining the sequencing data of tpi, bg, and gdh loci, according to Cacciò et al. (17). As a result, the concatenated sequences of 24 A assemblages were classified into three MLGs: two (AII-1 and AII-5) MLGs frequently reported throughout the world (4, 17, 41, 55) and one novel (AII-1N) MLG, with one SNP in subtype A2 tpi locus, considering a single nucleotide mutation sufficient to designate a new subtype (16, 41, 63, 64). Therefore, since 2008, when Cacciò et al. (17) proposed a genotyping nomenclature system based on MLG analysis of the tpi, bg, and gdh loci, new MLGs have frequently been identified based on different combinations of tpi, bg, and gdh (4, 41, 55, 64). However, the presence of highly overlapping nucleotide peaks in the sequencing profiles and the broad genetic variability among and within the three target genetic loci made classifying assemblage B isolates in nominated MLGs impossible. It has been proposed that the high sequence variability and double peaks are due to the high degrees of ASH, genetic recombination through cryptic sex involving two nuclei of Giardia, true mixed infections, or a combination of those factors (4, 37, 59, 65). Therefore, as mentioned (3, 7, 37), MLST is useful for the typing of assemblage A of G. duodenalis, although MLGs grouping of assemblage B is more complex as a result of its high inter-and intra-sequence variability. In addition, as shown in the concatenated phylogenetic tree (Figure 4), the MLST is a practical tool for separating A and B isolates from each other and host-specific assemblages (C–G) and constructs host-specific clusters with high bootstrap support. Therefore, applying MLST allowed us to characterize G. duodenalis isolates circulating in Tehran and identify their genetic diversity.
The AII sub-assemblage is mainly reported in humans, considering that human and non-human primates are the predominant hosts of assemblage B and is much less frequent in wildlife and dogs (4, 7). Our identification of anthroponotic assemblages and sub-assemblages (B and AII) of G. duodenalis suggest that humans are likely a potential source of infection and person-to-person transmission probably occurs in Tehran. However, the main limitation of this hypothesis is the limited data on non-human giardiasis in Iran. To address this issue, comprehensive molecular studies to determine the genotype/subtype of Giardia infection of humans and companion and livestock animals that cohabit or occur in the same location, as well as environmental G. duodenalis isolates, are essential. Moreover, typing of assemblage B isolates should be performed separately using the MLST scheme with the newly identified best-performing genes developed in recent years to shed light on the transmission cycle of this mysterious parasite.
Conclusions
Assemblages A and B are equally represented in G. duodenalis-infected individuals in the current study. The multilocus sequence analysis reveals genetic diversity in both assemblages and novel MLG of assemblage A. However, the lack of a solid consensus around the nomenclature of genetic variants within the assemblage B of G. duodenalis at the sub-assemblage levels is challenging. The multilocus sequencing is useful for typing assemblage A and discriminating assemblages of G. duodenalis. Applying MLST also provides insight into the genetic diversity of G. duodenalis isolates. Our findings suggest that G. duodenalis is potentially transmitted via a person-to-person route in Tehran, although further MLST of Giardia isolates from humans, companion animals, livestock, and the environment is recommended to elucidate the mode of transmission.
Data availability statement
The datasets presented in this study can be found in online repositories. The names of the repository/repositories and accession number(s) can be found in the article/supplementary material.
Ethics statement
The studies involving human participants were reviewed and approved by the Ethics Committee of Iran University of Medical Sciences (IUMS) with the code number: IR.IUMS.REC.1394.25787. Written informed consent to participate in this study was provided by the participants' legal guardian/next of kin.
Author contributions
SH-H: methodology, validation, formal analysis, investigation, resources, data curation, visualization, and writing—original draft. ARM: methodology, validation, resources, and writing—review and editing. MM and NH: resources. SS-M: methodology, validation, formal analysis, and writing—review and editing. ER: conceptualization, methodology, validation, formal analysis, resources, data curation, writing—original draft, writing—review and editing, visualization, supervision, project administration, and funding acquisition. All authors contributed to the article and approved the submitted version.
Funding
This study was financially supported by the Iran University of Medical Sciences under grant number 94-01-30-25787 to ER. Research in the Laboratory of Mucosal Immunology was supported by a startup fund (20344-8015) from the Department of Biomedical Sciences, School of Medicine and Health Sciences, the University of North Dakota to SS-M, a Dean's Meritorious Pilot Grant, School of Medicine and Health Sciences, the University of North Dakota to SS-M, and by NIH/NIGMSP20GM113123 to SS-M.
Acknowledgments
We are grateful to the patients and laboratory staff for their help in providing samples, especially the staff of Milad Hospital, Tehran. We are indebted to Dr. Alan Pike and Kathleen Hills, from the Lucidus Consultancy for a critical review, helpful advice, and their kindly editing comments to improve this paper. This work represents the MSc dissertation of SH-H. Part of this work was presented by ER at the 27th European Congress of Clinical Microbiology and Infectious Diseases; it is available at ESCMID; on 25 April 2017 (https://www.escmid.org/escmid_publications/escmid_elibrary/).
Conflict of interest
The authors declare that the research was conducted in the absence of any commercial or financial relationships that could be construed as a potential conflict of interest.
Publisher's note
All claims expressed in this article are solely those of the authors and do not necessarily represent those of their affiliated organizations, or those of the publisher, the editors and the reviewers. Any product that may be evaluated in this article, or claim that may be made by its manufacturer, is not guaranteed or endorsed by the publisher.
References
1. Solaymani-Mohammadi S, Singer SM. Giardia duodenalis: the double-edged sword of immune responses in giardiasis. Exp Parasitol. (2010) 126:292–7. doi: 10.1016/j.exppara.2010.06.014
2. Ryan UM, Feng Y, Fayer R, Xiao L. Taxonomy and molecular epidemiology of Cryptosporidium and Giardia – a 50 year perspective (1971–2021). Int J Parasitol. (2021) 51:1099–119. doi: 10.1016/j.ijpara.2021.08.007
3. Feng Y, Xiao L. Zoonotic potential and molecular epidemiology of Giardia species and giardiasis. Clin Microbiol Rev. (2011) 24:110–40. doi: 10.1128/CMR.00033-10
4. Cai W, Ryan U, Xiao L, Feng Y. Zoonotic giardiasis: an update. Parasitol Res. (2021) 120:4199–218. doi: 10.1007/s00436-021-07325-2
5. Capewell P, Krumrie S, Katzer F, Alexander CL, Weir W. Molecular epidemiology of Giardia infections in the genomic era. Trends Parasitol. (2021) 37:142–53. doi: 10.1016/j.pt.2020.09.013
6. Solaymani-Mohammadi S, Singer SM. Host immunity and pathogen strain contribute to intestinal disaccharidase impairment following gut infection. J Immunol. (2011) 187:3769–75. doi: 10.4049/jimmunol.1100606
7. Ryan U, Cacciò SM. Zoonotic potential of Giardia. Int J Parasitol. (2013) 43:943–56. doi: 10.1016/j.ijpara.2013.06.001
8. Thompson RCA, Ash A. Molecular epidemiology of Giardia and Cryptosporidium infections. Infect Genet Evol. (2016) 40:315–23. doi: 10.1016/j.meegid.2015.09.028
9. Abdel-Moein KA, Saeed H. The zoonotic potential of Giardia intestinalis assemblage E in rural settings. Parasitol Res. (2016) 115:3197–202. doi: 10.1007/s00436-016-5081-7
10. Fantinatti M, Bello AR, Fernandes O, Da-Cruz AM. Identification of Giardia lamblia assemblage E in humans points to a new anthropozoonotic cycle. J Infect Dis. (2016) 214:1256–9. doi: 10.1093/infdis/jiw361
11. Garcia-R JC, Ogbuigwe P, Pita AB, Velathanthiri N, Knox MA, et al. First report of novel assemblages and mixed infections of Giardia duodenalis in human isolates from New Zealand. Acta Trop. (2021) 220:105969. doi: 10.1016/j.actatropica.2021.105969
12. Cacciò SM, Lalle M, Svärd SG. Host specificity in the Giardia duodenalis species complex. Infect Genet Evol. (2018) 66:335–45. doi: 10.1016/j.meegid.2017.12.001
13. Costache C, Kalmár Z, Colosi HA, Baciu AM, Opriş RV, Györke A, et al. First multilocus sequence typing (MLST) of Giardia duodenalis isolates from humans in Romania. Parasit Vect. (2020) 13:387. doi: 10.1186/s13071-020-04248-2
14. Seabolt MH, Konstantinidis KT, Roellig DM, Björkroth J. Hidden diversity within common protozoan parasites as revealed by a novel genomotyping scheme. Appl Environ Microbiol. (2021) 87:e02275–e02220. doi: 10.1128/AEM.02275-20
15. Wielinga CM, Thompson RCA. Comparative evaluation of Giardia duodenalis sequence data. Parasitology. (2007) 134:1795–821. doi: 10.1017/S0031182007003071
16. Cacciò SM, Ryan U. Molecular epidemiology of giardiasis. Mol Biochem Parasitol. (2008) 160:75–80. doi: 10.1016/j.molbiopara.2008.04.006
17. Cacciò SM, Beck R, Lalle M, Marinculic A, Pozio E. Multilocus genotyping of Giardia duodenalis reveals striking differences between assemblages A and B. Int J Parasitol. (2008) 38:1523–1. doi: 10.1016/j.ijpara.2008.04.008
18. Hemmati N, Razmjou E, Hashemi-Hafshejani S, Motevalian A, Akhlaghi L, Meamar AR. Prevalence and risk factors of human intestinal parasites in Roudehen, Tehran Province, Iran. Iran J Parasitol. (2017) 12:364–73.
19. Mohebali M, Keshavarz H, Abbaszadeh Afshar MJ, Hanafi-Bojd AA, Hassanpour G. Spatial distribution of common pathogenic human intestinal protozoa in Iran: a systematic review. Iran J Public Health. (2021) 50:69–82. doi: 10.18502/ijph.v50i1.5073
20. Teimouri A, Keshavarz H, Mohtasebi S, Goudarzi F, Mikaeili F, Borjian A, et al. Intestinal parasites among food handlers in Iran: a systematic review and meta-analysis. Food Microbiol. (2021) 95:103703. doi: 10.1016/j.fm.2020.103703
21. Babaei Z, Oormazdi H, Akhlaghi L, Rezaie S, Razmjou E, Soltani-Arabshahi SK, et al. Molecular characterization of the Iranian isolates of Giardia lamblia: application of the glutamate dehydrogenase gene. Iran J Public Health. (2008) 37:75–82.
22. Rayani M, Unyah NZ, Hatam G. Molecular identification of Giardia duodenalis isolates from Fars province, Iran. Iran J Parasitol. (2014) 9:70–8.
23. Tappeh KH, Manafi G, Asgharzadeh M, Manafi F. Incidence of Giardia lamblia subspecies by PCR-RFLP in stool specimens of hospitalized children at Urmia Mutahhari hospital, West Azerbaijan Province, Iran. Iran J Parasitol. (2014) 9:541–7.
24. Bahrami F, Zamini G, Haghighi A, Khademerfan M. Detection and molecular identification of human Giardia isolates in the West of Iran. Biomed Res. (2017) 28:5687–92.
25. Hooshyar H, Ghafarinasab S, Arbabi M, Delavari M, Rasti S. Genetic variation of Giardia lamblia isolates from food-handlers in Kashan, central Iran. Iran J Parasitol. (2017) 12:83–9.
26. Kasaei R, Carmena D, Jelowdar A, Beiromvand M. Molecular genotyping of Giardia duodenalis in children from Behbahan, southwestern Iran. Parasitol Res. (2018) 117:1425–31. doi: 10.1007/s00436-018-5826-6
27. Coklin T, Farber J, Parrington L, Dixon B. Prevalence and molecular characterization of Giardia duodenalis and Cryptosporidium spp. in dairy cattle in Ontario, Canada. Vet Parasitol. (2007) 150:297–305. doi: 10.1016/j.vetpar.2007.09.014
28. Babaei Z, Oormazdi H, Rezaie S, Rezaeian M, Razmjou E. Giardia intestinalis: DNA extraction approaches to improve PCR results. Exp Parasitol. (2011) 128:159–62. doi: 10.1016/j.exppara.2011.02.001
29. Verweij JJ, Pit DSS, van Lieshout L, Baeta SM, Dery GD, Gasser RB, et al. Determining the prevalence of Oesophagostomum bifurcum and Necator americanus infections using specific PCR amplification of DNA from faecal samples. Trop Med Int Health. (2001) 6:726–31. doi: 10.1046/j.1365-3156.2001.00770.x
30. Sulaiman IM, Fayer R, Bern C, Gilman RH, Trout JM, Schantz PM, et al. Triosephosphate isomerase gene characterization and potential zoonotic transmission of Giardia duodenalis. Emerg Infect Dis. (2003) 9:1444–52. doi: 10.3201/eid0911.030084
31. Geurden T, Geldhof P, Levecke B, Martens C, Berkvens D, Casaert S, et al. Mixed Giardia duodenalis assemblage A and E infections in calves. Int J Parasitol. (2008) 38:259–64. doi: 10.1016/j.ijpara.2007.07.016
32. Geurden T, Levecke B, Cacciò SM, Visser A, De Groote G, Casaert S, et al. Multilocus genotyping of Cryptosporidium and Giardia in non-outbreak related cases of diarrhoea in human patients in Belgium. Parasitology. (2009) 136:1161–8. doi: 10.1017/S0031182009990436
33. Read CM, Monis PT, Andrew Thompson RC. Discrimination of all genotypes of Giardia duodenalis at the glutamate dehydrogenase locus using PCR-RFLP. Infect Genet Evol. (2004) 4:125–30. doi: 10.1016/j.meegid.2004.02.001
34. Cacciò SM, De Giacomo M, Pozio E. Sequence analysis of the β-giardin gene and development of a polymerase chain reaction–restriction fragment length polymorphism assay to genotype Giardia duodenalis cysts from human faecal samples. Int J Parasitol. (2002) 32:1023–30. doi: 10.1016/S0020-7519(02)00068-1
35. Lalle M, Pozio E, Capelli G, Bruschi F, Crotti D, Cacciò SM. Genetic heterogeneity at the (-giardin locus among human and animal isolates of Giardia duodenalis and identification of potentially zoonotic subgenotypes. Int J Parasitol. (2005) 35:207–13. doi: 10.1016/j.ijpara.2004.10.022
36. Huey CS, Mahdy MAK, Al-Mekhlafi HM, Nasr NA, Lim YAL, Mahmud R, et al. Multilocus genotyping of Giardia duodenalis in Malaysia. Infect Genet Evol. (2013) 17:269–76. doi: 10.1016/j.meegid.2013.04.013
37. Woschke A, Faber M, Stark K, Holtfreter M, Mockenhaupt F, Richter J, et al. Suitability of current typing procedures to identify epidemiologically linked human Giardia duodenalis isolates. PLoS Negl Trop Dis. (2021) 15:e0009277. doi: 10.1371/journal.pntd.0009277
38. Tamura K. Estimation of the number of nucleotide substitutions when there are strong transition-transversion and G+C-content biases. Mol Biol Evol. (1992) 9:678–87.
39. Kumar S, Stecher G, Li M, Knyaz C, Tamura K. MEGA X: Molecular evolutionary genetics analysis across computing platforms. Mol Biol Evol. (2018) 35:1547–9. doi: 10.1093/molbev/msy096
40. Minetti C, Lamden K, Durband C, Cheesbrough J, Fox A, Wastling JM. Determination of Giardia duodenalis assemblages and multi-locus genotypes in patients with sporadic giardiasis from England. Parasit Vect. (2015) 8:444. doi: 10.1186/s13071-015-1059-z
41. Faria CP, Zanini GM, Dias GS, da Silva S, Sousa MDC. New multilocus genotypes of Giardia lamblia human isolates. Infect Genet Evol. (2017) 54(Suppl. C):128–37. doi: 10.1016/j.meegid.2017.06.028
42. Manouchehri Naeini K, Hosseini SA, Gholipour A, Babaei Z, Taghipoor S. Genotyping of Giardia duodenalis isolates in individuals with and without chronic diarrhea using polymerase chain reaction. J Mazand Univ Med Sci. (2012) 22:39–46.
43. Sarkari B, Ashrafmansori A, Hatam G, Motazedian M, Asgari Q, Mohammadpour I. Genotyping of Giardia lamblia isolates from human in southern Iran. Trop Biomed. (2012) 29:366–71.
44. Babaei Z, Malihi N, Zia-Ali N, Sharifi I, Mohammadi MA, Kagnoff MF, et al. Adaptive immune response in symptomatic and asymptomatic enteric protozoal infection: evidence for a determining role of parasite genetic heterogeneity in host immunity to human giardiasis. Microbes Infect. (2016) 18:687–95. doi: 10.1016/j.micinf.2016.06.009
45. Nooshadokht M, Kalantari-Khandani B, Sharifi I, Kamyabi H, Liyanage NPM, Lagenaur LA, et al. Stool antigen immunodetection for diagnosis of Giardia duodenalis infection in human subjects with HIV and cancer. J Microbiol Methods. (2017) 141:35–41. doi: 10.1016/j.mimet.2017.07.004
46. Kashinahanji M, Haghighi A, Bahrami F, Fallah M, Saidijam M, Matini M, et al. Giardia lamblia assemblages A and B isolated from symptomatic and asymptomatic persons in Hamadan, west of Iran. J Parasit Dis. (2019) 43:616–23. doi: 10.1007/s12639-019-01139-x
47. Mirrezaie E, Beiromvand M, Tavalla M, Teimoori A, Mirzavand S. Molecular genotyping of Giardia duodenalis in humans in the Andimeshk county, southwestern Iran. Acta Parasitol. (2019) 64:376–83. doi: 10.2478/s11686-019-00051-x
48. Rafiei A, Baghlaninezhad R, Köster PC, Bailo B, Hernández de Mingo M, Carmena D, et al. Multilocus genotyping of Giardia duodenalis in Southwestern Iran A community survey. PLoS ONE. (2020) 15:e0228317. doi: 10.1371/journal.pone.0228317
49. Tamer GS, Kasap M, Er DK. Genotyping and phylogenetic analysis of Giardia duodenalis isolates from Turkish children. Med Sci Monit. (2015) 21:526–32. doi: 10.12659/MSM.892318
50. Turki NM, Mallah MO, Kremsh YD. Iraqi genotyping of Giardia lambila (A, B, E, F) in human stool in AL-muthanna province–Iraq. Int J Adv Res. (2015) 3:757–71.
51. Skhal D, Aboualchamat G, Al Mariri A, Al Nahhas S. Prevalence of Giardia duodenalis assemblages and sub-assemblages in symptomatic patients from Damascus city and its suburbs. Infect Genet Evol. (2017) 47:155–60. doi: 10.1016/j.meegid.2016.11.030
52. Al-Mohammed HI. Genotypes of Giardia intestinalis clinical isolates of gastrointestinal symptomatic and asymptomatic Saudi children. Parasitol Res. (2011) 108:1375–81. doi: 10.1007/s00436-010-2033-5
53. Helmy MMF, Abdel-Fattah HS, Rashed L. Real-time PCR/RFLP assay to detect Giardia intestinalis genotypes in human isolates with diarrhea in Egypt. J Parasitol. (2009) 95:1000–4. doi: 10.1645/GE-1670.1
54. Traub RJ, Inpankaew T, Reid SA, Sutthikornchai C, Sukthana Y, Robertson ID, et al. Transmission cycles of Giardia duodenalis in dogs and humans in temple communities in Bangkok–A critical evaluation of its prevalence using three diagnostic tests in the field in the absence of a gold standard. Acta Trop. (2009) 111:125–32. doi: 10.1016/j.actatropica.2009.03.006
55. Lecová L, Weisz F, Tumová P, Tolarová V, Nohýnková E. The first multilocus genotype analysis of Giardia intestinalis in humans in the Czech Republic. Parasitology. (2018) 145:1577–87. doi: 10.1017/S0031182018000409
56. Gelanew T, Lalle M, Hailu A, Pozio E, Cacciò SM. Molecular characterization of human isolates of Giardia duodenalis from Ethiopia. Acta Trop. (2007) 102:92–9. doi: 10.1016/j.actatropica.2007.04.003
57. Fahmy HM, El-Serougi AO, El Deeb HK, Hussein HM, Abou-Seri HM, Klotz C, et al. Giardia duodenalis assemblages in Egyptian children with diarrhea. Eur J Clin Microbiol Infect Dis. (2015) 34:1573–81. doi: 10.1007/s10096-015-2389-7
58. Wang H, Qi M, Zhang K, Li J, Huang J, Ning C, et al. Prevalence and genotyping of Giardia duodenalis isolated from sheep in Henan Province, central China. Infect Genet Evol. (2016) 39:330–5. doi: 10.1016/j.meegid.2016.02.006
59. Wegayehu T, Karim MR, Li J, Adamu H, Erko B, Zhang L, et al. Multilocus genotyping of Giardia duodenalis isolates from children in Oromia Special Zone, central Ethiopia. BMC Microbiol. (2016) 16:1–10. doi: 10.1186/s12866-016-0706-7
60. Brynildsrud O, Tysnes KR, Robertson LJ, Debenham JJ. Giardia duodenalis in primates: classification and host specificity based on phylogenetic analysis of sequence data. Zoonoses Public Health. (2018) 65:637–47. doi: 10.1111/zph.12470
61. Messa AJr, Köster PC, Garrine M, Gilchrist C, Bartelt LA, Nhampossa T, et al. Molecular diversity of Giardia duodenalis in children under 5 years from the Manhiça district, Southern Mozambique enrolled in a matched case-control study on the aetiology of diarrhoea. PLoS Negl Trop Dis. (2021) 15:e0008987. doi: 10.1371/journal.pntd.0008987
62. Oliveira-Arbex AP, David EB, Oliveira-Sequeira TCG, Bittencourt GN, Guimarães S. Genotyping of Giardia duodenalis isolates in asymptomatic children attending daycare centre: evidence of high risk for anthroponotic transmission. Epidemiol Infect. (2016) 144:1418–28. doi: 10.1017/S0950268815002514
63. Sprong H Cacciò SM van der Giessen JWB on on behalf of the Znap. Identification of zoonotic genotypes of Giardia duodenalis. PLoS Negl Trop Dis. (2009) 3:e558. doi: 10.1371/journal.pntd.0000558
64. Broglia A, Weitzel T, Harms G, Cacció SM, Nöckler K. Molecular typing of Giardia duodenalis isolates from German travellers. Parasitol Res. (2013) 112:3449–56. doi: 10.1007/s00436-013-3524-y
Keywords: Giardia duodenalis, multilocus sequence typing, triosephosphate isomerase (tpi), β-giardin (bg), glutamate dehydrogenase (gdh), Tehran, Iran
Citation: Hashemi-Hafshejani S, Meamar AR, Moradi M, Hemmati N, Solaymani-Mohammadi S and Razmjou E (2022) Multilocus sequence typing of Giardia duodenalis genotypes circulating in humans in a major metropolitan area. Front. Med. 9:976956. doi: 10.3389/fmed.2022.976956
Received: 24 June 2022; Accepted: 20 September 2022;
Published: 05 October 2022.
Edited by:
Ana Afonso, Universidade NOVA de Lisboa, PortugalReviewed by:
Jacek Sroka, National Veterinary Research Institute (NVRI), PolandTapan Bhattacharyya, University of London, United Kingdom
Panagiotis Karanis, University of Nicosia, Cyprus
Fanfan Shu, South China Agricultural University, China
Copyright © 2022 Hashemi-Hafshejani, Meamar, Moradi, Hemmati, Solaymani-Mohammadi and Razmjou. This is an open-access article distributed under the terms of the Creative Commons Attribution License (CC BY). The use, distribution or reproduction in other forums is permitted, provided the original author(s) and the copyright owner(s) are credited and that the original publication in this journal is cited, in accordance with accepted academic practice. No use, distribution or reproduction is permitted which does not comply with these terms.
*Correspondence: Elham Razmjou, razmjou.e@iums.ac.ir