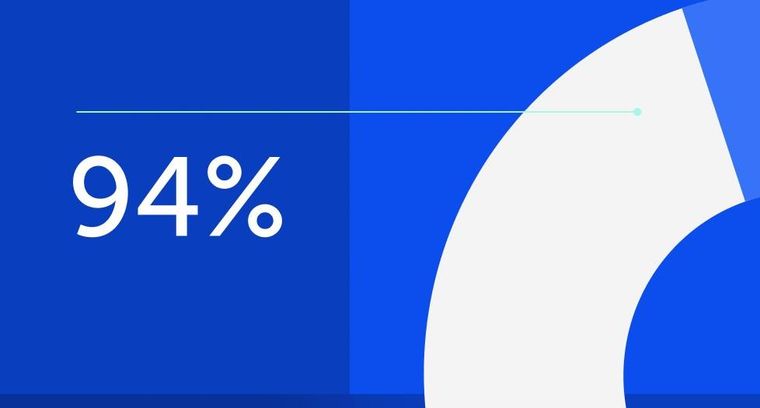
94% of researchers rate our articles as excellent or good
Learn more about the work of our research integrity team to safeguard the quality of each article we publish.
Find out more
SYSTEMATIC REVIEW article
Front. Med., 06 October 2022
Sec. Infectious Diseases: Pathogenesis and Therapy
Volume 9 - 2022 | https://doi.org/10.3389/fmed.2022.975666
Background: Despite the surge in the number of antibiotics used to treat preclinical osteomyelitis (OM), their efficacy remains inadequately assessed.
Objective: To establish network comparisons on the efficacy of antibiotic regimens on OM in animal studies.
Methods: PubMed, Embase, Web of Science, and The Cochrane Library were searched from inception to March 2022 for relevant articles. Odds ratios (ORs) were generated for dichotomous variants, and the standard mean difference (SMD) was calculated for constant variables. The predominant outcomes were the effective rate of sterility, also known as sterility rates, as well as the bacterial counts at the end of the experiments and antibiotic concentrations in serum or bone. All the network meta-analyses were performed using STATA MP 16.0. This study was registered in the International Prospective Register of Systematic Reviews (PROSPERO; no. CRD42022316544).
Results: A total of 28 eligible studies with 1,488 animals were included for data analysis, including 13 antibiotic regimens. Regarding the effective rate of sterility, glycopeptides (GLY), linezolid (LIN), rifampicin (RIF)+β-Lactam, and β-Lactam showed significant efficacy compared with placebo (OR ranging from 0.01 to 0.08). For radiological grade, only RIF+GLY (SMD: −5.92, 95%CI: −11.65 to −0.19) showed significant efficacy compared with placebo. As for reducing bacteria count, fosfomycin (FOS), tigecycline (TIG), GLY, LIN, RIF, RIF+β-Lactam, RIF+GLY, aminoglycosides (AMI), and clindamycin (CLI) showed significant efficacy compared with placebo (SMD ranging from −6.32 to −2.62). Moreover, the bone concentrations of GLY were higher 1 h after administration and the higher blood concentrations were higher after 1 h and 4 h compared with the other antibiotics.
Conclusion: Multiple antibiotic regimens showed significant efficacy in animals with OM, including increasing effective rates of sterility, reducing bacterial counts, and lowering radiological scores. Among them, RIF+GLY was the most promising treatment regimen owing to its optimal efficacy. Based on the preclinical studies included in our meta-analysis, head-to-head clinical randomized controlled trials are required to confirm these findings in humans.
Osteomyelitis (OM) is a disease of progressive bone destruction caused by infectious microorganisms and is an intractable complication observed following orthopedic surgery, trauma, or arthroplasty (1). Staphylococcus aureus (S. aureus) is the most common disease-causing pathogen, accounting for 65–80% of the microbes found in patients (2). With more advanced detection means, the diagnostic rate of OM has increased worldwide in recent years. Notwithstanding, the mortality rates of adult spinal OM was remain as high as 6% in developed countries (3).
In general, the standard treatment procedures for OM include thorough surgical debridement and systemic antibiotic administration. Although surgical treatment is indispensable, the antibiotics used to fight infections and inhibit biofilm should be chosen carefully because they can have a significant impact on the outcomes.
Currently, for OM with S. aureus including Methicillin-resistant S. aureus (MRSA) and Methicillin-sensitive S. aureus (MSSA) infection, long-term use of sensitive glycopeptides (GLY) antibiotics such as vancomycin and teicoplanin is recommended (1). In addition to the classical antibiotic regimens mentioned above, broad-spectrum antibiotics are used as a monotherapy or as an adjuvant medication for the treatment of OM, such as cephalosporins, aminoglycosides (AMI), penicillins, carbapenems, sulfonamides, and quinolones (QUI) with satisfactory efficacy. The combination of antibiotics for the treatment of OM has become a research hotspot in recent years. For instance, rifampicin (RIF) in combination with QUI has been shown to be effective in implant-associated OM in several studies, given its good permeability and bioavailability (4, 5). Other combination regimens, including RIF combined with GLY or β-Lactam antibiotics, have also been shown to achieve satisfactory results (6, 7).
With so many regimen combinations to use, surgeons face the issue of being overwhelmed with the specific regimen choice. Hence, direct comparisons are required to quantify efficacy. However, after performing a thorough search of the literature in both Chinese and English databases, it was found that the number of studies was lower than the number of interventions possible, thus it was determined that the literature on clinical trials was inadequate for performing network meta-analysis. Consequently, we adopted animal studies to compare efficacy instead: A network meta-analysis on the effects of antibiotics on OM animal models was, therefore, performed. A total of 13 antibiotic regimens were compared: RIF, tigecycline (TIG), linezolid (LIN), fosfomycin (FOS), azithromycin (AZI), clindamycin (CLI), trimethoprim (TRI), GLY, AMI, β-Lactam, QUI, RIF+β-Lactam, and RIF+GLY.
This study was performed in accordance with the PRISMA guidelines (see Supplementary Table 1) and the protocol for this meta-analysis can be found on the PROSPERO website (www.crd.york.ac.uk/PROSPERO/; no. CRD42022316544).
PubMed, Embase, Web of Science, and The Cochrane library were searched from inception to March 2022 for relevant articles using the corresponding Medical Subject Headings (MeSH) “osteomyelitis,” “chronic osteomyelitis,” “anti-bacterial agents,” “antibiotic agent,” “animal,” and “animal experimentation” with Boolean modifiers as appropriate. The in-depth search strategy for the four electronic databases is shown in Supplementary Table 2.
1. All subjects were OM animal models with no limits on species, age, sex, or weight;
2. The antibiotic regimens included any type of antibiotic regimen or combination of two antibiotics;
3. The study included a negative control group;
4. The outcomes compared the effective rates of sterility, bacterial counts, radiological grades, and antibiotic concentrations in serum or bone.
1. Clinical research and non-controlled studies using animals;
2. Secondary literature (e.g., literature comment or review);
3. Studies limited to in vitro experiments;
4. Studies assessing interventions other than antibiotics (e.g., antibiotic carriers or scaffolds).
All identified studies were independently screened by two researchers (X. Shi and Y. Wu) based on the titles and abstracts. Subsequently, they prudently and independently performed the extracted data based on the pre-established data extraction checklist. The extracted data were finally collated into a table and included: (1) basic characteristics of eligibility studies, including the author's name, year of publication, and region of study; (2) basic information on experimental animals, weights, sample sizes, modeling methods, interventions, total therapy time, and other adverse events; (3) outcome indicators: effective rates of sterility, bacterial counts, radiological scores of bone, and antibiotic concentrations in serum or bone. The means and standard deviations (SD) or standard error means (SEM) were extracted from both the antibiotic regimens and control groups as outcome indicators. Any discrepancy was adjudicated by a senior investigator (Y. Xu).
The Systematic Review Center for Laboratory animal Experimentation Risk of Bias (SYRCLE's RoB) tool for animal studies was utilized by two well-trained investigators (X. Shi and Y. Wu) independently to assess the quality of enrolled studies (8). Any discrepancy was adjudicated by a senior investigator (Y. Xu). This SYRCLE's RoB tool is based on the Cochrane Collaboration risk of bias tool (9) and has been adjusted for the risk of bias assessment in animal studies with a total of 10 entries related to six types of bias (selection bias, performance bias, attrition bias, follow-up bias, reporting bias, and other biases). Moreover, specific evaluation criteria were as follows: a “yes” judgment to the assessment question indicates a low risk of bias and a “no” indicated a high risk of bias. When there were insufficient details based on reports to properly assess the risk of bias, “unclear” was assigned to indicate this.
The primary outcomes were the effective rates of sterility (sterility rates after treatment), radiological grades, and bacteria counts.
The secondary outcomes were antibiotic concentrations in serum or bone.
Odds ratios (ORs) were generated for dichotomous variants, while the standard mean difference (SMD) was calculated for constant variables regardless of the type of meta-analysis. P < 0.05 was considered statistically significant with a 95% confidence interval (CI). For network meta-analysis, the node analysis model was used to check for inconsistencies. P < 0.05 indicated that there was an inconsistency between direct comparison and indirect comparison. Surface under the cumulative ranking curve (SUCRA) values were calculated and matrices were generated; a higher value for the former indicated a higher possibility of being the best treatment. Random effect models were used for network meta-analysis. Moreover, matrices were implemented to detect whether the difference between any one pair with corresponding SUCRAs reached significance. To reinforce the results, overall and loops inconsistency tests and consistency tests were performed in each outcome, and the small sample effect was explored using a network funnel plot. All the network meta-analyses were performed using STATA MP 16.0.
In total, 28 controlled studies consisting of a total of 1488 animals were included, and a total of 13 antibiotic regimens were used. The flow diagram of the selection criteria is shown in Figure 1. Of the included studies, experimental animal species included New Zealand White rabbits (11–23), Sprague-Dawley (SD) rats (24–29), Wistar rats (30–35), Madorin rats (36, 37), or RAR rats (38). The animal infection models included S. aureus, MRSA, Methicillin-resistant Staphylococcus epidermidis (MRSE), Klebsiella pneumoniae, or Morganella-infected animals. The methods of OM induction in six studies were implant-based models, and the remaining studies were all post-traumatic models. The duration of administration ranged from 4 to 28 days. In addition, antibiotic regimens were administrated intraperitoneally, subcutaneously, intravenously, intramuscularly, or orally during the treatment phase. The basic characteristics of the animal studies included are shown in Supplementary Table S1.
Figure 1. PRISMA flow diagram of the study selection process (10).
Of the 28 controlled studies included, no studies reported detailed randomization grouping methods and generation processes; 18 studies reported similar baseline characteristics of animals; three studies described in detail that experimental animals were placed in a randomized housing environment. Only one study described a blinded assessment of outcomes. In five studies, animal deaths were reported after antibiotic treatment. All studies reported expected results. The results of the methodological quality assessment are shown in Figures 2A,B.
Figure 2. Risk of bias (A) graph showing the risk of bias; (B) individual risk of bias for each of the included animal studies.
A total of 12 out of 28 studies were included for this endpoint. Network graphs of each pairwise comparison of all regimens on effective rates of sterility are shown in Figure 3A. RIF and GLY had the highest number of studies. From the matrix (Table 1), out of 10 interventions, only GLY, LIN, RIF+β-Lactam, and β-Lactam showed significant efficacy compared with the placebo, whereas the rest were inefficient. Additionally, RIF+β-Lactam was equivalent to GLY, LIN, and β-Lactam. The network funnel plot suggested that the small sample effect existed in the comparison between AMI and GLY on total effective rates of sterility (Figure 3B).
Figure 3. Network meta-analysis of the total effective rates of sterility (A) network graph; (B) the network funnel plot.
Table 1. Matrix of pairwise comparations of regimens on effective rates of sterility [shown as odds ratios (ORs) and 95% confidence intervals (CIs)].
Of the 28 studies, eight were included for this endpoint. A network graph of each of the pairwise comparisons of all the regimens on effective rates of sterility is shown in Figure 4A. RIF and GLY had the highest number of studies. According to the matrix (Table 2), out of 10 interventions, only RIF+GLY showed significant efficacy compared to placebo, while the others were ineffective. The network funnel plot suggested that the small sample effect existed in the comparison between RIF+GLY and the placebo on radiological grades (Figure 4B).
Figure 4. Network meta-analysis of radiological grades (A) network graph; (B) the network funnel plot.
Table 2. Matrix of pairwise comparations of regimens on radiological grades [shown as SMD (Standardized mean difference) and 95% confidence intervals (CIs)].
Of the 28 studies, 14 were included for this endpoint. A network graph of each of the pairwise comparisons of all regimens on bacterial counts is shown in Figure 5A. GLY and RIF+GLY had the highest number of studies. From the matrix (Table 3), nine of the 13 interventions showed significant efficacy compared with the placebo, including AMI, CLI, FOS, GLY, LIN, RIF, RIF+GLY, RIF+β-Lactam, and TIG. AZI was significantly inferior to FOS, GLY, LIN, RIF, and TIG. FOS was significantly superior to AMI, AZI, CLI, QUI, RIF+GLY, and β-Lactam. AMI was significantly superior to RIF+β-Lactam and LIN. The network funnel plot suggested that the small sample effect existed in the comparison between FOS and the placebo on bacteria counts (Figure 5B).
Table 3. Matrix of pairwise comparations of regimens on bacterial counts [shown as SMD (Standardized mean difference) and 95% confidence intervals (CIs)].
Antibiotic concentrations in serum 1 hour after administration (μg/ml): a network graph of each pairwise comparison of all the regimens is shown in Supplementary Figure S1A. GLY and AMI had the highest number of studies. From the matrix (Supplementary Table S2), RIF was significantly lower to GLY (SMD: −6.51, 95%CI: −9.28 to −3.75), AMI (SMD: −3.56, 95%CI: −5.27 to −1.84), and β-Lactam (SMD: −3.48, 95%CI: −5.20 to −1.75). The network funnel plot suggested that the small sample effect existed in the comparison between GLY and RIF on the antibiotic concentration of serum 1 h after administration (Supplementary Figure S1B).
Antibiotic concentrations in the serum 4 h after administration (μg/ml): a network graph of each pairwise comparison of all the regimens is shown in Supplementary Figure S2A. RIF and GLY had the highest number of studies. From the matrix (Supplementary Table S3), GLY was significantly higher than RIF (SMD: 3.20, 95%CI: 0.52 to 5.89), AMI (SMD: 5.90, 95%CI: 2.46 to 9.17), TRI (SMD: 5.80, 95%CI: 2.01 to 9.58), and β-Lactam (SMD: 6.64, 95%CI: 3.36 to 9.92). The network funnel plot suggested that the small sample effect existed in the comparison between RIF and β-Lactam on the antibiotic concentration of serum after 4 h after administration (Supplementary Figure S2B).
Antibiotic concentrations in the bone 1 h after administration (μg/g): a network graph of each pairwise comparison of all the regimens is shown in Supplementary Figure S3A. GLY and AMI had the highest number of studies. From the matrix (Supplementary Table S4), GLY was significantly higher than β-Lactam (SMD: 4.40, 95%CI: 0.32 to 8.47), RIF (SMD: 5.28, 95%CI: 1.89 to 8.67), and TRI (SMD: 6.28, 95%CI: 1.62 to 10.95). The network funnel plot suggested that the small sample effect existed in the comparison between GLY and RIF on the antibiotic concentration of bone after 1 h after administration (Supplementary Figure S3B).
Antibiotic concentrations in the bone 4 h after administration (μg/g): a network graph of each pairwise comparison of all the regimens is shown in Supplementary Figure S4A. RIF and GLY had the highest number of studies. From the matrix (Supplementary Table S5), we conducted pairwise comparisons among five antibiotic regimens. However, there was no significant difference between these regimens. The network funnel plot suggested that the small sample effect existed in the comparison between RIF and TRI on the antibiotic concentrations in the bone 4 h after administration (Supplementary Figure S4B).
To the best of our knowledge, this is the first network meta-analysis comparing the efficacy of antibiotic regimens on OM in preclinical animal models. We found that the combination of RIF+GLY was more effective than the placebo in the analysis of radiological grades and bacteria counts after treatment and a similar animal study on OM came to the same conclusion (39). There was only one previous meta-analysis with antibiotics for OM (40), and their analysis showed no difference in the efficacy of quinolones and β-Lactams in treating patients with OM, results similar to those obtained in our network meta-analysis.
Interestingly, we analyzed blood and bone concentrations after antibiotic administration and found that GLY bone concentrations were higher 1 h after administration and blood concentrations were higher 1 and 4 h after administration compared with the other antibiotics. Current guidelines recommend serum vancomycin concentrations of 15–20 μg/ml in adult patients with S. aureus infection in the clinic (41), and our analysis described serum concentrations of GLY in OM models, in which the mean serum concentration at 4 h was 18.7 ± 5 μg/ml, approaching the guideline recommendations. However, due to the significant difference in metabolic rates between rats and humans (42, 43), we should be very careful in extrapolating results from animal models to humans. Additionally, the local concentration of antibiotics in the tissue must exceed the MIC of the bacteria to eradicate the infection (44). In our included studies, MIC-matched 18–24 h blood and bone concentrations were not provided, and therefore the exact efficacy of the drug could not be assessed.
The causative agents of OM include several types of bacteria, including Gram-positive, Gram-negative, and multi-species mixed infections. In general, the antibiotic must cross the outer membrane and cytoplasmic membrane of the bacteria before entering the cytoplasm to exert its anti-bacterial effects. More importantly, the aggregation of surface-associated microorganisms forms biofilms, and the penetration specificity of antibiotics is directly related to the efficacy of OM (45, 46). In addition to biofilm formation, both Gram-negative and Gram-positive bacteria can actively expel antibiotics from cells via efflux pumps, which is another major drug resistance mechanism (47). Gram-negative bacteria are usually more resistant to the action of anti-bacterial drugs than Gram-positive bacteria due to the presence of porins (48, 49).
Rifampicin is a broad-spectrum antimicrobial agent with penetration specificity for biofilms produced by S. aureus and it can kill adherent bacteria (50). Because of the powerful biofilm penetration capacity, RIF is used as a basic therapeutic measure in the clinical treatment of OM. Nevertheless, the use of RIF alone in the treatment of S. aureus-associated infections is likely to rapidly develop drug resistance, increase MIC, and reduce the effectiveness of antibiotics (51, 52). Therefore, the antibiotic regimen of RIF combined with drugs has been used in preclinical and clinical studies for nearly two decades. GLY antibiotics lyse bacteria by binding to their cell wall peptidoglycans and primarily target most Gram-positive bacteria; however, they have significant limitations, such as poor tissue and intracellular permeability, lack of activity against biofilms, and a slow bactericidal effect (53). More importantly, RIF enhances the activity of vancomycin against S. aureus in biofilms (54), compensating for the above disadvantages of GLY. In terms of clinical efficacy, GLY antibiotics such as vancomycin remain first-line agents for the treatment of S. aureus or MRSA-induced OM. Recently, a case of successfully treated polymicrobial calcaneal OM using oral RIF in combination with intravenous vancomycin was described (55).
The combination of RIF and β-Lactam is a common pairing in the clinical treatment of bone infections. β-Lactam antibiotics inhibit the synthesis of bacterial cell walls and affect normal bacterial growth and development (56, 57). Among β-Lactams, cefuroxime and cephalothin have been shown to be effective in the presence of biofilm (58). However, β-Lactams are the most common cause of most antibiotics-associated adverse events, including severe kidney or liver toxicity, neurotoxicity, and cytopenia (59). One study showed a 2.5% incidence of gastrointestinal events with β-Lactam and a higher incidence of diarrhea when the above combination was used (60). More importantly, the β-Lactams assessed in this study included only first and second-generation cephalosporins, and no valid direct evidence could be produced to support the efficacy of the novel cephalosporins on OM.
Fosfomycin is a broad-spectrum antibiotic that inhibits the biosynthesis of peptidoglycan in the bacterial cell wall by inhibiting MurA enzyme activity and it exhibits bactericidal activity against a wide range of Gram-negative bacteria (61, 62). Although FOS demonstrated a significant advantage in reducing bacterial counts compared to RIF+GLY in our analysis, FOS is commonly used in the treatment of complicated urinary tract infections, and its use as a clinical treatment for OM has not been reported. Importantly, the incidence of hypokalemia with intravenous FOS infusion was as high as 26%, a very high incredible rate (63). In contrast, the nephrotoxicity of vancomycin could be avoided by reducing the dose of the drug or by replacing it with teicoplanin, which exhibits less nephrotoxicity. The primary adverse reactions of RIF were gastrointestinal reactions and certain hepatotoxic effects (transaminase levels increased to 3–5 times normal levels). Perveen et al. (64), Ibrahim et al. (65); however, the gastrointestinal symptoms and hepatotoxicity of RIF could also be addressed by lowering the dose (66).
In terms of cost, the median daily drug price of RIF for infection was less than 0.115 dollars (67). For the treatment of S. aureus-associated infection, the cost of vancomycin was generally very low, while for MRSA the cost increased accordingly. The problem of refractory MRSA-associated OM has been solved with the use of new GLY antibiotics including dalbavancin and teicoplanin, which also have the advantage of shorter hospital stays and low total costs.
Thus, RIF+GLY may be the most promising clinical option for OM treatment in terms of biofilm penetration, clinical feasibility, cost-effectiveness, and safety. However, the drug–drug interactions between RIF and co-antibiotics may reduce the effective blood levels of the co-antibiotics (68). Conversely, it is worth paying attention to the high incidence of side effects of RIF combinations, which highlights the potential need for therapeutic drug monitoring and rational administration during clinical treatment.
In the present study, only a few studies briefly reported adverse reactions in animals after administration, including seven rabbits in the TIG group that died from gastrointestinal inflammation and seven rabbits in the RIF+β-Lactam group that died from gastrointestinal reactions (diarrhea and gastrointestinal inflammation). Moreover, two rabbits and three rats in the RIF+GLY group, and one rabbit in the GLY group had an unknown cause of death. Gastrointestinal inflammation in the TIG and RIF+β-Lactam groups may be caused by extensive destruction of the normal intestinal flora (20). Additionally, gastrointestinal adverse effects of RIF include common symptoms, such as loss of appetite and diarrhea; however, gastrointestinal inflammation is rare (69). Therefore, in future, the adverse effects of antibiotic combinations should be considered in the selection of antibiotic regimens for the treatment of OM.
First, due to a lack of clinical literature and the absence of primary outcomes, we used preclinical animal studies to assess the efficacy of various antibiotic regimens. Nevertheless, we could give clear recommendations based on the existing studies on humans.
Second, the pathogenic bacteria used to establish the OM animal model were not identical and included some uncommon bacteria, such as Klebsiella pneumoniae and Morganella. After conventional meta-analysis low heterogeneity in conventional meta-analysis for S. aureus and MRSA separately, but this might still affect the interpretation of efficacy.
Third, in our selection of animal model species for OM, we included only the most common rat and rabbit as target models, and therefore the efficacy results should be interpreted with caution. The primary reason for this is that most of the current comparative studies on antibiotic treatments for OM have focused on rat and rabbit models of OM, and other models such as dog, pig, and sheep lack valid comparative evidence.
First, selection of animal models: Current therapeutic animal models for OM are limited to rats and rabbits, such animals differ greatly from humans in vivo, particularly with regard to the pathological molecular biology, thus they have limited reference value regarding clinical treatment (70). Therefore, animal models that are more similar to humans, such as monkeys and apes, should be considered in future to better simulate the pathological conditions of clinical OM.
Second, safety indicators: Only a very small number of studies have reported the gastrointestinal adverse effects of antibiotics, and most studies focused on efficacy and bacterial eradication rates. Therefore, attention should be paid to the safety of antibiotics in future to avoid adverse events in clinical applications. In addition, preclinical trials for OM treatment should take into account the rigor of design and the standardization of outcome assessment to improve the quality of the study and provide a reference for the clinical treatment of OM as much as possible.
Third, as antimicrobial resistance continues to increase worldwide, the biofilm penetration of vancomycin and β-lactam antibiotics for Gram-positive bacteria is diminishing. Novel glycopeptide antibiotics such as oritavancin and telavancin, and fifth-generation cephalosporin antibiotics such as ceftobiprole should be given more attention in future and applied in preclinical and clinical studies to provide a reliable basis for antibiotic treatment options for OM.
Fourth, local antibiotic delivery systems have become the key development direction of preclinical and clinical research for the treatment of OM in recent years (71). In addition to the mechanical support, biocompatibility, biodegradability, and osteoinductive ability of the new material, the combination of new antibiotics also need more research in the animal models of OM to achieve the final clinical application.
Fifth, the vast majority of comparative studies on the efficacy of antibiotics use rabbits and rats as model animals to study OM. However, it has been reported that, in comparison to the serious adverse reactions of rabbits after antibiotic treatment, the gastrointestinal flora of the pig is similar to that of humans and can be used to assess systemic antibiotic treatments (72, 73), and the mechanical and biological functions of dogs' tibia and femur are similar to those of humans (74). Therefore, researchers need to further explore other animal models of OM that are more similar to human bodies and evaluate the efficacy of antibiotics, in order to provide theoretical support for the clinical treatment of OM with antibiotics in future.
In conclusion, multiple antibiotic regimens have shown significant value in animal models of OM, including increased efficacy, reduced bacterial counts, and lower radiological scores. Among them, RIF + GLY was significantly effective and possibly the most promising treatment regimen. However, it is necessary for future preclinical studies to provide more reliable evidence for the clinical treatment of OM using this regimen.
The original contributions presented in the study are included in the article/Supplementary material, further inquiries can be directed to the corresponding authors.
XS and YX conceived the study and wrote the manuscript. HN, YW, and XC carried out the data collection and data analysis. XS and YW assessed the quality of the studies. All authors reviewed the results and approved the final version of the manuscript.
This study was funded by National Natural Science Foundation of China (Grant Nos. 81772367 and 82072392), the Yunnan Traumatology and Orthopedics Clinical Medical Center (Grant No. ZX20191001), and the Grants from Yunnan Orthopedics and Sports Rehabilitation Clinical Medicine Research Center (Grant No. 202102AA310068).
The authors declare that the research was conducted in the absence of any commercial or financial relationships that could be construed as a potential conflict of interest.
All claims expressed in this article are solely those of the authors and do not necessarily represent those of their affiliated organizations, or those of the publisher, the editors and the reviewers. Any product that may be evaluated in this article, or claim that may be made by its manufacturer, is not guaranteed or endorsed by the publisher.
The Supplementary Material for this article can be found online at: https://www.frontiersin.org/articles/10.3389/fmed.2022.975666/full#supplementary-material
1. Lew DP, Waldvogel FA. Osteomyelitis. Lancet. (2004) 364:369–79. doi: 10.1016/S0140-6736(04)16727-5
2. Darouiche RO. Treatment of infections associated with surgical implants. N Engl J Med. (2004) 350:1422–9. doi: 10.1056/NEJMra035415
3. Grammatico L, Baron S, Rusch E, Lepage B, Surer N, Desenclos JC, et al. Epidemiology of vertebral osteomyelitis (VO) in France: analysis of hospital-discharge data 2002-2003. Epidemiol Infect. (2008) 136:653–60. doi: 10.1017/S0950268807008850
4. Schwank S, Rajacic Z, Zimmerli W, Blaser J. Impact of bacterial biofilm formation on in vitro and in vivo activities of antibiotics. Antimicrob Agents Chemother. (1998) 42:895–8. doi: 10.1128/AAC.42.4.895
5. Zimmerli W, Widmer AF, Blatter M, Frei R, Ochsner PE. Role of rifampin for treatment of orthopedic implant-related staphylococcal infections: a randomized controlled trial. Foreign-Body Infection (FBI) Study Group. Jama. (1998) 279:1537–41. doi: 10.1001/jama.279.19.1537
6. Drancourt M, Stein A, Argenson JN, Roiron R, Groulier P, Raoult D. Oral treatment of Staphylococcus spp. infected orthopaedic implants with fusidic acid or ofloxacin in combination with rifampicin. J Antimicrob Chemother. (1997) 39:235–40. doi: 10.1093/jac/39.2.235
7. Karlsen ØE, Borgen P, Bragnes B, Figved W, Grøgaard B, Rydinge J, et al. Rifampin combination therapy in staphylococcal prosthetic joint infections: a randomized controlled trial. J Orthop Surg Res. (2020) 15:365. doi: 10.1186/s13018-020-01877-2
8. Hooijmans CR, Rovers MM, De Vries RB, Leenaars M, Ritskes-Hoitinga M, Langendam MW. SYRCLE's risk of bias tool for animal studies. BMC Med Res Methodol. (2014) 14:43. doi: 10.1186/1471-2288-14-43
9. Higgins JP, Altman DG, Gøtzsche PC, Jüni P, Moher D, Oxman AD, et al. The Cochrane Collaboration's tool for assessing risk of bias in randomised trials. Bmj. (2011) 343:d5928. doi: 10.1136/bmj.d5928
10. Page MJ, McKenzie JE, Bossuyt PM, Boutron I, Hoffmann TC, Mulrow CD, et al. The PRISMA 2020 statement: an updated guideline for reporting systematic reviews. BMJ. (2021) 372:n71. doi: 10.1136/bmj.n71
11. Norden CW. Experimental osteomyelitis. IV Therapeutic trials with rifampin alone and in combination with gentamicin, sisomicin, and cephalothin. J Infect Dis. (1975) 132:493–9. doi: 10.1093/infdis/132.5.493
12. Norden CW, Keleti E. Treatment of experimental staphylococcal osteomyelitis with rifampin and trimethoprim, alone and in combination. Antimicrob Agents Chemother. (1980) 17:591–4. doi: 10.1128/AAC.17.4.591
13. Norden CW. Experimental chronic staphylococcal osteomyelitis in rabbits: treatment with rifampin alone and in combination with other antimicrobial agents. Rev Infect Dis. (1983) 5 Suppl 3:S491–4. doi: 10.1093/clinids/5.Supplement_3.S491
14. Norden CW, Shaffer M. Treatment of experimental chronic osteomyelitis due to staphylococcus aureus with vancomycin and rifampin. J Infect Dis. (1983) 147:352–7. doi: 10.1093/infdis/147.2.352
15. Norden CW, Shaffer M. Treatment of experimental chronic osteomyelitis caused by Morganella morganii with mezlocillin and cloxacillin. Chemotherapy. (1984) 30:188–93. doi: 10.1159/000238267
16. Mader JT, Morrison LT, Adams KR. Comparative evaluation of A-56619, A-56620, and nafcillin in the treatment of experimental Staphylococcus aureus osteomyelitis. Antimicrob Agents Chemother. (1987) 31:259–63. doi: 10.1128/AAC.31.2.259
17. Mader JT, Adams K. Comparative evaluation of daptomycin (LY146032) and vancomycin in the treatment of experimental methicillin-resistant Staphylococcus aureus osteomyelitis in rabbits. Antimicrob Agents Chemother. (1989) 33:689–92. doi: 10.1128/AAC.33.5.689
18. Shirtliff ME, Mader JT, Calhoun J. Oral rifampin plus azithromycin or clarithromycin to treat osteomyelitis in rabbits. Clin Orthop Relat Res. (1999) 359:229–36. doi: 10.1097/00003086-199902000-00026
19. Shirtliff ME, Calhoun JH, Mader JT. Comparative evaluation of oral levofloxacin and parenteral nafcillin in the treatment of experimental methicillin-susceptible Staphylococcus aureus osteomyelitis in rabbits. J Antimicrob Chemother. (2001) 48:253–8. doi: 10.1093/jac/48.2.253
20. Yin LY, Lazzarini L, Li F, Stevens CM, Calhoun JH. Comparative evaluation of tigecycline and vancomycin, with and without rifampicin, in the treatment of methicillin-resistant Staphylococcus aureus experimental osteomyelitis in a rabbit model. J Antimicrob Chemother. (2005) 55:995–1002. doi: 10.1093/jac/dki109
21. Lefebvre M, Jacqueline C, Amador G, Le Mabecque V, Miegeville A, Potel G, et al. Efficacy of daptomycin combined with rifampicin for the treatment of experimental meticillin-resistant Staphylococcus aureus (MRSA) acute osteomyelitis. Int J Antimicrob Agents. (2010) 36:542–4. doi: 10.1016/j.ijantimicag.2010.07.008
22. Saleh-Mghir A, Dumitrescu O, Dinh A, Boutrad Y, Massias L, Martin E, et al. Ceftobiprole efficacy in vitro against Panton-Valentine leukocidin production and in vivo against community-associated methicillin-resistant Staphylococcus aureus osteomyelitis in rabbits. Antimicrob Agents Chemother. (2012) 56:6291–7. doi: 10.1128/AAC.00926-12
23. Crémieux AC, Dinh A, Nordmann P, Mouton W, Tattevin P, Ghout I, et al. Efficacy of colistin alone and in various combinations for the treatment of experimental osteomyelitis due to carbapenemase-producing Klebsiella pneumoniae. J Antimicrob Chemother. (2019) 74:2666–75. doi: 10.1093/jac/dkz257
24. Kandemir O, Oztuna V, Colak M, Akdag A, Camdeviren H. Comparison of the efficacy of tigecycline and teicoplanin in an experimental methicillin-resistant Staphylococcus aureus osteomyelitis model. J Chemother. (2008) 20:53–7. doi: 10.1179/joc.2008.20.1.53
25. Poeppl W, Tobudic S, Lingscheid T, Plasenzotti R, Kozakowski N, Lagler H, et al. Daptomycin, fosfomycin, or both for treatment of methicillin-resistant Staphylococcus aureus osteomyelitis in an experimental rat model. Antimicrob Agents Chemother. (2011) 55:4999–5003. doi: 10.1128/AAC.00584-11
26. Poeppl W, Lingscheid T, Bernitzky D, Schwarze UY, Donath O, Perkmann T, et al. Efficacy of fosfomycin compared to vancomycin in treatment of implant-associated chronic methicillin-resistant Staphylococcus aureus osteomyelitis in rats. Antimicrob Agents Chemother. (2014) 58:5111–6. doi: 10.1128/AAC.02720-13
27. Kussmann M, Obermueller M, Berndl F, Reischer V, Veletzky L, Burgmann H, et al. Dalbavancin for treatment of implant-related methicillin-resistant Staphylococcus aureus osteomyelitis in an experimental rat model. Sci Rep. (2018) 8:9661. doi: 10.1038/s41598-018-28006-8
28. Karau MJ, Schmidt-Malan SM, Yan Q, Greenwood-Quaintance KE, Mandrekar J, Lehoux D, et al. Exebacase in Addition to Daptomycin Is More Active than Daptomycin or Exebacase Alone in Methicillin-Resistant Staphylococcus aureus Osteomyelitis in Rats. Antimicrob Agents Chemother. (2019) 63. doi: 10.1128/AAC.01235-19
29. Lou FL, Zheng ZH, Fu FJ. Therapeutic Effects of Terazolamide on Methicillin-Resistant Staphylococcus Epidermidis Implant Associated Osteomyelitis. Zhongguo Yi Yuan Yong Yao Ping Jia Yu Fen Xi. (2021) 21:420–3.
30. Gracia E, Laclériga A, Monzón M, Leiva J, Oteiza C, Amorena B. Application of a rat osteomyelitis model to compare in vivo and in vitro the antibiotic efficacy against bacteria with high capacity to form biofilms. J Surg Res. (1998) 79:146–53. doi: 10.1006/jsre.1998.5416
31. Kalteis T, Beckmann J, Schröder HJ, Handel M, Grifka J, Lehn N, et al. Moxifloxacin superior to vancomycin for treatment of bone infections–a study in rats. Acta Orthop. (2006) 77:315–9. doi: 10.1080/17453670610046082
32. Vergidis P, Rouse MS, Euba G, Karau MJ, Schmidt SM, Mandrekar JN, et al. Treatment with linezolid or vancomycin in combination with rifampin is effective in an animal model of methicillin-resistant Staphylococcus aureus foreign body osteomyelitis. Antimicrob Agents Chemother. (2011) 55:1182–6. doi: 10.1128/AAC.00740-10
33. Vergidis P, Schmidt-Malan SM, Mandrekar JN, Steckelberg JM, Patel R. Comparative activities of vancomycin, tigecycline and rifampin in a rat model of methicillin-resistant Staphylococcus aureus osteomyelitis. J Infect. (2015) 70:609–15. doi: 10.1016/j.jinf.2014.12.016
34. Brinkman CL, Schmidt-Malan SM, Mandrekar JN, Patel R. Rifampin-Based Combination Therapy Is Active in Foreign-Body Osteomyelitis after Prior Rifampin Monotherapy. Antimicrob Agents Chemother. (2017) 61:e01822–16. doi: 10.1128/AAC.01822-16
35. Karau MJ, Schmidt-Malan SM, Albano M, Mandrekar JN, Rivera CG, Osmon DR, et al. Novel use of rifabutin and rifapentine to treat methicillin-resistant staphylococcus aureus in a rat model of foreign body osteomyelitis. J Infect Dis. (2020) 222:1498–504. doi: 10.1093/infdis/jiaa401
36. Dworkin R, Modin G, Kunz S, Rich R, Zak O, Sande M. Comparative efficacies of ciprofloxacin, pefloxacin, and vancomycin in combination with rifampin in a rat model of methicillin-resistant Staphylococcus aureus chronic osteomyelitis. Antimicrob Agents Chemother. (1990) 34:1014–6. doi: 10.1128/AAC.34.6.1014
37. O'Reilly T, Kunz S, Sande E, Zak O, Sande MA, Täuber MG. Relationship between antibiotic concentration in bone and efficacy of treatment of staphylococcal osteomyelitis in rats: azithromycin compared with clindamycin and rifampin. Antimicrob Agents Chemother. (1992) 36:2693–7. doi: 10.1128/AAC.36.12.2693
38. Luu QN, Buxton TB, Nelson DR, Rissing JP. Treatment of chronic experimental Staphylococcus aureus osteomyelitis with LY146032 and vancomycin. Eur J Clin Microbiol Infect Dis. (1989) 8:562–3. doi: 10.1007/BF01967482
39. Park KH, Greenwood-Quaintance KE, Schuetz AN, Mandrekar JN, Patel R. Activity of tedizolid in methicillin-resistant staphylococcus epidermidis experimental foreign body-associated osteomyelitis. Antimicrob Agents Chemother. (2017) 61:e01644–16. doi: 10.1128/AAC.01644-16
40. Karamanis EM, Matthaiou DK, Moraitis LI, Falagas ME. Fluoroquinolones versus beta-lactam based regimens for the treatment of osteomyelitis: a meta-analysis of randomized controlled trials. Spine. (2008) 33:E297–304. doi: 10.1097/BRS.0b013e31816f6c22
41. Rybak M, Lomaestro B, Rotschafer JC, Moellering RJr, Craig W, Billeter M, et al. Therapeutic monitoring of vancomycin in adult patients: a consensus review of the American Society of Health-System Pharmacists, the Infectious Diseases Society of America, and the Society of Infectious Diseases Pharmacists. Am J Health Syst Pharm. (2009) 66:82–98. doi: 10.2146/ajhp080434
42. Nair AB, Jacob S. A simple practice guide for dose conversion between animals and human. J Basic Clin Pharm. (2016) 7:27–31. doi: 10.4103/0976-0105.177703
43. Morris TH. Antibiotic therapeutics in laboratory animals. Lab Anim. (1995) 29:16–36. doi: 10.1258/002367795780740393
44. Jacobs MR. Optimisation of antimicrobial therapy using pharmacokinetic and pharmacodynamic parameters. Clin Microbiol Infect. (2001) 7:589–96. doi: 10.1046/j.1198-743x.2001.00295.x
45. Masters EA, Ricciardi BF, Bentley KLM, Moriarty TF, Schwarz EM, Muthukrishnan G. Skeletal infections: microbial pathogenesis, immunity and clinical management. Nat Rev Microbiol. (2022) 20:385–400. doi: 10.1038/s41579-022-00686-0
46. González-Martín M, Silva V, Poeta P, Corbera JA, Tejedor-Junco MT. Microbiological aspects of osteomyelitis in veterinary medicine: drawing parallels to the infection in human medicine. Vet Q. (2022) 42:1–11. doi: 10.1080/01652176.2021.2022244
47. Nag A, Mehra S. Involvement of the SCO3366 efflux pump from S. coelicolor in rifampicin resistance and its regulation by a TetR regulator. Appl Microbiol Biotechnol. (2022) 106:2175–90. doi: 10.1007/s00253-022-11837-x
48. Braun V, Bös C, Braun M, Killmann H. Outer membrane channels and active transporters for the uptake of antibiotics. J Infect Dis. (2001) 183 Suppl 1:S12–6. doi: 10.1086/318840
49. Malanovic N, Lohner K. Gram-positive bacterial cell envelopes: The impact on the activity of antimicrobial peptides. Biochim Biophys Acta. (2016) 1858:936–46. doi: 10.1016/j.bbamem.2015.11.004
50. Fazly Bazzaz BS, Khameneh B, Zarei H, Golmohammadzadeh S. Antibacterial efficacy of rifampin loaded solid lipid nanoparticles against Staphylococcus epidermidis biofilm. Microb Pathog. (2016) 93:137–44. doi: 10.1016/j.micpath.2015.11.031
51. Levin-Reisman I, Ronin I, Gefen O, Braniss I, Shoresh N, Balaban NQ. Antibiotic tolerance facilitates the evolution of resistance. Science. (2017) 355:826–30. doi: 10.1126/science.aaj2191
52. Liu J, Gefen O, Ronin I, Bar-Meir M, Balaban NQ. Effect of tolerance on the evolution of antibiotic resistance under drug combinations. Science. (2020) 367:200–4. doi: 10.1126/science.aay3041
53. Guskey MT, Tsuji BT. A comparative review of the lipoglycopeptides: oritavancin, dalbavancin, and telavancin. Pharmacotherapy. (2010) 30:80–94. doi: 10.1592/phco.30.1.80
54. Jørgensen NP, Skovdal SM, Meyer RL, Dagnæs-Hansen F, Fuursted K, Petersen E. Rifampicin-containing combinations are superior to combinations of vancomycin, linezolid and daptomycin against Staphylococcus aureus biofilm infection in vivo and in vitro. Pathog Dis. (2016) 74:ftw019. doi: 10.1093/femspd/ftw019
55. Brinkman MB, Fan K, Shiveley RL, Van Anglen LJ. Successful treatment of polymicrobial calcaneal osteomyelitis with telavancin, rifampin, and meropenem. Ann Pharmacother. (2012) 46:e15. doi: 10.1345/aph.1Q331
56. Yun HC, Ellis MW, Jorgensen JH. Activity of ceftobiprole against community-associated methicillin-resistant Staphylococcus aureus isolates recently recovered from US military trainees. Diagn Microbiol Infect Dis. (2007) 59:463–6. doi: 10.1016/j.diagmicrobio.2007.06.023
57. Leonard SN, Cheung CM, Rybak MJ. Activities of ceftobiprole, linezolid, vancomycin, and daptomycin against community-associated and hospital-associated methicillin-resistant Staphylococcus aureus. Antimicrob Agents Chemother. (2008) 52:2974–6. doi: 10.1128/AAC.00257-08
58. Anwar H, Strap JL, Costerton JW. Eradication of biofilm cells of Staphylococcus aureus with tobramycin and cephalexin. Can J Microbiol. (1992) 38:618–25. doi: 10.1139/m92-102
59. Vardakas KZ, Kalimeris GD, Triarides NA, Falagas ME. An update on adverse drug reactions related to β-lactam antibiotics. Expert Opin Drug Saf. (2018) 17:499–508. doi: 10.1080/14740338.2018.1462334
60. Lagacé-Wiens PR, Rubinstein E. Pharmacokinetic and pharmacodynamics evaluation of ceftobiprole medocaril for the treatment of hospital-acquired pneumonia. Expert Opin Drug Metab Toxicol. (2013) 9:789–99. doi: 10.1517/17425255.2013.788150
61. Schintler MV, Traunmüller F, Metzler J, Kreuzwirt G, Spendel S, Mauric O, et al. High fosfomycin concentrations in bone and peripheral soft tissue in diabetic patients presenting with bacterial foot infection. J Antimicrob Chemother. (2009) 64:574–8. doi: 10.1093/jac/dkp230
62. Falagas ME, Kastoris AC, Kapaskelis AM, Karageorgopoulos DE. Fosfomycin for the treatment of multidrug-resistant, including extended-spectrum beta-lactamase producing, Enterobacteriaceae infections: a systematic review. Lancet Infect Dis. (2010) 10:43–50. doi: 10.1016/S1473-3099(09)70325-1
63. Florent A, Chichmanian RM, Cua E, Pulcini C. Adverse events associated with intravenous fosfomycin. Int J Antimicrob Agents. (2011) 37:82–3. doi: 10.1016/j.ijantimicag.2010.09.002
64. Perveen S, Kumari D, Singh K, Sharma R. Tuberculosis drug discovery: Progression and future interventions in the wake of emerging resistance. Eur J Med Chem. (2022) 229:114066. doi: 10.1016/j.ejmech.2021.114066
65. Ibrahim SM, Pithavala YK, Vourvahis M, Chen J. A literature review of liver function test elevations in rifampin drug-drug interaction studies. Clin Transl Sci. (2022) 15:1561–80. doi: 10.1111/cts.13281
66. Hagihara M, Crandon JL, Nicolau DP. The efficacy and safety of antibiotic combination therapy for infections caused by Gram-positive and Gram-negative organisms. Expert Opin Drug Saf. (2012) 11:221–33. doi: 10.1517/14740338.2012.632631
67. Chow I, Lemos EV, Einarson TR. Management and prevention of diabetic foot ulcers and infections: a health economic review. Pharmacoeconomics. (2008) 26:1019–35. doi: 10.2165/0019053-200826120-00005
68. Pushkin R, Iglesias-Ussel MD, Keedy K, Maclauchlin C, Mould DR, Berkowitz R, et al. A randomized study evaluating oral fusidic acid (CEM-102) in combination with oral rifampin compared with standard-of-care antibiotics for treatment of prosthetic joint infections: a newly identified drug-drug interaction. Clin Infect Dis. (2016) 63:1599–604. doi: 10.1093/cid/ciw665
69. Forget EJ, Menzies D. Adverse reactions to first-line antituberculosis drugs. Expert Opin Drug Saf. (2006) 5:231–49. doi: 10.1517/14740338.5.2.231
70. Reizner W, Hunter JG, O'Malley NT, Southgate RD, Schwarz EM, Kates SL. A systematic review of animal models for Staphylococcus aureus osteomyelitis. Eur Cell Mater. (2014) 27:196–212. doi: 10.22203/eCM.v027a15
71. Cobb LH, Mccabe EM, Priddy LB. Therapeutics and delivery vehicles for local treatment of osteomyelitis. J Orthop Res. (2020) 38:2091–103. doi: 10.1002/jor.24689
72. Jensen LK, Koch J, Dich-Jorgensen K, Aalbaek B, Petersen A, Fuursted K, et al. Novel porcine model of implant-associated osteomyelitis: A comprehensive analysis of local, regional, and systemic response. J Orthop Res. (2017) 35:2211–21. doi: 10.1002/jor.23505
73. Ozawa S, Mans C, Szabo Z, Di Girolamo N. Epidemiology of bacterial conjunctivitis in chinchillas (Chinchilla lanigera): 49 cases (2005 to 2015). J Small Anim Pract. (2017) 58:238–45. doi: 10.1111/jsap.12644
Keywords: osteomyelitis, antibiotic regimens, bacteria, network meta-analysis, animal
Citation: Shi X, Wu Y, Ni H, Chen X and Xu Y (2022) Comparing the efficacy of different antibiotic regimens on osteomyelitis: A network meta-analysis of animal studies. Front. Med. 9:975666. doi: 10.3389/fmed.2022.975666
Received: 23 June 2022; Accepted: 12 September 2022;
Published: 06 October 2022.
Edited by:
Eduardo Rodriguez-Noriega, Civil Hospital of Guadalajara, MexicoReviewed by:
Lauren B. Priddy, Mississippi State University, United StatesCopyright © 2022 Shi, Wu, Ni, Chen and Xu. This is an open-access article distributed under the terms of the Creative Commons Attribution License (CC BY). The use, distribution or reproduction in other forums is permitted, provided the original author(s) and the copyright owner(s) are credited and that the original publication in this journal is cited, in accordance with accepted academic practice. No use, distribution or reproduction is permitted which does not comply with these terms.
*Correspondence: Yongqing Xu, ZG9jeHV5b25ncWluZ0BzaW5hLmNvbQ==
†These authors have contributed equally to this work
Disclaimer: All claims expressed in this article are solely those of the authors and do not necessarily represent those of their affiliated organizations, or those of the publisher, the editors and the reviewers. Any product that may be evaluated in this article or claim that may be made by its manufacturer is not guaranteed or endorsed by the publisher.
Research integrity at Frontiers
Learn more about the work of our research integrity team to safeguard the quality of each article we publish.