- 1Central Laboratory, Department of Liver Diseases, ShuGuang Hospital, Affiliated to Shanghai University of Chinese Traditional Medicine, Shanghai, China
- 2Institute of Infection Diseases, Shanghai University of Chinese Traditional Medicine, Shanghai, China
Drug-induced liver injury (DILI) is a disease that remains difficult to predict and prevent from a clinical perspective, as its occurrence is hard to fully explain by the traditional mechanisms. In recent years, the risk of the DILI for microbiota dysbiosis has been recognized as a multifactorial process. Amoxicillin-clavulanate is the most commonly implicated drug in DILI worldwide with high causality gradings based on the use of RUCAM in different populations. Antibiotics directly affect the structure and diversity of gut microbiota (GM) and changes in metabolites. The depletion of probiotics after antibiotics interference can reduce the efficacy of hepatoprotective agents, also manifesting as liver injury. Follow-up with liver function examination is essential during the administration of drugs that affect intestinal microorganisms and their metabolic activities, such as antibiotics, especially in patients on a high-fat diet. In the meantime, altering the GM to reconstruct the hepatotoxicity of drugs by exhausting harmful bacteria and supplementing with probiotics/prebiotics are potential therapeutic approaches. This review will provide an overview of the current evidence between gut microbiota and DILI events, and discuss the potential mechanisms of gut microbiota-mediated drug interactions. Finally, this review also provides insights into the “double-edged sword” effect of antibiotics treatment against DILI and the potential prevention and therapeutic strategies.
Introduction
Drug-induced liver injury (DILI) is an underappreciated adverse drug reaction, in that the diagnosis of it still relies on the causality assessment, and that the Roussel Uclaf Causality Assessment Method (RUCAM) (1, 2) is the most commonly used scale recommended by various liver and Gastrointestinal associations (3–5). DILI can mimic features of various liver diseases, such as acute liver failure, drug-induced autoimmune hepatitis, and drug-associated fatty liver disease. The dramatic increase in drug-induced acute liver failure, setbacks in anti-tumor treatment, and herbal-related liver injury have raised global public health concerns (6). Specifically, the epidemiology and etiology of DILI differ in various countries and populations (7). The incidence rates range from 2.3 per 100,000 people in Sweden (8) to 19.1 per 100,000 people in Iceland (9). Even with rigorous preclinical toxicology tests, DILI events still occur unpredictably (5).
Since their invention in 1928, antibiotics have become life-saving medicines (10). Removal of bacterial taxa involved in the occurrence and progression of liver injury by vancomycin could alleviate liver disease in recent years (11, 12). However, the irrational use of antibiotics is a serious public health issue, and antibiotics appear to be a common cause of DILI, according to the etiology studies on causative agents tested for causality by RUCAM (13–15). Recently, antibiotics have been reported as the major agents responsible for DILI events in COVID-19 patients, which were the second only to antiviral drugs (16) (Supplementary Table 1). The drug-drug interactions (DDIs) mediated by the GM may trigger unpredictable adverse effects known as idiosyncratic DILI (iDIL) events (17). Notably, the use of antibiotics can also be involved in the protection and detoxification of DILI, and the mechanism and clinical manifestation of these two opposite situations may microbiologically share some common features as well as diversities (12, 18).
Metabolism has an overall effect on generating new toxicity or eliminating drug toxicity (19). Aside from the liver, the microbiota dispersed throughout the human body, especially in the intestine, also plays a crucial role in drug metabolism (20, 21). On the other hand, genomics links human genome variation to the unpredictability of DILI. Genome-wide association studies (GWAS) is a well-established field revealing how human leukocyte antigen (HLA) alleles or non-HLA variants increase susceptibility to DILI (22, 23). Nevertheless, their application in the diagnosis and management of DILI has been difficult (24). The microbial genome is considered to be the second human genome. The concept of pharmaceutical microbiomes brings a prospective approach to understand and address drug safety issues, as it is a modifiable pharmacogenomics (25).
Gut microbiota (GM) is an abundant and complex ecosystem containing 1013 microorganisms, mainly including Firmicutes (79.4%), Bacteroides (16.9%), Actinobacteria (2.5%), and Proteobacteria (1%) (26, 27). GM formation is influenced by multivariate determinants, such as host genetic factors, diet and lifestyle, environments, and oral drug use (28–30). Antibiotics appear to be a correlative drug for the microbiota remodeling (31), especially in newborns (32). GM and its metabolites are significant partakers in liver physiological functions, such as energy metabolism regulation, immune regulation, and modulation of resistance to infection (33, 34), which may also result in liver diseases such as inflammation, hepatic steatosis, and fibrosis (35–38).
In recent years, a model based on sterile or antibiotic-supplemented microbiota-depleted rodents has been used to explore the role of microbial intervention in experimental pharmacomicrobiomics. Summarizing the results of these experiments will help us to further understand the interaction between antibiotics and microorganisms, as well as their relationship with drug-induced hepatotoxicity. However, no past review had comprehensively examined whether GM could mitigate or aggravate DILI. This review will provide an overview of the current evidence between GM and DILI events, and discuss the potential mechanisms underlying the gut microbiota-mediated drug interactions. Finally, this review will also provide insights into the “double-edged sword” effect of antibiotics treatment against DILI and the potential prevention and therapeutic strategies. Finally, we hope that this frontier and conceptual research can at least provide a different tack to better understand the mechanism of iDILI, and find ways to prevent or treat it.
The role of gut microbiota in drug-induced liver injury
Gut-liver axis is the structural basis of drug-induced liver injury
Numerous viewpoints have emphasized the importance of a balanced intestinal microbiome in liver physiology and pathology (39). GM forms an axis with the liver primarily through a portal circulation, known as the gut-liver axis (40). This symbiotic relationship allows GM and its metabolites to be transferred from the gastrointestinal tract to the liver. In return, the liver secretes bile acids and antibodies to the intestinal lumen to regulate the composition and distribution of microbiota (41).
A functional gut-liver axis relies on a complete and solid intestinal barrier. So, there are several layers of defense that make up the intestinal barrier. The outermost layer is the mucus barrier, containing the bacterial colonization layer and the adhesive aseptic layer (42). In addition to immobilizing bacteria, the mucus barrier is also a source of nutrients for bacteria. For example, Akkermansia municiphila is a potential hepatoprotective bacterium that can maintain its growth by degrading mucins (43). The second layer is the epithelial barrier formed by a monolayer of epithelial cells, providing protection through its chemical and physical stability. Adjacent epithelial cells are closely connected by tight junctions, forming a physical barrier (44). Antimicrobial peptides and secreted IgA on the intestinal mucosa can block intestinal immune responses induced by microbial pathogens stimulation. Interactions among epithelial cells, immune cells, and mesenchymal cells create the intestinal mucosal ecological network that enables intestinal homeostasis (45). Gut vascular barrier (GVB) is another layer that prevents bacteria and their metabolites from entering the portal circulation when the outer mucus and epithelial barrier are broken (46).
Disruption of the three-layer barrier increases intestinal permeability, and the translocation of bacterial and microbial metabolites to the liver plays a key role in the pathogenesis of DILI (47, 48). Antigens derived from pathogenic microorganisms or drugs, such as lipopolysaccharides (LPS), cause microbiota-associated molecular pattern (MAMP) to activate nuclear factors κB (NF-κB) through toll-like receptors (TLRs) and nod-like receptors (NLRs), subsequently the released inflammatory cytokines and chemokines enter the portal circulation and reach the liver (49), where they trigger the proinflammatory cascade (50, 51). These antigens also lead to a series of excessive hepatic innate or acquired immune activation, such as NK cells, macrophages, and the release amounts of proinflammatory cytokines (52).
Interestingly, barrier damage may cause the involvement of activated stellate cells in fibrosis and, in turn, cirrhosis allows GM to enter the portal-venous circulation by destroying these barriers (42, 53). Cytokines downregulate the expression of tight junction proteins, thus changing the tight junction, of which the increase of intestinal permeability may be the mechanism (54).
Composition and metabolites of gut microbiota and drug-induced liver injury
DILI is considered the consequence of a combination of variable host or non-host risk factors (55, 56), which may act by influencing the gut microbiota composition and metabolites (Table 1). Numerous studies have indicated that the reduced abundance of GM species and genes are the drivers of individual susceptibility to DILI (37, 52, 57–60). Microorganisms are enriched in genes encoding various enzymes which influence drug metabolism and increase the potential for liver injury (61, 62). Yip et al. (59) detected that Lactobacillus, Bacteroides, and Enterobacteriaceae, which would produce β-glucuronidase, were enriched in the strong responder groups (AST elevation ≥ 3 measurements) compared to the non-responder groups. Meanwhile, administration of β-glucuronidase to rats prior to administration of tacrine further confirmed it enhanced the potential hepatotoxicity induced by tacrine. In addition, host genes can also shape GM composition (35, 63). Previous studies have reported that modulation of key hepatic cytochrome enzymes expression results in individual differences in pharmacokinetics (64, 65). The expression of cyp3a11 was significantly higher in male mice than in female mice, whereas in mice lacking GM, the gender difference and cyp3a11 expression were approximately the same in both genders, indicating that gender differences and the composition/function of GM may importantly affect the patient’s response to drugs. Another microbiome-circadian rhythm study reported that circadian changes in GM could mediate different susceptibility to APAP-induced liver injury. Meanwhile, alcohol abuse would also aggravate the likelihood of DILI (4, 66). A recent review showed that alcoholic liver disease resulted in small intestinal bacterial overgrowth (SIBO), such as E. coli, and Enterococcus spp. (39). E. coli, Enterococcus spp. has been confirmed to generate 1-phenyl-1,2-propanedione (PPD), a microbial metabolite that could synergistically reinforce APAP-induced liver injury (37).
Apart from PPD, microbial metabolites such as bile acids, para–cresol, lysozyme, and lysophosphatide play pivotal roles in drug metabolism pathways in the liver (37, 57, 58, 67, 68). According to available studies, trigeminal, campesterol, and lithocholic acid varied significantly with the severity of liver injury (69). P-cresol produced by Clostridium difficile would increase toxicity by competing for the glutathione-dependent detoxification of phenolic drugs such as acetaminophen (68). In addition, abnormal metabolism of bile acids (BAs) would lead to decreased activation of nuclear receptors farnesoid X receptor (FXR) and TGR5 in ileal, thereby exacerbating hepatic steatosis and inflammation (70, 71).
The role of antibiotics in gut microbiota
DILI is one of the adverse drug events (ADEs) following inappropriate or appropriate use of antibiotics (72). Nevertheless, emerging evidence has suggested that the administration of antibiotics had both negative and positive influences on the initiation and progression of DILI (Table 2). Positive influences include that hepatotoxic drugs no longer induced liver damage in mice after the mice were pretreated with antibiotics, while further studies found that it may be because the GM that causes liver inflammation was removed (37, 57). Here, we preliminarily focus on the mechanisms underlying the negative effects of antibiotics treatment on DILI.
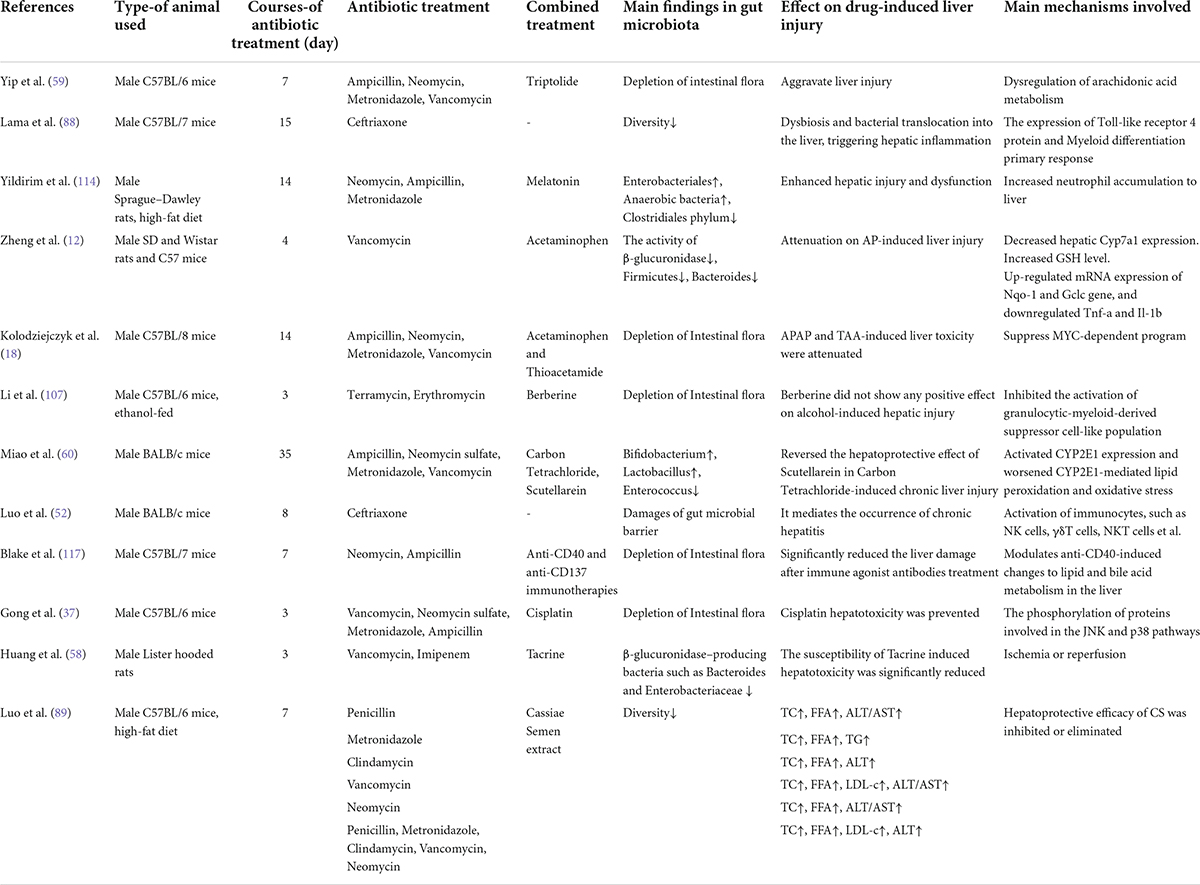
Table 2. List of main studies in animals associating antibiotics treatment and drug induced liver injury.
Since the discovery in 1950 that terramycin can affect the GM in human (73), there has been increasing evidence that antibiotics may cause dysbiosis through the reactive proliferation of potentially pathogenic microorganisms, depletion of beneficial bacteria, loss of α-diversity, and leakage of gut activities (73). Dysbiosis causes irreversible variation and functional impairment of GM at the gene or protein level, leading to disturbances of the immune system in intestinal epithelial cells, ultimately affecting hepatic metabolism (74) (Figure 1). However, it must be emphasized that some antibiotics had effects on intestinal barrier function and resulted in major changes in the microbiome, but not all, for example, metronidazole treatment had no effect on the microbiota (75). Due to different classes of antibiotics and individual responses, these effects could leave a few days or permanent imprints in the intestinal environment (76, 77), which might explain the significant difference in the onset time and course of various antibiotic-mediated liver injury.
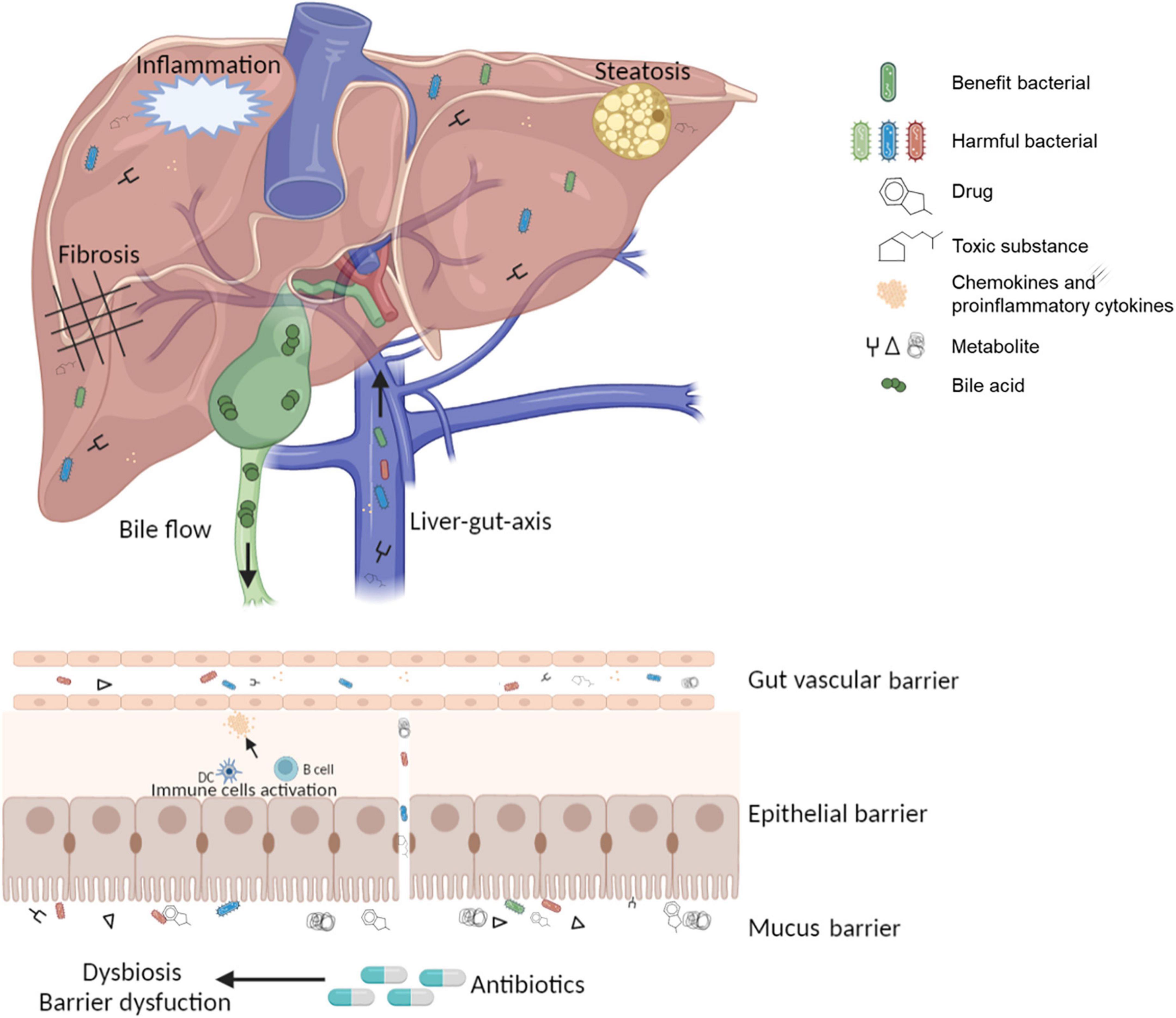
Figure 1. The pathological state of the gut-liver-immune axis and gut microbiota under antibiotic treatment.
Several human cohort studies have demonstrated the association between antibiotics use and changes in microbial composition and function (29, 78). Antibiotic therapy aims to eliminate pathogenic bacteria, however, this microbial clearance will also reduce the beneficial commensal bacteria, which has important pathological implications for the liver (36, 79). β-lactam antibiotics and the antibiotics cocktails were observed to increase the Bacteroidetes/Firmicutes ratio and decrease the microbial diversity. In particular, the abundance of Firmicutes and Actinobacteria (31, 60, 80), which contained bacteria that played an important role in reducing oxidative stress, inflammation, and liver-protective properties, such as Bifidobacterium and Lactobacillus (81–83). Noteworthy, antibiotics also increase the abundance of pathogenic bacteria, such as Enterococcus, which may exacerbate the progression of liver diseases (84).
Moreover, a handful of studies have proposed that flora changes could also alter the distribution of bacterial metabolites (85). For example, ciprofloxacin, a novel quinolone antibiotic with antimicrobial activity against lithocholic acid-producing bacteria, might lead to a reduction of lithocholic acid in the liver, thereby reducing the expression of the hepatic drug-metabolizing enzyme CYP3A (86).
Antibiotics can lead to impairment of the intestinal biological barrier and affect intestinal permeability. Ceftriaxone and ciprofloxacin have been proven to cause severe damage and histomorphological changes in the intestinal villus wall in an animal model (87). Expression of Toll-like receptor 4 protein and Myeloid differentiation primary response (Myd) 88 mRNA, which activated the NF-κB signaling pathway, was increased in both gut and liver after ceftriaxone treatment (88). In a separate study (89), lower expression of occludin and occludens-1 (ZO-1) mRNA in the ileum, which was the most critical component in tight junction proteins and functional organization to protect intestinal barrier permeability and epithelial integrity, was seen in different groups treated with ampicillin, vancomycin, neomycin, metronidazole, and mixtures of them (90–92).
On the other hand, the leakage of the gut activates the overexpression of nitric oxide (NO) synthesis (39, 93). NO induce the enhancement of tubulin nitration and oxidation, leading to further disruption of the barrier function by the microtubule cytoskeleton. In addition, increased NO synthesis leads to oxidative stress in hepatocytes (94). Numerous studies have demonstrated that lipopolysaccharide (LPS) spilled into the systemic circulation through the permeable intestine, resulting in hepatic immune activation (95).
Another related concept is colonization resistance, which plays an important role in preventing pathogen colonization and protecting intestinal function (96). Some animal and human studies have suggested that antibiotics could sabotage this ability (97, 98). However, how this destruction affects the occurrence of liver diseases is not completely understood.
Administration of antibiotics as a factor in drug-induced liver injury
Sole antibiotic treatment
A couple of studies have highlighted the association between antibiotics and DILI by affecting GM. Luo et al. (52) have documented that ceftriaxone significantly reduced GM diversity, increased the levels of pathogenic bacteria such as Firmicutes, Tendericutes, and Vibrio bacteria, caused damage to intestinal barrier, promoted the expression of LPS, and activated liver lymphocytes. The H&E staining of the liver showed hepatic steatosis and hepatitis. And the expression levels of ALT, AST, IL-6, and TNF- α in serum increased. These findings were consistent with similar findings in other studies of different classes of antibiotics (88, 99). In another study (80), it was confirmed that various antibiotic combinations had different effects on host BA metabolism. In particular, a stronger effect was observed in combination of two antibiotics than in single antibiotics.
Antibiotics combined with chemical agents
To date, there are not enough studies showing that the combination of antibiotics and chemical agents might lead to potential DDIs and impact the development of DILI (100, 101). Yoo et al. (102) indicated that antibiotic treatment of patients taking lovastatin might lead to adverse pharmacokinetic effects by suppressing GM. The failure of plasma cholesterol control exerted an influence on hepatic steatosis (103). Besides, hepatotoxic chemicals could also be exposed to the environment, and in an animal model, an antibiotics cocktail containing ampicillin, vancomycin, neomycin, and metronidazole increased polychlorinated biphenyls-induced inflammation but decreased hepatic fibrosis (91).
Antibiotics combined with herbal agents
Similarly, the disturbance of GM by antibiotics also modulated the susceptibility to natural compounds-induced transaminitis (33, 104). Clearance of GM before Triptolide treatment could increase bile acids and long-chain fatty acids in plasma and liver (58). The accumulation of bile acids may lead to necrosis and apoptosis of hepatocytes, and stimulate the release of TNF-α, IL-6 and IL-8 (105, 106). The study also found that the mRNA levels of inflammatory indicators in the liver were significantly elevated in the TP + antibiotic group, but downregulated in the antibiotics alone group, which has identified the risk of TP and its preparations administrated in combination with antibiotics. However, co-administration with propionate almost eliminated this inflammatory response.
Another hypothesis was that antibiotics interfered with GM and significantly reduced or even reversed the hepatoprotective effects of other drugs. Berberine did not show any positive effect on alcohol-induced liver injury in an antibiotic molded pseudo-germ-free (PGF) mouse model (107). Scutellarein was a herbal flavonoid thought to have hepatoprotective potential (108), however, Miao et al. (60) confirmed that when scutellarein was used in combination with antibiotics, it activated IκBα/NF-κB pathway, CYP2E1 expression, and aggravated CYP2E1-mediated lipid peroxidation and oxidative stress through intestinal ecosystem disorder (60), which was consistent with another study (89). The hepatoprotective effects of Cassiae Semen on mice were weakened or eliminated in different classes of antibiotics groups. The antibiotic-induced liver injury needs to be vigilant, especially during hepatoprotective therapy.
Recommendation on the safety of combination therapy: Perspective of gut microbiota
RUCAM, as a well-established diagnostic scale, can accurately assess the case of iDILI through the well-described clinical characteristics. However, the variability of clinical characteristics of iDILI, the difficulty in performing rigorous mechanistic studies in humans, and the lack of an animal model of experimental iDILI that can mimic the genetic requirements of human iDILI, make it impossible to obtain satisfactory and specific biomarkers for individuals with iDILI. The changes of different antibiotics on different GM provide a new idea and method for the experimental animal model, which can imitate the genetic requirements of iDILI patients. Collecting biological samples such as feces from iDILI patients for omics analysis is the most common and meaningful means to identify biomarkers, but the screening of the iDILI cases must be careful. Further investigations in patients with idiosyncratic DILI with high causality gradings based on the use of RUCAM is an integral evaluating mechanistic step (109).
Antibiotics aggravate the susceptibility to DILI by causing dysbiosis and barrier dysfunction affecting the disposal and action of other drugs (25). For liver injury induced by drug combination, DDIs should be explored, and the detailed mechanism could help prevent unexpected accidents and determine appropriate diagnosis and treatment. The drug combination therapy might cause DDIs through the regulation of drug metabolic enzymes and drug efflux pumps. However, when drugs were used in combination with antibiotics, subsequent changes in xenobiotics metabolism mediated by gut microbial enzymes would occur (110). Similarly, even if primary antibiotic treatment was safe, increased therapy might also cause liver inflammation (59), which suggested that follow-up of liver function test was essential during the administration of drugs such as antibiotics that affect intestinal microorganisms and their metabolic activities. In addition, we recommended that avoiding the risk for liver injury should be considered when establishing individual therapy, including the nature, duration and intervention time of antibiotics, which would affect the efficacy and toxicity of drugs.
Although some drugs have surprising curative effects, they also have the risk of inducing the outbreak of liver failure (57, 111). Altering the GM to reconstruct the hepatotoxicity of drugs by exhausting harmful bacteria, and supplementing probiotics/prebiotics or fecal microbiota transplantation are therapeutically potential. Monitoring the composition and metabolic activity of GM can provide a new target for early diagnosis or prevention and treatment strategies for DILI (112). Antibiotics are the most significant microbiome-targeted drugs to alleviate drug-induced liver failure (18). Pharmacological mechanisms include reducing bacterial density, eliminating target harmful bacteria, inhibiting secondary bacterial proliferation, and reducing bacterial translocation. But the appropriate target remains an unknown problem, which can be accomplished in multiomic integration, including combining transcriptomics, genomics, and metabolomics. Disappointingly, most studies used antibiotic mixtures or broad-spectrum antibiotics to deplete all GM, which could only explain the causal relationship between GM and DILI. Since the GM have been proven to have a protective effect on a variety of diseases, whether it could target specific flora had little practical significance for the prevention and treatment of DILI. Therefore, the therapeutic significance of “sterilization” to human health needs to be further explored. And the choice of antibiotics is also crucial. The effects of antibiotics on the overall composition of the microbiome and the downstream effects on the microbiome and host should be clarified in research. Another potential therapeutic approach is probiotics/prebiotics, for instance, Myxophilic bacteria has potential therapeutic value to reduce oxidative stress and inflammation in the liver by modulating GM composition and metabolic function, thereby alleviating APAP-induced liver injury (34).
Conclusion
In general, the heterogeneous response of drugs presents significant challenges for drug development and patient management. With the intention to establish a valid diagnosis, the use of a diagnostic scale such as RUCAM is recommended. Orally administered drugs may become toxic after being metabolized by GM before entering the liver. Combination with antibiotics leads to DDIs mediated by intestinal metabolism, suggesting that the possibility of hepatotoxicity caused by this combination should be vigilant. Other drugs metabolized by intestinal microbial enzymes may also produce similar antibiotic-induced pharmacokinetic effects. Therefore, a wider range of drugs need to be further studied (102). In the foreseeable future, the regulation of GM to improve treatment will ameliorate clinical practice.
Author contributions
LHF and YQG contributed to the concept and design of the study. LHF and YHQ wrote, edited, and reviewed of the manuscript. XNK and XHS revised the manuscript. ZS designed the tables and figures. All authors contributed to manuscript revision, read, and approved the submitted version.
Funding
This work was supported by the National Natural Science Foundation of China (grant nos. 82070633, 81874436 and 82074336 to XHS) and funds from the Science and Technology Committee of Shanghai (grant no. 20Z21900100).
Acknowledgments
The Figure 1 was modified from Biorender (https://app.biorender.com/).
Conflict of interest
The authors declare that the research was conducted in the absence of any commercial or financial relationships that could be construed as a potential conflict of interest.
Publisher’s note
All claims expressed in this article are solely those of the authors and do not necessarily represent those of their affiliated organizations, or those of the publisher, the editors and the reviewers. Any product that may be evaluated in this article, or claim that may be made by its manufacturer, is not guaranteed or endorsed by the publisher.
Supplementary material
The Supplementary Material for this article can be found online at: https://www.frontiersin.org/articles/10.3389/fmed.2022.972518/full#supplementary-material
References
1. Teschke R, Wolff A, Frenzel C, Schwarzenboeck A, Schulze J, Eickhoff A. Drug and herb induced liver injury: council for international organizations of medical sciences scale for causality assessment. World J Hepatol. (2014) 6:17–32. doi: 10.4254/wjh.v6.i1.17
2. Danan G, Teschke R. Rucam in drug and herb induced liver injury: the update. Int J Mol Sci. (2016) 17:14. doi: 10.3390/ijms17010014
3. Xiao X, Li X, Zhu Y, Wang J, Li L, Zhang T, et al. Clinical guidelines for diagnosis and treatment of liver injury related to chinese herbal medicine. J Clin Hepatol. (2016) 36:1165–72.
4. Devarbhavi H, Aithal G, Treeprasertsuk S, Takikawa H, Mao Y, Shasthry SM, et al. Drug-induced liver injury: Asia pacific association of study of liver consensus guidelines. Hepatol Int. (2021) 15:258–82. doi: 10.1007/s12072-021-10144-3
5. Andrade RJ, Aithal GP, Björnsson ES, Kaplowitz N, Kullak-Ublick GA, Larrey D, et al. Easl clinical practice guidelines: drug-induced liver injury. J Hepatol. (2019) 70:1222–61. doi: 10.1016/j.jhep.2019.02.014
6. Chalasani NP, Maddur H, Russo MW, Wong RJ, Reddy KR. Acg clinical guideline: diagnosis and management of idiosyncratic drug-induced liver injury. Am J Gastroenterol. (2021) 116:878–98. doi: 10.14309/ajg.0000000000001259
7. Li X, Tang J, Mao Y. Incidence and risk factors of drug−induced liver injury. Liver Int. (2022) 42:1999–2014. doi: 10.1111/liv.15262
8. De Valle MB, Av Klinteberg V, Alem N, Olsson R, Björnsson E. Drug−induced liver injury in a swedish university hospital out−patient hepatology clinic. Aliment Pharmacol Ther. (2006) 24:1187–95. doi: 10.1111/j.1365-2036.2006.03117.x
9. Björnsson ES, Bergmann OM, Björnsson HK, Kvaran RB, Olafsson S. Incidence, presentation, and outcomes in patients with drug-induced liver injury in the general population of iceland. Gastroenterology. (2013) 144:1419–25.e1–3. doi: 10.1053/j.gastro.2013.02.006
10. Gaynes R. The discovery of penicillin—new insights after more than 75 years of clinical use. Emerg Infect Dis. (2017) 23:849–53. doi: 10.3201/eid2305.161556
11. Singh V, Yeoh BS, Abokor AA, Golonka RM, Tian Y, Patterson AD, et al. Vancomycin prevents fermentable fiber-induced liver cancer in mice with dysbiotic gut microbiota. Gut Microbes. (2020) 11:1077–91. doi: 10.1080/19490976.2020.1743492
12. Zheng N, Gu Y, Hong Y, Sheng L, Chen L, Zhang F, et al. Vancomycin pretreatment attenuates acetaminophen-induced liver injury through 2-hydroxybutyric acid. J Pharm Anal. (2020) 10:560–70. doi: 10.1016/j.jpha.2019.11.003
13. Björnsson E, Olsson R. Outcome and prognostic markers in severe drug-induced liver disease. Hepatology. (2005) 42:481–9. doi: 10.1002/hep.20800
14. Hussaini SH, O’Brien CS, Despott EJ, Dalton HR. Antibiotic therapy: a major cause of drug-induced jaundice in Southwest England. Eur J Gastroenterol Hepatol. (2007) 19:15–20. doi: 10.1097/01.meg.0000250581.77865.68
15. Teschke R. Top-ranking drugs out of 3312 drug-induced liver injury cases evaluated by the roussel uclaf causality assessment method. Expert Opin Drug Metab Toxicol. (2018) 14:1169–87. doi: 10.1080/17425255.2018.1539077
16. Teschke R, Méndez-Sánchez N, Eickhoff A. Liver injury in covid-19 patients with drugs as causatives: a systematic review of 996 dili cases published 2020/2021 based on rucam as causality assessment method. Int J Mol Sci. (2022) 23:4828. doi: 10.3390/ijms23094828
17. Zhang J, Sun Y, Wang R, Zhang J. Gut microbiota-mediated drug-drug interaction between amoxicillin and aspirin. Sci Rep. (2019) 9:16194. doi: 10.1038/s41598-019-52632-5
18. Kolodziejczyk AA, Federici S, Zmora N, Mohapatra G, Dori-Bachash M, Hornstein S, et al. Acute liver failure is regulated by myc- and microbiome-dependent programs. Nat Med. (2020) 26:1899–911. doi: 10.1038/s41591-020-1102-2
19. Xie Y, Hu F, Xiang D, Lu H, Li W, Zhao A, et al. The metabolic effect of gut microbiota on drugs. Drug Metab Rev. (2020) 52:139–56. doi: 10.1080/03602532.2020.1718691
20. Zimmermann M, Zimmermann-Kogadeeva M, Wegmann R, Goodman AL. Separating host and microbiome contributions to drug pharmacokinetics and toxicity. Science. (2019) 363:eaat9931. doi: 10.1126/science.aat9931
21. O’Hara AM, Shanahan F. The gut flora as a forgotten organ. EMBO Rep. (2006) 7:688–93. doi: 10.1038/sj.embor.7400731
22. Lucena MI, Molokhia M, Shen Y, Urban TJ, Aithal GP, Andrade RJ, et al. Susceptibility to amoxicillin-clavulanate-induced liver injury is influenced by multiple hla class I and II alleles. Gastroenterology. (2011) 141:338–47. doi: 10.1053/j.gastro.2011.04.001
23. Cirulli ET, Nicoletti P, Abramson K, Andrade RJ, Bjornsson ES, Chalasani N, et al. A missense variant in Ptpn22 is a risk factor for drug-induced liver injury. Gastroenterology. (2019) 156:1707–1716.e2. doi: 10.1053/j.gastro.2019.01.034
24. Nicoletti P, Aithal GP, Chamberlain TC, Coulthard S, Alshabeeb M, Grove JI, et al. Drug-induced liver injury due to flucloxacillin: relevance of multiple human leukocyte antigen alleles. Clin Pharmacol Ther. (2019) 106:245–53. doi: 10.1002/cpt.1375
25. Weersma RK, Zhernakova A, Fu J. Interaction between drugs and the gut microbiome. Gut. (2020) 69:1510–9. doi: 10.1136/gutjnl-2019-320204
26. Human Microbiome Project Consortium. Structure, function and diversity of the healthy human microbiome. Nature. (2012) 486:207–14. doi: 10.1038/nature11234
27. Rinninella E, Cintoni M, Raoul P, Lopetuso LR, Scaldaferri F, Pulcini G, et al. Food components and dietary habits: keys for a healthy gut microbiota composition. Nutrients. (2019) 11:2393. doi: 10.3390/nu11102393
28. Rinninella E, Raoul P, Cintoni M, Franceschi F, Miggiano GA, Gasbarrini A, et al. What is the healthy gut microbiota composition? A changing ecosystem across age, environment, diet, and diseases. Microorganisms. (2019) 7:14. doi: 10.3390/microorganisms7010014
29. Vich Vila A, Collij V, Sanna S, Sinha T, Imhann F, Bourgonje AR, et al. Impact of commonly used drugs on the composition and metabolic function of the gut microbiota. Nat Commun. (2020) 11:362. doi: 10.1038/s41467-019-14177-z
30. Jourová L, Vavreckova M, Zemanova N, Anzenbacher P, Langova K, Hermanova P, et al. Gut microbiome alters the activity of liver cytochromes p450 in mice with sex-dependent differences. Front Pharmacol. (2020) 11:01303. doi: 10.3389/fphar.2020.01303
31. Singh K, Mohan M, Nautiyal S. Comparing cefixime, cefpodoxime and ofloxacin as anti-microbial agents and their effects on gut microbiota. Int J Sci Res Sci Technol. (2020) 7:131–52. doi: 10.32628/IJSRST207619
32. Coker MO, Hoen AG, Dade E, Lundgren S, Li Z, Wong AD, et al. Specific class of intrapartum antibiotics relates to maturation of the infant gut microbiota: a prospective cohort study. BJOG. (2020) 127:217–27. doi: 10.1111/1471-0528.15799
33. Conlon MA, Bird AR. The impact of diet and lifestyle on gut microbiota and human health. Nutrients. (2015) 7:17–44. doi: 10.3390/nu7010017
34. Xia J, Lv L, Liu B, Wang S, Zhang S, Wu Z, et al. Akkermansia muciniphila ameliorates acetaminophen-induced liver injury by regulating gut microbial composition and metabolism. Microbiol Spectrum. (2022) 10:e1596–1521. doi: 10.1128/spectrum.01596-21
35. Henao-Mejia J, Elinav E, Jin C, Hao L, Mehal WZ, Strowig T, et al. Inflammasome-mediated dysbiosis regulates progression of nafld and obesity. Nature. (2012) 482:179–85. doi: 10.1038/nature10809
36. Grąt M, Wronka KM, Krasnodębski M, Masior Ł, Lewandowski Z, Kosięska I, et al. Profile of gut microbiota associated with the presence of hepatocellular cancer in patients with liver cirrhosis. Transplant Proc. (2016) 48:1687–91. doi: 10.1016/j.transproceed.2016.01.077
37. Gong S, Lan T, Zeng L, Luo H, Yang X, Li N, et al. Gut microbiota mediates diurnal variation of acetaminophen induced acute liver injury in mice. J Hepatol. (2018) 69:51–9. doi: 10.1016/j.jhep.2018.02.024
38. Wang J, Guo X, Cao J, Zhang X, Zhang J, Sun D, et al. Prolonged pretreatment of mice with cholera toxin, but not isoproterenol, alleviates acute lethal systemic inflammatory response. Int Immunopharmacol. (2014) 23:60–5. doi: 10.1016/j.intimp.2014.07.035
39. Malaguarnera G, Giordano M, Nunnari G, Bertino G, Malaguarnera M. Gut microbiota in alcoholic liver disease: pathogenetic role and therapeutic perspectives. World J Gastroenterol. (2014) 20:16639–48. doi: 10.3748/wjg.v20.i44.16639
40. Konturek PC, Harsch IA, Konturek K, Schink M, Konturek T, Neurath MF, et al. Gut–liver axis: how do gut bacteria influence the liver? Med Sci. (2018) 6:79. doi: 10.3390/medsci6030079
41. Cesaro C, Tiso A, Del Prete A, Cariello R, Tuccillo C, Cotticelli G, et al. Gut microbiota and probiotics in chronic liver diseases. Dig Liver Dis. (2011) 43:431–8. doi: 10.1016/j.dld.2010.10.015
42. Albillos A, de Gottardi A, Rescigno M. The gut-liver axis in liver disease: pathophysiological basis for therapy. J Hepatol. (2020) 72:558–77. doi: 10.1016/j.jhep.2019.10.003
43. Derrien M, Van Baarlen P, Hooiveld G, Norin E, Muller M, de Vos W. Modulation of mucosal immune response, tolerance, and proliferation in mice colonized by the mucin-degrader akkermansia muciniphila. Front Microbiol. (2011) 2:166. doi: 10.3389/fmicb.2011.00166
44. Kho ZY, Lal SK. The human gut microbiome – a potential controller of wellness and disease. Front Microbiol. (2018) 9:1835. doi: 10.3389/fmicb.2018.01835
45. Kurashima Y, Kiyono H. Mucosal ecological network of epithelium and immune cells for gut homeostasis and tissue healing. Annu Rev Immunol. (2017) 35:119–47. doi: 10.1146/annurev-immunol-051116-052424
46. Brescia P, Rescigno M. The gut vascular barrier: a new player in the gut–liver–brain axis. Trends Mol Med. (2021) 27:844–55. doi: 10.1016/j.molmed.2021.06.007
47. Fouts DE, Torralba M, Nelson KE, Brenner DA, Schnabl B. Bacterial translocation and changes in the intestinal microbiome in mouse models of liver disease. J Hepatol. (2012) 56:1283–92. doi: 10.1016/j.jhep.2012.01.019
48. Sorribas M, de Gottardi A, Moghadamrad S, Hassan M, Spadoni I, Rescigno M, et al. Isoproterenol disrupts intestinal barriers activating gut-liver-axis: effects on intestinal mucus and vascular barrier as entry sites. Digestion. (2020) 101:717–29. doi: 10.1159/000502112
49. Yiu JHC, Dorweiler B, Woo CW. Interaction between gut microbiota and toll-like receptor: from immunity to metabolism. J Mol Med. (2017) 95:13–20. doi: 10.1007/s00109-016-1474-4
50. Tilg H, Moschen AR, Szabo G. Interleukin-1 and inflammasomes in alcoholic liver disease/acute alcoholic hepatitis and nonalcoholic fatty liver disease/nonalcoholic steatohepatitis. Hepatology. (2016) 64:955–65. doi: 10.1002/hep.28456
51. Cani PD, Bibiloni R, Knauf C, Neyrinck AM, Neyrinck AM, Delzenne NM, et al. Changes in gut microbiota control metabolic endotoxemia-induced inflammation in high-fat diet-induced obesity and diabetes in mice. Diabetes. (2008) 57:1470–81. doi: 10.2337/db07-1403
52. Luo X, Xu B, Xiong T, Su Y, Liu C, Ye Y, et al. Hepatic dysfunction induced by intestinal dysbacteriosis mainly manifests as immunologic abnormity in mice. Pathog Dis. (2020) 78:ftaa041. doi: 10.1093/femspd/ftaa041
53. Sorribas M, Jakob MO, Yilmaz B, Li H, Stutz D, Noser Y, et al. Fxr modulates the gut-vascular barrier by regulating the entry sites for bacterial translocation in experimental cirrhosis. J Hepatol. (2019) 71:1126–40. doi: 10.1016/j.jhep.2019.06.017
54. Luck H, Tsai S, Chung J, Clemente-Casares X, Ghazarian M, Revelo XS, et al. Regulation of obesity-related insulin resistance with gut anti-inflammatory agents. Cell Metab. (2015) 21:527–42. doi: 10.1016/j.cmet.2015.03.001
55. Cavaco MJ, Alcobia C, Oliveiros B, Mesquita LA, Carvalho A, Matos F, et al. Clinical and genetic risk factors for drug-induced liver injury associated with anti-tuberculosis treatment a study from patients of portuguese health centers. J Personal Med. (2022) 12:790. doi: 10.3390/jpm12050790
56. Lammert C, Imler T, Teal E, Chalasani N. Patients with chronic liver disease suggestive of nonalcoholic fatty liver disease may be at higher risk for drug-induced liver injury. Clin Gastroenterol Hepatol. (2019) 17:2814–5. doi: 10.1016/j.cgh.2018.12.013
57. Gong S, Feng Y, Zeng Y, Zhang H, Pan M, He F, et al. Gut microbiota accelerates cisplatin-induced acute liver injury associated with robust inflammation and oxidative stress in mice. J Transl Med. (2021) 19:147. doi: 10.1186/s12967-021-02814-5
58. Huang J-F, Zhao Q, Dai M-Y, Xiao X-R, Zhang T, Zhu W-F, et al. Gut microbiota protects from triptolide-induced hepatotoxicity: key role of propionate and its downstream signalling events. Pharmacol Res. (2020) 155:104752. doi: 10.1016/j.phrs.2020.104752
59. Yip LY, Aw CC, Lee SH, Hong YS, Ku HC, Xu WH, et al. The liver-gut microbiota axis modulates hepatotoxicity of tacrine in the rat. Hepatology. (2018) 67:282–95. doi: 10.1002/hep.29327
60. Miao Z, Lai Y, Zhao Y, Chen L, Zhou J, Li C, et al. Scutellarein aggravated carbon tetrachloride-induced chronic liver injury in gut microbiota-dysbiosis mice. Evid Based Complement Alternat Med. (2020) 2020:8811021. doi: 10.1155/2020/8811021
61. Arora T, Backhed F. The gut microbiota and metabolic disease: current understanding and future perspectives. J Intern Med. (2016) 280:339–49. doi: 10.1111/joim.12508
62. Colotti G, Rinaldi T. The central role of gut microbiota in drug metabolism and personalized medicine. Future Med Chem. (2020) 12:1197–200. doi: 10.4155/fmc-2020-0023
63. Duparc T, Plovier H, Marrachelli VG, Van Hul M, Essaghir A, Stahlman M, et al. Hepatocyte Myd88 affects bile acids, gut microbiota and metabolome contributing to regulate glucose and lipid metabolism. Gut. (2017) 66:620–32. doi: 10.1136/gutjnl-2015-310904
64. Wilson ID, Nicholson JK. Gut microbiome interactions with drug metabolism, efficacy, and toxicity. Transl Res. (2017) 179:204–22. doi: 10.1016/j.trsl.2016.08.002
65. Kuno T, Hirayama-Kurogi M, Ito S, Ohtsuki S. Effect of intestinal flora on protein expression of drug-metabolizing enzymes and transporters in the liver and kidney of germ-free and antibiotics-treated mice. Mol Pharmaceut. (2016) 13:2691–701. doi: 10.1021/acs.molpharmaceut.6b00259
66. Danan G, Benichou C. Causality assessment of adverse reactions to drugs .1. a novel method based on the conclusions of international consensus meetings - application to drug-induced liver injuries. J Clin Epidemiol. (1993) 46:1323–30. doi: 10.1016/0895-435690101-6
67. Tan Y, Li Y, Zhou F, Guo J, Wang T, Shi Y, et al. Administration of a mixture of triterpenoids from yeyachun and phenolic acids from danshen ameliorates carbon tetrachloride-induced liver fibrosis in mice by the regulation of intestinal flora. J Pharmacol Sci. (2020) 143:165–75. doi: 10.1016/j.jphs.2020.04.007
68. Dawson LF, Donahue EH, Cartman ST, Barton RH, Bundy J, McNerney R, et al. The analysis of para-cresol production and tolerance in clostridium difficile 027 and 012 strains. BMC Microbiol. (2011) 11:86. doi: 10.1186/1471-2180-11-86
69. Jiang H, Yan R, Wang K, Wang Q, Chen X, Chen L, et al. Lactobacillus reuteri Dsm 17938 alleviates D-galactosamine-induced liver failure in rats. Biomed Pharmacother. (2021) 133:111000. doi: 10.1016/j.biopha.2020.111000
70. Sinal CJ, Tohkin M, Miyata M, Ward JM, Lambert G, Gonzalez FJ. Targeted disruption of the nuclear receptor Fxr/Bar impairs bile acid and lipid homeostasis. Cell. (2000) 102:731–44. doi: 10.1016/S0092-867400062-3
71. de Oliveira MC, Gilglioni EH, de Boer BA, Runge JH, de Waart DR, Salgueiro CL, et al. Bile acid receptor agonists int747 and int777 decrease oestrogen deficiency-related postmenopausal obesity and hepatic steatosis in mice. BBA Mol Basis Dis. (2016) 1862:2054–62. doi: 10.1016/j.bbadis.2016.07.012
72. Mahana D, Trent CM, Kurtz ZD, Bokulich NA, Battaglia T, Chung J, et al. Antibiotic perturbation of the murine gut microbiome enhances the adiposity, insulin resistance, and liver disease associated with high-fat diet. Genome Med. (2016) 8:48. doi: 10.1186/s13073-016-0297-9
73. Dicaprio JM, Rantz LA. Effects of terramycin on the bacterial flora of the bowel in man. AMA Arch Intern Med. (1950) 86:649–57. doi: 10.1001/archinte.1950.00230170002001
74. Yoon MY, Yoon SS. Disruption of the gut ecosystem by antibiotics. Yonsei Med J. (2018) 59:4–12. doi: 10.3349/ymj.2018.59.1.4
75. Tulstrup MV-L, Christensen EG, Carvalho V, Linninge C, Ahrne S, Hojberg O, et al. Antibiotic treatment affects intestinal permeability and gut microbial composition in wistar rats dependent on antibiotic class. PLoS One. (2015) 10:e0144854. doi: 10.1371/journal.pone.0144854
76. Zimmermann P, Curtis N. The effect of antibiotics on the composition of the intestinal microbiota - a systematic review. J Infect. (2019) 79:471–89. doi: 10.1016/j.jinf.2019.10.008
77. Dethlefsen L, Relman DA. Incomplete recovery and individualized responses of the human distal gut microbiota to repeated antibiotic perturbation. Proc Natl Acad Sci. (2011) 108(Suppl 1.):4554–61. doi: 10.1073/pnas.1000087107
78. Jackson MA, Verdi S, Maxan M-E, Shin CM, Zierer J, Bowyer RCE, et al. Gut microbiota associations with common diseases and prescription medications in a population-based cohort. Nat Commun. (2018) 9:2655. doi: 10.1038/s41467-018-05184-7
79. Xue L, He J, Gao N, Lu X, Li M, Wu X, et al. Probiotics may delay the progression of nonalcoholic fatty liver disease by restoring the gut microbiota structure and improving intestinal endotoxemia. Sci Rep. (2017) 7:45176. doi: 10.1038/srep45176
80. Zhang Y, Limaye PB, Renaud HJ, Klaassen CD. Effect of various antibiotics on modulation of intestinal microbiota and bile acid profile in mice. Toxicol Appl Pharmacol. (2014) 277:138–45. doi: 10.1016/j.taap.2014.03.009
81. Ma S, Sun Y, Zheng X, Yang Y. Gastrodin attenuates perfluorooctanoic acid-induced liver injury by regulating gut microbiota composition in mice. Bioengineered. (2021) 12:11546–56. doi: 10.1080/21655979.2021.2009966
82. Cheng M, Zhang X, Miao Y, Cao J, Wu Z, Weng P. The modulatory effect of (-)-epigallocatechin 3-o-(3-o-methyl) gallate (egcg3”me) on intestinal microbiota of high fat diet-induced obesity mice model. Food Res Int. (2017) 92:9–16. doi: 10.1016/j.foodres.2016.12.008
83. Kato K, Nagao M, Miyamoto K, Oka K, Takahashi M, Yamamoto M, et al. Longitudinal analysis of the intestinal microbiota in liver transplantation. Transplant Direct. (2017) 3:e144. doi: 10.1097/TXD.0000000000000661
84. Zhang H-L, Yu L-X, Yang W, Tang L, Lin Y, Wu H, et al. Profound impact of gut homeostasis on chemically-induced pro-tumorigenic inflammation and hepatocarcinogenesis in rats. J Hepatol. (2012) 57:803–12. doi: 10.1016/j.jhep.2012.06.011
85. Cho CE, Taesuwan S, Malysheva OV, Bender E, Tulchinsky NF, Yan J, et al. Trimethylamine-N-Oxide (Tmao) response to animal source foods varies among healthy young men and is influenced by their gut microbiota composition: a randomized controlled trial. Mol Nutr Food Res. (2017) 61:1600324. doi: 10.1002/mnfr.201600324
86. Toda T, Ohi K, Kudo T, Yoshida T, Ikarashi N, Ito K, et al. Ciprofloxacin suppresses Cyp3a in mouse liver by reducing lithocholic acid-producing intestinal flora. Drug Metab Pharmacokinet. (2009) 24:201–8. doi: 10.2133/dmpk.24.201
87. Chen Z, Xie J, Wang B, Tang J. Effect of Γ-aminobutyric acid on digestive enzymes, absorption function, and immune function of intestinal mucosa in heat-stressed chicken1 1this work is supported by research grants from national natural science foundation of China (Nsfc31060312; 31260555, Beijing). Poult Sci. (2014) 93:2490–500. doi: 10.3382/ps.2013-03398
88. Lama A, Annunziata C, Coretti L, Pirozzi C, Di Guida F, Izzo AN, et al. N-(1-Carbamoy1-2-Phenylethyl) butyramide reduces antibiotic-induced intestinal injury, innate immune activation and modulates microbiota composition. Sci Rep. (2019) 9:4832. doi: 10.1038/s41598-019-41295-x
89. Luo H, Wu H, Wang L, Xiao S, Lu Y, Liu C, et al. Hepatoprotective effects of cassiae semen on mice with non-alcoholic fatty liver disease based on gut microbiota. Commun Biol. (2021) 4:1357. doi: 10.1038/s42003-021-02883-8
90. Robinson K, Deng Z, Hou Y, Zhang G. Regulation of the intestinal barrier function by host defense peptides. Front Vet Sci. (2015) 2:57. doi: 10.3389/fvets.2015.00057
91. Su H, Liu J, Wu G, Long Z, Fan J, Xu Z, et al. Homeostasis of gut microbiota protects against polychlorinated biphenyl 126-induced metabolic dysfunction in liver of mice. Sci Total Environ. (2020) 720:137597. doi: 10.1016/j.scitotenv.2020.137597
92. Suzuki T. Regulation of intestinal epithelial permeability by tight junctions. Cell Mol Life Sci. (2013) 70:631–59. doi: 10.1007/s00018-012-1070-x
93. Ma TY, Iwamoto GK, Hoa NT, Akotia V, Pedram A, Boivin MA, et al. Tnf-A -induced increase in intestinal epithelial tight junction permeability requires Nf-K b activation. Am J Physiol Gastrointest Liver Physiol. (2004) 286:G367–76. doi: 10.1152/ajpgi.00173.2003
94. Banan A, Zhang LJ, Shaikh M, Fields JZ, Farhadi A, Keshavarzian A. Inhibition of oxidant-induced nuclear factor-K b activation and inhibitory-K bα degradation and instability of F-Actin cytoskeletal dynamics and barrier function by epidermal growth factor: key role of phospholipase-Γ isoform. J Pharmacol Exp Ther. (2004) 309:356–68. doi: 10.1124/jpet.103.062232
95. Pinzone MR, Celesia BM, Di Rosa M, Cacopardo B, Nunnari G. Microbial translocation in chronic liver diseases. Int J Microbiol. (2012) 2012:694629. doi: 10.1155/2012/694629
96. Vollaard EJ, Clasener HA. Colonization resistance. Antimicrob Agents Chemother. (1994) 38:409–14. doi: 10.1128/AAC.38.3.409
97. Vanderwaaij D, Devrieshospers HG, Welling GW. The influence of antibiotics on gut colonization. J Antimicrob Chemother. (1986) 18:155–8. doi: 10.1093/jac/18.Supplement_C.155
98. Schubert AM, Sinani H, Schloss PD. Antibiotic-induced alterations of the murine gut microbiota and subsequent effects on colonization resistance against clostridium difficile. MBIO. (2015) 6:e00974. doi: 10.1128/mBio.00974-15
99. Jena PK, Sheng L, Liu H-X, Kalanetra KM, Mirsoian A, Murphy WJ, et al. Western diet–induced dysbiosis in farnesoid x receptor knockout mice causes persistent hepatic inflammation after antibiotic treatment. Am J Pathol. (2017) 187:1800–13. doi: 10.1016/j.ajpath.2017.04.019
100. Ning C, Su S, Li J, Kong D, Cai H, Qin Z, et al. Evaluation of a clinically relevant drug-drug interaction between rosuvastatin and clopidogrel and the risk of hepatotoxicity. Front Pharmacol. (2021) 12:715577. doi: 10.3389/fphar.2021.715577
101. Li J, Chen B, Xi W-Q, Jia W, Zhang W-X, Bian X-L. Drug-drug interactions and disease status are associated with irinotecan-induced hepatotoxicity: a cross-sectional study in Shanghai. J Clin Pharmacol. (2022) 62:1160–9. doi: 10.1002/jcph.2059
102. Yoo D-H, Kim IS, Thi Kim Van L, Jung I-H, Yoo HH, Kim D-H. Gut microbiota-mediated drug interactions between lovastatin and antibiotics. Drug Metab Dispos. (2014) 42:1508–13. doi: 10.1124/dmd.114.058354
103. Razmpour F, Mohammadi M, Ramezani-jolfaie N, Razmpour M, Javdan G. Association of hypercholesterolemia or hypertriglyceridemia with degree of hepatic steatosis in patients with non-alcoholic fatty liver disease. Clin Nutr ESPEN. (2021) 46:S603. doi: 10.1016/j.clnesp.2021.09.173
104. Chen F, Wen Q, Jiang J, Li H-L, Tan Y-F, Li Y-H, et al. Could the gut microbiota reconcile the oral bioavailability conundrum of traditional herbs? J Ethnopharmacol. (2016) 179:253–64. doi: 10.1016/j.jep.2015.12.031
105. Perez MJ, Briz O. Bile-acid-induced cell injury and protection. World J Gastroenterol. (2009) 15:1677–89. doi: 10.3748/wjg.15.1677
106. Krishnan S, Ding Y, Saedi N, Choi M, Sridharan GV, Sherr DH, et al. Gut microbiota-derived tryptophan metabolites modulate inflammatory response in hepatocytes and macrophages. Cell Rep. (2018) 23:1099–111. doi: 10.1016/j.celrep.2018.03.109
107. Li S, Wang N, Tan H-Y, Chueng F, Zhang Z-J, Yuen M-F, et al. Modulation of Gut Microbiota Mediates Berberine-Induced Expansion of Immuno-Suppressive Cells to against Alcoholic Liver Disease. Clin Transl Med. (2020) 10:e112. doi: 10.1002/ctm2.112
108. Wang L, Ma Q. Clinical Benefits and Pharmacology of Scutellarin: A Comprehensive Review. Pharmacol Ther. (2018) 190:105–27. doi: 10.1016/j.pharmthera.2018.05.006
109. Teschke R, Uetrecht J. Mechanism of Idiosyncratic Drug Induced Liver Injury (Dili): Unresolved Basic Issues. Ann Trans Med. (2021) 9:730. doi: 10.21037/atm-2020-ubih-05
110. Yoo HH, Kim IS, Yoo D-H, Kim D-H. Effects of Orally Administered Antibiotics on the Bioavailability of Amlodipine: Gutmicrobiota-Mediated Drug Interaction. J Hypertens. (2016) 34:156–62. doi: 10.1097/HJH.0000000000000773
111. Bernal W, Lee WM, Wendon J, Larsen FS, Williams R. Acute Liver Failure: A Curable Disease by 2024? J Hepatol. (2015) 62(1 Suppl.):S112–20. doi: 10.1016/j.jhep.2014.12.016
112. Chen T, Ding R, Chen X, Lu Y, Shi J, Lu Y, et al. Firmicutes and Blautia in Gut Microbiota Lessened in Chronic Liver Diseases and Hepatocellular Carcinoma Patients: A Pilot Study. Bioengineered. (2021) 12:8233–46. doi: 10.1080/21655979.2021.1982273
113. Clayton TA, Baker D, Lindon John C, Everett Jeremy R, Nicholson Jeremy K. Pharmacometabonomic Identification of a Significant Host-Microbiome Metabolic Interaction Affecting Human Drug Metabolism. Proc Natl Acad Sci. (2009) 106:14728–33. doi: 10.1073/pnas.0904489106
114. Yildirim A, Tamer SA, Sahin D, Bagriacik F, Kahraman MM, Onur ND, et al. The Effects of Antibiotics and Melatonin on Hepato-Intestinal Inflammation and Gut Microbial Dysbiosis Induced by a Short-Term High-Fat Diet Consumption in Rats. Br J Nutr. (2019) 122:841–55. doi: 10.1017/S0007114519001466
115. Sun J, Zhao F, Lin B, Feng J, Wu X, Liu Y, et al. Gut Microbiota Participates in Antithyroid Drug Induced Liver Injury through the Lipopolysaccharide Related Signaling Pathway. Front Pharmacol. (2020) 11:598170. doi: 10.3389/fphar.2020.598170
116. Yin R, Liu S, Jiang X, Zhang X, Wei F, Hu J. The Qingchangligan Formula Alleviates Acute Liver Failure by Regulating Galactose Metabolism and Gut Microbiota. Front Cell Infect Microbiol. (2022) 11:771483. doi: 10.3389/fcimb.2021.771483
Keywords: gut microbiota, drug-induced liver injury, antibiotics, gut liver axis, probiotics
Citation: Fu LH, Qian YH, Shang Z, Sun XH, Kong XN and Gao YQ (2022) Antibiotics enhancing drug-induced liver injury assessed for causality using Roussel Uclaf Causality Assessment Method: Emerging role of gut microbiota dysbiosis. Front. Med. 9:972518. doi: 10.3389/fmed.2022.972518
Received: 18 June 2022; Accepted: 22 August 2022;
Published: 09 September 2022.
Edited by:
Guoxun Chen, The University of Tennessee, Knoxville, United StatesReviewed by:
Yiling Li, The First Affiliated Hospital of China Medical University, ChinaRolf Teschke, Hospital Hanau, Germany
Copyright © 2022 Fu, Qian, Shang, Sun, Kong and Gao. This is an open-access article distributed under the terms of the Creative Commons Attribution License (CC BY). The use, distribution or reproduction in other forums is permitted, provided the original author(s) and the copyright owner(s) are credited and that the original publication in this journal is cited, in accordance with accepted academic practice. No use, distribution or reproduction is permitted which does not comply with these terms.
*Correspondence: Xiaoni Kong, xiaonikong@shutcm.edu.cn; Yueqiu Gao, gaoyueqiu@hotmail.com
†These authors have contributed equally to this work and share first authorship
‡These authors have contributed equally to this work