- 1Alliance for Vascular Access Teaching and Research Group, Nathan, QLD, Australia
- 2School of Nursing, Midwifery and Social Work, The University of Queensland, St Lucia, QLD, Australia
- 3Metro North Health, Herston Infectious Disease Institute, Herston, QLD, Australia
- 4School of Information and Communication Technology, Griffith University, Nathan, QLD, Australia
- 5Digital Metro North, Metro North Hospital and Health Service, Herston, QLD, Australia
- 6Centre for Health Services Research, Faculty of Medicine, University of Queensland, Herston, QLD, Australia
- 7Digital Solutions, Griffith University, Nathan, QLD, Australia
- 8School of Nursing and Midwifery, Menzies Health Institute Queensland, Griffith University, Nathan, QLD, Australia
- 9Division of Surgery, Princess Alexandra Hospital, Brisbane, QLD, Australia
- 10Nursing and Midwifery Research Centre, Royal Brisbane and Women’s Hospital, Brisbane, QLD, Australia
Background and significance: Intravascular (IV) catheters are the most invasive medical device in healthcare. Localized priority-setting related to IV catheter quality surveillance is a key objective of recent healthcare reform in Australia. We sought to determine the plausibility of using electronic health record (EHR) data for catheter surveillance by mapping currently available data across state-wide platforms. This work has identified barriers and facilitators to a state-wide EHR surveillance initiative.
Materials and methods: Data variables were generated and mapped from routinely used EHR sources across Queensland, Australia through a systematic search of gray literature and expert consultation with clinical information specialists. EHR systems were eligible for inclusion if they collected data related to IV catheter insertion, care, or outcomes of hospitalized patients. Generated variables were mapped against international recommendations for IV catheter surveillance, with data linkage and data export capacity narratively summarized.
Results: We identified five EHR systems, namely, iEMR, MetaVision ICU®, Multiprac, RiskMan, and the Nephrology Registry. Systems were used across jurisdictions and hospital wards. Data linkage was not evident across systems. Extraction processes for catheter data were not standardized, lacking clear and reliable extraction techniques. In combination, EHR systems collected 43/50 international variables recommended for catheter surveillance, however, individual systems collected a median of 24/50 (IQR 22, 30) variables. We did not identify integrated clinical analytic systems (incorporating machine learning) to support clinical decision making or for risk stratification (e.g., catheter-related infection).
Conclusion: Current data linkage across EHR systems limits the development of an IV catheter quality surveillance system to provide timely data related to catheter complications and harm. To facilitate reliable and timely surveillance of catheter outcomes using clinical informatics, substantial work is needed to overcome existing barriers and transform health surveillance.
Introduction
Within the last decade, complications associated with intravascular (IV) catheters have increased in focus as new evidence reveals the burden of catheter failure in hospitalized patients (1, 2). Globally, IV catheters are the most prevalent invasive medical device used in healthcare (3, 4). Around 200 million catheters are used in the United States annually (5, 6) and approximately one in three United Kingdom inpatients have a peripheral IV catheter in situ (7, 8). Despite their widespread use, IV catheter-related complications are common and associated with increased clinical and economic burden, including increased mean hospitalization cost, protracted length of stay, and greater risk of death than patients without such complications (9–11).
Measurement of process, system, and outcome parameters related to IV catheters has been performed in hospitals using basic audit methods (12) with varied success for decades. This approach to quality surveillance is resource intensive and limits multijurisdictional benchmarking (13) and performance reporting (14) due to heterogeneity in items and case definitions (15). However, the reliability and validity of this data are becoming increasingly important with an increasing regulatory environment and persisting patient safety problems related to IV catheters. In 2021, the Australian Commission on Safety and Quality in Healthcare released a National Strategy to reduce the impact of IV catheter-related complications on hospitalized Australians. The clinical care standard sets out several quantifiable targets against which hospitals can measure quality and progress on IV catheter improvement initiatives. With the recent publication of international recommendations for vascular access surveillance (a minimum dataset; 16) there is an opportunity for jurisdictions to standardize IV catheter surveillance initiatives. This has led to health systems increasingly seeking platforms to routinely monitor patient and service-level outcomes and seek out new technologies to support enhanced healthcare quality and safety (17).
The broad adoption of electronic health records (EHRs) across the continuum of care has seen new opportunities arise to support health services monitor IV catheter quality. As a result, an increasing number of health professionals and researchers are now leveraging data sets from routine clinical care to improve health outcomes (18). However, the diversity and complexity of EHR data sets have created challenges, specifically in the collection and comparison of data items for effective analysis of quality and safety queries (19). Data linkage, interoperability, and heterogenous data items have been flagged as barriers to the implementation of standardized surveillance platforms in many health disciplines. However, these challenges have yet to be fully explored in IV catheter care provision (13), which crosses disciplines. An IV surveillance platform would depend largely on the fundamental structural design and utilization of relevant datasets (20, 21). Data captured on EHR platforms can yield powerful insights (17, 22, 23), such as evaluation of IV catheter quality improvement initiatives (24). Therefore, we sought to map, in an Australian case study, EHR data sources that collect indwelling IV catheter data to explore the benefits, challenges, and future directions of digitally enabled quality surveillance. Secondary aims include (1) comparing currently collected data variables against international recommendations for IV surveillance and monitoring; and (2) determining the current scope of clinical analytic frameworks integrated with EHRs related to IV catheters.
Materials and methods
Design
We conducted a scoping review and modified mapping study (25–27) to identify EHRs and IV data variables across Queensland Health systems. The main focus of these methods was classification by conducting thematic analysis. The search strategy included two components, a gray literature search and consultation with EMR experts, to answer the research questions (Supplementary material 1). The study was underpinned by the EHR Usability Evaluation and Use Case Framework by the Agency for Healthcare Research and Quality (28). Ethical approval was obtained from Griffith University (GU Ref: 2020/710).
Setting
The study was conducted in Queensland, Australia. Located in northeastern Australia, the State of Queensland has an estimated population of 5.16 million (29). Public health services in Queensland are managed by Queensland Health and are funded by both the state and federal governments. At the time of study undertaking, integrated electronic medical record (ieMR) had been successfully implemented in 16 of 30 Queensland Health facilities, with regular application updates slowly digitalizing the previous paper-based processes (30). All 16 hospitals use the same systems based on the initial iEMR configuration rolled out and piloted in a single Queensland site.
Search for gray literature
Our gray literature search comprised a (1) targeted search of known clinical datasets through government and healthcare agencies (national health agencies and safety and quality organizations), and (2) a Google search on the topic. For the latter, we adopted best practices for web-based searching in health research (31). EHRs were identified by key word searches including IV catheter, vascular access, central venous access device, peripherally inserted central catheter, healthcare-associated infection, central line associated bloodstream infection, and clinical/device/quality registry. The inclusion criteria were limited to studies that discussed EHRs. The broad search strategy was employed to help overcome anticipated barriers to locating data items including different data formats (different proprietary formats) and data linkage (variable scope of individual data sources and different data sources used within the health service; 31, 32). If further information was required to determine the eligibility of identified platform, the dataset manager was contacted via email correspondence. EHR eligibility criteria were: (1) currently utilized electronic or digital platform, and (2) minimum dataset included items related to indwelling IV catheter care, processes, or outcomes.
Consultation with clinical information experts
Following ethics approval and literature search results, we consulted with 12 clinical information specialists (CISs; 20 contacted; 60% response rate), to augment and validate mapped EHRs. We received input from platform owners (e.g., Ocean Health Systems, HammondCare), health services (e.g., Metro North Hospital and Health Service CISs), Health and research institutes, and specialist providers (e.g., the Vascular Access Surveillance Team, Infection Control, and Intensive Care Unit) with deep content expertise in IV catheters. Purposive sampling with snowballing was used with potential experts identified through investigator networks. Experts were contacted via email (maximum 2 emails) or via phone with a short request to provide feedback on a working list of EHRs and IV catheter data elements contained in each system. We asked them to help identify any gaps in electronic data sources that might collect statewide IV catheter data. Participants were contacted in November 2020 and requested to respond within a month. If participants did not respond, it was assumed that they declined to participate in the study. Data sources identified through expert consultation were reviewed independently by two investigators (DB and JS) with discrepancies resolved by a third investigator (CS).
Collating, summarizing, and reporting the results
All identified EHRs and data items were collated using a standardized data extraction form in Microsoft Excel®. We were interested in capturing broad features of EHRs including:
• EHR source host.
• Intravascular catheter data items and their definitions.
• Linkage capabilities.
The synthesis and classification scheme comprised the following steps: (1) enumeration of the number of EHRs and the number of items per EHR source; (2) presenting the visualization of the EHRs and data items included in the analysis; and (3) presenting a narrative summary of the principal findings (33). Finally collected IV catheter data items were mapped against international recommendations for IV catheter quality surveillance to determine alignment (see Supplementary material 2, republished with permission; 16).
Results
Mapping question 1. Which electronic health records collect intravascular catheter data across Queensland health?
We identified 5 EHRs, namely, ieMR, MetaVision ICU, Multiprac, RiskMan, and the Nephrology Registry. As no ongoing linkages existed between datasets, the analyses presented represent EHR-level reports for each separate system (Figure 1). The gray literature search identified three EHRs; consultation with the experts identified a further two. Table 1 outlines the EHRs, host organizations, data source features, and availability of data.
Mapping question 2. What intravascular catheter data are currently collected across electronic health records, and how do they align with international recommendations?
The number of IV catheter items collected across EHRs was limited and focused on device characteristics (e.g., device type, insertion site; Figure 2). Cerner applications collected the most data items (PowerChart 38/50; 76%) in line with the 50-item international recommendations. EHRs collected a median of 24/50 (IQR 22, 30) variables. MetaVision ICU collected 30/50 (60%). The Nephrology Registry dataset collected 24/50 (48%) variables, while Multiprac collected 21/50 (42%) and RiskMan collected 2/50 (4%). Collectively, 43 of the 50 recommended items were captured. Items not collected included: (i) pain relief, (ii) blood sampling, (iii) line fracture, (iv) replacement required, (v) internal malposition, and (vi) can the patient identify the reason for the device?
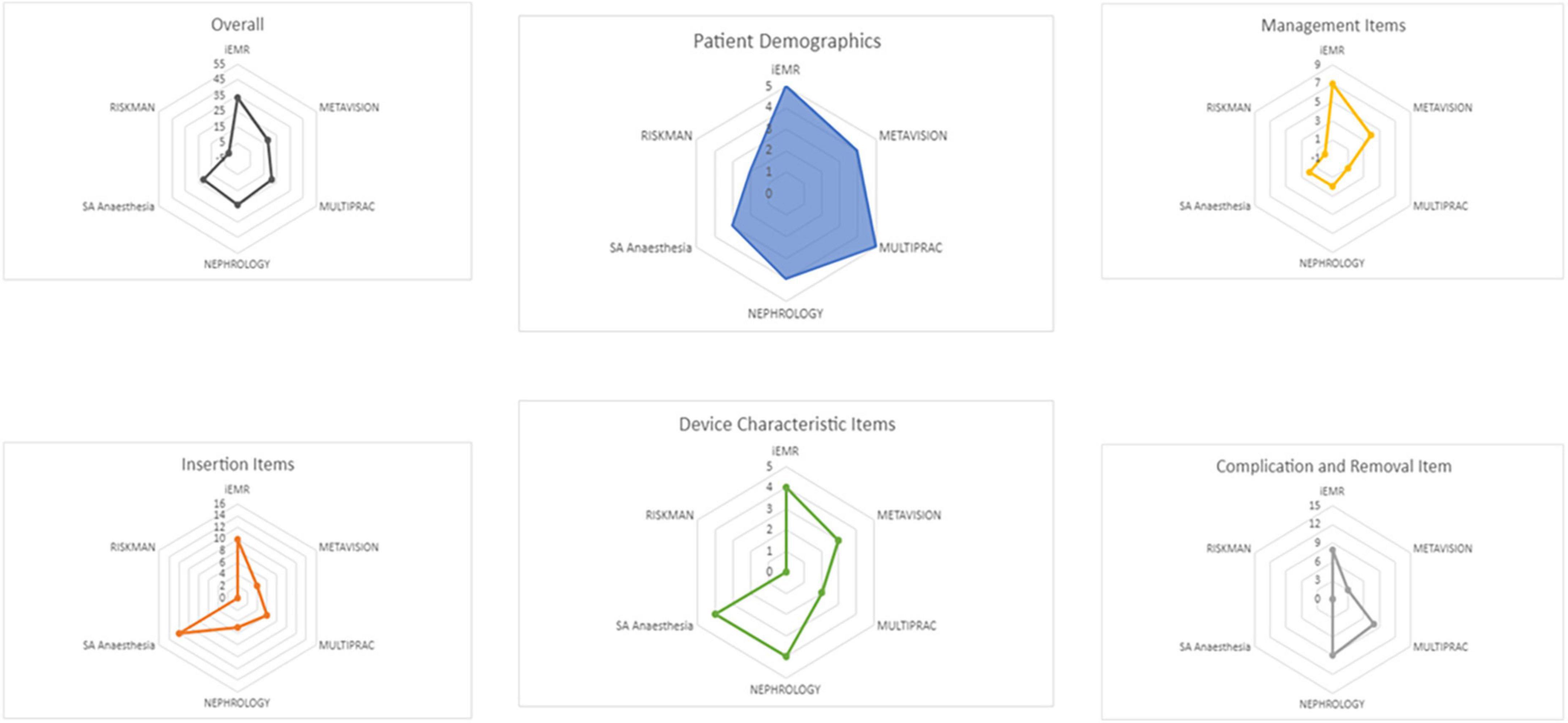
Figure 2. Intravascular catheter data items collected in Queensland electronic data sources compared to international recommendations.
Systematized Nomenclature of Medicine—Clinical Terms were embedded in ieMR and Multiprac, but not in MetaVision ICU, RiskMan, or Nephrology platforms. Few platforms had keyword search capacity for IV catheter, central venous access device, peripherally inserted central catheter, healthcare-associated infections, central line associated bloodstream infections, and/or clinical/device/quality registry. No EHR included mandatory fields (which a clinician must complete) related to IV catheter insertion, management, complications, or removal.
Mapping question 3. What clinical decision support systems are currently in use to support intravascular catheter surveillance and care?
We identified no clinical decision support systems for IV catheters within the included electronic data platforms.
Data sharing and linkage possibilities
All five EHRs were developed and are owned by separate enterprises (Cerner, iMDSoft, Ocean Healthcare Systems, HammondCare, Queensland Health). PowerChart, MetaVision ICU, RiskMan, and Multiprac systems currently link with the Hospital Based Corporate Information System (HBCIS), a statewide patient administration system that captures admitted and non-admitted patient, clinical, and administrative data. Multiprac additionally automatically draws positive bloodstream infection data from AUSLAB (statewide pathology system). The Nephrology Registry does not link to any systems within Queensland Health, as the platform was built on legacy database software that is no longer supported and is incompatible with the current version of Microsoft Windows software. As a result, clinicians must manually insert patient identifiers to track devices. At present, Cerner applications (ieMR) link with other Cerner applications within Queensland Health. MetaVision ICU (iMDSoft) links with HBCIS but not ieMR, meaning that ICU clinicians input replicated data into the Cerner platform, resulting in inefficiencies and duplication of clinical data for patients transitioning from ICU to the wards.
Data extraction and reporting capability
As per Figure 3, IV catheter data consistent with international recommendations can be extracted from all platforms, with the exception of RiskMan. The PowerChart (inpatient application) component of the ieMR automatically extracts IV catheter device and line data from SA Anesthesia into a procedure tab within the interactive view, however, it does not extract data into the lines and devices tab within Powerchart. Multiprac users tracking bloodstream infections can generate reports on positive bloodstream infection data for the organization, facility, specialty, unit, and ward. The data is then reportable currently via crystal reports, a Windows-based report writer solution allowing users to create specific reports, or as a downloaded Excel or portable digital file extract. Intensive care unit clinicians can track MetaVision ICU data through the structured query language server reporting service, which automatically extracts data from MetaVision ICU and generates a daily report for vascular access monitoring. Renal transplant clinicians can generate reports from the Nephrology Registry dataset through the SQL, which automatically extracts data for reporting. As RiskMan is a risk management and prevention database that relies on reporters entering data relating to vascular access devices into free text report fields, it is unable to generate reports.
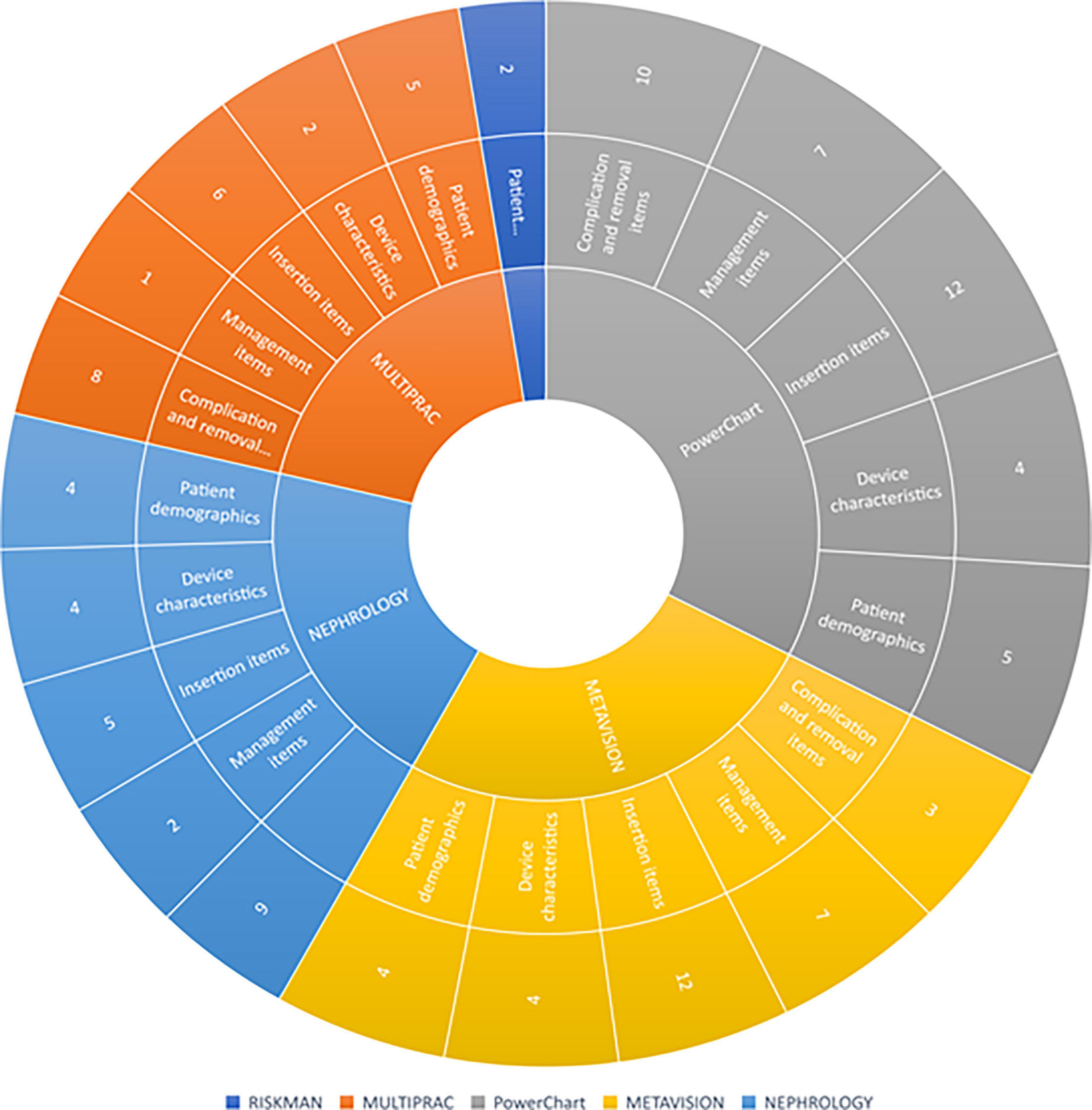
Figure 3. Sunburst diagram of vascular access items collected in Queensland Health electronic platforms. Each concentric circle represents a level of navigation from the top of the tree. In the majority of platforms, data items are displayed separately on different “pages” or “screens” from other items, requiring the user to view multiple screens to get a comprehensive clinical overview of vascular access items collected.
Discussion
In this scoping and mapping project of electronic surveillance capability for IV catheter usage, we identified five EHRs that capture IV catheter-related data across Queensland Health. Across all EHRs, 86% of internationally recommended quality surveillance items are collected, however, unlinked systems mean health jurisdictions are unable to utilize these systems to their maximum potential for catheter quality surveillance requirements. Importantly, patient reported experience measures were not captured across any EHR (e.g., can the patient identify the reason for the device; 14), a notable limitation of existing systems. Our findings confirm that despite the rapid digitalization of health care, many EHR systems are yet to reach their full potential to support hospital quality surveillance and proactive monitoring of IV catheter care. The effects of catheter quality improvement and harm prevention initiatives should be measured using data derived from reliable, standardized EHR surveillance programs. Such an EHR could support the development of clinical decision support tools for vascular access, and the testing of algorithms with the use of real-time clinical data (34).
A key finding of this study was that semantic interoperability (the ability of systems to exchange data with a shared meaning) will be a challenge for health systems in the future when integrating IV catheter data across EHRs (35). Different platforms and terminologies for expressing the same data entry (e.g., one type of catheter complication was variously described as puffy, leaking, infiltration, or extravasation) limit the aggregation and extraction capacity of patient data. Although reflective of the Queensland landscape, our findings are consistent with national and international reports, which highlight a “mismatch” in data terminology and interactions in the healthcare context (13, 36). This problem is not unique to IV catheters. It is likely that in time, programmers will adapt to existing platforms, addressing issues such as data fragmentation and ineffective interaction or display features to reflect clinical assumptions and workflows (37, 38). However, for now, this finding can be interpreted as a barrier to the exportation and use of clinical data to inform clinical decision support tools and improve the quality of vascular access care.
The potential of clinical analytics and support decision making in the context of IV catheter care is yet to be realized. We are yet to take advantage of emerging technologies (intelligent learning systems and trial-integrated clinical decision support software) to stratify patients’ risk of IV catheter complications (e.g., blood-stream infection). While this limitation is largely due to the feasibility of integrating this technology with current systems, progressing this advanced clinical technology would require improved data linkage and data exportation processes across EHR systems. An increasing number of researchers are developing predictive models for catheter complications (39–41), however, the practical application and value of machine learning models to reduce IV catheter complications and adverse health outcomes are yet to be demonstrated. Several international institutions (42, 43) have created IV catheter databases for a variety purposes such as risk factor analysis, health economic evaluations, and hospital-specific information (outcomes; 44, 45). Yet in Australia, HER surveillance and clinical analytic functionalities in the context of IV catheter care are yet to be fully explored. A limitation that likely stems from the variation in uptake of EHRs to measure quality and safety on a larger health service scale (46).
Intravascular catheters are a vital device to facilitate treatment, meaning the quality of care that patients receive is an important factor affecting rates of hospital-acquired complications and infection (47). Quality standards for vascular access care exist, but at present we have limited ability to evaluate or benchmark how safe vascular access care is. The implementation of standardized IV catheter surveillance will be challenging, improving data linkage capabilities between all current systems will be costly but would increase the power to detect associations in clinical characteristics and risks. Further improved interoperability and item standardization will result in increased efficiencies which will facilitate easier, more reliable reporting and analysis of clinical and surveillance data (14). This information can be used to inform targeted catheter improvement programs, which will in turn improve patient outcomes and decrease healthcare utilization and costs.
Implications for clinical practice and future research
We identified no clinical decision support systems working alongside or integrated with electronic platforms to support complex decision-making related to IV catheter care. This is a notable omission in the current healthcare infrastructure that future research and quality improvement efforts could seek to address, given their adoption and demonstrated benefit in other disciplines (48). In future years, clinicians and clinical informaticians will need to address fragmented ontologies and complicated exportation algorithms to make use of the currently collected data to facilitate better quality surveillance and improvement initiatives.
Limitations and strengths
This study has limitations. First, while conducting the gray literature search, we noted platform developers provide very brief and limited information within their webpages, and not all replied to requests for more information. Our findings may therefore be incomplete. Further, while our approach was rigorous, data pertained to the healthcare system in Queensland may not be generalizable to other states, and we may not have identified all statewide datasets. Strengths of the study included consultation with experts and professionals who use these systems daily, and finally validation by clinical informaticians specializing in each of the identified platforms.
Conclusion
Overall, we identified that current EHRs for IV catheter data collection have limited capacity to support a “learning healthcare system” where continuous improvement in indwelling IV catheter care is possible—using data to analyze and predict which treatments are more effective. While the optimal interaction design of each software is challenging, we must first address the standardization and export capacity of existing systems to optimize improvements in patient safety relative to vascular access care.
Data availability statement
The original contributions presented in this study are included in the article/Supplementary material; further inquiries can be directed to the corresponding author.
Ethics statement
Ethical approval was obtained from Griffith University (GU Ref: 2020/710). Written informed consent for participation was not required for this study in accordance with the national legislation and the institutional requirements.
Author contributions
JS and CR conceived the study, designed the protocol, obtained ethical approval, contributed to data collection and analysis, wrote, and revised the final manuscript. DB conceived the study, designed the protocol, obtained ethical and governance approval, led to data collection and analysis, contributed to the writing, and revision of the final manuscript. CS, NR, GR-B, and BS contributed to protocol design, provided content expertise, contributed to the writing, and revision of the final manuscript. RW provided site-specific expertise, contributed to the refinement of the manuscript, and approved the final version for publication. All authors contributed to the article and approved the submitted version.
Acknowledgments
The authors gratefully acknowledge Karina Charles for her assistance with figure preparation.
Conflict of interest
JS has received grant funding from Griffith University, the Children’s Hospital Foundation, and investigator-initiated research and educational grants provided to Griffith University by vascular access product manufacturers (Becton Dickinson), unrelated to this project. GR-B previous employer, Griffith University has received on her behalf speaker fees and consultancy payments from Ausmed, 3M, Becton Dickinson, and Wolters Kluwer unrelated to this project. RW reports investigator-initiated research grants provided to Griffith University by vascular access product manufacturer Becton Dickinson, unrelated to this project. BS has received funding from CRC Blue Economy, Sustainable Built Environment National Research Centre, SEQ Water, The National Environmental Science Programme, and North Coast Primary Health Network, all unrelated to this project. CR reports investigator-initiated research grants and speaker fees provided to Griffith University by vascular access product manufacturers (3M, BD-Bard, Cardinal Health, Eloquest), unrelated to this project.
The remaining authors declare that the research was conducted in the absence of any commercial or financial relationships that could be construed as a potential conflict of interest.
Publisher’s note
All claims expressed in this article are solely those of the authors and do not necessarily represent those of their affiliated organizations, or those of the publisher, the editors and the reviewers. Any product that may be evaluated in this article, or claim that may be made by its manufacturer, is not guaranteed or endorsed by the publisher.
Supplementary material
The Supplementary Material for this article can be found online at: https://www.frontiersin.org/articles/10.3389/fmed.2022.962130/full#supplementary-material
References
1. Cooke M, Ullman AJ, Ray-Barruel G, Wallis M, Corley A, Rickard CM, et al. Not “just” an intravenous line: consumer perspectives on peripheral intravenous cannulation (PIVC). An international cross-sectional survey of 25 countries. PLoS One. (2018) 13:e0193436. doi: 10.1371/journal.pone.0193436
2. Yasuda H, Rickard CM, Marsh N, Yamamoto R, Kotani Y, Kishihara Y, et al. Risk factors for peripheral intravascular catheter-related phlebitis in critically ill patients: analysis of 3429 catheters from 23 Japanese intensive care units. Ann Intensive Care. (2022) 12:33. doi: 10.1186/s13613-022-01009-5
3. Rickard CM, Ray-Barruel G. Peripheral intravenous catheter assessment: beyond phlebitis. Lancet Haematol. (2017) 4:e402–3. doi: 10.1016/S2352-3026(17)30145-X
4. Alexandrou E, Ray-Barruel G, Carr PJ, Frost SA, Inwood S, Higgins N, et al. Use of short peripheral intravenous catheters: characteristics, management, and outcomes worldwide. J Hosp Med. (2018) 13:61–73. doi: 10.12788/jhm.3039
5. Maki DG, Kluger DM, Crnich CJ. The risk of bloodstream infection in adults with different intravascular devices: a systematic review of 200 published prospective studies. Mayo Clin Proc. (2006) 81:1159–71. doi: 10.4065/81.9.1159
6. Rickard CM, Webster J, Wallis MC, Marsh N, McGrail MR, French V, et al. Routine versus clinically indicated replacement of peripheral intravenous catheters: a randomised controlled equivalence trial. Lancet. (2012) 380:1066–74. doi: 10.1016/s0140-6736(12)61082-4
7. Boyd S, Aggarwal I, Davey P, Logan M, Nathwani D. Peripheral intravenous catheters: the road to quality improvement and safer patient care. J Hosp Infect. (2011) 77:37–41. doi: 10.1016/j.jhin.2010.09.011
8. Franklin BD, Deelchand V, Cooke M, Holmes A, Vincent C. The safe insertion of peripheral intravenous catheters: a mixed methods descriptive study of the availability of the equipment needed. Antimicrob Resist Infect Control. (2012) 1:15. doi: 10.1186/2047-2994-1-15
9. Lim S, Gangoli G, Adams E, Hyde R, Broder MS, Chang E, et al. Increased clinical and economic burden associated with peripheral intravenous catheter-related complications: analysis of a us hospital discharge database. Inquiry. (2019) 56:46958019875562. doi: 10.1177/0046958019875562
10. Marsh N, Webster J, Larson E, Cooke M, Mihala G, Rickard CM. Observational study of peripheral intravenous catheter outcomes in adult hospitalized patients: a multivariable analysis of peripheral intravenous catheter failure. J Hosp Med. (2018) 13:83–9. doi: 10.12788/jhm.2867
11. Tuffaha HW, Marsh N, Byrnes J, Gavin N, Webster J, Cooke M, et al. Cost of vascular access devices in public hospitals in Queensland. Aust Health Rev. (2018) 43:511–5. doi: 10.1071/AH18102
12. Marsh N, Larsen E, Hewer B, Monteagle E, Ware RS, Schults J, et al. ‘How many audits do you really need?’: learnings from 5-years of peripheral intravenous catheter audits. Infect Dis Health. (2021) 26:182–8. doi: 10.1016/j.idh.2021.03.001
13. Schults JA, Woods C, Cooke M, Kleidon T, Marsh N, Barruel GR, et al. Healthcare practitioner perspectives and experiences regarding vascular access device data: an exploratory study. Int J Healthc Manag. (2020) 14:948–55. doi: 10.1080/20479700.2020.1721750
14. Schults J, Paterson R, Kleidon T, Cooke M, Ullman A, McNeil K, et al. Understanding consumer preference for vascular access safety and quality measurement: an international survey. Aust Health Rev. (2021) 46:12–20.
15. Schults JA, Rickard CM, Kleidon T, Hughes R, Macfarlane F, Hung J, et al. Building a global, pediatric vascular access registry: a scoping review of trial outcomes and quality indicators to inform evidence-based practice. Worldviews Evid Based Nurs. (2019) 16:51–9. doi: 10.1111/wvn.12339
16. Schults JA, Kleidon T, Chopra C, Cooke M, Paterson R, Ullman AJ, et al. International recommendations for a vascular access minimum data set: a Delphi consensus-building study. BMJ Qual. Saf. (2020) 30:722–30. doi: 10.1136/bmjqs-2020-011274
17. Linnen D. The promise of big data: improving patient safety and nursing practice. Nursing. (2016) 46:28–34. doi: 10.1097/01.nurse.0000482256.71143.09
18. Barnett A, Winning M, Canaris S, Cleary M, Staib A, Sullivan C. Digital transformation of hospital quality and safety: real-time data for real-time action. Aust Health Rev. (2019) 43:656–61. doi: 10.1071/AH18125
19. Shi J, Zheng M, Yao L, Ge Y. Developing a healthcare dataset information resource (DIR) based on Semantic Web. BMC Med Genomics. (2018) 11(Suppl. 5):102–73. doi: 10.1186/s12920-018-0411-5
20. Palanisamy V, Thirunavukarasu R. Implications of big data analytics in developing healthcare frameworks – A review. J King Saud Univ Comput Inf Sci. (2019) 31:415–25. doi: 10.1016/j.jksuci.2017.12.007
21. Al-Jarrah OY, Yoo PD, Muhaidat S, Karagiannidis GK, Taha K. Efficient machine learning for big data: a review. Big Data Res. (2015) 2:87–93. doi: 10.1016/j.bdr.2015.04.001
22. Cowie MR, Blomster JI, Curtis LH, Duclaux S, Ford I, Fritz F, et al. Electronic health records to facilitate clinical research. Clin Res Cardiol. (2017) 106:1–9. doi: 10.1007/s00392-016-1025-6
23. Samuels JG, McGrath RJ, Fetzer SJ, Mittal P, Bourgoine D. Using the electronic health record in nursing research: challenges and opportunities. West J Nurs Res. (2015) 37:1284–94. doi: 10.1177/0193945915576778
24. Mermel LA. How should surveillance systems account for concurrent intravascular catheters? JAMA Network Open. (2020) 3:e200400. doi: 10.1001/jamanetworkopen.2020.0400
25. Petersen K, Feldt R, Mujtaba S, Mattsson M. “Systematic mapping studies in software engineering,” in Proceedings of the 12th international conference on Evaluation and Assessment in Software Engineering, BCS Learning & Development Ltd, Italy (2008). p. 68–77.
26. Arksey H, O’Malley L. Scoping studies: towards a methodological framework. Int J Soc Res Methodol. (2005) 8:19–32.
27. Petersen K, Vakkalanka S, Kuzniarz L. Guidelines for conducting systematic mapping studies in software engineering: an update. Inf Softw Technol. (2015) 64:1–18. doi: 10.1016/j.infsof.2015.03.007
28. Armijo D, McDonnell C, Werner K. Electronic health record usability: evaluation and use case framework. In: MD Rockville editor. Quality AfHRa. Rockville, MA: Agency for Healthcare Research and Quality (2009).
29. Australian Bureau of Statistics. National, State and Territory Population 2020. (2020). Available online at: https://www.abs.gov.au/statistics/people/population/national-state-and-territory-population/latest-release (accessed October 21, 2020).
30. Queensland Government. Integrated Electronic Medical Record (ieMR) Queensland Health: Queensland Health. (2019). Available online at: https://www.health.qld.gov.au/clinical-practice/innovation/digital-health-initiatives/queensland/integrated-electronic-medical-record-iemr (accessed November 22, 2020).
31. Ripple AS. Expert googling: best practices and advanced strategies for using google in health sciences libraries. Med Ref Serv Q. (2006) 25:97–107. doi: 10.1300/J115v25n02_08
32. Wang L, Wang J, Wang M, Li Y, Liang Y, Xu D. Using internet search engines to obtain medical information: a comparative study. J Med Internet Res. (2012) 14:e74. doi: 10.2196/jmir.1943
33. Karampela M, Isomursu M, Porat T, Maramis C, Mountford N, Giunti G, et al. The extent and coverage of current knowledge of connected health: systematic mapping study. J Med Internet Res. (2019) 21:e14394. doi: 10.2196/14394
34. Celi LA, Mark RG, Stone DJ, Montgomery RA. Big data” in the intensive care unit. Closing the data loop. Am J Respir Crit Care Med. (2013) 187:1157–60. doi: 10.1164/rccm.201212-2311ED
35. Dolin RH, Alschuler L. Approaching semantic interoperability in health level seven. J Am Med Inform Assoc. (2011) 18:99–103. doi: 10.1136/jamia.2010.007864
36. Schults J, Rickard C, Kleidon T, Paterson R, Macfarlane F, Ullman A. Difficult peripheral venous access in children: an international survey and critical appraisal of assessment tools and escalation pathways. J Nurs Scholarsh. (2019) 51:537–46. doi: 10.1111/jnu.12505
37. Senathirajah Y, Wang J, Borycki E, Kushniruk A. Mapping the electronic health record: a method to study display fragmentation. Stud Health Technol Inform. (2017) 245:1138–42.
38. Peleg M, Keren S, Denekamp Y. Mapping computerized clinical guidelines to electronic medical records: knowledge-data ontological mapper (KDOM). J Biomed Inform. (2008) 41:180–201. doi: 10.1016/j.jbi.2007.05.003
39. Figueroa-Phillips LM, Bonafide CP, Coffin SE, Ross ME, Guevara JP. Development of a clinical prediction model for central line-associated bloodstream infection in children presenting to the emergency department. Pediatr Emerg Care. (2020) 36:e600–5. doi: 10.1097/pec.0000000000001835
40. Parreco JP, Hidalgo AE, Badilla AD, Ilyas O, Rattan R.l. Predicting central line-associated bloodstream infections and mortality using supervised machine learning. J Crit Care. (2018) 45:156–62. doi: 10.1016/j.jcrc.2018.02.010
41. Zhang H. Development of a risk prediction model for central-line–associated bloodstream infection (CLABSI) in patients with continuous renal replacement therapy. Infect Control Hosp Epidemiol. (2020) 41:s515. doi: 10.1017/ice.2020.1197
43. Jemcov T, Dimkovic N. Vascular access registry of Serbia: a 4-year experience. Int Urol Nephrol. (2017) 49:319–24. doi: 10.1007/s11255-016-1378-9
44. Girgenti C, Moureau N. The need for comparative data in vascular access: the rationale and design of the PICC registry. J Assoc Vasc Access. (2013) 18:219–24. doi: 10.1016/j.java.2013.07.003
45. Chopra V, Kaatz S, Conlon A, Paje D, Grant PJ, Rogers MAM, et al. The Michigan risk score to predict peripherally inserted central catheter-associated thrombosis. J Thromb Haemost. (2017) 15:1951–62. doi: 10.1111/jth.13794
46. Classen DC, Holmgren AJ, Co Z, Newmark LP, Seger D, Danforth M, et al. National trends in the safety performance of electronic health record systems from 2009 to 2018. JAMA Network Open. (2020) 3:e205547. doi: 10.1001/jamanetworkopen.2020.5547
47. Dube WC, Jacob JT, Zheng Z, Huang Y, Robichaux C, Steinberg JP, et al. Comparison of rates of central line–associated bloodstream infections in patients with 1 vs 2 central venous catheters. JAMA Network Open. (2020) 3:e200396. doi: 10.1001/jamanetworkopen.2020.0396
Keywords: intravascular catheter, quality surveillance, electronic health record, clinical informatics, patient – centered care
Citation: Schults JA, Ball DL, Sullivan C, Rossow N, Ray-Barruel G, Walker RM, Stantic B and Rickard CM (2022) Mapping progress in intravascular catheter quality surveillance: An Australian case study of electronic medical record data linkage. Front. Med. 9:962130. doi: 10.3389/fmed.2022.962130
Received: 06 June 2022; Accepted: 18 July 2022;
Published: 11 August 2022.
Edited by:
Ata Murat Kaynar, University of Pittsburgh, United StatesReviewed by:
Masaaki Sakuraya, Hiroshima General Hospital, JapanYuki Kotani, Kameda Medical Center, Japan
Copyright © 2022 Schults, Ball, Sullivan, Rossow, Ray-Barruel, Walker, Stantic and Rickard. This is an open-access article distributed under the terms of the Creative Commons Attribution License (CC BY). The use, distribution or reproduction in other forums is permitted, provided the original author(s) and the copyright owner(s) are credited and that the original publication in this journal is cited, in accordance with accepted academic practice. No use, distribution or reproduction is permitted which does not comply with these terms.
*Correspondence: Jessica A. Schults, ai5zY2h1bHRzQHVxLmVkdS5hdQ==