- 1School of Public Health, Kyoto University, Kyoto, Japan
- 2National Institute of Infectious Diseases, Tokyo, Japan
Background: Public health and social measures (PHSM) against COVID-19 in Japan involve requesting the public to voluntarily reduce social contact; these measures are not legally binding. The effectiveness of such PHSM has been questioned with emergence of the SARS-CoV-2 Alpha variant (B.1.1.7), which exhibited elevated transmissibility.
Materials and Methods: We investigated the epidemic dynamics during the fourth epidemic wave in Japan from March to June 2021 involving pre-emergency measures and declaration of a state of emergency (SoE). We estimated the effective reproduction number (Rt) before and after these interventions, and then analyzed the relationship between lower Rt values and each PHSM.
Results: With implementation of pre-emergency measures (PEM) in 16 prefectures, the Rt was estimated to be < 1 in six prefectures; its average relative reduction ranged from 2 to 19%. During the SoE, 8 of 10 prefectures had an estimated Rt < 1, and the average relative reduction was 26%–39%. No single intervention was identified that uniquely resulted in an Rt value < 1.
Conclusion: An SoE can substantially reduce the Rt and may be required to curb a surge in cases caused by future SARS-CoV-2 variants of concern with elevated transmissibility. More customized interventions did not reduce the Rt value to < 1 in this study, but that may be partly attributable to the greater transmissibility of the Alpha variant.
Introduction
Public health and social measures (PHSM) against COVID-19 are non-specific countermeasures, previously referred to as non-pharmaceutical interventions, which have been implemented in many countries to control the pandemic. Whereas published studies report a strong overall epidemiological impact of lockdowns or PHSM in reducing cases (1–14), other studies suggest that lockdown itself may not be very effective if individual measures, such as wearing masks and restricting the use of restaurants and public facilities, are properly implemented (15, 16). Additional scientific evidence on the effectiveness of individual non-specific countermeasures is called for (e.g., school closures, bans on eating out and on large gatherings) (17–34). When the SARS-CoV-2 Alpha variant (B.1.1.7) with elevated transmissibility started to become widespread in Europe, strict lockdown measures were instated in European countries, which precluded an evaluation of each PHSM other than movement restriction of entire communities (35).
In Japan, PHSM have primarily comprised request-based nationwide cooperation in voluntarily reducing social contact and have not involved any legally binding penalties. The legal and administrative basis of such countermeasures is the state of emergency (SoE), which in Japan is declared based on the Act on Special Measures for Pandemic Influenza and New Infectious Diseases Preparedness and Response (2002) (36). In Japan, the first SoE was declared in April 2020 owing to a surge in cases during the first epidemic wave. Although the first SoE was lifted in May 2020, an SoE was declared twice, in January and April 2021, for municipalities in the Tokyo metropolitan area and the Kansai region owing to increasing pressure on health care services during the third and fourth epidemic waves. During the fourth wave, a larger number of people were infected with the Alpha variant (B.1.1.7), which has greater transmissibility than other previously circulating SARS-CoV-2 strains (37, 38). Because an SoE involves substantial adverse social and economic effects, pre-emergency measures (PEM) were devised as an alternative to an SoE. More customized countermeasures began from February 2021, and PEM were newly implemented based on the Revised Special Measures for Pandemic Influenza and New Infectious Diseases Preparedness and Response (2020), in the hopes of avoiding an SoE (39). In the PEM, the local governor of the target area can decide which sub-regional areas (e.g., cities, towns, and villages) will be subject to the countermeasures. PEM is a general term for interventions that do not involve restrictions on movement; instead, the countermeasures are more customized and focused on high-risk settings, including eating and drinking establishments that serve alcohol (40). That is, whereas SoE may be regarded as self-restrained contact reduction or a voluntary lockdown measure, PEM are more customized pre-lockdown policies that are expected to be implemented early in the epidemic and that represent more targeted intervention in high-risk settings only. Customized policies are in line with the scientific evidence that high-risk situations include drinking alcohol late at night and attending indoor gatherings, which sometimes trigger super-spreading events (41, 42).
During the fourth epidemic wave, an SoE and PEM were declared multiple times, targeting municipalities across Japan. However, the effectiveness of these countermeasures in controlling infections has yet to be evaluated, especially considering the spread of the highly transmissible Alpha variant (43). These countermeasures have been diverse. In areas of intervention, different series of countermeasures have been implemented, including a request for residents to refrain from unnecessary movement and restricting the use of public facilities. In particular, the content, intensity, and duration of PEM have not been uniform because local governments decide the details regarding countermeasures. Like an SoE, PEM are not legally binding. Thus, the effectiveness of these measures depends on the compliance of residents and employers in areas of intervention and how well contact can be avoided that could lead to infection. Objectively assessing the effectiveness of such countermeasures remains a scientific challenge.
With emergence of the Alpha variant, which led to a surge in COVID-19 cases close to the Christmas holiday season, many European countries immediately imposed strict lockdown policies and did not permit customized interventions to be implemented (44). In many prefectures of Japan, PEM with various customized interventions were implemented, which were subsequently followed by an SoE that enforced restrictions on free movement. The fourth wave involving the Alpha variant in Japan thus offers a unique opportunity to evaluate the effectiveness of such countermeasures. Considering that there may be additional opportunities to implement PHSM in the future (45), and complete reliance on pharmaceutical interventions and vaccination is not possible (46), it is vital to explicitly assess those interventions. Thus, in the present study, we aimed to estimate the effectiveness of PHSM in Japan.
Materials and Methods
Epidemiological Data and Interventions
The present study was focused on the fourth epidemic wave in Japan, from March 1st to June 30, 2021, involving more than 370,000 confirmed COVID-19 cases and 6000 confirmed deaths across Japan. COVID-19 cases in Japan are confirmed by means of reverse transcription polymerase chain reaction (RT-PCR) and antigen testing, and all diagnosed cases are mandatorily notified to the government via the local health center. During the study period, two types of antigen detection kits using immunochromatography were approved in Japan, but PCR testing with a Taqman probe was used consistently for all confirmatory diagnosis (47). We used the incidence of confirmed cases as a function of the date of diagnosis and the date of illness onset as registered in the Health Center Real-Time Information-sharing System on COVID-19 (HER-SYS) (48). During the study period, random sampling of PCR-positive patients in Japan was conducted to screen for the N501Y point mutation that was commonly seen in the Alpha variant (B.1.1.7) using real time RT-PCR, so as to estimate the percentage of infections with this variant (49). As of March 28, approximately 30% of all infected patients identified on each day were screened (50). Some were tested using whole-genome sequencing, but the sequencing results were not used to determine the proportion of variants, because it takes about two weeks to report and cannot provide a timely picture of the infection situation in real time. The results of these screenings were entered into the HER-SYS along with other epidemiological information. We estimated the number of infections with the Alpha variant in the country according to the number of infected persons per day, the number of screening tests per day, and the number of cases that were positive for the N501Y point mutation.
Information regarding the types and length of PHSM were systematically collected according to prefecture and local government. According to the type of intervention, PHSM were classified into seven different categories: (a) official requests not to sell and serve alcoholic beverages and shortened business hours for restaurants at night; (b) closure of public facilities where large gatherings of people could be expected; (c) stay-at-home measures, combined with requests of the public not to travel across prefectures; (d) school closures; (e) requests to not organize public events; (f) requests to not engage in free movement within a city/ward; and (g) enlarging of the geographic area in which the above six countermeasures were implemented. To understand the impact of the epidemiological “stage” on reducing the effective reproduction number (Rt), the following datasets were also collected and examined in relation to Rt: hospital bed occupancy (both in general wards and intensive care units), the daily number of newly reported cases, daily PCR-positivity rate, which is the number of reported PCR-positive patients divided by the total number of PCR tests (51), and the proportion of unlinked infections among confirmed infections (i.e., the proportion of cases whose source could not be identified) (52). We used these variables to determine the epidemiological stage in Japan, leading to different levels of PHSM.
Estimation of the Effective Reproduction Number
We used methods proposed by Nakajo and Nishiura for calculating Rt (53, 54). The Rt of COVID-19 was estimated as the epidemiological outcome, particularly its absolute and relative changes before and after the start of interventions. Rt was estimated using the incidence according to the date of illness onset (21). Letting ct be the incidence according to date of illness onset t,
where E(.) is the expectation, fs is the probability mass function of the incubation period duration s, and λu is the probability mass function of secondary transmission as a function of the time since illness onset u. The maximum likelihood method was used for the estimation of Rt. It should be noted that the resulting estimated Rt is a function of the date of infection.
Following Nakajo and Nishiura (53, 54) we assumed that x is the duration of infectiousness prior to illness onset, and we set x = 5 days (i.e., cases became infectious 5 days prior to the illness onset date). fs was assumed to follow a lognormal distribution with mean 5.2 days and variance 14.9 (55), and λu was assumed to follow a gamma distribution with mean 12.9 days and variance 8.1 (56).
The abovementioned equation was further applied to estimate the Rt for the Alpha variant only. For this, we reconstructed the incidence of infections with variant Alpha using the partially screened dataset of real time RT-PCR screening of cases for the N501Y mutation. As proposed by Murayama et al. (57), a hypergeometric distribution was used to estimate the incidence, i.e., on each day, the total number of cases infected with variant Alpha represents a random selection of screening tests conducted among the total number of confirmed cases and a random selection of cases that were positive for the Alpha variant among all screened cases.
The effectiveness of PEM or SoE was calculated by comparing the change in Rt, that is, between (i) the Rt value 7 or 14 days prior to implementation of the PHSM and (ii) the first 7 days or total days of the PHSM. To facilitate this estimation, we used a piecewise constant model for Rt (i.e., handling Rt as a step function) for the respective periods. A 7-day period was specifically chosen for period (ii) because that period was followed by the so-called Golden Week, a spring holiday period in Japan of more than 10 consecutive days, which influences people’s mobility characteristics. In addition to relative and absolute risk reductions in secondary transmission, we also explored whether the Rt value during each intervention period (with PEM or SoE) was < 1, indicating that the incidence of COVID-19 infection was in a declining trend. We truncated the last 10 days of data and conducted analysis through May 27, 2021 because the most recent Rt estimation using onset data is an underestimate owing to reporting delay. We varied the combinations of duration of periods (i) and (ii) to assess the reductions in Rt; for example, we alternatively used the estimated Rt for the entire period of intervention rather than using a fixed length of 7 days as part of sensitivity analysis. Among prefectures in which interventions were implemented, Miyagi was excluded from our analysis because most COVID-19 cases in Miyagi were caused by a SARS-CoV-2 strain with the E484K mutation, which was not classified as either a variant of concern or variant of interest (23). To avoid underestimation of Rt, the dataset for the period under PEM in Hokkaido was analyzed for only 6 days post intervention; similarly, the dataset under the SoE in Okayama was analyzed for the first 5 days of intervention owing to right truncation. In Hokkaido, Gifu, and Mie prefectures, the 10-day national holiday period overlapped with the 7-day period immediately before the implementation of PEM, potentially overestimating their effectiveness. Thus, we also compared the Rt values before and after intervention, using the 7-day period before the start of the national holiday as an alternative baseline.
To explore the statistical association between a decline in Rt value and each individual countermeasure, we performed the Wilcoxon signed-rank test. Likewise, we carried out univariate analysis to explore the relationship between the stage of the epidemic when PHSM were implemented and a reduction in the Rt value.
Results
Changes in the Effective Reproduction Number
Figure 1 shows the Rt in six prefectures where both PEM and an SoE were implemented, using a step function for the first 7 days before and during intervention. Overall, a decreasing trend in the Rt was noted during the countermeasure period.
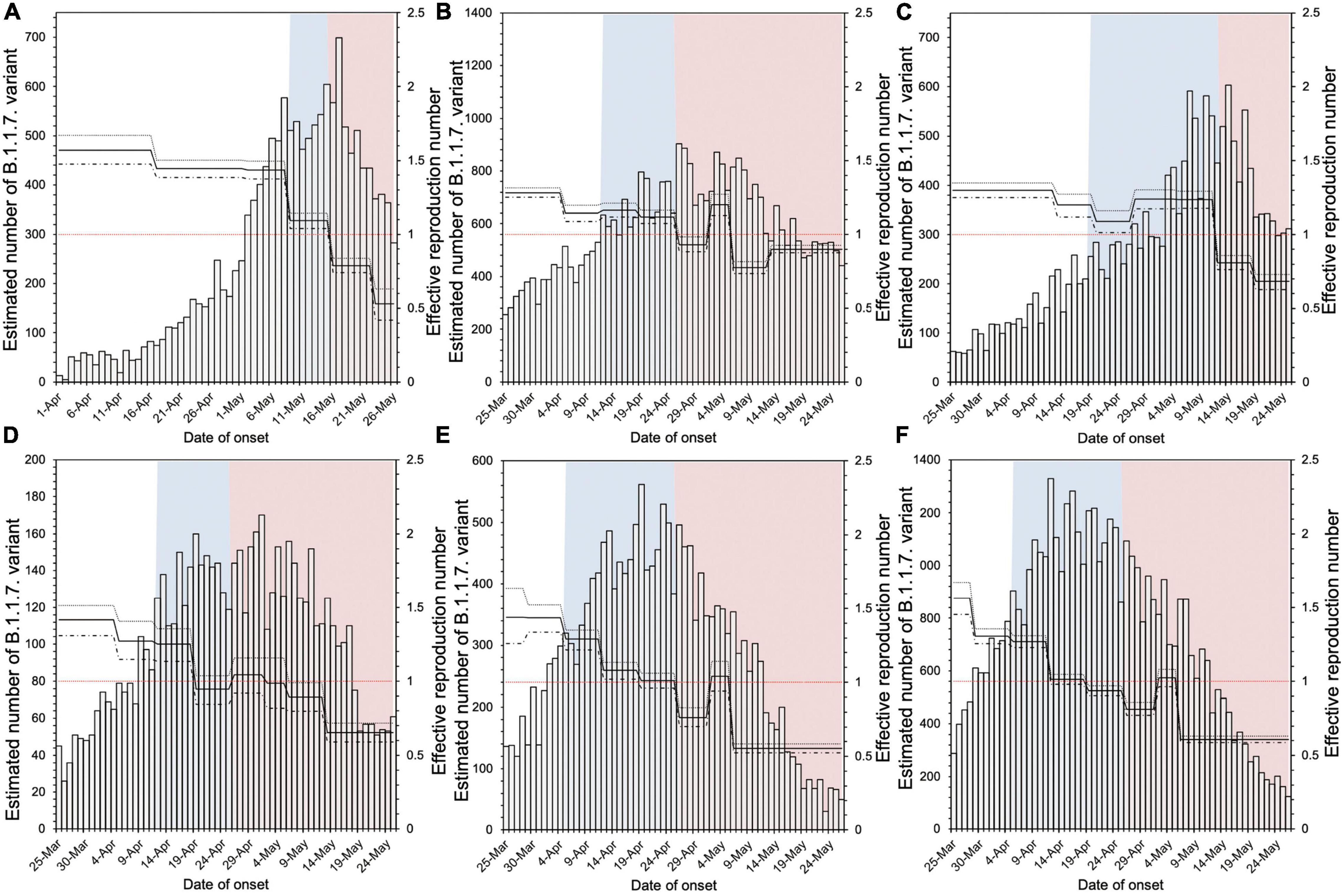
Figure 1. Estimated effective reproduction number in six prefectures implementing both pre-emergency measures and a state of emergency. (A–F) in the figure correspond to Hokkaido, Tokyo, Aichi, Kyoto, Osaka, and Hyogo prefectures, respectively. The blue shaded areas indicate the period of pre-emergency measures, and the red shaded areas indicate the state of emergency period. The bar graph is the estimated number of infections with the B.1.1.7 variant by onset date, and the solid line is the effective reproduction number (Rt). Using the 7 days before implementation of pre-emergency measures as the baseline, we compared the Rt for the 7 days immediately after the start of each measure.
The relative reduction in Rt values after PEM implementation was estimated to range from −110.9% to 43.0% (Table 1), where negative values represent a failure to reduce the Rt value. Among a total of 16 prefectures following PEM, six prefectures achieved an Rt value < 1 (Gunma, Gifu, Mie, Ehime, Kumamoto, and Okinawa). The average and median relative reduction in Rt in these 16 prefectures was 2.0% and 9.0%, respectively. Supplementary Table 1 shows the relative reduction in Rt, analyzing the values for the entire period of PEM implementation. The relative reduction in Rt was estimated to range from −15.9 to 51.6%, and the average and median relative reduction in Rt was estimated to be 11.3% and 14.8%, respectively, among a total of 16 prefectures. Using the Rt of the 14 days prior to PEM implementation, the relative reduction in Rt was estimated to range from −18.5 to 49.7% and the average and median relative reduction in Rt was estimated to be 19.4% and 20.6%, respectively.
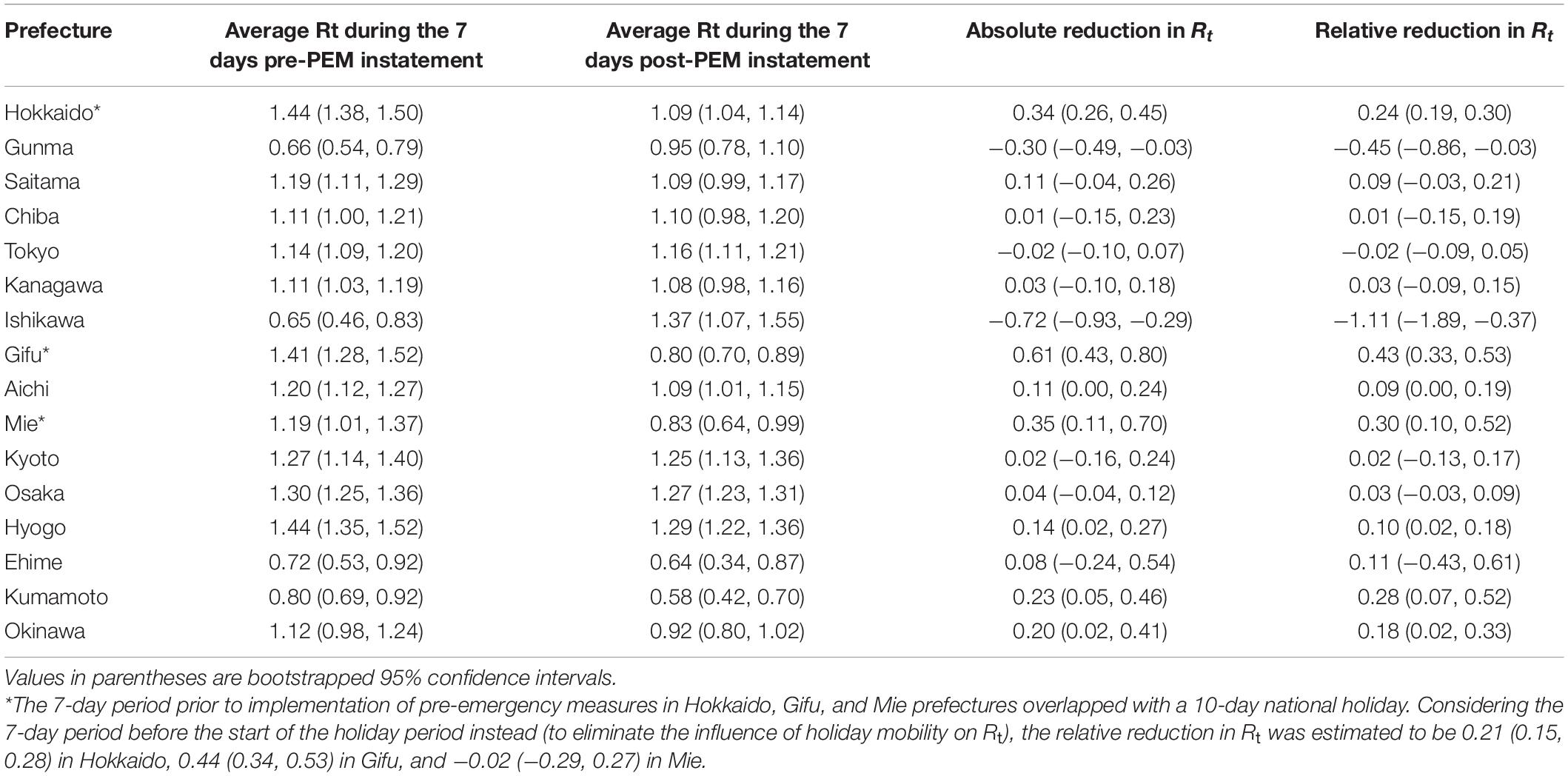
Table 1. Effective reproduction number (Rt) during the 7 days before and after pre-emergency measures (PEM) were instated.
Similar analysis was conducted for the SoE (Table 2). Among the 10 prefectures under an SoE, the relative reduction in Rt values under the SoE was estimated to range from −13.4 to 47.1%, using the 7-day average Rt before intervention as the baseline. The average and median relative reduction in Rt was estimated to be 25.9% and 31.5%, respectively. Kyoto and Okinawa did not achieve an Rt < 1 within 7 days of the start of the SoE; however, the Rt in the remaining eight prefectures was estimated to be < 1. Supplementary Table 2 shows the results for the SoE in different comparison periods before and after the intervention. The relative reduction in Rt under the SoE was estimated to range from −28.2 to 52.7%, using the 7-day average Rt before the intervention as the baseline. The average and median relative reduction in Rt was estimated to be 27.6% and 37.7%, respectively. Using the Rt of the 14 days before the start of the SoE, the relative reduction in Rt was estimated to range from −18.5 to 61.0%, and the median relative reduction in Rt was estimated to be 38.7% and 48.6%, respectively.
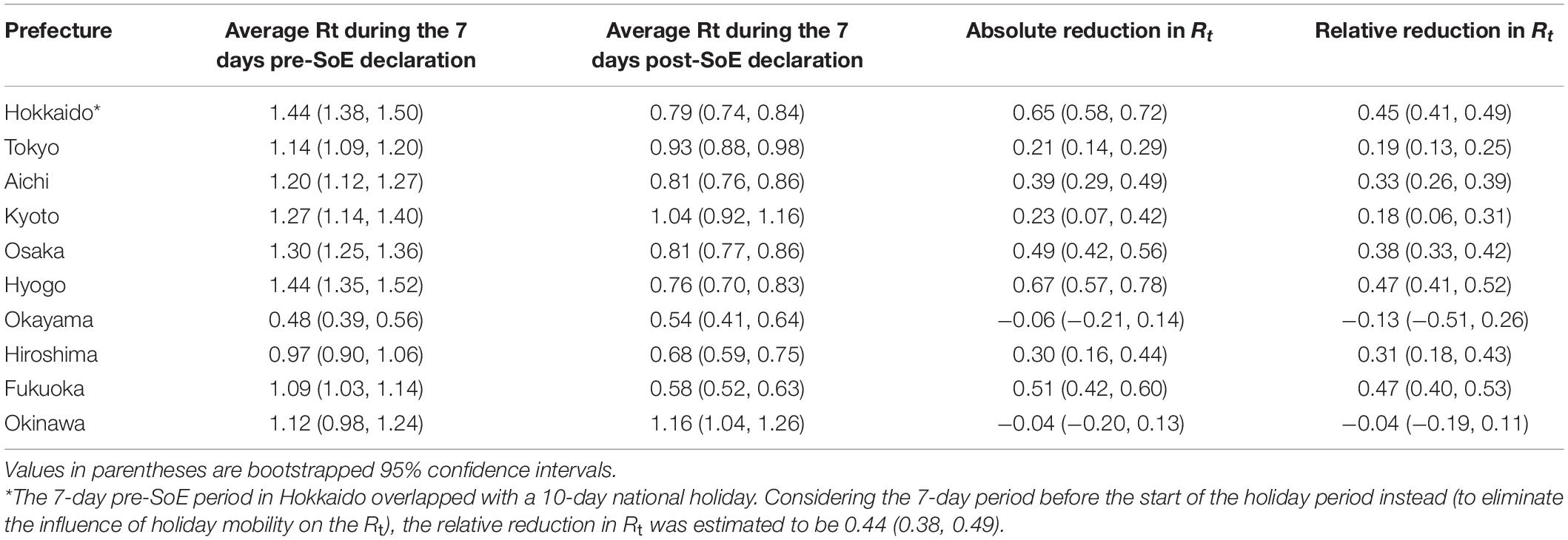
Table 2. Effective reproduction number (Rt) during the 7 days before and after declaration of a state of emergency (SoE).
Content and Timing of Interventions
Figure 2 shows distributions of the absolute reduction and relative reduction in Rt values, grouped according to content of the PEM. The reduction in Rt tended to be greater in prefectures implementing school-related interventions than in the remaining prefectures. Interventions associated with eating and drinking establishments, recreational and community facilities, and large gatherings or events were implemented in all prefectures during the PEM period; therefore, we were unable to make comparisons for these measures.
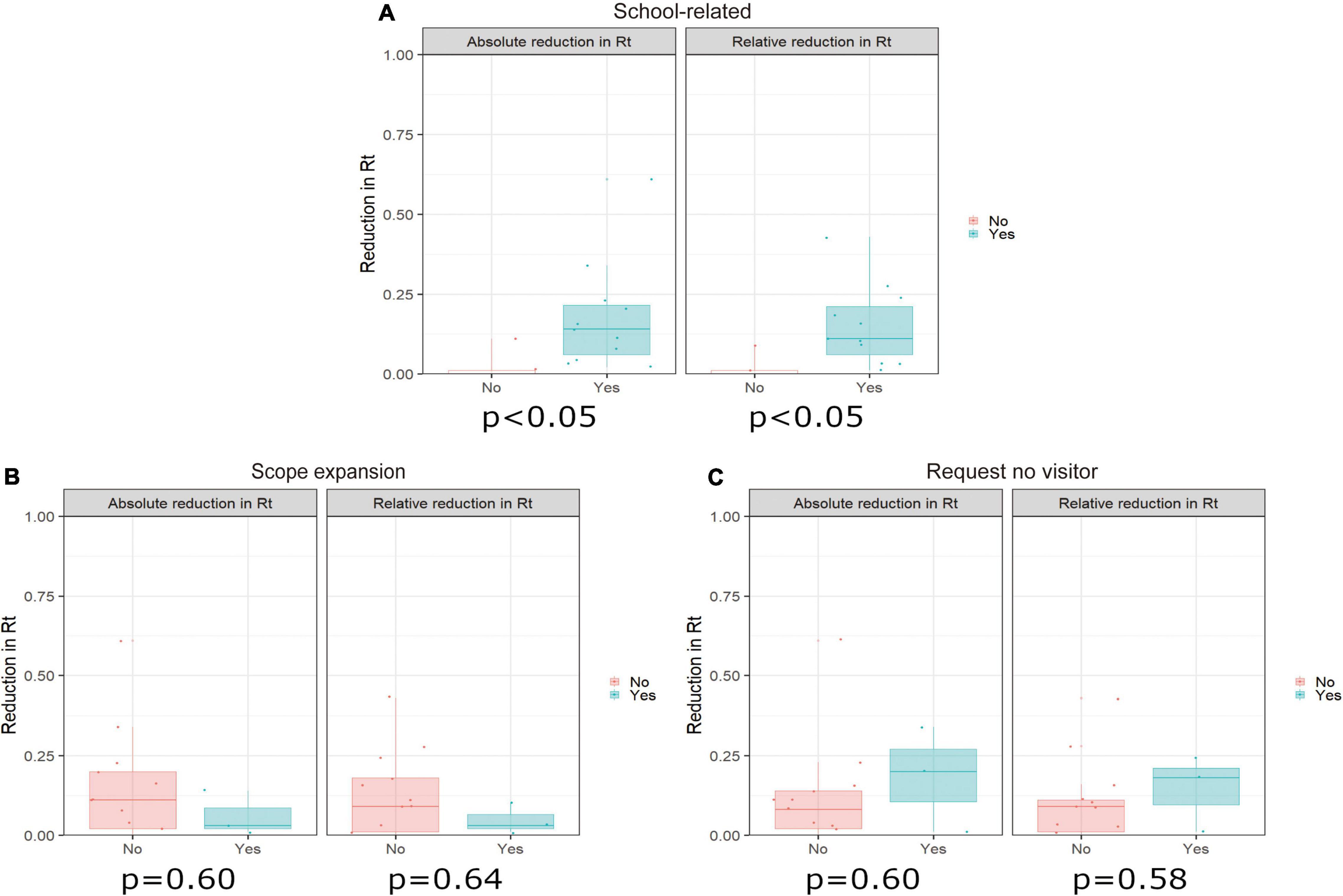
Figure 2. Relationship between pre-emergency measure (PEM) categories and reduction in the effective reproduction number (Rt) compared with the 7 days before intervention (baseline period). This figure shows the relationship between the reduction in Rt during the 7 days prior to the intervention and during the 7 days after the intervention and presence of each PEM intervention. The only PEM categories for which there were differences in adoption among prefectures were (A) school-related measures, (B) expansion of the intervention scope (a pre-emergency intervention originally implemented in only part of a given prefecture was expanded to additional areas), and (C) requests (from the prefecture) for no out-of-prefecture travel. We calculated p-values using the Wilcoxon signed-rank test. The left-hand panel in each figure shows the absolute reduction in Rt and the right-hand panel shows the relative reduction in Rt.
Supplementary Figure 1 shows results regarding the SoE, which were similar to those of the PEM. We did not identify any significant differences regarding the reduction in Rt according to different types of intervention. These findings were consistent across different durations of baseline and intervention periods.
Figure 3 illustrates distributions of the absolute reduction and relative reduction in Rt according to the stage of the epidemic when interventions were carried out (as classified into four categories; see Figure 3 legend for the definitions). The reduction in Rt values in stage 4 of the PCR positivity rate tended to be greater than that in stages 2 and 3; the daily PCR positivity rate is defined here as: 5% or more of all tests positive is stage 3 and 10% or more is stage 4. Supplementary Figure 2 shows the results of analysis of stages at the time of SoE implementation. No marked association was identified between the stage of the epidemic and relative reduction in Rt during the SoE. This finding was maintained across varying durations of the baseline and intervention periods.
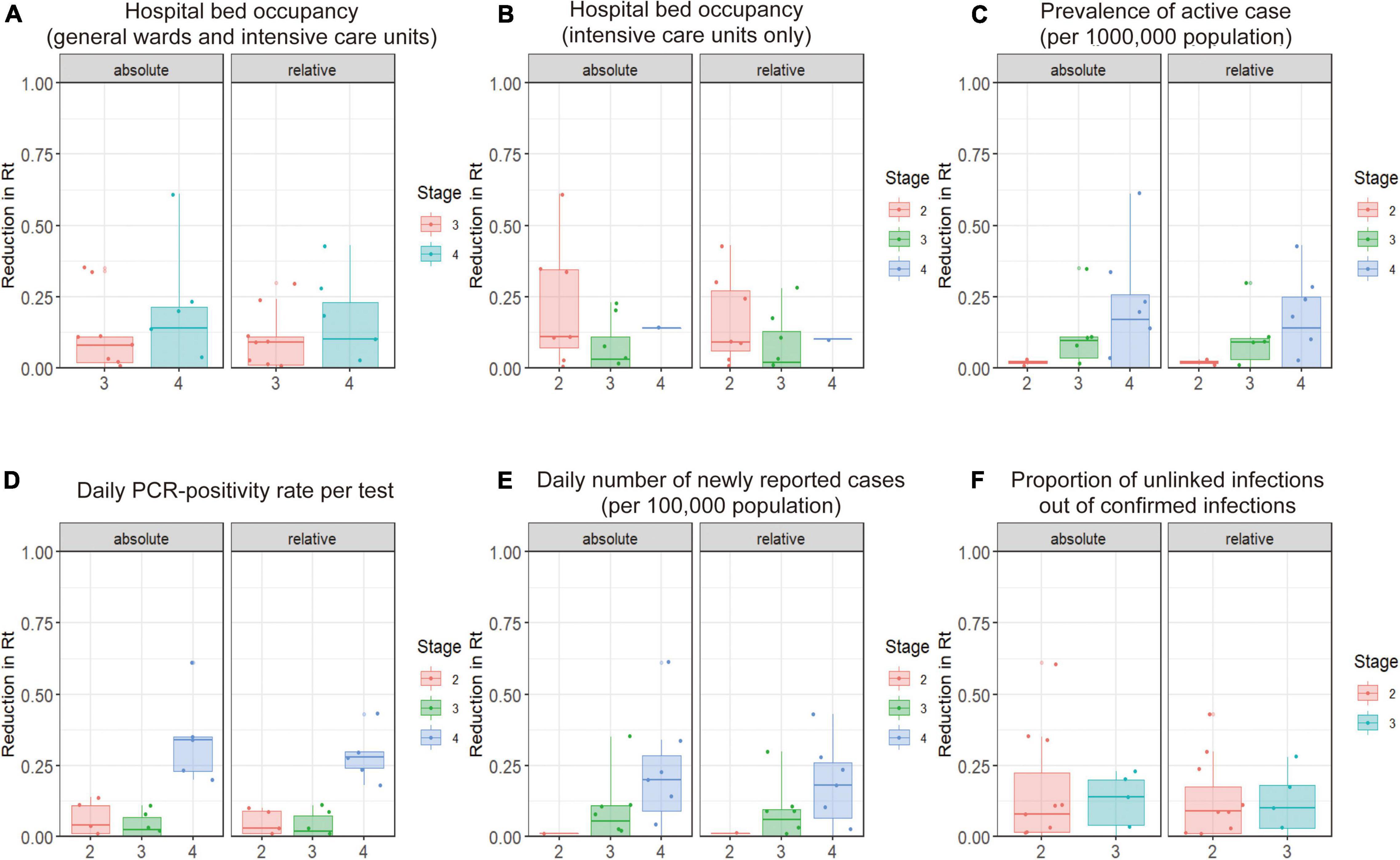
Figure 3. Relationship between prefectural COVID-19 epidemic stage at the start of pre-emergency measures (PEM) and reduction in the effective reproduction number (Rt) during the PEM period compared with the 7 days before intervention (baseline period). This figure shows the relationship between the reduction in Rt during the 7 days prior to the intervention and during the 7 days after the intervention and the epidemic stage at the time of the intervention. The horizontal axis is the “stage” of the COVID-19 epidemic, according to definitions of the Japanese government. (A,B) Hospital-bed occupancy is defined as stage 3 when 20% of COVID-19 beds are occupied and stage 4 when 50% of beds are occupied. (C) The prevalence of active cases is defined as the number of patients who are hospitalized or under observation at home. Twenty or more cases per 100,000 population in a prefecture is defined as stage 3, and 30 or more cases is defined as stage 4. (D) The daily PCR-positivity rate is defined as stage 3 with 5% or more positive test results among the total tests and stage 4 with 10% or more. (E) The daily number of newly reported cases is defined as stage 3 with 15 or more newly reported cases per 100,000 population and stage 4 with 25 or more newly reported cases per 100,000. (F) The percentage of unlinked cases is defined as 50% or more for stage 3 and less than 50% for stage 2. The left-hand panel shows the absolute reduction in the effective reproduction number (Rt) and the right-hand panel shows the relative reduction. We calculated p-values using analysis of variance or the Wilcoxon signed-rank test.
Discussion
In the present study, we evaluated the impact of the PEM and SoE on the epidemiological dynamics of COVID-19 from March to June 2021 in different regions of Japan, during which time the SARS-CoV-2 Alpha variant was predominant in the country. When PEM were implemented in 16 prefectures, the Rt was reduced to < 1 in only six of these prefectures, with the average relative reduction ranging from 2 to 19%. However, implementation of an SoE led to an average Rt value < 1 in 8 of the 10 prefectures where implemented, with an average relative reduction in the Rt ranging from 26 to 39%. For individual interventions, only school closures during periods of PEM implementation showed significant differences in reducing the Rt; no other interventions helped to explain variations in the relative reduction in Rt. Although there was no significant association between the relative reduction in Rt and the timing of initiating interventions, an extremely high positivity rate in PCR testing may predict a substantial reduction in the Rt. An increasing trend over time in the positivity rate for PCR testing results has been shown to predict a forthcoming epidemic wave (58). The present study findings further showed that PCR testing results that are extremely high, i.e., a very high positivity rate, may be one signature of a forthcoming reduction in the Rt, perhaps because a very high positivity rate is usually a sign of the need for strong intervention measures.
The findings of the present study revealed is that customized interventions (referred to as PEM in our study) in high-risk transmission settings may be insufficient to lower the Rt to < 1. Before the introduction of variant Alpha in Japan, a simple modeling study showed that Rt decreased to < 1 only after instating customized PHSM focused on high-risk settings. During the summer of 2020, interventions were centered on eating and drinking establishments operating at night and public facilities for mass gatherings and included shortening of opening hours, requests to not serve alcohol in Tokyo, restricting the number of people eating at the same table to four, and closure of nightlife areas in Osaka (59). Through that experience, such focused interventions were legally formalized by the Japanese government and categorized as PEM to help avoid unnecessary adverse social and economic impacts on the entire population. Unfortunately, with introduction of the more-transmissible Alpha variant, our findings showed that in many prefectures, the introduction of PEM alone was not sufficient to reduce the Rt value to below 1 during the spring of 2021. From July 2021, the SARS-CoV-2 Delta variant, which is even more transmissible than variant Alpha (60, 61), was introduced and rapidly became predominant among all virus strains circulating in Japan (62). Whereas elevated temperatures may help to slightly lower the Rt value (63), only stronger restrictions such as an SoE can suppress a sharp rise in COVID-19 infections (64, 65). Therefore, swift decisions regarding declaration of an SoE are required to bring an epidemic under control.
The PHSM explored in this present study are not accompanied by legal penalties In Japan; instead, both PEM and an SoE involve an official request from national and local governmental bodies to adhere to the policies (36). Furthermore, even without government intervention, people may precautionarily adopt risk-avoidance behavior, especially when the number of infected individuals is increased. Because the PEM and SoE were not legally binding in principle, we cannot explicitly determine whether people actually reduced their risky behaviors voluntarily (but actively) under the interventions (66). This characteristic complicates the evaluation in two ways. First, we may not always be able to anticipate whether public reactions and behavioral responses to PEM or an SoE will be the same as those observed from March to June 2021. If the general public has difficulty enduring restrictions on high-risk behaviors, the findings of the present study may not be applicable and cannot be expected in the future (67). In this sense, request-based (voluntary) restriction of social contact behaviors cannot be causally evaluated and the effectiveness of repeated implementation cannot be ensured. Second, psychological impacts could influence our outcomes. The declaration of an SoE itself might have had the effect of preventing risky behaviors, but such behavioral changes might have been induced primarily by elevated risk awareness, e.g., the declaration led to voluntary cancelation of travel and large gatherings or events (68). As such, it is vital to remember that the PEM and SoE in Japan rely on voluntary cooperation of the general public and employers, and people’s psychological responses to such requests have a key role in the effect of intervention. In these respects, it is inherently difficult to separate the effects of intervention from the effects of voluntary risk-avoidance behaviors. We performed additional analyses with the aim to strengthen our findings. As shown in Supplementary Table 3, we made a comparison of the Rt at 1 and 2 weeks prior to the implementation of PEM. Indeed, whereas the Rt decreased before the start of PEM in some prefectures and this decrease was large, the same trend was not observed in many prefectures. Supplementary Figure 3 depicts the change in Google mobility before and after intervention. Although in some prefectures, mobility had already decreased before the PEM, it was found that mobility generally decreased with the intervention. These supplementary analyses do not refute the effect of voluntary risk-avoidance behaviors. Rather, the important point here is that many prefectures were unable to reduce the Rt to below 1 with customized PEM that mostly targeted eating and drinking establishments whereas the SoE was able to flatten the epidemic curve.
It is worth mentioning that in a part of our analysis, we adopted a baseline period of the 7 days prior to the implementation of PHSM. Of course, risk awareness had been gradually increasing owing to the increasing epidemic size prior to the start of PHSM; therefore, people’s social contact behavior could have already begun to change during the 7-day baseline period. Thus, as part of the sensitivity analysis, the duration of the baseline period was altered. Comparing the Rt 7 days prior to the intervention and during the entire intervention period, PEM and the SoE reduced the Rt by an average of 11.3% and 27.6%, respectively. When the baseline was set to 14 days, the PEM and SoE decreased the Rt by an average of 19.4% and 38.7%, respectively. However, this analysis did not substantially alter our findings.
Our results regarding school closures during PEM were consistent with those of published studies (69–74). School closures during an SoE did not lead to a marked reduction in Rt; therefore, school closures would not be consistently effective across all possible epidemiological conditions (74, 75).
Caution is needed when discussing the timing of PHSM implementation (74). The short-term goals of PHSM may include to (i) suppress an epidemic, (ii) ease caseload pressure on health care facilities, and (iii) buy time to increase protection via pharmaceutical interventions (e.g., vaccination). If goal (iii) is not critical, early implementation of PHSM will always be the most effective owing to the containment of an epidemic at a local level. This is particularly true for PEM; the risk of an epidemic wave could have been greatly reduced if PEM had been implemented before a substantial increase in the COVID-19 incidence and if the PEM covered the areas or regions with increasing virus transmission. It must be remembered that the estimated relative reduction in Rt found in the present study is conditional on implementation at a given timing; in general, the effectiveness of PHSM is greater if implemented earlier (76, 77).
Certain limitations in this study must be acknowledged. First, the quantified risk reduction would not be causal, as mentioned above; thus, similar impacts of PHSM are not guaranteed (40). Second, our analysis relied on the change in Rt before and after intervention. We argued that this change may be attributable to extrinsic effects, namely, PHSM. However, other extrinsic factors (e.g., behavioral changes) and intrinsic factors (e.g., depletion of susceptibles) can also lead to a reduction in the Rt value. In fact, there were continual government announcements with respect to the ongoing risk of infection and requests to wear a face mask, maintain an appropriate social distance, and engage in preventive measures (76). Third, in our analysis, we did not evaluate the time-dependent variations in intervention effectiveness. During the later stages of PHSM, the degree of preventive effectiveness can sometimes be magnified, perhaps owing to a gradual reduction in high-risk contacts. Fourth, we used the prefecture as the unit in our analysis, and these were analyzed independently. Spatial correlations associated with travel across prefectures could not be controlled (40).
Although not causal, the present study provides important evidence indicating that achieving a substantial reduction in Rt in the presence of a highly transmissible SARS-CoV-2 variant requires implementation of an SoE. Continued epidemiological assessment of PHSM is critical, alongside further analysis of the heterogeneities in effectiveness among interventions.
Data Availability Statement
The original contributions presented in the study are included in the article/Supplementary Material, further inquiries can be directed to the corresponding author.
Ethics Statement
This study was approved by the Medical Ethics Board of the Graduate School of Medicine at Kyoto University (R2676).
Author Contributions
KH conceived the study. KH and HN conceptualized the study design and drafted early versions of the manuscript and figures. KH, AA, MF, AS, TK, and MS collected the data. KH, NL, AA, MF, AS, and TK performed the statistical analyses. All authors provided comments on the manuscript and approved the final version of the manuscript.
Funding
KH received funding from the Japan Society for the Promotion of Science (JSPS) KAKENHI (20K18953) and The Health Care Science Institute (IKEN). HN received funding from Health and Labour Sciences Research Grants (20CA2024, 20HA2007, 21HB1002, and 21HA2016), the Japan Agency for Medical Research and Development (JP20fk0108140, JP20fk0108535, and JP21fk0108612), the JSPS KAKENHI (21H03198), Environment Research and Technology Development Fund (JPMEERF20S11804) of the Environmental Restoration and Conservation Agency of Japan, the Japan Science and Technology Agency CREST program (JPMJCR1413), and the SICORP program (JPMJSC20U3 and JPMJSC2105). The funders had no role in the study design, data collection and analysis, decision to publish, or preparation of the manuscript.
Conflict of Interest
The authors declare that the research was conducted in the absence of any commercial or financial relationships that could be construed as a potential conflict of interest.
Publisher’s Note
All claims expressed in this article are solely those of the authors and do not necessarily represent those of their affiliated organizations, or those of the publisher, the editors and the reviewers. Any product that may be evaluated in this article, or claim that may be made by its manufacturer, is not guaranteed or endorsed by the publisher.
Acknowledgments
We thank Analisa Avila, MPH, ELS, of Edanz (https://jp.edanz.com/ac) for editing a draft of this manuscript. We also thank all local governments, public health centers, and institutes for conducting surveillance, laboratory testing, epidemiological investigations, and data collection.
Supplementary Material
The Supplementary Material for this article can be found online at: https://www.frontiersin.org/articles/10.3389/fmed.2022.937732/full#supplementary-material
Supplementary Figure 1 | Relationship between state of emergency (SoE) categories and reduction in the effective reproduction number (Rt) during an SoE compared with the 7 days before intervention (baseline period). This figure shows the relationship between the reduction in Rt during the 7 days prior to the intervention and during the 7 days after the intervention and presence of each SoE intervention. The only SoE categories for which there were differences in adoption among prefectures were (A) school-related measures, (B) expansion of the intervention scope (SoE originally implemented in only part of a given prefecture expanded to additional areas), and (C) requests (from the prefecture) for no out-of-prefecture travel. We calculated p-values using the Wilcoxon signed-rank test. The left-hand panel in each figure shows the absolute reduction in Rt and the right-hand panel shows the relative reduction in Rt.
Supplementary Figure 2 | Relationship between prefectural COVID-19 epidemic stage at the start of a state of emergency (SoE) and reduction in the effective reproduction number (Rt) during the SoE compared with the 7 days before intervention (baseline period). This figure shows the relationship between the reduction in Rt during the 7 days prior to the intervention and during the 7 days after the intervention and the epidemic stage at the time of the intervention. The horizontal axis is the “stage” of the COVID-19 epidemic according to definitions of the Japanese government. (A,B) Hospital-bed occupancy is defined as stage 3 when 20% of COVID-19 beds are occupied and stage 4 when 50% of beds are occupied. (C) The prevalence of active cases is defined as the number of patients who are hospitalized or under observation at home. Twenty or more cases per 100,000 population in a prefecture is defined as stage 3, and 30 or more cases is defined as stage 4. (D) The daily PCR-positivity rate is defined as stage 3 with 5% or more positive test results among the total tests and stage 4 with 10% or more. (E) The daily number of newly reported cases is defined as stage 3 with 15 or more newly reported cases per 100,000 population and stage 4 with 25 or more newly reported cases per 100,000. (F) The percentage of unlinked cases is defined as 50% or more for stage 3 and less than 50% for stage 2. The left-hand panel shows the absolute reduction in the effective reproduction number (Rt) and the right-hand panel shows the relative reduction. We calculated p-values using analysis of variance or the Wilcoxon signed-rank test.
Supplementary Figure 3 | Google mobility: retail and recreation percent change from baseline during and before intervention. The broken line shows the retail and recreation percent change from baseline for mobility provided by Google. The relative change in time spent compared with that at the baseline of January 2020 is shown. The blue shading in the figure indicates pre-emergency measures (PEM), the red shading indicates state of emergency (SoE), the yellow shading indicates PEM 1 week earlier, and the green shading indicates PEM 2 weeks earlier. Panels (A–F) in the figure correspond to Hokkaido, Tokyo, Aichi, Kyoto, Osaka, and Hyogo prefectures, respectively. In (A,D), mobility appears to have already started decreasing before the intervention. In the other panels, the effect of intervention appears to be working, especially for the SoE.
References
1. Flaxman S, Mishra S, Gandy A, Unwin HJT, Mellan TA, Coupland H, et al. Estimating the effects of non-pharmaceutical interventions on COVID-19 in Europe. Nature. (2020) 584:257–61.
2. Haug N, Geyrhofer L, Londei A, Dervic E, Desvars-Larrive A, Loreto V, et al. Ranking the effectiveness of worldwide COVID-19 government interventions. Nat Hum Behav. (2020) 4:1303–12.
3. Davies NG, Kucharski AJ, Eggo RM, Gimma A, Edmunds WJ. Centre for the Mathematical Modelling of Infectious Diseases COVID-19 working group. Effects of non-pharmaceutical interventions on COVID-19 cases, deaths, and demand for hospital services in the UK: a modelling study. Lancet Public Health. (2020) 5:e375–85. doi: 10.1016/S2468-2667(20)30133-X
4. Roques L, Klein EK, Papaïx J, Sar A, Soubeyrand S. Impact of Lockdown on the Epidemic Dynamics of COVID-19 in France. Front Med. (2020) 7:274. doi: 10.3389/fmed.2020.00274
5. Salvatore M, Basu D, Ray D, Kleinsasser M, Purkayastha S, Bhattacharyya R, et al. Comprehensive public health evaluation of lockdown as a non-pharmaceutical intervention on COVID-19 spread in India: national trends masking state-level variations. BMJ Open. (2020) 10:e041778.
6. Aguiar M, Van-Dierdonck JB, Stollenwerk N. Reproduction ratio and growth rates: measures for an unfolding pandemic. PLoS One. (2020) 15:e0236620. doi: 10.1371/journal.pone.0236620
7. Davies NG, Barnard RC, Jarvis CI, Russell TW, Semple MG. Association of tiered restrictions and a second lockdown with COVID-19 deaths and hospital admissions in England: a modelling study. Lancet Infect Dis. (2021) 21:482–92. doi: 10.1016/S1473-3099(20)30984-1
8. Santamaría L, Hortal J. COVID-19 effective reproduction number dropped during Spain’s nationwide dropdown, then spiked at lower-incidence regions. Sci Total Environ. (2021) 751:142257. doi: 10.1016/j.scitotenv.2020.142257
9. Hyafil A, Moriña D. Analysis of the impact of lockdown on the reproduction number of the SARS-Cov-2 in Spain. Gac Sanit. (2021) 35:453–8.
10. Gupta M, Mohanta SS, Rao A, Parameswaran GG, Agarwal M, Arora M, et al. Transmission dynamics of the COVID-19 epidemic in India and modeling optimal lockdown exit strategies. Int J Infect Dis. (2021) 103:579–89. doi: 10.1016/j.ijid.2020.11.206
11. Dainton C, Hay A. Quantifying the relationship between lockdowns, mobility, and effective reproduction number (Rt) during the COVID-19 pandemic in the Greater Toronto Area. BMC Public Health. (2021) 21:1658. doi: 10.1186/s12889-021-11684-x
12. Oraby T, Tyshenko MG, Maldonado JC, Vatcheva K, Elsaadany S, Alali WQ, et al. Modeling the effect of lockdown timing as a COVID-19 control measure in countries with differing social contacts. Sci Rep. (2021) 11:3354. doi: 10.1038/s41598-021-82873-2
13. Al-Harbi SK, Al-Tuwairqi SM. Modeling the effect of lockdown and social distancing on the spread of COVID-19 in Saudi Arabia. PLoS One. (2022) 17:e0265779. doi: 10.1371/journal.pone.0265779
14. Guzzetta G, Riccardo F, Marziano V, Poletti P, Trentini F, Bella A, et al. Impact of a Nationwide Lockdown on SARS-CoV-2 Transmissibility, Italy. Emerg Infect Dis. (2021) 27:267–70. doi: 10.3201/eid2701.202114
15. Quach H-L, Nguyen KC, Hoang N-A, Pham TQ, Tran DN, Le MTQ, et al. Association of public health interventions and COVID-19 incidence in Vietnam, January to December 2020. Int J Infect Dis. (2021) 110:S28–43.
16. Jayaweera M, Dannangoda C, Dilshan D, Dissanayake J, Perera H, Manatunge J, et al. Grappling with COVID-19 by imposing and lifting non-pharmaceutical interventions in Sri Lanka: a modeling perspective. Infect Dis Model. (2021) 6:820–31. doi: 10.1016/j.idm.2021.06.004
17. Brauner JM, Mindermann S, Sharma M, Johnston D, Salvatier J, Gavenčiak T, et al. Inferring the effectiveness of government interventions against COVID-19. Science. (2021) 371:eabd9338. doi: 10.1126/science.abd9338
18. Dighe A, Cattarino L, Cuomo-Dannenburg G, Skarp J, Imai N, Bhatia S, et al. Response to COVID-19 in South Korea and implications for lifting stringent interventions. BMC Med. (2020) 18:321. doi: 10.1186/s12916-020-01791-8
19. Chan LYH, Yuan B, Convertino M. COVID-19 non-pharmaceutical intervention portfolio effectiveness and risk communication predominance. Sci Rep. (2021) 11:10605. doi: 10.1038/s41598-021-88309-1
20. Soltesz K, Gustafsson F, Timpka T, Jaldén J, Jidling C, Heimerson A, et al. The effect of interventions on COVID-19. Nature. (2020) 588:E26–8.
21. Islam N, Sharp SJ, Chowell G, Shabnam S, Kawachi I, Lacey B, et al. Physical distancing interventions and incidence of coronavirus disease 2019: natural experiment in 149 countries. BMJ. (2020) 370:m2743. doi: 10.1136/bmj.m2743
22. Teslya A, Pham TM, Godijk NG, Kretzschmar ME, Bootsma MCJ, Rozhnova G. Impact of self-imposed prevention measures and short-term government-imposed social distancing on mitigating and delaying a COVID-19 epidemic: a modelling study. PLoS Med. (2020) 17:e1003166. doi: 10.1371/journal.pmed.1003166
23. Di Domenico L, Pullano G, Sabbatini CE, Boëlle P-Y, Colizza V. Impact of lockdown on COVID-19 epidemic in Île-de-France and possible exit strategies. BMC Med. (2020) 18:240. doi: 10.1186/s12916-020-01698-4
24. Jarvis CI, Van Zandvoort K, Gimma A, Prem K, CMMID Covid-19 working group, Klepac P, et al. Quantifying the impact of physical distance measures on the transmission of COVID-19 in the UK. BMC Med. (2020) 18:124. doi: 10.1186/s12916-020-01597-8
25. Viner RM, Russell SJ, Croker H, Packer J, Ward J, Stansfield C, et al. School closure and management practices during coronavirus outbreaks including COVID-19: a rapid systematic review. Lancet Child Adolesc Health. (2020) 4:397–404. doi: 10.1016/S2352-4642(20)30095-X
26. Caristia S, Ferranti M, Skrami E, Raffetti E, Pierannunzio D, Palladino R, et al. Effect of national and local lockdowns on the control of COVID-19 pandemic: a rapid review. Epidemiol Prev. (2020) 44:60–8. doi: 10.19191/EP20.5-6.S2.104
27. Yang B, Huang AT, Garcia-Carreras B, Hart WE, Staid A, Hitchings MDT, et al. Effect of specific non-pharmaceutical intervention policies on SARS-CoV-2 transmission in the counties of the United States. Nat Commun. (2021) 12:3560.
28. Brooks-Pollock E, Read JM, McLean AR, Keeling MJ, Danon L. Mapping social distancing measures to the reproduction number for COVID-19. Philos Trans R Soc Lond B Biol Sci. (2021) 376:20200276. doi: 10.1098/rstb.2020.0276
29. Liu Y, Morgenstern C, Kelly J, Lowe R, Cmmid Covid-19 Working Group, Jit M. The impact of non-pharmaceutical interventions on SARS-CoV-2 transmission across 130 countries and territories. BMC Med. (2021) 19:40. doi: 10.1186/s12916-020-01872-8
30. Li Y, Campbell H, Kulkarni D, Harpur A, Nundy M, Wang X, et al. The temporal association of introducing and lifting non-pharmaceutical interventions with the time-varying reproduction number (R) of SARS-CoV-2: a modelling study across 131 countries. Lancet Infect Dis. (2021) 21:193–202. doi: 10.1016/S1473-3099(20)30785-4
31. Post RAJ, Regis M, Zhan Z, van den Heuvel ER. How did governmental interventions affect the spread of COVID-19 in European countries? BMC Public Health. (2021) 21:411. doi: 10.1186/s12889-021-10257-2
32. Rypdal K. The tipping effect of delayed interventions on the evolution of COVID-19 incidence. Int J Environ Res Public Health. (2021) 18:4484. doi: 10.3390/ijerph18094484
33. Müller SA, Balmer M, Charlton W, Ewert R, Neumann A. Predicting the effects of COVID-19 related interventions in urban settings by combining activity-based modelling, agent-based simulation, and mobile phone data. PLoS One. (2021) 16:e0259037. doi: 10.1371/journal.pone.0259037
34. Sun KS, Lau TSM, Yeoh EK, Chung VCH, Leung YS, Yam CHK, et al. Effectiveness of different types and levels of social distancing measures: a scoping review of global evidence from earlier stage of COVID-19 pandemic. BMJ Open. (2022) 12:e053938. doi: 10.1136/bmjopen-2021-053938
35. Grint DJ, Wing K, Williamson E, McDonald HI, Bhaskaran K, Evans D, et al. Case fatality risk of the SARS-CoV-2 variant of concern B.1.1.7 in England, 16 November to 5 February. Euro Surveill. (2021) 26:2100256. doi: 10.2807/1560-7917.ES.2021.26.11.2100256
36. Cabinet Secretariat New Coronavirus Infectious Disease Control Promotion Office.Public Announcement Regarding Priority Measures Such As Prevention of the Spread of New Coronavirus Infectious Disease. (2020). Available online at: https://corona.go.jp/emergency/ (Accessed October 20, 2021).
37. Davies NG, Jarvis CI, CMMID Covid-19 Working Group, Edmunds WJ, Jewell NP, Diaz-Ordaz K, et al. Increased mortality in community-tested cases of SARS-CoV-2 lineage B.1.1.7. Nature. (2021) 593:270–4.
38. Davies NG, Abbott S, Barnard RC, Jarvis CI, Kucharski AJ, Munday JD, et al. Estimated transmissibility and impact of SARS-CoV-2 lineage B.1.1.7 in England. Science. (2021) 372:eabg3055. doi: 10.1126/science.abg3055
39. Ministry of Health, Labour and Welfare of Japan. Cabinet Secretariat Countermeasures Against New Coronavirus Infectious Diseases. (2021). Available online at: https://corona.go.jp/emergency/ (accessed June 20, 2022).
40. Kosfeld R, Mitze T, Rode J, Wälde K. The Covid-19 containment effects of public health measures: a spatial difference-in-differences approach. J Reg Sci. (2021). [Online ahead of print]. doi: 10.1111/jors.12536
41. Chang S, Pierson E, Koh PW, Gerardin J, Redbird B, Grusky D, et al. Mobility network models of COVID-19 explain inequities and inform reopening. Nature. (2021) 589:82–7. doi: 10.1038/s41586-020-2923-3
42. Li T, Liu Y, Li M, Qian X, Dai SY. Mask or no mask for COVID-19: a public health and market study. PLoS One. (2020) 15:e0237691. doi: 10.1371/journal.pone.0237691
43. Kuniya T. Evaluation of the effect of the state of emergency for the first wave of COVID-19 in Japan. Infect Dis Model. (2020) 5:580–7.
44. Volz E, Mishra S, Chand M, Barrett JC, Johnson R, Geidelberg L, et al. Assessing transmissibility of SARS-CoV-2 lineage B.1.1.7 in England. Nature. (2021) 593:266–9.
45. Kissler SM, Tedijanto C, Goldstein E, Grad YH, Lipsitch M. Projecting the transmission dynamics of SARS-CoV-2 through the postpandemic period. Science. (2020) 368:860–8. doi: 10.1126/science.abb5793
46. Gog JR, Hill EM, Danon L, Thompson RN. Vaccine escape in a heterogeneous population: insights for SARS-CoV-2 from a simple model. R Soc Open Sci. (2021) 8:210530. doi: 10.1098/rsos.210530
47. Ministry of Health, Labour and Welfare of Japan. Guidelines for the Use of Kits for Detection of SARS-CoV-2 Antigen. (2021). Available online at: https://www.mhlw.go.jp/content/000630270.pdf (accessed June 20, 2022).
48. Ministry of Health, Labour and Welfare of Japan. Information Grasping and Management Support System for People Infected With the New Coronavirus (HERSYS). (2021). Available online at: https://www.mhlw.go.jp/stf/seisakunitsuite/bunya/0000121431_00129.html (accessed June 20, 2022).
49. Ministry of Health, Labour and Welfare of Japan. Report on the Number of Tests for New Coronavirus Infection. (2022). Available online at: https://www.mhlw.go.jp/content/000740117.pdf (accessed June 20, 2022).
50. National Institute of Infectious Diseases. New Mutant Strain of a Novel Coronavirus (SARS-CoV-2) of Concern for Increased Infection and Transmissibility and Antigenic Changes (Report 8). (2021). Available online at: https://www.niid.go.jp/niid/ja/2019-ncov/2484-idsc/10280-covid19-41.html (accessed June 20, 2022).
51. Ministry of Health, Labour and Welfare of Japan. Ministry of Health, Labour and Welfare: Outbreak in Japan. (2021). Available online at: https://www.mhlw.go.jp/stf/covid-19/kokunainohasseijoukyou.html#h2_1 (accessed June 20, 2022).
52. Ministry of Health, Labour and Welfare of Japan. Survey on Medical Treatment Status, Number of Beds Accepted by Inpatients, etc. (2021). Available online at: https://www.mhlw.go.jp/stf/seisakunitsuite/newpage_00023.html (accessed June 20, 2022).
53. Nakajo K, Nishiura H. Assessing interventions against Coronavirus Disease 2019 (COVID-19) in Osaka, Japan: a modeling study. J Clin Med Res. (2021) 10:1256. doi: 10.3390/jcm10061256
54. Nakajo K, Nishiura H. Estimation of R(t) based on illness onset data: an analysis of 1907-1908 smallpox epidemic in Tokyo. Epidemics. (2022) 38:100545. doi: 10.1016/j.epidem.2022.100545
55. He X, Lau EHY, Wu P, Deng X, Wang J, Hao X, et al. Temporal dynamics in viral shedding and transmissibility of COVID-19. Nat Med. (2020) 26:672–5. doi: 10.1038/s41591-020-0869-5
56. Li Q, Guan X, Wu P, Wang X, Zhou L, Tong Y, et al. Early transmission dynamics in Wuhan, China, of novel coronavirus-infected pneumonia. N Engl J Med. (2020) 382:1199–207. doi: 10.1056/NEJMoa2001316
57. Murayama H, Kayano T, Nishiura H. Estimating COVID-19 cases infected with the variant alpha (VOC 202012/01): an analysis of screening data in Tokyo, January-March 2021. Theor Biol Med Model. (2021) 18:13. doi: 10.1186/s12976-021-00146-x
58. Furuse Y, Ko YK, Ninomiya K, Suzuki M, Oshitani H. Relationship of test positivity rates with COVID-19 epidemic dynamics. Int J Environ Res Public Health. (2021) 18:4655. doi: 10.3390/ijerph18094655
59. Kinoshita R, Anzai A, Jung S-M, Linton NM, Miyama T, Kobayashi T, et al. Containment, contact tracing and asymptomatic transmission of novel Coronavirus Disease (COVID-19): a modelling study. J Clin Med Res. (2020) 9:3125. doi: 10.3390/jcm9103125
60. Polack FP, Thomas SJ, Kitchin N, Absalon J, Gurtman A, Lockhart S, et al. Safety and Efficacy of the BNT162b2 mRNA Covid-19 Vaccine. N Engl J Med. (2020) 383:2603–15.
61. Ito K, Piantham C, Nishiura H. Predicted dominance of variant Delta of SARS-CoV-2 before Tokyo Olympic Games, Japan, July 2021. Euro Surveill. (2021) 26:2100570. doi: 10.2807/1560-7917.ES.2021.26.27.2100570
62. Campbell F, Archer B, Laurenson-Schafer H, Jinnai Y, Konings F, Batra N, et al. Increased transmissibility and global spread of SARS-CoV-2 variants of concern as at June 2021. Euro Surveill. (2021) 26:2100509. doi: 10.2807/1560-7917.ES.2021.26.24.2100509
63. Smith TP, Flaxman S, Gallinat AS, Kinosian SP, Stemkovski M, Unwin HJT, et al. Temperature and population density influence SARS-CoV-2 transmission in the absence of nonpharmaceutical interventions. Proc Natl Acad Sci USA. (2021) 118:e2019284118. doi: 10.1073/pnas.2019284118
64. Wood SN, Wit EC. Was R < 1 before the English lockdowns? On modelling mechanistic detail, causality and inference about Covid-19. PLoS One. (2021) 16:e0257455. doi: 10.1371/journal.pone.0257455
65. Chin V, Ioannidis JPA, Tanner MA, Cripps S. Effect estimates of COVID-19 non-pharmaceutical interventions are non-robust and highly model-dependent. J Clin Epidemiol. (2021) 136:96–132. doi: 10.1016/j.jclinepi.2021.03.014
66. Arroyo-Marioli F, Bullano F, Kucinskas S, Rondón-Moreno C. Tracking of COVID-19: a new real-time estimation using the Kalman filter. PLoS One. (2021) 16:e0244474. doi: 10.1371/journal.pone.0244474
67. Jung S-M, Endo A, Akhmetzhanov AR, Nishiura H. Predicting the effective reproduction number of COVID-19: inference using human mobility, temperature, and risk awareness. Int J Infect Dis. (2021) 113:47–54. doi: 10.1016/j.ijid.2021.10.007
68. Olney AM, Smith J, Sen S, Thomas F, Unwin HJT. Estimating the Effect of Social Distancing Interventions on COVID-19 in the United States. Am J Epidemiol. (2021) 190:1504–9.
69. Hyde Z. Difference in SARS-CoV-2 attack rate between children and adults may reflect bias. Clin Infect Dis. (2021) 74:152–5. doi: 10.1093/cid/ciab183
70. Gurdasani D, Alwan NA, Greenhalgh T, Hyde Z, Johnson L, McKee M, et al. School reopening without robust COVID-19 mitigation risks accelerating the pandemic. Lancet. (2021) 397:1177–8. doi: 10.1016/S0140-6736(21)00622-X
71. Di Domenico L, Pullano G, Sabbatini CE, Boëlle P-Y, Colizza V. Modelling safe protocols for reopening schools during the COVID-19 pandemic in France. Nat Commun. (2021) 12:1073. doi: 10.1038/s41467-021-21249-6
72. Vlachos J, Hertegård E, Svaleryd HB. The effects of school closures on SARS-CoV-2 among parents and teachers. Proc Natl Acad Sci USA. (2021) 118:e2020834118. doi: 10.1073/pnas.2020834118
73. Pei S, Kandula S, Shaman J. Differential effects of intervention timing on COVID-19 spread in the United States. Sci Adv. (2020) 6:eabd6370. doi: 10.1126/sciadv.abd6370
74. Laydon DJ, Mishra S, Hinsley WR, Samartsidis P, Flaxman S, Gandy A, et al. Modelling the impact of the tier system on SARS-CoV-2 transmission in the UK between the first and second national lockdowns. BMJ Open. (2021) 11:e050346. doi: 10.1136/bmjopen-2021-050346
75. Duan T, Jiang H, Deng X, Zhang Q, Wang F. Government Intervention, Risk Perception, and the Adoption of Protective Action Recommendations: evidence from the COVID-19 Prevention and Control Experience of China. Int J Environ Res Public Health. (2020) 17:3387. doi: 10.3390/ijerph17103387
76. Hunter PR, Colón-González FJ, Brainard J, Rushton S. Impact of non-pharmaceutical interventions against COVID-19 in Europe in 2020: a quasi-experimental non-equivalent group and time series design study. Euro Surveill. (2021) 26:2001401. doi: 10.2807/1560-7917.ES.2021.26.28.2001401
Keywords: coronavirus, statistical estimation, modeling, Infectious Disease, mathematical model, effective reproduction number
Citation: Hayashi K, Kayano T, Anzai A, Fujimoto M, Linton N, Sasanami M, Suzuki A, Kobayashi T, Otani K, Yamauchi M, Suzuki M and Nishiura H (2022) Assessing Public Health and Social Measures Against COVID-19 in Japan From March to June 2021. Front. Med. 9:937732. doi: 10.3389/fmed.2022.937732
Received: 06 May 2022; Accepted: 13 June 2022;
Published: 12 July 2022.
Edited by:
Reza Lashgari, Shahid Beheshti University, IranReviewed by:
Melanie Cash, University of Florida, United StatesMagdalena Djordjevic, University of Belgrade, Serbia
Igor Salom, University of Belgrade, Serbia
Gilberto Gonzalez-Parra, New Mexico Tech, United States
Copyright © 2022 Hayashi, Kayano, Anzai, Fujimoto, Linton, Sasanami, Suzuki, Kobayashi, Otani, Yamauchi, Suzuki and Nishiura. This is an open-access article distributed under the terms of the Creative Commons Attribution License (CC BY). The use, distribution or reproduction in other forums is permitted, provided the original author(s) and the copyright owner(s) are credited and that the original publication in this journal is cited, in accordance with accepted academic practice. No use, distribution or reproduction is permitted which does not comply with these terms.
*Correspondence: Hiroshi Nishiura, nishiura.hiroshi.5r@kyoto-u.ac.jp