- 1Unidad de Cardiología Intervencionista, Servicio de Cardiología, Hospital del Mar, Barcelona, Spain
- 2Biomedical Research in Heart Diseases (GREC), Instituto Hospital del Mar de Investigaciones Médicas (IMIM), Barcelona, Spain
- 3Servicio de Cardiología, Virgen del Rocío, Sevilla, Spain
- 4Servicio de Cardiología, Hospital Clínico Universitario de Valladolid, Valladolid, Spain
- 5Centro de investigación Biomédica en Red de Enfermedades Cardiovasculares (CIBERCV), Madrid, Spain
Coronavirus disease (COVID-19) is an infectious disease caused by the severe acute respiratory syndrome coronavirus 2 (SARS-CoV-2), with systemic organ damage in the most severe forms. Long-term complications of SARS-CoV-2 appear to be restricted to severe presentations of COVID-19, but many patients with persistent symptoms have never been hospitalized. Post-acute sequelae of COVID-19 (PASC) represents a heterogeneous group of symptoms characterized by cardiovascular, general, respiratory, and neuropsychiatric sequelae. The pace of evidence acquisition with PASC has been rapid, but the mechanisms behind it are complex and not yet fully understood. In particular, exercise intolerance shares some features with other classic respiratory and cardiac disorders. However, cardiopulmonary exercise testing (CPET) provides a comprehensive assessment and can unmask the pathophysiological mechanism behind exercise intolerance in gray-zone PASC. This mini-review explores the utility of CPET and aims to provide a comprehensive assessment of PASC by summarizing the current evidence.
Introduction
Long Coronavirus disease 2019 (COVID-19) or post-acute sequelae of severe acute respiratory syndrome coronavirus 2 infection (PASC) is expected to increase in prevalence and become a public health problem (1, 2). PASC is a heterogeneous clinical syndrome. The growing scientific evidence recognizes PASC as one of the conditions that cause exercise intolerance (2), but the relationships between severe acute respiratory syndrome coronavirus 2 (SARS-CoV-2) and exercise capacity remain unclear. A diminished exercise capacity has been associated with a poor quality of life and higher mortality in other conditions. Therefore, understanding the mechanism behind the limitation in exercise capacity of these patients is a fundamental step in improving patient outcomes.
A hallmark of exercise intolerance is dyspnea and fatigue upon exertion. Although it is intuitive to think that patients with PASC would be limited primarily by the cardiopulmonary system, studies indicate that most of these patients have (2) cardiac and pulmonary testing within normal values (3, 4). Exercise is dependent on the balance between oxygen supply, oxygen consumption, and clearance of toxic metabolites. These processes rely on the cardiovascular and pulmonary systems to achieve optimal exercise performance. Therefore, by studying external respiration in response to exercise, it is possible to address the functional competence of the organ systems by coupling external adjustments to cellular respiration. Cardiopulmonary exercise testing (CPET) offers the opportunity to study the cellular, cardiovascular, and ventilatory systems' responses simultaneously, providing an objective evaluation of exercise capacity (5, 6).
This contemporary review focuses on the essential role of CPET in the evaluation of patients with PASC and the potential mechanism behind exercise intolerance. Therefore, we explore and summarize the currently available evidence to increase awareness of this entity and improve the quality of care.
Post-Acute Sequelae of SARS-CoV-2 Infection
PASC represents the long-term sequelae of COVID-19 and it is classified according to the time frame of symptom persistence into a subacute (4–12 weeks) or chronic phase (>12 weeks) (1). PASC occurs in a heterogeneous group of patients with different clinical presentations (2), but it is characterized by a systemic involvement with the ability to impair patients' quality of life (4). Recent studies have looked at risk factors contributing to PASC observing an association with symptom burden during an active infection, female gender, and COVID-19 severity (7, 8).
The American College of Cardiology classifies PASC into two groups whether there is objective evidence of cardiovascular disease. Accordingly, PASC-cardiovascular disease is characterized by myocardial, pericardial, vascular, and/or arrhythmic conditions that appear beyond 4 weeks from the initial SARS-CoV-2 infection. Whereas, the term PASC-cardiovascular syndrome (PASC-CVS) is defined by the absence of cardiovascular disease, but on the contrary, also by the persistence of cardio-pulmonary symptoms. The two most commonly reported symptoms are fatigue and dyspnea, regardless of PASC time (9). Both are common in non-COVID patients with other cardiopulmonary conditions (10, 11) and in the convalescence phase of any critical illness (12), where exercise intolerance is also a characteristic feature (10, 11). Therefore, we should expect a high prevalence of exercise intolerance among COVID-19 survivors. Data regarding the pathophysiologic mechanism behind PASC-CVS are scarce, but it is not yet fully understood how this translates into reduced exercise capacity.
Evidence of Exercise Capacity in PASC-CVS
Despite there are no dedicated guidelines on the evaluation of PASC-CVS, patients should undergo a CPET evaluation to identify limiting factors for decreased maximal exertion that is usually found during the convalescence phase of any critical illness (12). Otherwise, the lack of evidence of residual cardiopulmonary damage may cause a delay in the diagnosis. Exercise capacity is evaluated through the assessment of oxygen consumption (Vo2) at peak exercise if a sustained maximal effort has been reached. Maximal performance is also age-, sex- and weight-adjusted to be reported as a percentage predictive of peak Vo2. Moreover, ventilatory threshold (VT) is effort-independent providing a more accurate assessment of aerobic efficiency and reflecting the Vo2 at submaximal exercise levels when supply does not match requirements triggering an anaerobic environment (5, 6).
Follow-up studies on SARS-CoV survivors observed a diminished exercise capacity (13, 14), but similar findings were observed during hospital discharge because of COVID-19 (15). To date, there is only scarce observational data on exercise capacity with PASC-CVS during follow-up. A small single-center retrospective study found during short-term follow-up a diminished exercise capacity (16). Similar findings were reported by a multi-center retrospective study of 200 patients, in which those with PASC (56%) had a lower peak Vo2 (25.8 ± 8.1 vs. 28.8 ± 9.6 mL/min/kg; p = 0.017) and a smaller chance of achieving the VT (OR: 0.38; 95% CI 0.20–0.72) (17). On the contrary, mid-to-long-term retrospective studies identify border-line exercise capacity in PASC patients (18) that was not different from controls with unexplained dyspnea (19).
These data were reproduced by prospective studies during short-term follow-up (20–24) and when stratified by COVID-19 severity no differences were found between groups (25); however, other studies reported that previous critical care unit admission, need for mechanical ventilation and a longer hospital stay were independently associated with peak Vo2 (26). The incorporation of unexplained dyspnea in PASC-CVS into the design and analysis of recent studies have yielded similar findings during follow-up. A single-center prospective study of 70 consecutive patients observed that PASC-CVS patients with persistent dyspnea (59%) experienced a smaller exercise capacity (78 vs. 99% of predicted peak Vo2; p < 0.001) than asymptomatic COVID-19 survivors (27). Accordingly, a multicenter prospective study that evaluated 156 patients also reported among PASC patients with persistent dyspnea (47%) a diminished exercise capacity (76 ± 16 vs. 89 ± 18% of predicted peak Vo2; p = 0.009) (28).
An unexplored scenario is the possibility of an immediate improvement in the functional capacity of PASC patients. A prospective study that monitored the persistence of exercise intolerance in PASC with serial CPET evaluation reported an improvement between 3-and-6 months of peak Vo2 (18 vs. 20.5 mL/kg/min; p = 0.001) and VT (9.7 vs. 10.4 mL/min/kg; p = 0.018). However, these improvements were not observed in all patients and were less evident when compared to healthy controls (29). Findings from other prospective studies confirm that exercise intolerance is also observed during mid-to-long term follow-up in PASC (30–35).
In general, low peak Vo2 is common among patients with PASC-CVS during follow-up (Table 1), but application and interpretation of CPET results are challenging. Peak Vo2 is defined by the Fick equation as the product of cardiac output and arteriovenous oxygen difference [C (a–v) O2]. This is important because cardiac output is the product of stroke volume times heart rate (HR) and arteriovenous oxygen difference reflects the peripheral oxygen tissue extraction (5, 6). Consequently, abnormalities in any of these variables can contribute to exercise intolerance in PASC-CVS.
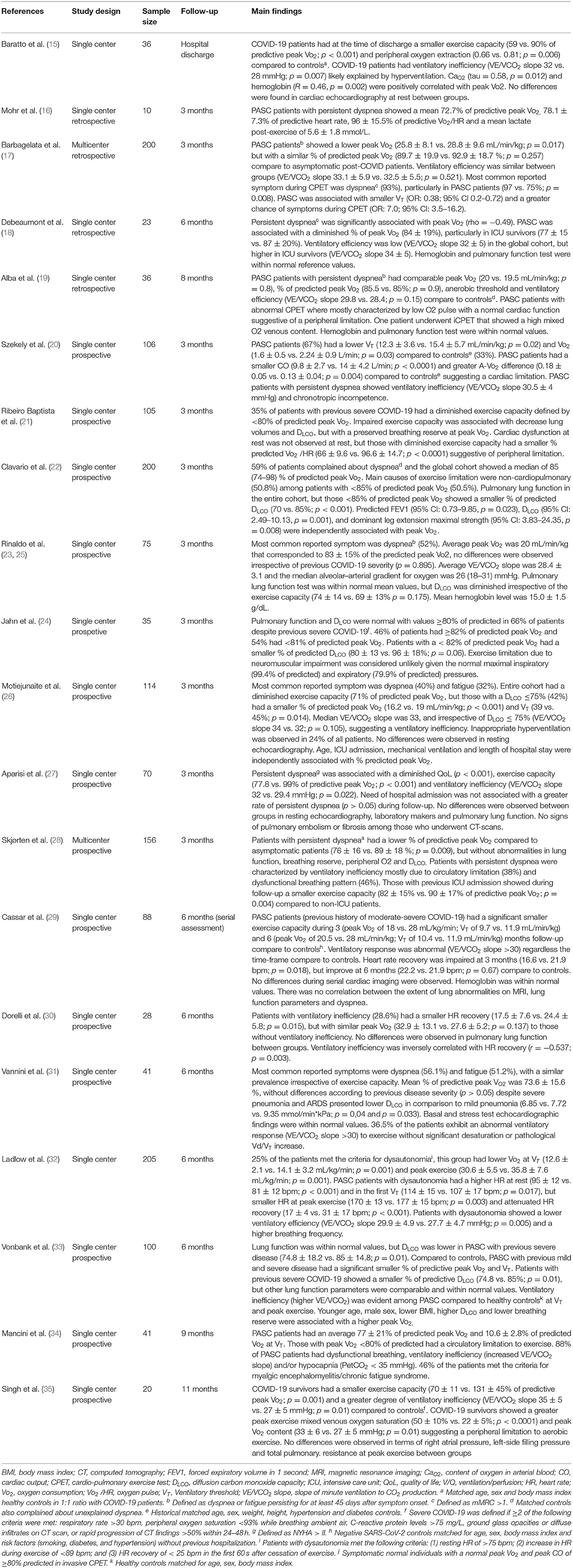
Table 1. Most Relevant Studies evaluating exercise capacity in Post-acute sequelae of COVID-19 with cardiopulmonary exercise test.
Contributors of Exercise Intolerance in PASC-CVS
Identification of patterns during CPET may identify the organ systems involved in the exercise intolerance referred by PASC patients as we cannot rely exclusively on a decreased peak Vo2 and VT (see Graphical Abstract). Therefore, CPET can be combined with the invasive and non-invasive tests to further phenotype more accurately PASC-CVS. However, given the systemic nature of COVID-19, we may expect a cardiac, ventilatory, peripheral, and/or pulmonary gas exchange limitation at exercise.
Cardiovascular Limitation
Cardiovascular limitation to exercise intolerance in PASC-CVS patients may be explained by several factors, but electrocardiographic changes and a pathological blood pressure response during exercise have not yet been reported. Moreover, low CO could explain exercise intolerance in most PASC patients; however, no left ventricular dysfunction has been reported in the studies that evaluated cardiac function at rest (15, 21, 26, 27, 29). Similarly, two prospective studies that evaluated cardiac function at rest and during CPET concluded that cardiac function was within normal values, regardless of previous COVID-19 severity (20, 31). However, Szekely et al. also observed a reduced stroke volume with a blunted peak HR and a higher peak arteriovenous difference among PASC patients (20), raising the possibility of a cardiac autonomic dysregulation as a major cause of exercise intolerance.
Modulation of the HR during exercise is a dynamic process tightly regulated by the autonomic nervous system and its imbalance may manifest during exercise as chronotropic incompetence or inadequate HR recovery. Some of the studies reported chronotropic incompetence (16, 20), while others observed an abnormal HR recovery (29, 30, 32). Interestingly, both were more commonly observed among PASC patients with evidence of ventilatory inefficiency.
Ventilatory and Pulmonary Vascular Limitation
Lung mechanical-related mechanism because of significant reduction of pulmonary function should be, in theory, the expected primary cause of exertional dyspnea in PASC. Contrary to that, most of the studies did not observe a correlation between abnormal lung functions and persistent dyspnea regardless of COVID-19 severity. This is further supported by normal breathing reserve among PASC patients (18, 19, 23, 27, 28, 30, 33). However, some studies reported a significant decrease in DLCO showed some discordant findings concerning peak VO2 (21, 22, 26, 29, 31, 33).
Under normal conditions, ventilation increases proportionally to CO2 production (36) but a common finding from the CPET of PASC patients is the ventilatory inefficiency (increased VE/VCO2 slope) suggesting an abnormal response (15, 17, 18, 20, 26–28, 30, 33, 35). Multiple mechanisms can explain it, but PASC may present with a characteristic pattern observed in pulmonary vascular or interstitial diseases (36). Pulmonary hypertension is typically seen with a diminished partial pressure of end-tidal CO2 (37), which has also been reported in PASC patients with ventilatory inefficiency (27, 34, 35). However, several findings argue against this hypothesis. First, despite COVID-19 being associated with pulmonary embolism or right ventricular dysfunction (38), none of the studies reported such findings in PASC patients (26, 27, 29, 31). Second, those studies with stress test echocardiogram or invasive CPET did not observe signs of exercise-induced pulmonary hypertension with exception of a few isolated cases (34, 35). Third, an increase in the physiological dead space/tidal volume ratio was not observed (15, 18, 30, 31, 34, 35) when a raise is expected with severe ventilation-perfusion mismatching (37). Finally, no peripheral oxygen desaturation was reported even in those with pathological DLCO (21–26, 33).
More recently, hyperventilation syndrome has been suggested to occur in PASC patients (26) given the increase VE/VCO2 and low PETCO2 observe during exercise without clear evidence of cardio-pulmonary diseases (39). Therefore, dysfunctional breathing characterized by exercise-induced hyperpnea may explain the persistence of symptoms in PASC-CVS.
Peripheral Limitation
The peripheral limitation has also been postulated as contributing to PASC. Alba et al. (19) found that a great number of PASC patients with abnormal CPET showed a low O2 pulse with a normal cardiac function, suggesting a peripheral limitation. In the same way, Ribeiro Baptista et al. (21) didn't observe cardiac dysfunction at rest, but those with diminished exercise capacity had a smaller predicted O2 pulse suggestive of peripheral limitation. As already mentioned, according to the Fick equation (peak VO2 is defined as the product of cardiac output and arteriovenous oxygen difference), a depressed peak VO2 can be the result of a blunted cardiac output response (impair oxygen delivery), and impaired peripheral oxygen extraction (diffusion defect) or both (5, 6).
In this sense, Singh et al. (35) performed invasive cardiopulmonary exercise testing on 10 patients who had recovered from COVID-19. These patients, in contrast to the control group, showed an increased peak exercise mixed venous oxygen saturation and peak venous O2 content. The authors concluded that the impaired oxygen extraction was attributed primarily to reduced oxygen diffusion in the peripheral microcirculation, exhibiting a peripheral limitation to aerobic exercise.
Underlying anemia can contribute to both reduced systemic oxygen delivery and extraction (5, 6). Several studies collected hemoglobin levels, with the mean being within normal ranges (18–20, 23, 27, 35), ruling out the presence of anemia as a contributing factor to reduced peak VO2 found in these patients. However, one study from Baratto et al. (15) found underlying anemia in patients who had recovered from COVID-19 at the time of hospital discharge. This reduction in hemoglobin levels leads to reduced arterial O2 content and therefore to a lower O2 delivery and reduced peak VO2.
Discussion
PASC is a disorder that occurs irrespective of previous disease severity and is characterized by a myriad of conditions and symptoms. Data suggest that dyspnea, fatigue, and exercise intolerance are the most common referred symptoms during outpatient follow-up. PASC cardiovascular disease is associated with structural or functional cardiovascular abnormalities that may explain the persistence of symptoms, but a non-negligible number of patients have no objective evidence of organ involvement. Therefore, PASC-CVS represents a heterogeneous group of patients with persistent symptoms that generally present a normal cardiopulmonary function (9). Because of a paucity of data, not much attention has been given to CPET despite its application can accurately evaluate PASC-CVS and improve the quality of care for these patients.
PASC-CVS is associated with an objective reduction of the exercise capacity during CPET (15–35). A long list of conditions can lead to poor physical conditions referred by PASC-CVS patients. Among all the potential causes and CPET variables, the following stand out: chronotropic incompetence, abnormal heart rate recovery, ventilatory inefficiency (high VE/VCO2 and low PETCO2), and diminished peripheral oxygen extraction. Patients presenting with pulmonary vasculopathy or interstitial lung disease demonstrate similar findings during CPET (36). PASC-CVS may share a common mechanism, but data from follow-up studies do not support that hypothesis as most show no evidence of cardio-pulmonary sequelae (15–35).
Therefore, given all key factors in determining oxygen availability (5, 6), it seems that the cornerstone of PASC-CVS may involve a peripheral limitation. This is further supported by invasive CPET findings, where a diminished peripheral tissue extraction during exercise led to a decreased exercise capacity (35). Notably, hyperventilation leads to a leftward shift of the hemoglobin oxygen affinity that is translated into a decreased O2 unloading and impaired diffusion (5, 6). However, such impaired diffusion could also be explained by direct damage to the endothelium (40) leading to exercise intolerance as observed in chronic fatigue syndrome (41). Similarly, endothelial dysfunction has been reported in PASC with and without chronic fatigue syndrome (42). Interestingly, all the aforementioned factors may be linked to autonomic dysregulation (43, 44).
Dysfunctional breathing has been widely described among PASC patients (45), with hyperventilation being characterized by a decreased exercise capacity and signs of ventilatory inefficiency without evidence of cardio-pulmonary dysfunction. Interestingly, a high respiratory rate causes sympathetic activation and vagal withdrawal leading to exercise intolerance not only through an impaired O2 diffusion but also through a diminished O2 delivery (43). The impairment in O2 delivery is supported by the evidence of cardiac autonomic dysfunction among PASC patients (32, 46, 47), where ventilatory inefficiency was also a common finding (16, 20). However, PASC-CVS may also manifest as dysfunctional breathing with a chaotic ventilatory pattern with normal peak VO2, PETCO2, and VE/VCO2 during CPET (48).
Finally, evidence of autonomic dysfunction in PASC is further supported by recent studies suggesting that some patients present with signs and symptoms suggestive of postural orthostatic tachycardia syndrome (49). Indeed, postural orthostatic tachycardia syndrome can explain the CPET findings as it is associated with sympathetic stimulation, vasoconstriction, and hyperpnea (50).
Thus, the most appropriate hypothesis seems to be cardiac and peripheral autonomic dysregulation creating a vicious cycle that alters the exercise capacity in PASC-CVS. Nevertheless, it is difficult to draw any definitive conclusions, as the observations might be time-sensitive. In addition, none of the studies reported the baseline physical activity and physiological status of individuals before getting COVID-19, which raises the possibility of a cause-effect bias.
Prognostic Utility of CPET in PASC-CVS
PASC-CVS is expected to become a major challenge as most recent findings suggest that it shares some features with chronic fatigue syndrome (50). Although younger age and shorter time since COVID-19 have been recently described as potential predictors of submaximal CPET in PASC (51), there are no published studies examining the long-term prognostic value unless some ideas are extrapolated from previous studies. In particular, in heart failure patients a peak Vo2 >14 ml/kg/min is associated with smaller 1-year mortality (52). Similarly, a high VE/VCO2 slope is also associated with the worst clinical outcomes among cardiac and pulmonary patients (53) with recent studies suggesting that a high VE/VCO2 in PASC-CVS is an independent predictor for endothelial dysfunction (54). Endothelial dysfunction has been associated with the worst outcomes in other medical conditions (44). Therefore, the presence of a diminished peak Vo2 or high VE/VCO2 slope could be associated with an increased risk of death during follow-up.
Interestingly, there is growing evidence that autonomic dysfunction might a fundamental factor in the observed symptoms in PASC-CVS (49). Theoretically, we could speculate about the potential utility of HR dynamics assessment in this group of patients during maximal effort and recovery. Previous studies have noted that chronotropic incompetence is associated with poor outcomes in heart failure patients (55). Furthermore, the detection of a heart rate recovery of ≤12 beats per minute is a strong predictor of all-cause mortality (RR: 2; 95% CI 1.5–2.7; p < 0.001) irrespective of previous cardiovascular risk factors and even in the absence of heart failure or myocardial perfusion defects (56). Nevertheless, risk stratification for PASC-CVS is limited. Thus, future studies with CPET both at baseline and follow-up are expected and will provide a more reliable estimation of long-term clinical outcomes in these patients with a special emphasis on previously known prognostic factors.
Conclusions
Our current understanding of PASC is vague, but exercise limitation is a common finding despite the absence of objective cardio-pulmonary sequelae in PASC-CVS. Physiological assessment with CPET may provide valuable information about the functional status of these patients and identify the potential pathogenic mechanism. Autonomic dysfunction might be the missing link. Future studies evaluating predictors of exercise intolerance and long-term prognosis are warranted, as it could have a positive effect on disease evolution and clinical outcomes.
Author Contributions
ÁA, JT, and JASR contributed to conception of the work. ÁA wrote the first draft of the manuscript. ÁA, RL, and CY-F wrote sections of the manuscript. All authors contributed to manuscript revision, read, and approved the submitted version.
Conflict of Interest
The authors declare that the research was conducted in the absence of any commercial or financial relationships that could be construed as a potential conflict of interest.
Publisher's Note
All claims expressed in this article are solely those of the authors and do not necessarily represent those of their affiliated organizations, or those of the publisher, the editors and the reviewers. Any product that may be evaluated in this article, or claim that may be made by its manufacturer, is not guaranteed or endorsed by the publisher.
Abbreviations
COVID-19, coronavirus disease 2019; CPET, cardiopulmonary exercise test; HR, heart rate; PASC, postacute sequelae of COVID-19; PASC-CVS, postacute sequelae of COVID-19 cardiovascular syndrome; Vo2, oxygen consumption; VT, anaerobic or ventilatory threshold; VE/VCO2, minute ventilation/CO2 output.
References
1. Nalbandian A, Sehgal K, Gupta A, Madhavan MV, McGroder C, Stevens JS, et al. Post-acute COVID-19 syndrome. Nat Med. (2021) 27:601–15. doi: 10.1038/s41591-021-01283-z
2. Alkodaymi MS, Omrani OA, Fawzy NA, Shaar BA, Almamlouk R, Riaz M, et al. Prevalence of post-acute COVID-19 syndrome symptoms at different follow-up periods: a systematic review and meta-analysis. Clin Microbiol Infec. (2022) 28:657–66. doi: 10.1016/j.cmi.2022.01.014
3. Crook H, Raza S, Nowell J, Young M, Edison P. Long covid—mechanisms, risk factors, and management. BMJ. (2021) 374:n1648. doi: 10.1136/bmj.n1648
4. Jiang DH, Roy DJ, Gu BJ, Hassett LC, McCoy RG. Postacute sequelae of severe acute respiratory syndrome coronavirus 2 infection. Jacc Basic Transl Sci. (2021) 6:796–811. doi: 10.1016/j.jacbts.2021.07.002
5. Balady GJ, Arena R, Sietsema K, Myers J, Coke L, Fletcher GF, et al. Clinician's guide to cardiopulmonary exercise testing in adults. Circulation. (2010) 122:191–225. doi: 10.1161/CIR.0b013e3181e52e69
6. Glaab T, Taube C. Practical guide to cardiopulmonary exercise testing in adults. Respir Res. (2022) 23:9. doi: 10.1186/s12931-021-01895-6
7. Tleyjeh IM, Saddik B, Ramakrishnan RK, AlSwaidan N, AlAnazi A, Alhazmi D, et al. Long term predictors of breathlessness, exercise intolerance, chronic fatigue and well-being in hospitalized patients with COVID-19: a cohort study with 4 months median follow-up. J Infect Public Heal. (2022) 15:21–8. doi: 10.1016/j.jiph.2021.11.016
8. Bai F, Tomasoni D, Falcinella C, Barbanotti D, Castoldi R, Mulè G, et al. Female gender is associated with long COVID syndrome: a prospective cohort study. Clin Microbiol Infec. (2022) 28:611.e9–611.e16. doi: 10.1016/j.cmi.2021.11.002
9. Committee W, Gluckman TJ, Bhave NM, Allen LA, Chung EH, Spatz ES, et al. 2022 ACC expert consensus decision pathway on cardiovascular sequelae of COVID-19 in adults: myocarditis and other myocardial involvement, post-acute sequelae of SARS-CoV-2 infection, and return to play. J Am Coll Cardiol. (2022) 79:1717–56. doi: 10.1016/j.jacc.2022.02.003
10. Molgat-Seon Y, Schaeffer MR, Ryerson CJ, Guenette JA. Exercise pathophysiology in interstitial lung disease. Clin Chest Med. (2019) 40:405–20. doi: 10.1016/j.ccm.2019.02.011
11. Buono MGD, Arena R, Borlaug BA, Carbone S, Canada JM, Kirkman DL, et al. Exercise intolerance in patients with heart failure JACC state-of-the-art review. J Am Coll Cardiol. (2019) 73:2209–25. doi: 10.1016/j.jacc.2019.01.072
12. Sangla F, Legouis D, Marti P-E, Sgardello SD, Brebion A, Saint-Sardos P, et al. One year after ICU admission for severe community-acquired pneumonia of bacterial, viral or unidentified etiology. What are the outcomes? PLoS ONE. (2020) 15:e0243762. doi: 10.1371/journal.pone.0243762
13. Hui DS, Wong KT, Ko FW, Tam LS, Chan DP, Woo J, et al. The 1-year impact of severe acute respiratory syndrome on pulmonary function, exercise capacity, and quality of life in a cohort of survivors. Chest. (2005) 128:2247–61. doi: 10.1378/chest.128.4.2247
14. Ong K-C, Ng AW-K, Lee LS-U, Kaw G, Kwek S-K, Leow MK-S, et al. Pulmonary function and exercise capacity in survivors of severe acute respiratory syndrome. Eur Respir J. (2004) 24:436–42. doi: 10.1183/09031936.04.00007104
15. Baratto C, Caravita S, Faini A, Perego GB, Senni M, Badano LP, et al. Impact of COVID-19 on exercise pathophysiology: a combined cardiopulmonary and echocardiographic exercise study. J Appl Physiol. (2021) 130:1470–8. doi: 10.1152/japplphysiol.00710.2020
16. Mohr A, Dannerbeck L, Lange TJ, Pfeifer M, Blaas S, Salzberger B, et al. Cardiopulmonary exercise pattern in patients with persistent dyspnoea after recovery from COVID-19. Multidiscip Resp Med. (2021) 16:732. doi: 10.4081/mrm.2021.732
17. Barbagelata L, Masson W, Iglesias D, Lillo E, Migone JF, Orazi ML, et al. Cardiopulmonary exercise testing in patients with post-COVID-19 syndrome. Med Clin-barcelona. (2021) 159:6–11. doi: 10.1016/j.medcli.2021.07.007
18. Debeaumont D, Boujibar F, Ferrand-Devouge E, Artaud-Macari E, Tamion F, Gravier F-E, et al. Cardiopulmonary exercise testing to assess persistent symptoms at 6 months in people with COVID-19 who survived hospitalization – a pilot study. Phys Ther. (2021) 101:pzab099. doi: 10.1093/ptj/pzab099
19. Alba GA, Ziehr DR, Rouvina JN, Hariri LP, Knipe RS, Medoff BD, et al. Exercise performance in patients with post-acute sequelae of SARS-CoV-2 infection compared to patients with unexplained dyspnea. Eclinicalmedicine. (2021) 39:101066. doi: 10.1016/j.eclinm.2021.101066
20. Szekely Y, Lichter Y, Sadon S, Lupu L, Taieb P, Banai A, et al. Cardiorespiratory abnormalities in patients recovering from coronavirus disease 2019. J Am Soc Echocardiog. (2021) 34:1273–1284.e9. doi: 10.1016/j.echo.2021.08.022
21. Baptista BR, d'Humières T, Schlemmer F, Bendib I, Justeau G, Al-Assaad L, et al. Identification of factors impairing exercise capacity after severe COVID-19 pulmonary infection: a 3-month follow-up of prospective COVulnerability cohort. Respir Res. (2022) 23:68. doi: 10.1186/s12931-022-01977-z
22. Clavario P, Marzo VD, Lotti R, Barbara C, Porcile A, Russo C, et al. Cardiopulmonary exercise testing in COVID-19 patients at 3 months follow-up. Int J Cardiol. (2021) 340:113–8. doi: 10.1016/j.ijcard.2021.07.033
23. Rinaldo RF, Mondoni M, Parazzini EM, Pitari F, Brambilla E, Luraschi S, et al. Deconditioning as main mechanism of impaired exercise response in COVID-19 survivors. Eur Respir J. (2021) 58:2100870. doi: 10.1183/13993003.00870-2021
24. Jahn K, Sava M, Sommer G, Schumann DM, Bassetti S, Siegemund M, et al. Exercise capacity impairment after COVID-19 pneumonia is mainly caused by deconditioning. Eur Respir J. (2022) 59:2101136. doi: 10.1183/13993003.01136-2021
25. Rinaldo RF, Mondoni M, Parazzini EM, Baccelli A, Pitari F, Brambilla E, et al. Severity does not impact on exercise capacity in COVID-19 survivors. Resp Med. (2021) 187:106577. doi: 10.1016/j.rmed.2021.106577
26. Motiejunaite J, Balagny P, Arnoult F, Mangin L, Bancal C, Vidal-Petiot E, et al. Hyperventilation as one of the mechanisms of persistent dyspnoea in SARS-CoV-2 survivors. Eur Respir J. (2021) 58:2101578. doi: 10.1183/13993003.01578-2021
27. Aparisi Á, Ybarra-Falcón C, García-Gómez M, Tobar J, Iglesias-Echeverría C, Jaurrieta-Largo S, et al. Exercise ventilatory inefficiency in post-COVID-19 syndrome: insights from a prospective evaluation. J Clin Med. (2021) 10:2591. doi: 10.3390/jcm10122591
28. Skjørten I, Ankerstjerne OAW, Trebinjac D, Brønstad E, Rasch-Halvorsen Ø, Einvik G, et al. Cardiopulmonary exercise capacity and limitations 3 months after COVID-19 hospitalisation. European Respir J. (2021) 58:2100996. doi: 10.1183/13993003.00996-2021
29. Cassar MP, Tunnicliffe EM, Petousi N, Lewandowski AJ, Xie C, Mahmod M, et al. Symptom persistence despite improvement in cardiopulmonary health – insights from longitudinal CMR, CPET and lung function testing post-COVID-19. E Clin Med. (2021) 41:101159. doi: 10.1016/j.eclinm.2021.101159
30. Dorelli G, Braggio M, Gabbiani D, Busti F, Caminati M, Senna G, et al. Importance of cardiopulmonary exercise testing amongst subjects recovering from COVID-19. Diagnostics. (2021) 11:507. doi: 10.3390/diagnostics11030507
31. Vannini L, Quijada-Fumero A, Martín MPR, Pina NC, Afonso JSH. Cardiopulmonary exercise test with stress echocardiography in COVID-19 survivors at 6 months follow-up. Eur J Intern Med. (2021) 94:101–4. doi: 10.1016/j.ejim.2021.10.004
32. Ladlow P, O'Sullivan O, Houston A, Barker-Davies R, May S, Mills D, et al. Dysautonomia following COVID-19 is not associated with subjective limitations or symptoms but is associated with objective functional limitations. Heart Rhythm. (2022) 19:613–20. doi: 10.1016/j.hrthm.2021.12.005
33. Vonbank K, Lehmann A, Bernitzky D, Gysan MR, Simon S, Schrott A, et al. Predictors of prolonged cardiopulmonary exercise impairment after COVID-19 infection: a prospective observational study. Front Med. (2021) 8:773788. doi: 10.3389/fmed.2021.773788
34. Mancini DM, Brunjes DL, Lala A, Trivieri MG, Contreras JP, Natelson BH. Use of cardiopulmonary stress testing for patients with unexplained dyspnea post–coronavirus disease. Jacc Hear Fail. (2021) 9:927–37. doi: 10.1016/j.jchf.2021.10.002
35. Singh I, Joseph P, Heerdt PM, Cullinan M, Lutchmansingh DD, Gulati M, et al. Persistent exertional intolerance after COVID-19 insights from invasive cardiopulmonary exercise testing. Chest. (2022) 161:54–63. doi: 10.1016/j.chest.2021.08.010
36. Phillips DB, Collins SÉ, Stickland MK. Measurement and interpretation of exercise ventilatory efficiency. Front Physiol. (2020) 11:659. doi: 10.3389/fphys.2020.00659
37. Weatherald J, Farina S, Bruno N, Laveneziana P. Cardiopulmonary exercise testing in pulmonary hypertension. Ann Am Thorac Soc. (2017) 14:S84–92. doi: 10.1513/AnnalsATS.201610-788FR
38. Li Y, Li H, Zhu S, Xie Y, Wang B, He L, et al. Prognostic value of right ventricular longitudinal strain in patients with COVID-19. Jacc Cardiovasc Imag. (2020) 13:2287–99. doi: 10.1016/j.jcmg.2020.04.014
39. Brat K, Stastna N, Merta Z, Olson LJ, Johnson BD, Cundrle I. Cardiopulmonary exercise testing for identification of patients with hyperventilation syndrome. PLoS ONE. (2019) 14:e0215997. doi: 10.1371/journal.pone.0215997
40. Coupé M, Fortrat JO, Larina I, Gauquelin-Koch G, Gharib C, Custaud MA. Cardiovascular deconditioning: from autonomic nervous system to microvascular dysfunctions. Resp Physiol Neurobi. (2009) 169:S10–2. doi: 10.1016/j.resp.2009.04.009
41. Scherbakov N, Szklarski M, Hartwig J, Sotzny F, Lorenz S, Meyer A, et al. Peripheral endothelial dysfunction in myalgic encephalomyelitis/chronic fatigue syndrome. Esc Hear Fail. (2020) 7:1064–71. doi: 10.1002/ehf2.12633
42. Haffke M, Freitag H, Rudolf G, Seifert M, Doehner W, Scherbakov N, et al. Endothelial dysfunction and altered endothelial biomarkers in patients with post-COVID-19 syndrome and chronic fatigue syndrome (ME/CFS). J Transl Med. (2022) 20:138. doi: 10.1186/s12967-022-03346-2
43. Crisafulli E, Vigna M, Ielpo A, Tzani P, Mangia A, Teopompi E, et al. Heart rate recovery is associated with ventilatory constraints and excess ventilation during exercise in patients with chronic obstructive pulmonary disease. Eur J Prev Cardiol. (2018) 25:1667–74. doi: 10.1177/2047487318789756
44. Amiya E, Watanabe M, Komuro I. The relationship between vascular function and the autonomic nervous system. Ann Vasc Dis. (2014) 7:109–19. doi: 10.3400/avd.ra.14-00048
45. Gruenewaldt A, Nylander E, Hedman K. Classification and occurrence of an abnormal breathing pattern during cardiopulmonary exercise testing in subjects with persistent symptoms following COVID-19 disease. Physiol Rep. (2022) 10:e15197. doi: 10.14814/phy2.15197
46. Asarcikli LD, Hayiroglu MI, Osken A, Keskin K, Kolak Z, Aksu T. Heart rate variability and cardiac autonomic functions in post-COVID period. J Interv Card Electr. (2022) 63:715–21. doi: 10.1007/s10840-022-01138-8
47. Baranauskas MN, Carter SJ. Evidence for impaired chronotropic responses to and recovery from 6-minute walk test in women with post-acute COVID-19 syndrome. Exp Physiol. (2021). doi: 10.1113/EP089965
48. Frésard I, Genecand L, Altarelli M, Gex G, Vremaroiu P, Vremaroiu-Coman A, et al. Dysfunctional breathing diagnosed by cardiopulmonary exercise testing in ‘long COVID' patients with persistent dyspnoea. Bmj Open Respir Res. (2022) 9:e001126. doi: 10.1136/bmjresp-2021-001126
49. Jamal SM, Landers DB, Hollenberg SM, Turi ZG, Glotzer TV, Tancredi J, et al. Prospective evaluation of autonomic dysfunction in post-acute sequela of COVID-19. J Am Coll Cardiol. (2022) 79:2325–30. doi: 10.1016/j.jacc.2022.03.357
50. Natelson BH, Brunjes DL, Mancini D. Chronic fatigue syndrome and cardiovascular disease JACC state-of-the-art review. J Am Coll Cardiol. (2021) 78:1056–67. doi: 10.1016/j.jacc.2021.06.045
51. Romero-Ortuno R, Jennings G, Xue F, Duggan E, Gormley J, Monaghan A. Predictors of submaximal exercise test attainment in adults reporting long COVID symptoms. J Clin Med. (2022) 11:2376. doi: 10.3390/jcm11092376
52. Guazzi M, Bandera F, Ozemek C, Systrom D, Arena R. Cardiopulmonary exercise testing what is its value? J Am Coll Cardiol. (2017) 70:1618–36. doi: 10.1016/j.jacc.2017.08.012
53. Shen Y, Zhang X, Ma W, Song H, Gong Z, Wang Q, et al. VE/VCO2 slope and its prognostic value in patients with chronic heart failure. Exp Ther Med. (2015) 9:1407–12. doi: 10.3892/etm.2015.2267
54. Ambrosino P, Parrella P, Formisano R, Perrotta G, D'Anna SE, Mosella M, et al. Cardiopulmonary exercise performance and endothelial function in convalescent COVID-19 patients. J Clin Med. (2022) 11:1452. doi: 10.3390/jcm11051452
55. Zweerink A, Lingen A-LCJ van der, Handoko ML, Rossum AC van, Allaart CP. Chronotropic incompetence in chronic heart failure. Circulat Hear Fail. (2018) 11:e004969. doi: 10.1161/CIRCHEARTFAILURE.118.004969
Keywords: post-acute sequelae COVID-19, cardiopulmonary exercise testing, autonomic dysfunction, exercise intolerance, hyperventilation
Citation: Aparisi Á, Ladrón R, Ybarra-Falcón C, Tobar J and San Román JA (2022) Exercise Intolerance in Post-Acute Sequelae of COVID-19 and the Value of Cardiopulmonary Exercise Testing- a Mini-Review. Front. Med. 9:924819. doi: 10.3389/fmed.2022.924819
Received: 20 April 2022; Accepted: 21 June 2022;
Published: 22 July 2022.
Edited by:
David Andaluz Ojeda, HM University Sanchinarro Hospital, SpainReviewed by:
Mahdieh Molanouri Shamsi, Tarbiat Modares University, IranJavier Mora, University of Costa Rica, Costa Rica
Copyright © 2022 Aparisi, Ladrón, Ybarra-Falcón, Tobar and San Román. This is an open-access article distributed under the terms of the Creative Commons Attribution License (CC BY). The use, distribution or reproduction in other forums is permitted, provided the original author(s) and the copyright owner(s) are credited and that the original publication in this journal is cited, in accordance with accepted academic practice. No use, distribution or reproduction is permitted which does not comply with these terms.
*Correspondence: Javier Tobar, javitobar10@gmail.com