- 1Department of Rheumatology, Ajou University School of Medicine, Suwon, South Korea
- 2Department of Molecular Science and Technology, Ajou University, Suwon, South Korea
Systemic lupus erythematosus (SLE) affects women more frequently than men, similar to the female predilection for other autoimmune diseases. Moreover, male patients with SLE exhibit different clinical features than female patients. Sex-associated differences in SLE required special considerations for disease management such as during pregnancy or hormone replacement therapy (HRT). Sex hormones, namely, estrogen and testosterone, are known to affect immune responses and autoimmunity. While estrogen and progesterone promote type I immune response, and testosterone enhances T-helper 1 response. Sex hormones also influence Toll-like receptor pathways, and estrogen receptor signaling is involved in the activation and tolerance of immune cells. Further, the clinical features of SLE vary according to hormonal changes in female patients. Alterations in sex hormones during pregnancy can alter the disease activity of SLE, which is associated with pregnancy outcomes. Additionally, HRT may change SLE status. Sex hormones affect the pathogenesis, clinical features, and management of SLE; thus, understanding the occurrence and exacerbation of disease caused by sex hormones is necessary to improve its management.
Introduction
Systemic lupus erythematosus (SLE), a chronic autoimmune inflammatory disease with heterogeneous clinical manifestations and course, affects multiple tissue and organ systems with, varying severity depending on the patient and duration of illness (1). Although many aspects of its etiology remain unclear, SLE is a complex disease known to result from the aberrant activity of the immune system due to environmental, genetic, epigenetic, and hormonal factors (2). Like other autoimmune diseases, such as rheumatoid arthritis, Sjogren’s syndrome, and systemic sclerosis, SLE has a much higher prevalence in women than in men, with a female-to-male ratio ranging from 8:1 to 15:1 (3, 4). The striking difference in prevalence appears during the peak reproductive age, whereas female predominance significantly decreases during pre-puberty and post-menopause (5).
Factors associated with sex differences in SLE include sex chromosome genes, sex-dependent environmental factors, and gut microbiome composition, but considerable evidence supports that sex hormones are a major factor (6). As previously mentioned, SLE is typically more prevalent in young women of childbearing age. Indeed, its prevalence in women is only double that in men during childhood and postmenopausal periods (7). Furthermore, the disease activity of SLE can vary depending on hormonal changes such as the menstrual cycle and menopause, with a flare rate of 45–70% in pregnancy (8). In some patients with SLE, symptoms worsen each month as menstruation begins, and estrogen-containing therapies, namely, oral contraceptives (O) and postmenopausal hormone replacement therapy (HRT), are associated with an increased risk of flare (9, 10). In addition, the frequency and severity of flares decrease in most menopausal patients with SLE (11). In a lupus-prone model in NZB × NZW F1 mice, disease development was prevalent and survival time was shorter in women than in men (12).
Sex hormones include estrogen, progesterone, testosterone, dihydrotestosterone, and dehydroepiandrosterone (13). Estrogen, progesterone, and androgens are produced by the ovary in women, while testosterone precursors are produced mostly by Leydig cells in the testis in men and in the adrenal gland and thecal cells of the ovary in women (14). In women, estrogen and progesterone levels fluctuated during the menstrual cycle and life span, namely, premenopause and menopause, whereas testosterone levels remain steady during the menstrual cycle and decrease after menopause. Serum testosterone levels are higher in men than in women and decrease with age. Estrogen mainly affects reproductive function and additional processes, such as bone mass and fat distribution, while testosterone regulates physiological processes in muscle mass and strength, bone mass, fat distribution, and production of sperm and red blood cells.
In addition, sex hormones are involved in the development and function of innate and adaptive immune responses, and dysregulation of these mechanisms contributes to autoimmune abnormalities (15). Progesterone and androgens mainly have immunosuppressive and anti-inflammatory effects, thus protecting against autoimmune diseases, whereas estrogen is generally regarded as pathogenic due to its immune-stimulatory effects (16). Considering the mechanisms underlying altered immune responses and female predilection, estrogen is widely perceived as contributing to the predisposition for SLE. Recently, various clinical studies have reported sex-dependent genetic and epigenetic changes in SLE, revealing the complex role of sex hormones in addition to estrogen in the pathogenesis of SLE (17–19).
Herein, we review the current evidence regarding the role of sex hormones in the pathogenesis of SLE and describe the clinical features according to sex hormonal changes.
Methods
A systemic search of all English-language studies was performed in the Medline/PubMed, Scopus, and EMBASE databases using the following keywords: “sex hormone,” “sex steroid,” “systemic lupus erythematosus,” “autoimmune disease,” “estrogen,” “progesterone,” “testosterone,” and “sex differences,” as well as their abbreviations. Additionally, all related studies were searched manually for relevant keywords such as “oral contraceptives” and “hormone replacement therapy.” Abstracts from relevant studies were reviewed, and appropriate articles were retrieved, and non-relevant papers and duplicate studies were excluded. All authors of this study conducted searches and articles were reviewed independently.
Results and discussion
Influence of sex hormones on immune response or autoimmunity (Figure 1)
Estrogen
Immune cells express two estrogen receptor (ER) subtypes, ERα and ERβ, and activation of ER-mediated or ER-independent pathways controls immune responses. ER subtypes and their mechanisms of action vary depending on the cell or environment, while hormone’ concentration, density, distribution, and receptor, subtype affect immune responses. Gene expression analysis demonstrated that the expression of ERα mRNA was increased, while that of ERβ was decreased in peripheral blood mononuclear cells (PBMCs) from patients with SLE compared to healthy controls (20). However, no unique variants in ERα, ERα splice variants, and ERβ were identified in PBMCs from 19 patients with SLE compared to 12 healthy individuals (21). Furthermore, the depletion of ERα attenuated the development of glomerulonephritis and anti-double-stranded DNA (dsDNA) antibodies, while prolonging the survival of NZB × NZW F1 mice, whereas ERβ deficiency had no effect on lupus manifestations (22, 23). Currently, the association between ER expression/action and SLE remains unclear.
B cells and autoantibody production
Estrogen stimulates B cell maturation and antibody secretion in the normal immune system. With respect to its impact on the autoimmune response, estrogen has been shown to increase the abundance of bone marrow progenitor B cells and enhance the survival of splenic B cells, which promotes the development of autoreactive B cells (24). In one study, estradiol (E2) treatment increased the production of anti-dsDNA antibodies and IgG in PBMCs from patients with active SLE, but not in patients with inactive disease or in a normal population (25). Additionally, E2 administration induced a lupus phenotype in BALB/c mice that expressed a transgene-encoded H chain of an anti-DNA antibody, rescued high-affinity DNA-reactive B cells, and led to increased Bcl-2 expression, which improved the survival of autoreactive B cells (26, 27). Meanwhile, tamoxifen treatment reduced levels of autoantibodies to IgG3, prolonged survival time, and was associated with weaker glomerular immune complex deposition in NZB × NZW F1 mice (28). Further, treatment with E2 increased levels of B cell-activating factor (BAFF) in immune cells, namely, macrophages, which promoted the survival of autoreactive B cells and autoantibody production, while ERα-knockout (KO) splenic cells showed decreased BAFF expression (29). In addition, NZB × NZW F1 mice treated with E2 exhibited more severe proteinuria and histological change in the glomerular tissue, along with increased levels of anti-C1q and anti-dsDNA antibodies (30).
T cells
Low estrogen levels enhance T-helper type 1 (TH1) cells and cell-mediated immunity, whereas high estrogen levels promote T-helper type 2 (TH2) cells and humoral immunity. E2 treatment was shown to enhance the expression of calcineurin, a T cell activation marker that acts through ERs, in the T cells of female patients with SLE, but not in those of HCs or male patients with SLE. Consistently, treatment with ERα and ERβ agonists increased the expression of calcineurin and CD154 in the T cells of patients with SLE (31, 32). E2 treatment stimulated T cells to express CD40 ligands in patients with SLE but not in normal women (33). E2 treatment induced the lupus phenotype, namely, nephritis, and production of TH2 cytokines and autoantibodies in wild-type mice, but not or minimally in ERα-KO mice (34). CD4-ERα KO mice had increased autoantibody production and CD4 + CD44 + CXCR5 + Bcl-6 + follicular helper T (TFH) cells, and E2 treatment decreased TFH cell responses, antigen-specific antibody production, and reduced IL-21 and Bcl-6 expression (35).
While different results had been reported in the populations of regulatory T (Treg) cells in SLE, incubation with E2 resulted in increased CD4, CD25, and FoxP3 mRNA expression in PBMCs from a healthy female, those from patients with SLE exhibited reduced FoxP3 mRNA expression (36). The deficiency of estrogen-related receptor γ (Esrrg), a murine lupus susceptibility gene associated with CD4 + T cell activation, has been shown to result in impaired function of Treg cells (37, 38). In addition, levels of human ESRRG, which is highly expressed in Treg cells, were reportedly lower in CD4 + T cells of patients with SLE than in those of HCs.
While increased population of CD4 + Th17 cells and IL-17A production has a pathogenic role in SLE, E2 treatment showed an inhibitory effect in Th17 cell differentiation in CNS autoimmunity (39). In addition, ERα KO mice showed TH1 and Th17 cell differentiation with IL-17 production, and estradiol inhibited Th17 cell differentiation through the downregulation of RORγt transcription (40). IL-17A production and IL-23R expression were increased in Th17 cells from female mice compared to those from male mice, both of which were decreased and Th17 cell proliferation was downregulated when ERα expression was suppressed (41).
Dendritic cells and toll-like receptor pathways
Dendritic cells (DCs), especially plasmacytoid DCs (pDCs), are activated in SLE to produce type I IFN through the Toll-like receptor (TLR)-7 or TLR-9 pathway through which endogenous DNA and RNA provoke autoimmune responses as self-antigen. Several DC subsets, namely, pDCs, express different patterns of ERs and affect ERα signaling (42). E2 treatment promoted the differentiation of DCs expressing high levels of cell surface MHC class II and CD86, whereas ER antagonists blocked DC differentiation (43). ERα deficient lupus-prone mice (strain NZM2410) had decreased frequency of pDCs and reduced endogenous expression of MHC-II and PDC-TREM, which modulates type I IFN production (44).
TLR-7-mediated IFN-α production was reportedly increased in the peripheral blood lymphocytes of healthy females compared to those of healthy males (45). E2 therapy enhanced TLR-7- and TLR-9-dependent production of IFN-α stimulated by nucleic acid-containing complexes in pDCs from postmenopausal women, while TLR-medicated IFN-α production by pDCs was restored in ERα-KO mice by E2 treatment (46). In another study, TLR-9 induced IL-6 and MCP-1 production by DCs was decreased in ERα-KO lupus-prone mice (strain NZM2410), and IL-1β and IL-23 expression were induced by a TLR-9 agonist in wild-type but not ERα-KO mice (47). Moreover, the delivery of recombinant IFN regulatory factor 5 (IRF5) protein into human pDCs increased TLR-7-mediated IFN-α secretion, while the genetic ablation of the estrogen receptor 1 gene in pDCs reduced irf5 mRNA expression as well as IFN-α production (48). Further, estrogen treatment enhanced the expressions of TLR-8 and endosomal TLR-7 and TLR-9 in the PBMCs of patients with SLE compared to that in the PBMCs of HCs (49). Additionally, E2 exposure exacerbated proteinuria and glomerular immune complex deposition in female lupus-prone MRLlpr mice through the induction of TLR-7 and -9 expression on splenic leukocytes and CD19 cells (50). Estrogen treatment was shown to enhance the expression of STAT1, which induces IFN-stimulated gene expression and upregulates TLR-8 expression (51). These data suggest that estrogen influences DC activation and IFN production through the TLR signaling pathways.
Epigenetic modulation
There were some reports finding the epigenetic changes related to the effects of estrogen or ER expression in SLE. DNA demethylation within the proximal promotor region relative to the transcription star site of the human ERα gene was associated with the overexpression of the ERα gene in SLE (52). And E2 inhibited DNA methyltransferase 1 (DNMT1) and enhances global DNA hypomethylation in SLE CD4 + T cells (53). ER agonists rescued downregulated DNMT1 and DNA hypomethylation.
E2 treatment enhances the activation of IFN-α signaling in SLE B cells via inhibitor of kappa B kinase ε (IKKε) by downregulating the expressions of let-7e-5p, miR-98-5p, and miR-145a-5p (54).
Estrogen treatment induced the overexpression of has-miR-10b-5p in T cells, and has-miR-10b-5p suppresses serine/arginine-rich splicing factor 1, which controls genes involved in T cell signaling and cytokine production. Has-miR-10b-5p expression was elevated in T cells from healthy women than healthy men, and elevated in T cells from patients with SLE, regardless of sex and SLE disease activity index (SLEDAI) (55).
Progesterone
Progesterone is a female reproductive steroid with immunomodulatory functions. Prolonged exposure to medroxyprogesterone acetate, synthetic progesterone used for contraception, led to lower serum IgG level, and decreased mortality in female NZB × NZW mice, although such treatment did not affect lupus phenotypes in other studies (56–58). The action of progesterone depends on its receptors, namely, progesterone receptor (PR), glucocorticoid receptor, and membrane PR. While low progesterone levels activate PRs and membrane PRs, high levels can bind not only to PRs and membrane PRs but also to glucocorticoid receptor, which is critical for reproduction. In one study, aged female PR-KO lupus-prone Nba2 mice exhibited increased IgG autoantibody production, and glomerular IgG deposition, inflammation, and damage compared to male mice (59). In addition, knockout of PR resulted in a lower splenic Treg cell population, but an increased proportion of follicular Th cells in aged female Nba2 mice.
Testosterone
Testosterone inhibits B cell differentiation in the bone marrow. Knockout of male androgen receptor, a testosterone receptor, resulted in increased levels of bone marrow B cell precursors in mice (60). In addition, male mice lacking androgen receptors had higher splenic B cell population and serum BAFF levels (61). Further, levels of plasma androgens, namely, testosterone and androstenedione, were lower in female patients with active SLE (62).
While some studies have reported the therapeutic effect of testosterone and other androgens against SLE disease activity, testosterone patches and 19-nortestosterone failed to improve disease activity or quality of life in patients with SLE (63–66). In vitro study assessing the effect of sex hormones on changes in Treg cells demonstrated that androgen/testosterone enhanced FoxP3 mRNA expression in CD4 + Treg cells of patients with SLE (36).
Influence of menstrual cycle on systemic lupus erythematosus
During the menstrual cycle, follicle-stimulating hormone (FSH) stimulates the ovarian follicles to produce E2. Thus, the plasma E2 concentration is increased, while that of progesterone is decreased in the follicular phase, and both E2 and progesterone plasma concentrations are high in the luteal phase (36). Increased E2 levels lead to a mid-cycle surge of luteinizing hormone, which initiates ovulation; if fertilization does not occur, progesterone levels drop. Luteolysis is inhibited during pregnancy, leading to prolonged high E2 and progesterone levels. In addition, E2 and progesterone levels are low during menopause along with the depletion of follicles.
A study comparing reproductive health histories between patients with SLE, and the general population reported no differences in hormone levels throughout the menstrual cycle (67). However, menopause occurred earlier in patients with SLE, and the use of HRT was frequent, and the family size was reduced in patients with lupus nephritis. Some data have shown that premature ovarian dysfunction is more common in patients with SLE than in HCs and is associated with the use of cyclophosphamide (68, 69). Compared to 30 HCs, abnormal and longer-length menstrual cycles were more frequent, and the median FSH level was higher, and that of progesterone was lower in 30 patients with juvenile SLE (70). A study analyzing a self-reported survey of patients with SLE revealed higher pain, fatigue, and disease activity during menses than during the hormonal surge phase, although recall bias and confusion with pre-menstrual syndrome existed (71). In examining the influence of the menstrual cycle or fertility on SLE, disease activity, use of medication (including glucocorticoids and cyclophosphamide), and individual differences should be considered.
Analysis of PBMCs has revealed significant changes in gene expressions, including that of TNF superfamily member 14 and signal regulatory protein-γ, during the menstrual cycle of patients with SLE compared to that of HCs (72). A study analyzing the expression of sex hormone receptor genes in PBMCs, and cell subsets reported that several immune response genes were more highly expressed during the ovulatory and mid-luteal phase (73). In addition, the level of sex hormone-binding globulin, a steroid hormone transport protein, was correlated with ERβ1 gene expression.
Characteristics of male systemic lupus erythematosus
Sex hormones and chromosomes in male systemic lupus erythematosus
Unsurprisingly, the role that sex hormones play in male and female SLE differs significantly, which has been demonstrated both in murine models and clinical studies (74). In the NZB × NZW F1 mouse model, an autoimmune disease resembling human SLE is characterized by high levels of antinuclear and anti-dsDNA antibodies, lymphadenopathy, splenomegaly, and immune complex-mediated glomerulonephritis. NZB × NZW F1 mice administered androgens exhibited reduced lupus-like phenotypes and improved survival rates, whereas autoantibodies and accelerated autoimmune disease appeared earlier in castrated NZB × NZW F1 mice administered estrogen (75, 76). A recent study with a lupus-prone mouse model reported that a male-driven immunoinhibitory milieu was related to B cell activation and differentiation, and ultimately delayed or prevented lupus-like disease, suggesting that androgens affect lupus pathogenesis and exert different therapeutic effects in males and females (77). Given the clear inhibitory effects of androgen on the immune system, a recent interest is to discover SLE therapies targeting immunomodulatory cells by elucidating mechanisms that affect the number and functionality of these cells such as regulator T/B cells, MDSCs, and M2 macrophages in genetically predisposed male mice (78).
In human studies, significantly lower androgen levels, which were inversely related to hypoandrogenism, testosterone levels, and disease activity, were detected in male patients with SLE compared to HCs (79–81). As in murine models, the clinical symptoms and serological features of men and women respond differently to synthetic androgen therapy as a treatment for SLE. Two studies have reported that testosterone supplementation improved the clinical symptoms of male lupus patients with Klinefelter’s syndrome (82, 83). Additionally, testosterone injections for cross-gender therapy resolved the skin symptoms of a transgender patient (female to male) with subcutaneous lupus erythematosus (84). In contrast, a small clinical trial reported that men treated with 19-nortestosterone exhibited decreased testosterone levels, increased serum anti-dsDNA antibody levels, and new clinical features, namely, Raynaud’s phenomenon and pleuropericardial disease (64). In another clinical trial, testosterone patches did not significantly affect disease activity, quality of life, or sexual function in male patients with SLE (63). The clinical efficacy of androgen treatment in male patients with SLE remains unclear, and further studies are warranted to determine whether such treatments should be more widely provided.
In addition to sex hormones, there is growing evidence that the role of the X chromosome helps explain whether more women than men develop autoimmune diseases, namely, SLE (85–87). The first evidence supporting that the factors associated with X chromosomes cause susceptibility for SLE was a report that the prevalence of Klinefelter’s syndrome (karyotype 47, XXY) is increased 14-fold in men with SLE compared to that in an unselected male population (85). With similar results, in one study, karyotype 47, XXX in females predicted an approximately 25-fold relative risk for SLE, and another report showed that 46, XX males (de la Chapelle’s syndrome) were excessively present among males with SLE (86, 87). These data support that the number of X chromosomes, not phenotypes, is related to the cause of sex bias in SLE. Recently, genes in the X chromosome are also observed to be attributed to the female bias in SLE. The X chromosome encodes a greater number of genes than the Y chromosome, and X-linked genes such as KDM6a, TLR-7, CXorf21, and IRAK1 are found to be overexpressed in females’ autoimmunity. Furthermore, recent data suggest that X-linked genetic factors are involved in epigenetic mechanisms to avoid X chromosome inactivation, thereby enhancing female susceptibility to autoimmune diseases (88).
Clinical features and outcomes in male systemic lupus erythematosus
Due to the perception that SLE is a disease in women of childbearing age, appropriate diagnosis and treatment are often delayed in men. Overcoming this challenge requires an awareness of the distinctive presentation of male SLE. Studies worldwide have confirmed that the peak ages of SLE incidence and prevalence are delayed for men compared to women (89). Although considerable variability is reported according to region and race, the mean age at diagnosis is 26−55 years for men, compared to 26.3−42.6 years for women (90). The peak incidence of SLE usually occurs for women in their 20s–50s, while that for men occurs in their 50s–70s (89). The prevalence curve by age tends to be similar to the distribution of incidence data, with the peak age of prevalence ranging from 45 to 69 years for women and 40 to 89 years for men (89, 91).
Serologically, anti-dsDNA and anti-Smith antibodies have been observed to occur more frequently in male SLE, whereas some studies have reported lower levels of anti-Ro/SSA and anti-La/SSB antibodies in male SLE (92–95). Although differences occur depending on the reported group, anti-U1-RNP and anti-cardiolipin antibodies and low complement appear to occur at almost the same rates in men and women (92, 96). Lupus anticoagulant positivity is more common in men, combined with smoking and alcohol use, which is related to a higher prevalence of thrombosis in male SLE than in female SLE (96–98). Additionally, renal, hematological, and neurological involvement, as well as serositis features prominently in male SLE, leading to rapid organ damage compared to female SLE (94, 99). The increase in autoantibody production and the development of lupus nephritis in male SLE is presumed to be due to the global deletion of ERα or especially in B cells (98).
Regarding skin involvement, discoid, and subacute lesions occurred more frequently in male SLE, while malar rash, photosensitivity, and Raynaud’s phenomenon were much less common (74, 90, 92, 94–104). Musculoskeletal involvement and alopecia were reported less frequently in male SLE, with fewer outpatient visits and emergency department visits than in female SLE (93, 101, 105).
In relation to disease activity, SLEDAI scores and lupus severity of disease index scores did not differ significantly between the sexes in most studies (90, 94, 95, 98, 104). Conversely, renal damage is a major concern in male SLE, as several studies reported that male sex is a strong predictor of baseline damage and men have a high risk of developing chronic renal failure (91, 92, 105–109). In cohorts in the United States and Taiwan, male sex was associated with a 2-fold greater risk of end-stage renal disease (99, 110). However, in a recent study using the national data system in the United States, similar rates for both sexes were reported for end-stage renal disease and mortality (105). Increased incidence of cardiovascular events due to ischemic heart disease or stroke was also reported among male patients with SLE (90, 98, 100). In this regard, male SLE is considered to have a poorer prognosis than female SLE due to renal involvement and concomitant cardiovascular diseases. Although survival rates vary, most studies did not report statistically significant differences between men and women (90, 92, 95, 105).
The clinical characteristics and outcomes of SLE display notable sex differences, which are most influenced by sex hormones. However, considering age, race, national health system, and small cohorts of male patients with SLE, further studies are needed to unravel the mystery of male SLE and potential therapeutic interventions for the disease.
Influence of hormone therapy on systemic lupus erythematosus
Oral contraceptives and systemic lupus erythematosus
17α-ethinyl estradiol, a synthetic analog of 17β-estradiol (E2), is a major component in OCPs and has been commonly used in hormone therapy. As the timing of pregnancy greatly contributes to success in SLE and flares cause adverse pregnancy outcomes, contraception is often considered for women with SLE, leading to the use of OCPs (111). Two major categories of OCPs, combined oral contraceptives (COCs) that contain estrogen and progesterone and progestin-only formulations, are commonly used as reversible contraceptives and are mostly safe (112). However, reflecting on the unpredictable and variable nature of SLE, OCPs have been considered unsafe and not often prescribed for women with SLE (113, 114). Several studies have reported controversial results regarding the use of OCPs in patients with SLE (Table 1). The role of exogenous estrogen as a flare factor was first reported in a case report in the 1960s (115). Early case reports and retrospective studies supported that the patients with established disease exposed to OCPs were at risk for developing SLE (105–120). A frequently cited and representative retrospective study reported by Jungers et al. found that flares occurred in 43% of patients with lupus nephritis when taking COCs, which was not observed with progestin-only formulations (117). In another retrospective study based on self-reported flares, 13% of patients experienced flares after initiating OCPs, particularly with musculoskeletal symptoms (120). Moreover, several cases of pulmonary hypertension and venous thromboembolism have been reported in patients with SLE after the use of OCPs (118, 121).
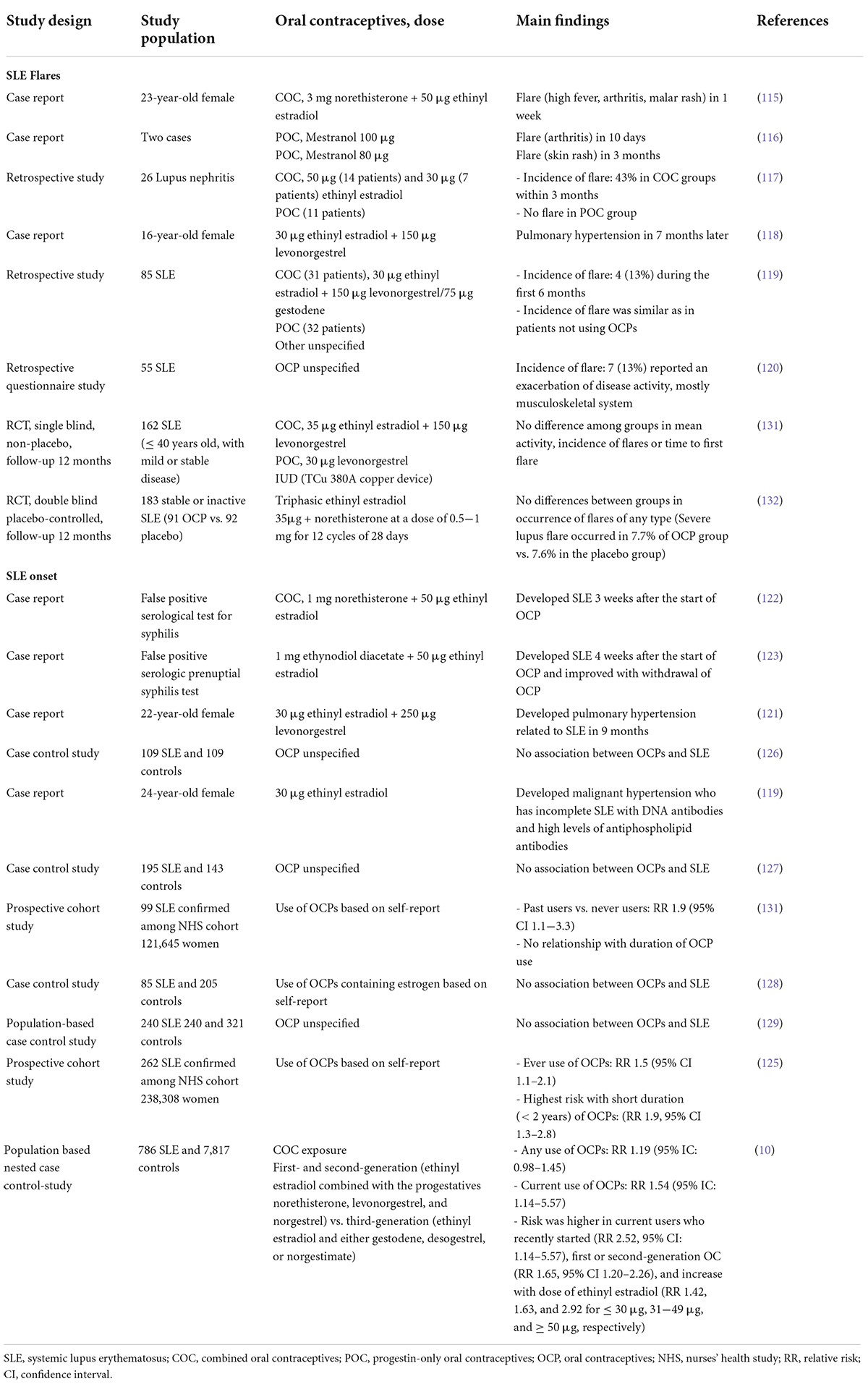
Table 1. List of studies on the risk of disease onset or flares in patients with systemic lupus erythematosus using oral contraceptives.
In addition to its association with disease activity, case reports and prospective cohort studies have reported newly diagnosed SLE after the initiation of OCPs (10, 119, 121–125). Sanchez-Guerrero et al. and Costenbader et al. reported associations between OCP use and SLE onset in 1997 and 2007, respectively, using the same population from the Nurses’ Health Study cohort (124, 125). The relative risk (RR) of OCP users compared to that of never users was 1.9 (95% confidence interval [CI] 1.1−3.3) in the first study and 1.4 (95% CI 0.9−2.1) in the second study. Another highly supportive study conducted in the United Kingdom evaluates the risk of SLE incidence related to COC use (10). In this study, COC use was associated with an increased risk of SLE (RR 1.19, 95% CI 0.98−1.45), especially in women who recently started using contraceptives or at higher doses. Malignant hypertension and vascular complication have also been reported in women with incomplete SLE featuring anti-DNA and antiphospholipid antibodies (119).
However, case-control studies have reported contradictory results (126–129). The first case-control study assessing the association between OCP exposure and risk of SLE was performed in 1985 with 109 cases of SLE and 109 HCs, reporting that recent use of OCPs was independent of SLE onset (odds ratio 0.5, 95% CI 0.11−2.3) (126). A case-control study from the United States with 195 cases of SLE and 143 HCs also showed no association between SLE and either any or recent use of OCPs (127). Although studies with similar results have been reported, limitations such as selection bias have hampered the precision of these studies (128, 129). Some studies have suggested that OCP use does not equally affect all individuals and triggers SLE by inducing antinuclear antibodies in predisposed individuals with autoimmune serologies (130).
Two higher-quality randomized controlled trials (RCT) were conducted to clarify this discrepancy between conflicting results (131, 132). The first RCT was a single-blinded, non-placebo study that followed 162 patients with stable SLE randomly assigned to a COC, intrauterine device, or progestin-only pill for 12 months. In this study, disease activity, flare incidence, and time-to-first flare did not differ significantly among the groups treated with different types of contraceptive therapy (131). The second double-blind RCT, the Safety of Estrogen in Lupus Erythematosus National Assessment (SELENA) study conducted in the United States, included 183 patients with inactive or stable active SLE randomly assigned to receive placebo or COC for 12 months (132). As with the previous RCT results, the flare rates were similar between the two groups, and the discontinuation rates did not differ for any reason.
Available evidence from RCTs supports the safety of OCPs, namely, COC, in most women with SLE. Based on these results, the World Health Organization published useful information regarding contraception for women with SLE, suggesting that most OCPs can be used by women with SLE if antiphospholipid antibodies are absent or cardiovascular risk factors are unclear (113). Since both RCTs excluded patients with SLE with unstable active diseases, the results may not be applicable to all women with SLE. Considering the data to date, the effects and risks of OCPs on SLE may vary depending on the dose, duration of use, and type of hormone used. Despite being theoretically possible, the use of OCPs should be fully discussed with respect to the balance of benefits and risks for each individual patient. Most importantly, OCPs are contraindicated for women with SLE displaying positive/unknown antiphospholipid antibodies or a history of thrombosis under all circumstances (133, 134).
Hormone replacement therapy and systemic lupus erythematosus
Hormone replacement therapy (HRT) is the most effective method for relieving menopausal symptoms such as vasomotor hot flashes, atrophic vaginitis, and urinary incontinence or frequency (135). In the 1980s and 1990s, early observational data supported that HRT reduced coronary heart disease and mortality, and initial data from the Women’s Health Initiative (WHI) RCT demonstrated a decreased incidence of osteoporosis-related fractures in postmenopausal women undergoing HRT (136, 137). Thus, HRT was widely used in menopausal women for 20 years. However, in the early 2000s, data from the WHI trial suggest that HRT was associated with an increased risk of invasive breast cancer, coronary heart disease, stroke, and pulmonary embolism, leading to an abrupt decline in the use of HRT in postmenopausal women worldwide (138, 139). Although the WHI was the largest RCT on HRT, only two hormone formulations were evaluated. Subsequent studies have changed the approach to HRT by evaluating different dosages and routes of estrogen therapy, namely, transdermal HRT or an ultra-low-dose oral product, and have recently demonstrated that the benefits outweigh the risks in women within 10 years of menopause and short-term use of low-dose HRT to alleviate menopause symptoms (140, 141).
Although HRT is generally considered to relieve menopausal symptoms in the short term and protect against chronic diseases in the long term for the general female population, many inconsistencies have been reported in patients with SLE. Table 2 lists the characteristics of studies investigating the relationship between HRT and SLE. A large prospective cohort study in 1995 reported that HRT was causally associated with an increased risk of developing SLE in postmenopausal women (142). In this study, the age-adjusted relative risks for the onset of SLE were 2.1 (95% CI 1.1−4.0) for ever users, 2.5 (CI 1.2−5.0) for current users, and 1.8 (CI, 0.8−4.1) for past users, compared with never users, and the duration of hormone use and risk of SLE were proportional. Additionally, a case-control study by Meier et al. reported that the risk of developing SLE increased as the duration of hormone use increased, and the magnitude of risk was related to estrogen dose (143). However, some studies have found no evidence of a significant increase in the incidence of SLE with HRT use (129).
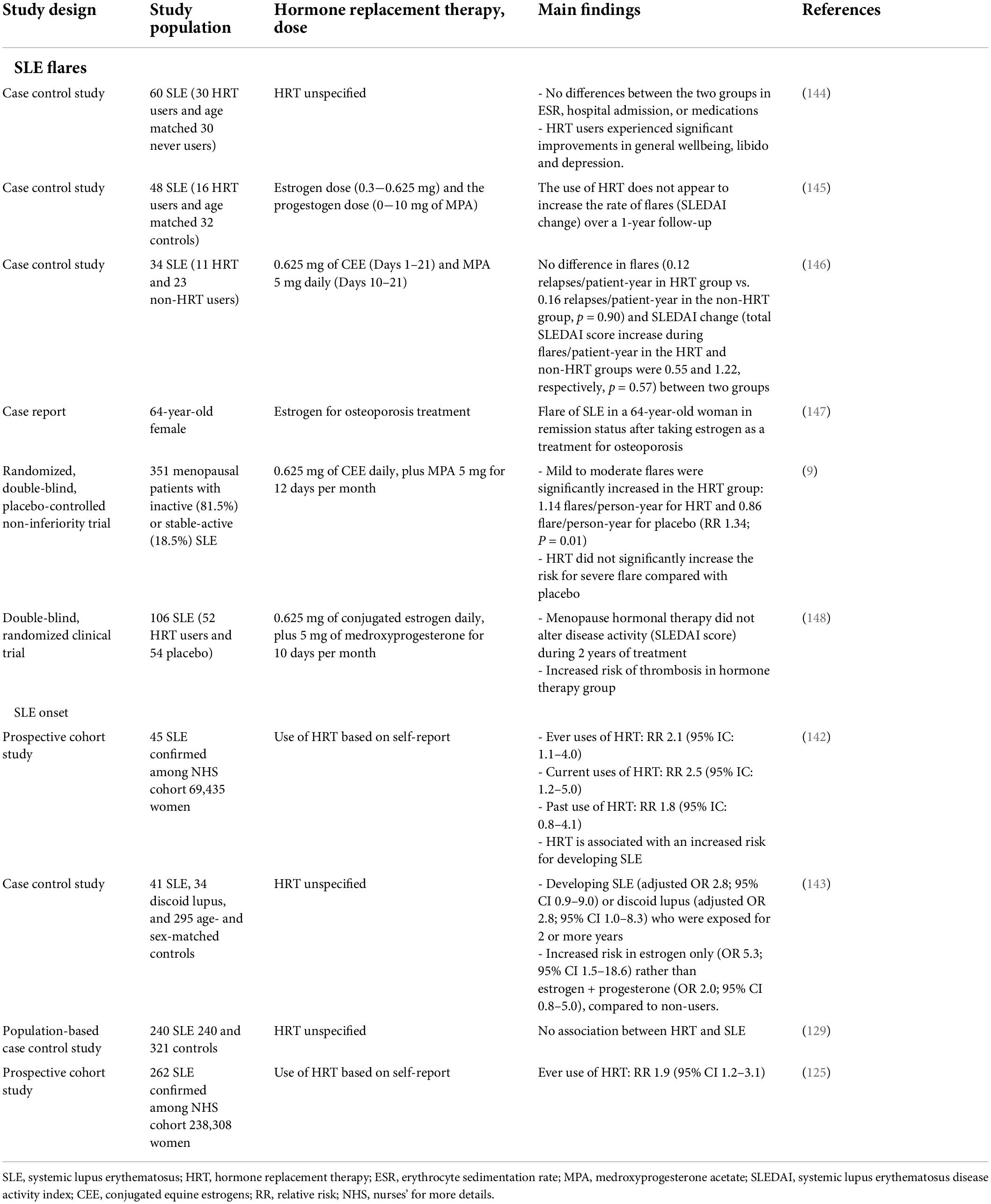
Table 2. List of studies on the risk of disease onset or flares in patients with systemic lupus erythematosus using hormone replacement therapy.
Several early retrospective case-control studies failed to find an association between flares and HRT (144–146). Although these were very small studies with insufficient data, the conclusion that HRT had a minimal effect on inflammatory markers and did not change disease activity (expressed by SLEDAI) was similar in all published observational studies. Conversely, case and prospective cohort studies have provided evidence linking HRT to flares (9, 147). In one case report, a woman diagnosed with SLE maintained remission status after menopause at 38 years of age but relapsed after taking estrogen as a treatment for osteoporosis at 64 years of age (147). The largest clinical trial to date investigating the effects of HRT on disease activity in patients with SLE was part of the SELENA trial, in which mild to moderate flares were significantly increased in the HRT group (9). However, neither the occurrence of severe flares nor the mean SLEDAI scores changed significantly between the HRT and placebo groups in this study. In another prospective study conducted by Sánchez-Guerrero et al. HRT use did not change the disease activity in SLE during 2 years of treatment (148).
As with taking OCPs, the greatest concern regarding HRT is the increased risk of arterial or venous thrombosis. Most studies on HRT in patients with SLE found a link between HRT use and thrombotic events (129, 144, 145, 148–151). Although the risk of developing thrombosis increases after HRT or menopause in healthy women, the incidence of thrombosis in women with SLE increased dramatically from 0.08 to 0.11 per 1,000 person/year to 5.1 per 1,000 person/year (149, 150, 152). Several RCTs reported that HRT use alone did not increase the risk of thrombosis or coronary heart disease for patients with SLE with inactive or stable active disease, negative antiphospholipid antibodies, and no history of thrombosis (9, 153, 154). The effects of hormones on thrombosis and the reported data indicate that HRT is not safe in patients with SLE with antiphospholipid antibodies or prior vascular thrombotic events. Smoking, old age, female sex, disease activity, and glucocorticoid dose are also known to increase the risk of thrombosis; therefore, HRT use should be cautioned for patients with these risk factors (155).
Furthermore, the influence of HRT on malignancy risk is a serious concern for women. In the general population, cancer risk was increased by 9% among users of HRT, which carried widely depending on the type of cancer and HRT regimen (156). In particular, the relationship between HRT and female reproductive organ cancers, such as breast, endometrial, and ovarian cancer, is of great interest. Fortunately, the risk of these cancers is rather decreased in women with SLE (157, 158). The tendency of patients with SLE to have a higher age at menarche and lower age at menopause compared to the general population leads to a decrease in lifetime estrogen exposure, reducing the incidence of female reproductive organ cancers (159). Studies on the causal relationship between cancer incidence and HRT in patients with SLE are rare, and the association between cancer and HRT in SLE has not yet been clarified in published studies (160).
In conclusion, HRT use needs to be individually tailored in consideration of various conditions. For women with SLE, transdermal or percutaneous estrogen formulations are preferred over oral preparations, and micronized progesterone or pregnane derivatives are preferred over non-pregnane when using combined estrogen and progesterone HRT. Moreover, if HRT is unavoidable in active disease, non-estrogenic drugs should be selected first (161).
Conclusion
The effects of sex hormones, estrogen, and their receptors, especially ERα, have been found to promote autoimmune responses, namely, autoantibody production, and Th17 differentiation. In addition, DC activation and a type I IFN signature are modulated through TLR-7 and TLR-9 by estrogen or its receptor. While androgens inhibit B cell activation, testosterone and other androgens have not demonstrated therapeutic effects against SLE. Although sex hormones change during the menstrual cycle, flares rarely occur according to the menstrual cycle. Defective androgens are associated with male SLE, which is characterized by more frequent skin involvement and higher risk for renal damage. Although conflicting results have been reported regarding the use of OCPs and HRT in women with SLE, their use raises the risk of flares or cardiovascular diseases in patients with antiphospholipid antibodies or a history of thrombosis; therefore, hormone therapy for patients with SLE should be decided through close consultation.
Data availability statement
The original contributions presented in this study are included in the article/supplementary material, further inquiries can be directed to the corresponding author/s.
Author contributions
J-WK and J-YJ: conceptualization, methodology, formal analysis, investigation, data curation, writing—original draft preparation, review and editing, and project administration. J-WK: visualization. J-YJ: supervision and funding acquisition. All authors have read and agreed to the published version of the manuscript.
Funding
This study was supported by a grant from the Korea Research-Driven Hospitals project (HR16C0001) of the Korea Health Industry Development Institute and the Ministry of Health and Welfare.
Acknowledgments
We would like to thank Editage (www.editage.co.kr) for English language editing.
Conflict of interest
The authors declare that the research was conducted in the absence of any commercial or financial relationships that could be construed as a potential conflict of interest.
Publisher’s note
All claims expressed in this article are solely those of the authors and do not necessarily represent those of their affiliated organizations, or those of the publisher, the editors and the reviewers. Any product that may be evaluated in this article, or claim that may be made by its manufacturer, is not guaranteed or endorsed by the publisher.
Abbreviations
BAFF, B cell-activating factor; COC, combined oral contraceptives; DC, dendritic cell; dsDNA, double-stranded DNA; E2, estradiol; ER, estrogen receptor; FSH, follicle-stimulating hormone; HC, healthy control; HRT, hormone replacement therapy; OCP, oral contraceptive; PBMC, peripheral blood mononuclear cell; pDC, plasmacytoid DC; RCT, randomized controlled trial; SLE, systemic lupus erythematosus; SLEDAI, SLE disease activity index; WHI, Women’s Health Initiative.
References
1. Tsokos GC. Systemic lupus erythematosus. N Engl J Med. (2011) 365:2110–21. doi: 10.1056/NEJMra1100359
2. Kiriakidou M, Ching CL. Systemic lupus erythematosus. Ann Intern Med. (2020) 172:Itc81–96. doi: 10.7326/AITC202006020
3. Christou EAA, Banos A, Kosmara D, Bertsias GK, Boumpas DT. Sexual dimorphism in SLE: above and beyond sex hormones. Lupus. (2019) 28:3–10. doi: 10.1177/0961203318815768
4. Qin B, Wang J, Yang Z, Yang M, Ma N, Huang F, et al. Epidemiology of primary Sjögren’s syndrome: a systematic review and meta-analysis. Ann Rheum Dis. (2015) 74:1983–9. doi: 10.1136/annrheumdis-2014-205375
5. Ortona E, Pierdominici M, Maselli A, Veroni C, Aloisi F, Shoenfeld Y. Sex-based differences in autoimmune diseases. Ann Ist Super Sanita. (2016) 52:205–12.
6. Klein SL, Flanagan KL. Sex differences in immune responses. Nat Rev Immunol. (2016) 16:626–38. doi: 10.1038/nri.2016.90
7. Petri M. Sex hormones and systemic lupus erythematosus. Lupus. (2008) 17:412–5. doi: 10.1177/0961203308090026
8. Kim JW, Jung JY, Kim HA, Yang JI, Kwak DW, Suh CH. Lupus low disease activity state achievement is important for reducing adverse outcomes in pregnant patients with systemic lupus erythematosus. J Rheumatol. (2021) 48:707–16. doi: 10.3899/jrheum.200802
9. Buyon JP, Petri MA, Kim MY, Kalunian KC, Grossman J, Hahn BH, et al. The effect of combined estrogen and progesterone hormone replacement therapy on disease activity in systemic lupus erythematosus: a randomized trial. Ann Intern Med. (2005) 142:953–62. doi: 10.7326/0003-4819-142-12_Part_1-200506210-00004
10. Bernier MO, Mikaeloff Y, Hudson M, Suissa S. Combined oral contraceptive use and the risk of systemic lupus erythematosus. Arthritis Rheum. (2009) 61:476–81. doi: 10.1002/art.24398
11. Mok CC, Lau CS, Ho CT, Wong RW. Do flares of systemic lupus erythematosus decline after menopause? Scand J Rheumatol. (1999) 28:357–62. doi: 10.1080/03009749950155346
12. Andrews BS, Eisenberg RA, Theofilopoulos AN, Izui S, Wilson CB, McConahey PJ, et al. Spontaneous murine lupus-like syndromes. Clinical and immunopathological manifestations in several strains. J Exp Med. (1978) 148:1198–215. doi: 10.1084/jem.148.5.1198
13. Davey RA, Grossmann M. Androgen receptor structure, function and biology: from bench to bedside. Clin Biochem Rev. (2016) 37:3–15.
14. Rothman MS, Carlson NE, Xu M, Wang C, Swerdloff R, Lee P, et al. Reexamination of testosterone, dihydrotestosterone, estradiol and estrone levels across the menstrual cycle and in postmenopausal women measured by liquid chromatography-tandem mass spectrometry. Steroids. (2011) 76:177–82. doi: 10.1016/j.steroids.2010.10.010
15. Hughes GC, Choubey D. Modulation of autoimmune rheumatic diseases by oestrogen and progesterone. Nat Rev Rheumatol. (2014) 10:740–51. doi: 10.1038/nrrheum.2014.144
16. Cutolo M, Straub RH. Sex steroids and autoimmune rheumatic diseases: state of the art. Nat Rev Rheumatol. (2020) 16:628–44. doi: 10.1038/s41584-020-0503-4
17. Billi AC, Kahlenberg JM, Gudjonsson JE. Sex bias in autoimmunity. Curr Opin Rheumatol. (2019) 31:53–61. doi: 10.1097/BOR.0000000000000564
18. Elderman M, de Vos P, Faas M. Role of microbiota in sexually dimorphic immunity. Front Immunol. (2018) 9:1018. doi: 10.3389/fimmu.2018.01018
19. Markle JG, Frank DN, Mortin-Toth S, Robertson CE, Feazel LM, Rolle-Kampczyk U, et al. Sex differences in the gut microbiome drive hormone-dependent regulation of autoimmunity. Science. (2013) 339:1084–8. doi: 10.1126/science.1233521
20. Inui H, Ogasawara T, Naito Sekigawa I, Takasaki Y, Hayashida Y, Takamori K, et al. Estrogen receptor expression by peripheral blood mononuclear cells of patients with systemic lupus erythematosus. Clin Rheumatol. (2007) 26:1675–8. doi: 10.1007/s10067-007-0568-3
21. Kassi EN, Vlachoyiannopoulos PG, Moutsopoulos HM, Sekeris CE, Moutsatsou P. Molecular analysis of estrogen receptor alpha and beta in lupus patients. Eur J Clin Invest. (2001) 31:86–93. doi: 10.1046/j.1365-2362.2001.00762.x
22. Bynoté KK, Hackenberg JM, Korach KS, Lubahn DB, Lane PH, Gould KA. Estrogen receptor-alpha deficiency attenuates autoimmune disease in (NZB x NZW)F1 mice. Genes Immun. (2008) 9:137–52. doi: 10.1038/sj.gene.6364458
23. Svenson JL, EuDaly J, Ruiz P, Korach KS, Gilkeson GS. Impact of estrogen receptor deficiency on disease expression in the NZM2410 lupus prone mouse. Clin Immunol. (2008) 128:259–68. doi: 10.1016/j.clim.2008.03.508
24. Grimaldi CM, Cleary J, Dagtas AS, Moussai D, Diamond B. Estrogen alters thresholds for B cell apoptosis and activation. J Clin Invest. (2002) 109:1625–33. doi: 10.1172/JCI0214873
25. Kanda N, Tsuchida T, Tamaki K. Estrogen enhancement of anti-double-stranded DNA antibody and immunoglobulin G production in peripheral blood mononuclear cells from patients with systemic lupus erythematosus. Arthritis Rheum. (1999) 42:328–37. doi: 10.1002/1529-0131(199902)42:2<328::AID-ANR16>3.0.CO;2-#
26. Grimaldi CM, Jeganathan V, Diamond B. Hormonal regulation of B cell development: 17 beta-estradiol impairs negative selection of high-affinity DNA-reactive B cells at more than one developmental checkpoint. J Immunol. (2006) 176:2703–10. doi: 10.4049/jimmunol.176.5.2703
27. Bynoe MS, Grimaldi CM, Diamond B. Estrogen up-regulates Bcl-2 and blocks tolerance induction of naive B cells. Proc Natl Acad Sci USA. (2000) 97:2703–8. doi: 10.1073/pnas.040577497
28. Sthoeger ZM, Zinger H, Mozes E. Beneficial effects of the anti-oestrogen tamoxifen on systemic lupus erythematosus of (NZBxNZW)F1 female mice are associated with specific reduction of IgG3 autoantibodies. Ann Rheum Dis. (2003) 62:341–6. doi: 10.1136/ard.62.4.341
29. Panchanathan R, Choubey D. Murine BAFF expression is upregulated by estrogen and interferons: implications for sex bias in the development of autoimmunity. Mol Immunol. (2013) 53:15–23. doi: 10.1016/j.molimm.2012.06.013
30. Bassi N, Luisetto R, Ghirardello A, Gatto M, Valente M, Della Barbera M, et al. 17-β-estradiol affects BLyS serum levels and the nephritogenic autoantibody network accelerating glomerulonephritis in NZB/WF1 mice. Lupus. (2015) 24:382–91. doi: 10.1177/0961203314559636
31. Rider V, Li X, Peterson G, Dawson J, Kimler BF, Abdou NI. Differential expression of estrogen receptors in women with systemic lupus erythematosus. J Rheumatol. (2006) 33:1093–101.
32. Rider V, Jones SR, Evans M, Abdou NI. Molecular mechanisms involved in the estrogen-dependent regulation of calcineurin in systemic lupus erythematosus T cells. Clin Immunol. (2000) 95:124–34. doi: 10.1006/clim.2000.4844
33. Rider V, Jones S, Evans M, Bassiri H, Afsar Z, Abdou NI. Estrogen increases CD40 ligand expression in T cells from women with systemic lupus erythematosus. J Rheumatol. (2001) 28:2644–9.
34. Feng F, Nyland J, Banyai M, Tatum A, Silverstone AE, Gavalchin J. The induction of the lupus phenotype by estrogen is via an estrogen receptor-alpha-dependent pathway. Clin Immunol. (2010) 134:226–36. doi: 10.1016/j.clim.2009.10.004
35. Kim DH, Park HJ, Park HS, Lee JU, Ko C, Gye MC, et al. Estrogen receptor α in T cells suppresses follicular helper T cell responses and prevents autoimmunity. Exp Mol Med. (2019) 51:1–9. doi: 10.1038/s12276-019-0237-z
36. Singh RP, Bischoff DS. Sex hormones and gender influence the expression of markers of regulatory T Cells in SLE patients. Front Immunol. (2021) 12:619268. doi: 10.3389/fimmu.2021.619268
37. Perry DJ, Yin Y, Telarico T, Baker HV, Dozmorov I, Perl A, et al. Murine lupus susceptibility locus Sle1c2 mediates CD4+ T cell activation and maps to estrogen-related receptor gamma. J Immunol. (2012) 189:793–803. doi: 10.4049/jimmunol.1200411
38. Li W, Gong M, Park YP, Elshikha AS, Choi SC, Brown J, et al. Lupus susceptibility gene Esrrg modulates regulatory T cells through mitochondrial metabolism. JCI Insight. (2021) 6:e143540. doi: 10.1172/jci.insight.143540
39. Lélu K, Laffont S, Delpy L, Paulet PE, Périnat T, Tschanz SA, et al. Estrogen receptor α signaling in T lymphocytes is required for estradiol-mediated inhibition of Th1 and Th17 cell differentiation and protection against experimental autoimmune encephalomyelitis. J Immunol. (2011) 187:2386–93. doi: 10.4049/jimmunol.1101578
40. Chen RY, Fan YM, Zhang Q, Liu S, Li Q, Ke GL, et al. Estradiol inhibits Th17 cell differentiation through inhibition of RORγT transcription by recruiting the ERα/REA complex to estrogen response elements of the RORγT promoter. J Immunol. (2015) 194:4019–28. doi: 10.4049/jimmunol.1400806
41. Fuseini H, Cephus JY, Wu P, Davis JB, Contreras DC, Gandhi VD, et al. ERα signaling increased IL-17A production in Th17 cells by upregulating IL-23R expression, mitochondrial respiration, and proliferation. Front Immunol. (2019) 10:2740. doi: 10.3389/fimmu.2019.02740
42. Laffont S, Seillet C, Guéry JC. Estrogen receptor-dependent regulation of dendritic cell development and function. Front Immunol. (2017) 8:108. doi: 10.3389/fimmu.2017.00108
43. Siracusa MC, Overstreet MG, Housseau F, Scott AL, Klein SL. 17beta-estradiol alters the activity of conventional and IFN-producing killer dendritic cells. J Immunol. (2008) 180:1423–31. doi: 10.4049/jimmunol.180.3.1423
44. Scott JL, Cunningham MA, Naga OS, Wirth JR, Eudaly JG, Gilkeson GS. Estrogen receptor α deficiency modulates TLR ligand-mediated PDC-TREM expression in plasmacytoid dendritic cells in lupus-prone mice. J Immunol. (2015) 195:5561–71. doi: 10.4049/jimmunol.1500315
45. Berghöfer B, Frommer T, Haley G, Fink L, Bein G, Hackstein H. TLR7 ligands induce higher IFN-α production in females. J Immunol. (2006) 177:2088–96. doi: 10.4049/jimmunol.177.4.2088
46. Seillet C, Laffont S, Trémollières F, Rouquié N, Ribot C, Arnal JF, et al. The TLR-mediated response of plasmacytoid dendritic cells is positively regulated by estradiol in vivo through cell-intrinsic estrogen receptor α signaling. Blood. (2012) 119:454–64. doi: 10.1182/blood-2011-08-371831
47. Cunningham MA, Naga OS, Eudaly JG, Scott JL, Gilkeson GS. Estrogen receptor α modulates toll-like receptor signaling in murine lupus. Clin Immunol. (2012) 144:1–12. doi: 10.1016/j.clim.2012.04.001
48. Griesbeck M, Ziegler S, Laffont S, Smith N, Chauveau L, Tomezsko P, et al. Sex differences in plasmacytoid dendritic cell levels of IRF5 drive higher IFN-α production in women. J Immunol. (2015) 195:5327–36. doi: 10.4049/jimmunol.1501684
49. Young NA, Wu LC, Burd CJ, Friedman AK, Kaffenberger BH, Rajaram MV, et al. Estrogen modulation of endosome-associated toll-like receptor 8: an IFNα-independent mechanism of sex-bias in systemic lupus erythematosus. Clin Immunol (2014) 151:66–77. doi: 10.1016/j.clim.2014.01.006
50. Edwards MR, Dai R, Heid B, Cowan C, Werre SR, Cecere T, et al. Low-dose 17alpha-ethinyl estradiol (EE) exposure exacerbates lupus renal disease and modulates immune responses to TLR7/9 agonists in genetically autoimmune-prone mice. Sci Rep. (2020) 10:5210. doi: 10.1038/s41598-020-62124-6
51. Young NA, Valiente GR, Hampton JM, Wu LC, Burd CJ, Willis WL, et al. Estrogen-regulated STAT1 activation promotes TLR8 expression to facilitate signaling via microRNA-21 in systemic lupus erythematosus. Clin Immunol. (2017) 176:12–22. doi: 10.1016/j.clim.2016.12.005
52. Liu HW, Lin HL, Yen JH, Tsai WC, Chiou SS, Chang JG, et al. Demethylation within the proximal promoter region of human estrogen receptor α gene correlates with its enhanced expression: implications for female bias in lupus. Mol Immunol. (2014) 61:28–37. doi: 10.1016/j.molimm.2014.05.002
53. Wu Z, Sun Y, Mei X, Zhang C, Pan W, Shi W. 17β-oestradiol enhances global DNA hypomethylation in CD4-positive T cells from female patients with lupus, through overexpression of oestrogen receptor-α-mediated downregulation of DNMT1. Clin Exp Dermatol. (2014) 39:525–32. doi: 10.1111/ced.12346
54. Ramanujan SA, Cravens EN, Krishfield SM, Kyttaris VC, Moulton VR. Estrogen-induced hsa-miR-10b-5p is elevated in T cells from patients with systemic lupus erythematosus and down-regulates serine/arginine-rich splicing factor 1. Arthritis Rheumatol. (2021) 73:2052–8. doi: 10.1002/art.41787
55. Dong G, Fan H, Yang Y, Zhao G, You M, Wang T, et al. 17β-estradiol enhances the activation of IFN-α signaling in B cells by down-regulating the expression of let-7e-5p, miR-98-5p and miR-145a-5p that target IKKε. Biochim Biophys Acta. (2015) 1852:1585–98. doi: 10.1016/j.bbadis.2015.04.019
56. Papapavlou G, Hellberg S, Raffetseder J, Brynhildsen J, Gustafsson M, Jenmalm MC, et al. Differential effects of estradiol and progesterone on human T cell activation in vitro. Eur J Immunol. (2021) 51:2430–40. doi: 10.1002/eji.202049144
57. Hughes GC, Martin D, Zhang K, Hudkins KL, Alpers CE, Clark EA, et al. Decrease in glomerulonephritis and Th1-associated autoantibody production after progesterone treatment in NZB/NZW mice. Arthritis Rheum. (2009) 60:1775–84. doi: 10.1002/art.24548
58. Keisler LW, Kier AB, Walker SE. Effects of prolonged administration of the 19-nor-testosterone derivatives norethindrone and norgestrel to female NZB/W mice: comparison with medroxyprogesterone and ethinyl estradiol. Autoimmunity. (1991) 9:21–32. doi: 10.3109/08916939108997120
59. Wong AH, Agrawal N, Hughes GC. Altered IgG autoantibody levels and CD4(+) T cell subsets in lupus-prone Nba2 mice lacking the nuclear progesterone receptor. Autoimmunity. (2015) 48:389–401. doi: 10.3109/08916934.2015.1030613
60. Wilhelmson AS, Stubelius A, Börjesson AE, Wu J, Stern A, Malin S, et al. Androgens regulate bone marrow B lymphopoiesis in male mice by targeting osteoblast-lineage cells. Endocrinology. (2015) 156:1228–36. doi: 10.1210/en.2014-1822
61. Wilhelmson AS, Lantero Rodriguez M, Stubelius A, Fogelstrand P, Johansson I, Buechler MB, et al. Testosterone is an endogenous regulator of BAFF and splenic B cell number. Nat commun. (2018) 9:2067. doi: 10.1038/s41467-018-04408-0
62. Lahita RG, Bradlow HL, Ginzler E, Pang S, New M. Low plasma androgens in women with systemic lupus erythematosus. Arthritis Rheum. (1987) 30:241–8. doi: 10.1002/art.1780300301
63. Gordon C, Wallace DJ, Shinada S, Kalunian KC, Forbess L, Braunstein GD, et al. Testosterone patches in the management of patients with mild/moderate systemic lupus erythematosus. Rheumatology. (2008) 47:334–8. doi: 10.1093/rheumatology/kem342
64. Lahita RG, Cheng CY, Monder C, Bardin CW. Experience with 19-nortestosterone in the therapy of systemic lupus erythematosus: worsened disease after treatment with 19-nortestosterone in men and lack of improvement in women. J Rheumatol. (1992) 19:547–55.
65. van Vollenhoven RF, Park JL, Genovese MC, West JP, McGuire JL. A double-blind, placebo-controlled, clinical trial of dehydroepiandrosterone in severe systemic lupus erythematosus. Lupus. (1999) 8:181–7. doi: 10.1191/096120399678847588
66. Petri MA, Mease PJ, Merrill JT, Lahita RG, Iannini MJ, Yocum DE, et al. Effects of prasterone on disease activity and symptoms in women with active systemic lupus erythematosus. Arthritis Rheum. (2004) 50:2858–68. doi: 10.1002/art.20427
67. Ekblom-Kullberg S, Kautiainen H, Alha P, Helve T, Leirisalo-Repo M, Julkunen H. Reproductive health in women with systemic lupus erythematosus compared to population controls. Scand J Rheumatol. (2009) 38:375–80. doi: 10.1080/03009740902763099
68. Ceccarelli F, Orefice V, Perrone G, Pirone C, Perricone C, Truglia S, et al. Premature ovarian failure in patients affected by systemic lupus erythematosus: a cross-sectional study. Clin Exp Rheumatol. (2020) 38:450–4.
69. Mayorga J, Alpízar-Rodríguez D, Prieto-Padilla J, Romero-Díaz J, Cravioto MC. Prevalence of premature ovarian failure in patients with systemic lupus erythematosus. Lupus. (2016) 25:675–83. doi: 10.1177/0961203315622824
70. Medeiros PB, Febronio MV, Bonfa E, Borba EF, Takiuti AD, Silva CA. Menstrual and hormonal alterations in juvenile systemic lupus erythematosus. Lupus. (2009) 18:38–43. doi: 10.1177/0961203308094652
71. Colangelo K, Haig S, Bonner A, Zelenietz C, Pope J. Self-reported flaring varies during the menstrual cycle in systemic lupus erythematosus compared with rheumatoid arthritis and fibromyalgia. Rheumatology. (2011) 50:703–8. doi: 10.1093/rheumatology/keq360
72. Kawasaki M, Sekigawa I, Nozawa K, Kaneko H, Takasaki Y, Takamori K, et al. Changes in the gene expression of peripheral blood mononuclear cells during the menstrual cycle of females is associated with a gender bias in the incidence of systemic lupus erythematosus. Clin Exp Rheumatol. (2009) 27:260–6.
73. Brundin PMA, Landgren BM, Fjallstrom P, Shamekh MM, Gustafsson JA, Johansson AF, et al. Expression of sex hormone receptor and immune response genes in peripheral blood mononuclear cells during the menstrual cycle. Front Endocrinol. (2021) 12:721813. doi: 10.3389/fendo.2021.721813
74. Lu LJ, Wallace DJ, Ishimori ML, Scofield RH, Weisman MH. Review: Male systemic lupus erythematosus: a review of sex disparities in this disease. Lupus. (2010) 19:119–29. doi: 10.1177/0961203309350755
75. Roubinian JR, Talal N, Greenspan JS, Goodman JR, Siiteri PK. Delayed androgen treatment prolongs survival in murine lupus. J Clin Invest. (1979) 63:902–11. doi: 10.1172/JCI109390
76. Roubinian JR, Talal N, Greenspan JS, Goodman JR, Siiteri PK. Effect of castration and sex hormone treatment on survival, anti-nucleic acid antibodies, and glomerulonephritis in NZB/NZW F1 mice. J Exp Med. (1978) 147:1568–83. doi: 10.1084/jem.147.6.1568
77. Trigunaite A, Khan A, Der E, Song A, Varikuti S, Jørgensen TN. Gr-1(high) CD11b+ cells suppress B cell differentiation and lupus-like disease in lupus-prone male mice. Arthritis Rheum. (2013) 65:2392–402. doi: 10.1002/art.38048
78. Jones JM, Jorgensen TN. Androgen-mediated anti-inflammatory cellular processes as therapeutic targets in lupus. Front Immunol. (2020) 11:1271. doi: 10.3389/fimmu.2020.01271
79. Stahl NI, Decker JL. Androgenic status of males with systemic lupus erythematosus. Arthritis Rheum. (1978) 21:665–8. doi: 10.1002/art.1780210609
80. Mok CC, Lau CS. Profile of sex hormones in male patients with systemic lupus erythematosus. Lupus. (2000) 9:252–7. doi: 10.1191/096120300680198926
81. Mackworth-Young CG, Parke AL, Morley KD, Fotherby K, Hughes GR. Sex hormones in male patients with systemic lupus erythematosus: a comparison with other disease groups. Eur J Rheumatol Inflamm. (1983) 6:228–32.
82. Olsen NJ, Kovacs WJ. Case report: testosterone treatment of systemic lupus erythematosus in a patient with Klinefelter’s syndrome. Am J Med Sci. (1995) 310:158–60. doi: 10.1097/00000441-199510000-00006
83. Sasaki N, Yamauchi K, Sato R, Masuda T, Sawai T, Inoue H. Klinefelter’s syndrome associated with systemic lupus erythematosus and autoimmune hepatitis. Mod Rheumatol. (2006) 16:305–8. doi: 10.1007/s10165-006-0511-5
84. Ocon A, Peredo-Wende R, Kremer JM, Bhatt BD. Significant symptomatic improvement of subacute cutaneous lupus after testosterone therapy in a female-to-male transgender subject. Lupus. (2018) 27:347–8. doi: 10.1177/0961203317734921
85. Scofield RH, Bruner GR, Namjou B, Kimberly RP, Ramsey-Goldman R, Petri M, et al. Klinefelter’s syndrome (47,XXY) in male systemic lupus erythematosus patients: support for the notion of a gene-dose effect from the X chromosome. Arthritis Rheum. (2008) 58:2511–7. doi: 10.1002/art.23701
86. Liu K, Kurien BT, Zimmerman SL, Kaufman KM, Taft DH, Kottyan LC, et al. X chromosome dose and sex bias in autoimmune diseases: increased prevalence of 47, XXX in systemic lupus erythematosus and Sjogren’s syndrome. Arthritis Rheumatol. (2016) 68:1290–300.
87. Dillon SP, Kurien BT, Li S, Bruner GR, Kaufman KM, Harley JB, et al. Sex chromosome aneuploidies among men with systemic lupus erythematosus. J Autoimmun. (2012) 38:129–34. doi: 10.1016/j.jaut.2011.10.004
88. Youness A, Miqual C-H, Guery J-C. Escape from X chromosome inactivation and the female predominance in autoimmune diseases. Int J Mol Sci. (2021) 22:1114. doi: 10.3390/ijms22031114
89. Rees F, Doherty M, Grainge MJ, Lanyon P, Zhang W. The worldwide incidence and prevalence of systemic lupus erythematosus: a systematic review of epidemiological studies. Rheumatology. (2017) 56:1945–61. doi: 10.1093/rheumatology/kex260
90. Murphy G, Isenberg D. Effect of gender on clinical presentation in systemic lupus erythematosus. Rheumatology. (2013) 52:2108–15. doi: 10.1093/rheumatology/ket160
91. Rees F, Doherty M, Grainge M, Davenport G, Lanyon P, Zhang W. The incidence and prevalence of systemic lupus erythematosus in the UK, 1999-2012. Ann Rheum Dis. (2016) 75:136–41. doi: 10.1136/annrheumdis-2014-206334
92. Garcia MA, Marcos JC, Marcos AI, Pons-Estel BA, Wojdyla D, Arturi A, et al. Male systemic lupus erythematosus in a Latin-American inception cohort of 1214 patients. Lupus. (2005) 14:938–46. doi: 10.1191/0961203305lu2245oa
93. Soto ME, Vallejo M, Guillén F, Simón JA, Arena E, Reyes PA. Gender impact in systemic lupus erythematosus. Clin Exp Rheumatol. (2004) 22:713–21.
94. Sayhi S, Achour TB, Mezri S, Hamdi MS, Nour G, Bilel A, et al. Clinical features of systemic lupus erythematosus in Tunisian males. Curr Rheumatol Rev. (2020) 16:139–42. doi: 10.2174/1573397115666190916142945
95. Renau AI, Isenberg DA. Male versus female lupus: a comparison of ethnicity, clinical features, serology and outcome over a 30 year period. Lupus. (2012) 21:1041–8. doi: 10.1177/0961203312444771
96. Riveros Frutos A, Casas I, Rúa-Figueroa I, López-Longo FJ, Calvo-Alén J, Galindo M, et al. Systemic lupus erythematosus in Spanish males: a study of the Spanish Rheumatology Society Lupus Registry (RELESSER) cohort. Lupus. (2017) 26:698–706. doi: 10.1177/0961203316673728
97. Andrade RM, Alarcón GS, Fernández M, Apte M, Vilá LM, Reveille JD. Accelerated damage accrual among men with systemic lupus erythematosus: XLIV. Results from a multiethnic US cohort. Arthritis Rheum. (2007) 56:622–30. doi: 10.1002/art.22375
98. Tabor DE, Gould KA. Estrogen receptor alpha promotes lupus in (NZB×NZW)F1 mice in a B cell intrinsic manner. Clin Immunol. (2017) 174:41–52. doi: 10.1016/j.clim.2016.10.011
99. Tan TC, Fang H, Magder LS, Petri MA. Differences between male and female systemic lupus erythematosus in a multiethnic population. J Rheumatol. (2012) 39:759–69. doi: 10.3899/jrheum.111061
100. Shaharir SS, Kadir WDA, Nordin F, Bakar FA, Ting MWH, Jamil A, et al. Systemic lupus erythematosus among male patients in Malaysia: how are we different from other geographical regions? Lupus. (2019) 28:137–44. doi: 10.1177/0961203318812676
101. Voulgari PV, Katsimbri P, Alamanos Y, Drosos AA. Gender and age differences in systemic lupus erythematosus. A study of 489 Greek patients with a review of the literature. Lupus. (2002) 11:722–9. doi: 10.1191/0961203302lu253oa
102. Stefanidou S, Benos A, Galanopoulou V, Chatziyannis I, Kanakoudi F, Aslanidis S, et al. Clinical expression and morbidity of systemic lupus erythematosus during a post-diagnostic 5-year follow-up: a male:female comparison. Lupus. (2011) 20:1090–4. doi: 10.1177/0961203311403640
103. de Carvalho JF, do Nascimento AP, Testagrossa LA, Barros RT, Bonfá E. Male gender results in more severe lupus nephritis. Rheumatol Int. (2010) 30:1311–5. doi: 10.1007/s00296-009-1151-9
104. Boodhoo KD, Liu S, Zuo X. Impact of sex disparities on the clinical manifestations in patients with systemic lupus erythematosus: A systematic review and meta-analysis. Medicine. (2016) 95:e4272. doi: 10.1097/MD.0000000000004272
105. Feldman CH, Broder A, Guan H, Yazdany J, Costenbader KH. Sex differences in health care utilization, end-stage renal disease, and mortality among Medicaid Beneficiaries with incident lupus nephritis. Arthritis Rheumatol. (2018) 70:417–26. doi: 10.1002/art.40392
106. Aranow C, Del Guidice J, Barland P, Weinstein A. Systemic lupus erythematosus disease severity in men and women: a case-control study. J Rheumatol. (2002) 29:1674–7.
107. Hwang J, Lee J, Ahn JK, Park EJ, Cha HS, Koh EM. Clinical characteristics of male and female Korean patients with systemic lupus erythematosus: a comparative study. Korean J Intern Med. (2015) 30:242–9. doi: 10.3904/kjim.2015.30.2.242
108. Hsu CY, Chiu WC, Yang TS, Chen CJ, Chen YC, Lai HM, et al. Age- and gender-related long-term renal outcome in patients with lupus nephritis. Lupus. (2011) 20:1135–41. doi: 10.1177/0961203311404912
109. Peng W, Tang Y, Tan L, Qin W. Clinicopathological study of male and female patients with lupus nephritis: a retrospective study. Int Urol Nephrol. (2018) 50:313–20. doi: 10.1007/s11255-017-1780-y
110. Lin WH, Guo CY, Wang WM, Yang DC, Kuo TH, Liu MF, et al. Incidence of progression from newly diagnosed systemic lupus erythematosus to end stage renal disease and all-cause mortality: a nationwide cohort study in Taiwan. Int J Rheum Dis. (2013) 16:747–53. doi: 10.1111/1756-185X.12208
111. Birru Talabi M, Himes KP, Clowse MEB. Optimizing reproductive health management in lupus and Sjogren’s syndrome. Curr Opin Rheumatol. (2021) 33:570–8. doi: 10.1097/BOR.0000000000000839
112. Christin-Maitre S. History of oral contraceptive drugs and their use worldwide. Best Pract Res Clin Endocrinol Metab. (2013) 27:3–12. doi: 10.1016/j.beem.2012.11.004
113. Benagiano G, Benagiano M, Bianchi P, D’Elios MM, Brosens I. Contraception in autoimmune diseases. Best Pract Res Clin Obstet Gynaecol. (2019) 60:111–23. doi: 10.1016/j.bpobgyn.2019.05.003
114. Petri M. Exogenous estrogen in systemic lupus erythematosus: oral contraceptives and hormone replacement therapy. Lupus. (2001) 10:222–6. doi: 10.1191/096120301676707393
115. Pimstone B. Systemic lupus erythematosus exacerbated by oral contraceptives. S Afr Med J. (1966) 4:62–3.
116. Chapel TA, Burns R. Oral contraceptives and exacerbation of lupus erythematosus. Am J Obstet Gynecol. (1971) 110:366–9. doi: 10.1016/0002-9378(71)90730-7
117. Jungers P, Dougados M, Pélissier C, Kuttenn F, Tron F, Lesavre P, et al. Influence of oral contraceptive therapy on the activity of systemic lupus erythematosus. Arthritis Rheum. (1982) 25:618–23. doi: 10.1002/art.1780250603
118. Miller MH. Pulmonary hypertension, systemic lupus erythematosus, and the contraceptive pill: another report. Ann Rheum Dis. (1987) 46:159–61. doi: 10.1136/ard.46.2.159
119. Julkunen H, Kaaja R, Jouhikainen T, Teppo AM, Friman C. Malignant hypertension and antiphospholipid antibodies as presenting features of SLE in a young woman using oral contraceptives. Br J Rheumatol. (1991) 30:471–2. doi: 10.1093/rheumatology/30.6.471
120. Buyon JP, Kalunian KC, Skovron ML, Petri M, Lahita R, Merrill J, et al. Can women with systemic lupus erythematosus safely use exogenous estrogens? J Clin Rheumatol. (1995) 1:205–12. doi: 10.1097/00124743-199508000-00002
121. Todd GR, McAteer EJ, Jack CM, Haire M, Roberts SD, Buchanan KD. Pulmonary hypertension, systemic lupus erythematosus, and the contraceptive pill. Ann Rheum Dis. (1985) 44:266–7. doi: 10.1136/ard.44.4.266
122. Travers RL, Hughes GR. Oral contraceptive therapy and systemic lupus erythematosus. J Rheumatol. (1978) 5:448–51.
123. Garovich M, Agudelo C, Pisko E. Oral contraceptives and systemic lupus erythematosus. Arthritis Rheum. (1980) 23:1396–8. doi: 10.1002/art.1780231213
124. Sanchez-Guerrero J, Karlson EW, Liang MH, Hunter DJ, Speizer FE, Colditz GA. Past use of oral contraceptives and the risk of developing systemic lupus erythematosus. Arthritis Rheum. (1997) 40:804–8. doi: 10.1002/art.1780400505
125. Costenbader KH, Feskanich D, Stampfer MJ, Karlson EW. Reproductive and menopausal factors and risk of systemic lupus erythematosus in women. Arthritis Rheum. (2007) 56:1251–62. doi: 10.1002/art.22510
126. Grimes DA, LeBolt SA, Grimes KR, Wingo PA. Systemic lupus erythematosus and reproductive function: a case-control study. Am J Obstet Gynecol. (1985) 153:179–86. doi: 10.1016/0002-9378(85)90108-5
127. Strom BL, Reidenberg MM, West S, Snyder ES, Freundlich B, Stolley PD. Shingles, allergies, family medical history, oral contraceptives, and other potential risk factors for systemic lupus erythematosus. Am J Epidemiol. (1994) 140:632–42. doi: 10.1093/oxfordjournals.aje.a117302
128. Bengtsson AA, Rylander L, Hagmar L, Nived O, Sturfelt G. Risk factors for developing systemic lupus erythematosus: a case-control study in southern Sweden. Rheumatology. (2002) 41:563–71. doi: 10.1093/rheumatology/41.5.563
129. Cooper GS, Dooley MA, Treadwell EL, St Clair EW, Gilkeson GS. Hormonal and reproductive risk factors for development of systemic lupus erythematosus: results of a population-based, case-control study. Arthritis Rheum. (2002) 46:1830–9. doi: 10.1002/art.10365
130. Kennedy JM. Oral contraceptives and ANA positivity. Arthritis Rheum. (1977) 20:1567–9. doi: 10.1002/art.1780200832
131. Sánchez-Guerrero JS, Uribe AG, Jiménez-Santana L, Mestanza-Peralta M, Lara-Reyes P, Seuc AH, et al. A trial of contraceptive methods in women with systemic lupus erythematosus. N Engl J Med. (2005) 353:2539–49. doi: 10.1056/NEJMoa050817
132. Petri M, Kim MY, Kalunian KC, Grossman J, Hahn BH, Sammaritano LR, et al. Combined oral contraceptives in women with systemic lupus erythematosus. N Engl J Med. (2005) 353:2550–8. doi: 10.1056/NEJMoa051135
133. Duarte C, Inês L. Oral contraceptives and systemic lupus erythematosus: what should we advise to our patients? Acta Reumatol Port. (2010) 35:133–40.
134. Rojas-Villarraga A, Torres-Gonzalez JV, Ruiz-Sternberg M. Safety of hormonal replacement therapy and oral contraceptives in systemic lupus erythematosus: a systematic review and meta-analysis. PLoS One. (2014) 9:e104303. doi: 10.1371/journal.pone.0104303
135. Lobo RA. Hormone-replacement therapy: current thinking. Nat Rev Endocrinol. (2017) 13:220–31. doi: 10.1038/nrendo.2016.164
136. Wolf PH, Madans JH, Finucane FF, Higgins M, Kleinman JC. Reduction of cardiovascular disease—related mortality among postmenopausal women who use hormones: evidence from a national cohort. Am J Obstet Gynecol. (1991) 164:489–94. doi: 10.1016/S0002-9378(11)80006-2
137. Cauley JA, Robbins J, Chen Z, Cummings SR, Jackson RD, LaCroix AZ, et al. Effects of estrogen plus progestin on risk of fracture and bone mineral density: the Women’s Health Initiative randomized trial. JAMA. (2003) 290:1729–38. doi: 10.1001/jama.290.13.1729
138. Rossouw JE, Anderson GL, Prentice RL, LaCroix AZ, Kooperberg C, Stefanick ML, et al. Writing Group for the Women’s Health Initiative Investigators. Risks and benefits of estrogen plus progestin in healthy postmenopausal women: principal results from the Women’s Health Initiative randomized controlled trial. JAMA. (2002) 288:321–33. doi: 10.1001/jama.288.3.321
139. Anderson GL, Limacher M, Assaf AR, Bassford T, Beresford SA, Black H, et al. Effects of conjugated equine estrogen in postmenopausal women with hysterectomy: the Women’s Health Initiative randomized controlled trial. JAMA. (2004) 291:1701–12. doi: 10.1001/jama.291.14.1701
140. Lundberg G, Wu P, Wenger N. Menopausal hormone therapy: a comprehensive review. Curr Atheroscler Rep. (2020) 22:33. doi: 10.1007/s11883-020-00854-8
141. Palacios S, Stevenson JC, Schaudig K, Lukasiewicz M, Graziottin A. Hormone therapy for first-line management of menopausal symptoms: practical recommendations. Womens Health. (2019) 15:1745506519864009. doi: 10.1177/1745506519864009
142. Sánchez-Guerrero JS, Liang MH, Karlson EW, Hunter DJ, Colditz GA. Postmenopausal estrogen therapy and the risk for developing systemic lupus erythematosus. Ann Intern Med. (1995) 122:430–3. doi: 10.7326/0003-4819-122-6-199503150-00005
143. Meier CR, Sturkenboom MC, Cohen AS, Jick H. Postmenopausal estrogen replacement therapy and the risk of developing systemic lupus erythematosus or discoid lupus. J Rheumatol. (1998) 25:1515–9.
144. Arden N, Lloyd M, Spector T, Hughes G. Safety of hormone replacement therapy (HRT) in systemic lupus erythematosus (SLE). Lupus. (1994) 3:11–3. doi: 10.1177/096120339400300104
145. Kreidstein S, Urowitz M, Gladman D, Gough J. Hormone replacement therapy in systemic lupus erythematosus. J Rheumatol. (1997) 24:2149–52.
146. Mok CL, Ho CTK, Lee KW, Mok MY, Wong RWS, Safety CC. of hormonal replacement therapy in postmenopausal patients with systemic lupus erythematosus. Scand J Rheumatol. (1998) 27:342–6. doi: 10.1080/03009749850154357
147. Barrett C, Neylon N, Snaith M. Oestrogen-induced systemic lupus erythematosus. Rheumatology. (1986) 25:300–1. doi: 10.1093/rheumatology/25.3.300
148. Sánchez-Guerrero JS, González-Pérez M, Durand-Carbajal M, Lara-Reyes P, Jiménez-Santana L, Romero-Díaz J, et al. Menopause hormonal therapy in women with systemic lupus erythematosus. Arthritis Rheum. (2007) 56:3070–9. doi: 10.1002/art.22855
149. Somers E, Magder LS, Petri M. Antiphospholipid antibodies and incidence of venous thrombosis in a cohort of patients with systemic lupus erythematosus. J Rheumatol. (2002) 29:2531–6.
150. Sarabi ZS, Chang E, Bobba R, Ibanez D, Gladman D, Urowitz M, et al. Incidence rates of arterial and venous thrombosis after diagnosis of systemic lupus erythematosus. Arthritis Rheum. (2005) 53:609–12. doi: 10.1002/art.21314
151. Hulley S, Grady D, Bush T, Furberg C, Herrington D, Riggs B, et al. Randomized trial of estrogen plus progestin for secondary prevention of coronary heart disease in postmenopausal women. Heart and Estrogen/progestin Replacement Study (HERS) Research Group. JAMA. (1998) 280:605–13. doi: 10.1001/jama.280.7.605
152. Daly E, Vessey MP, Hawkins MM, Carson JL, Gough P, Marsh S. Risk of venous thromboembolism in users of hormone replacement therapy. Lancet. (1996) 348:977–80. doi: 10.1016/S0140-6736(96)07113-9
153. Hochman J, Urowitz MB, Ibañez D, Gladman DD. Hormone replacement therapy in women with systemic lupus erythematosus and risk of cardiovascular disease. Lupus. (2009) 18:313–7. doi: 10.1177/0961203308097475
154. Fernández M, Calvo-Alén J, Bertoli AM, Bastian HM, Fessler BJ, McGwin G Jr., et al. Systemic lupus erythematosus in a multiethnic US cohort (LUMINA L II): relationship between vascular events and the use of hormone replacement therapy in postmenopausal women. J Clin Rheumatol. (2007) 13:261–5. doi: 10.1097/RHU.0b013e318156bbf5
155. Grygiel-Górniak B, Puszczewicz MJ. The influence of endogenous and exogenous sex hormones on systemic lupus erythematosus in pre- and postmenopausal women. Prz Menopauzalny. (2014) 13:262–6. doi: 10.5114/pm.2014.45003
156. Simin J, Tamimi R, Lagergren J, Adami HO, Brusselaers N. Menopausal hormone therapy and cancer risk: an overestimated risk? Eur J Cancer. (2017) 84:60–8. doi: 10.1016/j.ejca.2017.07.012
157. D’Alonzo M, Bounous VE, Villa M, Biglia N. Current evidence of the oncological benefit-risk profile of hormone replacement therapy. Medicina. (2019) 55:573. doi: 10.3390/medicina55090573
158. Fanouriakis A, Tziolos N, Bertsias G, Boumpas DT. Update on the diagnosis and management of systemic lupus erythematosus. Ann Rheum Dis. (2021) 80:14–25. doi: 10.1136/annrheumdis-2020-218272
159. Ladouceur A, Clarke AE, Ramsey-Goldman R, Bernatsky S. Malignancies in systemic lupus erythematosus: an update. Curr Opin Rheumatol. (2019) 31:678–81. doi: 10.1097/BOR.0000000000000648
160. Bernatsky S, Clarke A, Ramsey-Goldman R, Joseph L, Boivin JF, Rajan R, et al. Hormonal exposures and breast cancer in a sample of women with systemic lupus erythematosus. Rheumatology. (2004) 43:1178–81. doi: 10.1093/rheumatology/keh282
Keywords: systemic lupus erythematosus, sex hormone, clinical characteristic, pathogenesis, hormone therapy
Citation: Kim J-W, Kim H-A, Suh C-H and Jung J-Y (2022) Sex hormones affect the pathogenesis and clinical characteristics of systemic lupus erythematosus. Front. Med. 9:906475. doi: 10.3389/fmed.2022.906475
Received: 28 March 2022; Accepted: 26 July 2022;
Published: 11 August 2022.
Edited by:
Veena Taneja, Mayo Clinic, United StatesReviewed by:
Francesca Gilli, Dartmouth College, United StatesRam Raj Singh, University of California, Los Angeles, United States
Copyright © 2022 Kim, Kim, Suh and Jung. This is an open-access article distributed under the terms of the Creative Commons Attribution License (CC BY). The use, distribution or reproduction in other forums is permitted, provided the original author(s) and the copyright owner(s) are credited and that the original publication in this journal is cited, in accordance with accepted academic practice. No use, distribution or reproduction is permitted which does not comply with these terms.
*Correspondence: Ju-Yang Jung, anV5YW5nanVuZzIwQGdtYWlsLmNvbQ==