- 1Internal Medicine Department, Faculty of Medicine, Cairo University, Cairo, Egypt
- 2General Surgery Department, Faculty of Medicine, Cairo University, Cairo, Egypt
- 3Internal Medicine Department, Faculty of Medicine, Mansoura University, Mansoura, Egypt
- 4Department of Endemic Medicine and Hepatology, Faculty of Medicine, Cairo University, Cairo, Egypt
- 5Tropical and Gastroenterology Department, National Hepatology and Tropical Medicine Research Institute, Cairo, Egypt
- 6General Surgery Department, National Hepatology and Tropical Medicine Research Institute, Cairo, Egypt
- 7Department of General Surgery, Faculty of Medicine, Al-Azhar University, Cairo, Egypt
- 8Radiodiagnosis Department, Faculty of Medicine, Alazhar University, Cairo, Egypt
Purpose: Obesity is associated with fat accumulation in ectopic sites such as the pancreas, the so-called pancreatic steatosis (PS). Bariatric surgery has been shown to be associated with reducing pancreatic fat. This study investigated the effect of laparoscopic sleeve gastrectomy (LSG) on pancreatic volume and its fat content and glucose homeostasis.
Methods: The study enrolled 54 patients subjected to LSG. Metabolic variables and pancreatic exocrine function were assessed immediately before surgery and 12 months after. MRI of the abdomen was performed to measure pancreatic fat content and its total volume and visceral adipose tissue (VAT).
Results: Surgery resulted in a significant reduction in body weight and BMI. HbA1c, fasting insulin, C-peptide levels, HOMA-IR, and Hs-CRP levels decreased significantly. Surgery resulted in significant improvement in lipid profile except for HDL-cholesterol and liver function tests. Total VAT volume decreased significantly. Total pancreas volume decreased by a mean of 9.0 cm3 (95% CI: 6.6–11.3). The median change of pancreatic fat was −26.1% (range: −55.6 to 58.3%). Pancreatic lipase decreased significantly (P < 0.001). There was a positive correlation between the percentage of total weight loss and decrease in pancreatic fat volume (r = 0.295, P = 0.030).
Conclusion: Weight loss after LSG is associated with a reduction of total VAT volume, total pancreatic volume, and pancreatic fat content. These changes are associated with improved glucose homeostasis, reduced systemic inflammation, and decreased pancreatic lipase secretion.
Introduction
Obesity leads to adipose tissue dysfunction in which hypertrophic adipocytes secrete adipokines resulting in the recruitment of pre-adipocytes that differentiate into mature adipocytes (1). This process leads to fat accumulation in ectopic sites such as visceral depots, liver, skeletal muscles, and pancreas (2, 3). It was shown that visceral adipose tissue (VAT) accumulation is strongly associated with insulin resistance (IR), metabolic syndrome (MetS), predisposition to diabetes, local and systemic inflammation, and higher mortality rate (4, 5).
Fat accumulation in the pancreas is called pancreatic steatosis (PS), the most common benign pathologic condition of the pancreas in adults (6). It was demonstrated that nearly 2/3 of obese individuals has pancreatic fat deposition (7). Pancreatic fat content is significantly correlated with higher body mass index (BMI) and advanced age (8). Besides, ectopic pancreatic fat has been linked to β-cell dysfunction and type-2 diabetes mellitus (T2DM) (9, 10). Also, PS was shown to accelerate acute pancreatitis due to elevated levels of lipolysis and inflammation (11).
Bariatric surgery is widely recognized as the most effective treatment approach for obesity with a relatively low odds of obesity remission (12). Patients may lose nearly half of their subcutaneous and visceral fat within the first year after surgery (13). Subsequent weight loss is mainly from visceral depots, which correlates with the extent of diabetes remission (14). Some reports have shown a reduction of ectopic pancreatic fat following bariatric surgery, an effect that might promote improved glucose metabolism (14–16).
This study aimed to explore the effects of weight loss after laparoscopic sleeve gastrectomy on pancreatic volume and its fat content and glucose homeostasis.
Subjects and Methods
The study enrolled 54 subjects with morbid obesity who underwent laparoscopic sleeve gastrectomy (LSG). The inclusion criteria were an age of 18 years or more of both sexes having a preoperative body mass index (BMI) between 35 and 50 kg/m2. Patients were excluded if they were diagnosed with type-1 diabetes mellitus, gave a history of acute/chronic pancreatitis, were currently treated or have been treated with incretin-based therapies for T2DM, had evidence of current abuse of drugs or alcohol, or a history of severe claustrophobia. In addition, those with pacemakers, joint replacements, coronary stents, metallic splinters, nerve stimulators, cochlear implants, and pregnant women were precluded from the analysis.
Laboratory Analysis
Metabolic variables and parameters of pancreatic exocrine function were assessed immediately before surgery and 12 months after. The glycemic variables, lipids, and liver function tests were performed following 10-h overnight fasting. Photometric assessment of glucose, lipids, and liver enzymes were performed on a Siemens Dimension VISTA 1500 System (Siemens Healthcare Diagnostics, Eschborn, Germany). Pancreatic enzymes and high-sensitivity C-reactive protein (hs-CRP) levels were assessed as pancreatic and systemic inflammation indicators. For the quantitative measurements of insulin and C-peptide on the ADVIA Centauer XP (Siemens), a two-site immunoassay was employed according to the manufacturer's directives.
A standard 75 g OGTT was carried out to determine glucose-stimulated plasma levels of GLP-1, insulin, and C-peptide. Samples were gathered at 120 min with chilled EDTA tubes containing dipeptidyl-peptidase 4 (DPP4) inhibitor. Tubes were cooled on ice water for 15 min and were then centrifuged at 3,000 g and 4°C for 15 min. Plasma was separated into aliquots of 500 μl each and frozen at −80°C until analysis. GLP-1 concentrations in plasma were determined by radioimmunoassay after extraction of plasma with 70% ethanol. Carboxy-terminal GLP-1 immunoreactivity was measured using antiserum 89390, which has an absolute requirement for the intact amidated carboxy-terminus of GLP-1 7–36 amide and cross-reacts <0.01% with carboxy-terminally truncated fragments and 89% with GLP-1 9–36 amide, the primary metabolite of DPP-4-mediated degradation. The sum of the two components (total GLP-1 concentration) reflects the rate of secretion of the L cell. We measured total rather than active GLP-1 as total levels reflect secretion more efficiently than levels of active GLP-1 (17). Sensitivity was below 1 pmol/L, and intra-assay coefficient of variation below 5% (18).
Imaging Protocols
MRI of the abdomen was performed immediately before and 12 months post LSG, and no contrast media were given. Each subject was scanned once after a 10-h overnight fast in a 3 Tesla Ingenia scanner workstation (MR Systems Ingenia 3.0T, Philips Healthcare, Germany) in the supine position that first involved a set of localizer images. We employed a “Single Shot Fast Spin Echo,” which is a 3-plane localizer. Then, a respiratory-triggered T2–weighted 3D transverse fast spin-echo (TSE) sequence combined with a 2-point Dixon sequence was done. The pancreas was specified on each slice based on typical anatomical landmarks (19). Organ size was outlined by hand in each transaxial plane using OsiriX (OsiriX v.4.1, 32-bit, Pixmeo SARL), as shown in Figure 1.
For the measurement of pancreatic fat, 1–2 regions of interest (ROIs) of 0.3–0.4 cm2 in area were put in the head, body, and tail of the pancreas. The mean of the three selected ROIs of the pancreas was calculated to estimate the average fat fraction of the entire pancreas. Mean pixel signal intensity (SI) levels for each ROI were recorded. The fat fraction was calculated from the mean pixel SI data through the formula: Fat fraction FF (%) = [(SI in-phase – SI opposed-phase)/(2 × SI in-phase)] × 100 according to Rampton et al. (20). Pancreatic tissue fraction TF (%) was calculated as: 100% – FF (%).VAT areas of single axial slices (AVAT-1) centered at specific anatomic landmarks according to BMI and gender were calculated to predict total VAT volume (VVAT-T) as previously described (21, 22). A single experienced radiologist performed an estimation of pancreas and VAT volume.
Statistical Methods
Statistical analysis was done using IBM© SPSS© Statistics version 26 (IBM© Corp., Armonk, NY, USA). Numerical data were expressed as mean and standard deviation or median and range as appropriate. Qualitative data were expressed as frequency and percentage. Chi-square test (Fisher's exact test) was used to examine the relation between qualitative variables. For quantitative data, comparison of repeated measures was made using paired t-test. Pearson product-moment was used to estimate the correlation between numerical variables. A P-value < 0.05 was considered significant.
Results
All patients were available in the last follow-up visit after 12 months. The mean age of the studied group was 44.3 ± 7.0 years. They were 29 males and 25 females. Twelve patients (22.2%) had diabetes. Of them, eight were males. Mean duration of DM was 5 + 2.1 years. Of the diabetic patients, five were on insulin therapy, four on dual insulin and oral anti-diabetic drugs and three were on oral drugs only. Surgery resulted in a significant reduction in body weight and BMI (Table 1). The percentage of total weight loss (%TWL) after 12 months was 20.3 ± 4.2%.
HbA1c decreased by a median of 0.3% (range: −3.2 to 0.6%). Fasting insulin and C-peptide levels were significantly reduced after surgery (P < 0.001). Also, HOMA-IR decreased from 5.4 ± 0.8 to 2.8 ± 0.5 (P < 0.001). Seven patients showed complete remission of DM with stoppage of their medications and five showed partial remission. None of the partial remission group needed insulin therapy. Surgery also resulted in significant improvement in lipid profile except for HDL-cholesterol and liver function tests. Hs-CRP level as a parameter of chronic systemic inflammation decreased after surgery (P < 0.001). GLP-1 increased significantly after surgery.
Changes in Visceral Adipose Tissue and Pancreatic Volumes
Total VAT volume significantly decreased by a mean of 2,175 cm3 (95% CI: 1,832–2,518). The total pancreas volume decreased by a mean of 9.0 cm3 (95% CI: 6.6–11.3). The median change of pancreatic fat was −26.1% (range: −55.6 to 58.3%), while that of pancreatic parenchyma was −10% (range: −27.0 to 6.9%). The baseline pancreatic fat content was 26.8 ± 7.2%, it decreased to 20.0 ± 7.3% after surgery (Table 2). The mean change was 6.8% (95% CI: 5.1–8.5).
Changes in Pancreatic Enzymes
After surgery, there was a trivial non-significant change of pancreatic amylase (P = 0.472). Meanwhile, pancreatic lipase decreased significantly (P < 0.001).
Correlation Study
There was a positive correlation between %TWL and the decrease in pancreatic fat volume (r = 0.295, P = 0.030). Conversely, %TWL was negatively correlated with the change in pancreatic parenchymal volume (r = −0.278, P = 0.042). However, %TWL was not correlated with change of insulin, C-peptide or HOMA-IR (Table 3). C-peptide change was negatively correlated with pancreatic fat volume reduction and positively correlated with pancreatic parenchymal volume reduction. We did not find a correlation between the decrease in pancreatic fat volume and HOMA-IR drop after surgery (Table 3).
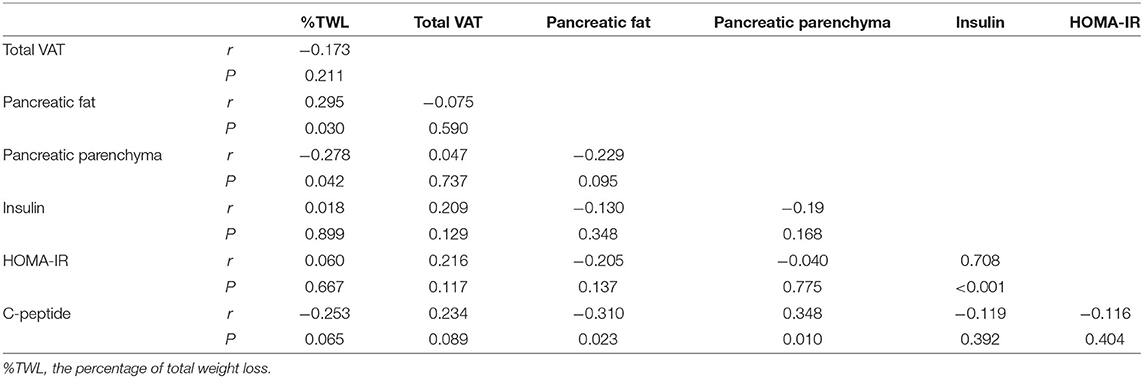
Table 3. Correlation between weight loss and changes of VAT and pancreatic volume and insulin resistance.
Discussion
This study demonstrated that weight loss after LSG was associated with a significant reduction of total pancreatic volume and pancreatic fat content with a concomitant decrease of total VAT volume. These changes were associated with significant improvement of glucose homeostasis, systemic inflammation reduction, and pancreatic lipase decline. %TWL after surgery was positively correlated with pancreatic fat volume reduction and negatively correlated with pancreatic parenchymal volume reduction. Pancreatic fat volume reduction was negatively correlated with C-peptide change but not with HOMA-IR decline after surgery.
In obese subjects, fat infiltration is commonly found in the exocrine pancreas and interlobular pancreatic spaces (23). When associated with obesity, pancreatic steatosis is called non-alcoholic fatty pancreas disease (NAFPD) (24). CT image analysis demonstrates a 10%−15% increase of parenchymal pancreas mass and about 70% increase of pancreatic fat mass in the presence of obesity (25, 26).
It is logical to expect a reduction of pancreatic volume after bariatric surgery in a similar analogy to its increase with obesity. The current study found a significant decrease in pancreatic volume that was more marked in fat content compared to the parenchyma. Pancreatic fat decreased by 26.1% (range: −55.6 to 58.3%), while parenchyma decreased by 10% (range: −27.0 to 6.9%). Few studies focused on the change in pancreatic volume after bariatric surgery. Honka et al. reported a significant decrease in pancreatic fat volume and fatty acid uptake 6 months after surgery. This change was associated with favorable glucose homeostasis and improved β-cell function. The authors did not observe a significant change in pancreatic parenchyma (27). Similarly, Umemura et al. reported a significant reduction of pancreatic volume (PV) 6 months after sleeve gastrectomy. The decreased PV correlated with decreased fasting blood sugar and insulin (28). Lautenbach et al. (29) found PV reduction reversal of pancreatic steatosis to normal levels in 11 patients 6 months after RYGB, which was associated with improvement of glucose homeostasis. However, the three studies involved small sample sizes besides evaluating pancreatic changes after only 6 months. To the best of our knowledge, the current study included the largest series to demonstrate the effect of bariatric surgery on pancreatic fat after a longer follow-up period of 1 year.
We demonstrated significant improvement of glucose homeostasis concomitantly with partial resolution of pancreatic steatosis. Fasting blood glucose, insulin, and C-peptide levels were reduced significantly after surgery. Also, HOMA-IR and HA1c decreased. Many studies found that increased pancreatic fat can lead to β-cell dysfunction and insulin resistance (30–32). However, other studies reported conflicting results about the association between pancreatic fat content and beta-cell function (33, 34). It has been shown that fatty pancreas is an independent risk factor for developing DM (35). The fat fraction in the pancreatic tail was shown to significantly predict individuals at risk for the T2DM group with a sensitivity of 45.5% and specificity of 81.3% (36).
Many findings indicate a negative impact of lipotoxicity due to pancreas fat on beta-cell function. A rodent study showed that fat accumulation in pancreatic islets was associated with beta-cell dysfunction and apoptosis (37). The excess fatty acid in the pancreatic islets worsens beta-cell function and insulin secretion (38). Also, leptin, TNF-α, and adipocytokines secreted from pancreas adipocytes may induce beta-cell damage (39).
However, findings about the effects of pancreatic fat on glucose metabolism are inconsistent [74]. Histological studies did not detect significant differences between diabetic and non-diabetic subjects in pancreatic fat area (40, 41). Other reports showed that increased pancreatic fat enhances the development of glucose intolerance (12, 33). A negative association between pancreatic fat and insulin secretion has been found in subjects with a high genetic risk for diabetes (33). Also, high pancreatic fat was associated with a higher risk of T2DM in non-obese subjects (42). Other studies did not find a relationship between pancreatic fat content and beta-cell function (41, 43, 44). These inconsistencies may be due to the heterogeneous distribution of pancreatic fat. Also, most pancreatic lipids are stored in adipocytes existing in the exocrine pancreas and, to a lesser extent, in the endocrine pancreas (45, 46).
In the current study, the initial pancreatic fat content was 26.8 ± 7.2%. Surgery resulted in a mean reduction of fat content by 6.8% (95% CI: 5.1–8.5%). Previous studies reported variable pancreatic fat content in obese and normal-weight individuals. Based on pooled data from various MR methods, a meta-analysis estimated a normal mean pancreatic fat of 4.5 ± 0.9% (16). However, there is no accurate consensus on the upper limit of normal pancreatic fat content. This meta-analysis suggested a cutoff of 6.2% to differentiate normal from high pancreatic fat content (16). In a large cohort of 3,000 normal-weight adults, Wong et al. (9) reported a fat content ranging from 1.8 to 10.4%. In another study, the pancreatic fat fraction was 4.9% in normal subjects compared to 6.2 and 8.6% in those with prediabetes and diabetes, respectively (47). In a study of 267 patients, ectopic pancreatic fat was evaluated by MRI. The average fat content in the pancreas was 5.98% in patients with normal BMI, 9.36% in overweight people, and 11.69% in obese patients (48). In a recent study, the fat fraction in obese individuals was 10.3 ± 5.1% (49). An old autopsy study revealed an average of 17.1% of pancreatic fat content in obese patients compared to 9.3% fat accumulation in non-obese patients (50).
Currently, there is a number of diagnostic methods for quantifying pancreatic fat with various feasibility and reproducibility. These include ultrasonography (51), endoscopic ultrasonography (52), MRI (32), CT (51), and proton magnetic resonance spectroscopy (1H-MRS) (53). The most developed method is multi-echo Dixon MRI (54). MRI is considered the most accurate approach for fat quantification (55). This is contributed to the dependence of MR signal on fat content. Besides, MRI avoids the observer variability of ultrasonography and the ionizing radiation of CT (56).
The present study is not without limitations. The presence of steatopancreatitis should be better evaluated in further studies, especially regarding the inflammatory cascade. Liver volume and correlation with pancreatic fat may be needed to be assessed as well and correlated to hepatic enzymes. Also, further studies may take into account more detailed characteristics of diabetic patients such as classifications by ABCD score or IMS score and changes of beta cell function (insulinogenic index, Matsuda index, disposition index).
Despite the existence of some limitations, the current work adds a momentum to the literature regrading this novel topic. Besides, among the strengths of this work is the reasonable number of cases with a quite acceptable follow-up period. Furthermore, this work may point to potential mechanisms that may be underlying the beneficial impact of metabolic procedures on the pancreas and may provide new horizons in terms of this aspect.
We can conclude that there is an association of weight loss after LSG with a reduction of total VAT volume, total pancreatic volume, and pancreatic fat content. Weight reduction is positively correlated with the decrease of pancreatic fat content, while it is negatively correlated with pancreatic parenchymal volume reduction. The changes in pancreatic fat content are associated with improved glucose homeostasis, reduced systemic inflammation, and decreased pancreatic lipase secretion.
Data Availability Statement
The raw data supporting the conclusions of this article will be made available by the authors, without undue reservation.
Ethics Statement
The studies involving human participants were reviewed and approved by Cairo University Hospitals. The patients/participants provided their written informed consent to participate in this study.
Author Contributions
All authors listed have made a substantial, direct, and intellectual contribution to the work and approved it for publication.
Conflict of Interest
The authors declare that the research was conducted in the absence of any commercial or financial relationships that could be construed as a potential conflict of interest.
Publisher's Note
All claims expressed in this article are solely those of the authors and do not necessarily represent those of their affiliated organizations, or those of the publisher, the editors and the reviewers. Any product that may be evaluated in this article, or claim that may be made by its manufacturer, is not guaranteed or endorsed by the publisher.
References
1. Goossens GH, Blaak EE. Adipose tissue dysfunction and impaired metabolic health in human obesity: a matter of oxygen? Front Endocrinol. (2015) 6:55. doi: 10.3389/fendo.2015.00055
2. Kim SM, Lun M, Wang M, Senyo SE, Guillermier C, Patwari P, et al. Loss of white adipose hyperplastic potential is associated with enhanced susceptibility to insulin resistance. Cell Metab. (2014) 20:1049–58. doi: 10.1016/j.cmet.2014.10.010
3. Guillermier C, Fazeli PK, Kim S, Lun M, Zuflacht JP, Milian J, et al. Imaging mass spectrometry demonstrates age-related decline in human adipose plasticity. JCI Insight. (2017) 2:e90349. doi: 10.1172/jci.insight.90349
4. Elffers TW, de Mutsert R, Lamb HJ, de Roos A, Willems van Dijk K, Rosendaal FR, et al. Body fat distribution, in particular visceral fat, is associated with cardiometabolic risk factors in obese women. PloS ONE. (2017) 12:e0185403. doi: 10.1371/journal.pone.0185403
5. Sato F, Maeda N, Yamada T, Namazui H, Fukuda S, Natsukawa T, et al. Association of epicardial, visceral, and subcutaneous fat with cardiometabolic diseases. Circ J. (2018) 82:502–8. doi: 10.1253/circj.CJ-17-0820
6. Coulier B. Pancreatic lipomatosis: an extensive pictorial review. J Belg Soc Radiol. (2016) 100:39. doi: 10.5334/jbr-btr.1014
7. Uygun A, Kadayifci A, Demirci H, Saglam M, Sakin YS, Ozturk K, et al. The effect of fatty pancreas on serum glucose parameters in patients with nonalcoholic steatohepatitis. Eur J Intern Med. (2015) 26:37–41. doi: 10.1016/j.ejim.2014.11.007
8. Pinte L, Balaban DV, Băicuş C, Jinga M. Non-alcoholic fatty pancreas disease – practices for clinicians. Rom J Intern Med. (2019) 57:209–19. doi: 10.2478/rjim-2019-0005
9. Wong VW-S, Wong GL-H, Yeung DK-W, Abrigo JM, Kong AP-S, Chan RS-M, et al. Fatty pancreas, insulin resistance, and β-cell function: a population study using fat-water magnetic resonance imaging. Am J Gastroenterol. (2014) 109:589–97. doi: 10.1038/ajg.2014.1
10. Steven S, Hollingsworth KG, Small PK, Woodcock SA, Pucci A, Aribisala B, et al. Weight loss decreases excess pancreatic triacylglycerol specifically in type 2 diabetes. Diabetes Care. (2016) 39:158–65. doi: 10.2337/dc15-0750
11. Noel P, Patel K, Durgampudi C, Trivedi RN, de Oliveira C, Crowell MD, et al. Peripancreatic fat necrosis worsens acute pancreatitis independent of pancreatic necrosis via unsaturated fatty acids increased in human pancreatic necrosis collections. Gut. (2016) 65:100–11. doi: 10.1136/gutjnl-2014-308043
12. Angrisani L, Santonicola A, Iovino P, Formisano G, Buchwald H, Scopinaro N. Bariatric surgery worldwide 2013. Obes Surg. (2015) 25:1822–32. doi: 10.1007/s11695-015-1657-z
13. Galanakis CG, Daskalakis M, Manios A, Xyda A, Karantanas AH, Melissas J. Computed tomography-based assessment of abdominal adiposity changes and their impact on metabolic alterations following bariatric surgery. World J Surg. (2015) 39:417–23. doi: 10.1007/s00268-014-2826-2
14. Toro-Ramos T, Goodpaster BH, Janumala I, Lin S, Strain GW, Thornton JC, et al. Continued loss in visceral and intermuscular adipose tissue in weight-stable women following bariatric surgery. Obesity. (2015) 23:62–9. doi: 10.1002/oby.20932
15. Gaborit B, Abdesselam I, Kober F, Jacquier A, Ronsin O, Emungania O, et al. Ectopic fat storage in the pancreas using 1H-MRS: importance of diabetic status and modulation with bariatric surgery-induced weight loss. Int J Obes. (2015) 39:480–7. doi: 10.1038/ijo.2014.126
16. Singh RG, Yoon HD, Wu LM, Lu J, Plank LD, Petrov MS. Ectopic fat accumulation in the pancreas and its clinical relevance: a systematic review, meta-analysis, and meta-regression. Metabolism. (2017) 69:1–13. doi: 10.1016/j.metabol.2016.12.012
17. Wewer Albrechtsen NJ, Bak MJ, Hartmann B, Christensen LW, Kuhre RE, Deacon CF, et al. Stability of glucagon-like peptide 1 and glucagon in human plasma. Endocr Connect. (2015) 4:50–7. doi: 10.1530/EC-14-0126
18. Orskov C, Rabenhøj L, Wettergren A, Kofod H, Holst JJ. Tissue and plasma concentrations of amidated and glycine-extended glucagon-like peptide I in humans. Diabetes. (1994) 43:535–9. doi: 10.2337/diab.43.4.535
19. Geraghty EM, Boone JM, McGahan JP, Jain K. Normal organ volume assessment from abdominal CT. Abdom Imaging. (2004) 29:482–90. doi: 10.1007/s00261-003-0139-2
20. Rampton JW, Young PM, Fidler JL, Hartman RP, Herfkens RJ. Putting the fat and water protons to work for you: a demonstration through clinical cases of how fat-water separation techniques can benefit your body MRI practice. AJR Am J Roentgenol. (2013) 201:1303–8. doi: 10.2214/AJR.13.10606
21. Linder N, Schaudinn A, Garnov N, Blüher M, Dietrich A, Schütz T, et al. Age and gender specific estimation of visceral adipose tissue amounts from radiological images in morbidly obese patients. Sci Rep. (2016) 6:22261. doi: 10.1038/srep22261
22. Schaudinn A, Linder N, Garnov N, Kerlikowsky F, Blüher M, Dietrich A, et al. Predictive accuracy of single- and multi-slice MRI for the estimation of total visceral adipose tissue in overweight to severely obese patients. NMR Biomed. (2015) 28:583–90. doi: 10.1002/nbm.3286
23. Saisho Y, Butler AE, Manesso E, Elashoff D, Rizza RA, Butler PC. β-cell mass and turnover in humans: effects of obesity and aging. Diabetes Care. (2013) 36:111–7. doi: 10.2337/dc12-0421
24. Paul J, Shihaz AVH. Pancreatic steatosis: a new diagnosis and therapeutic challenge in gastroenterology. Arq Gastroenterol. (2020) 57:216–20. doi: 10.1590/s0004-2803.202000000-27
25. Saisho Y. Pancreas volume with obesity in Asians: comparison with whites. Pancreas. (2014) 43:657–9. doi: 10.1097/MPA.0000000000000064
26. Kou K, Saisho Y, Jinzaki M, Itoh H. Relationship between body mass index and pancreas volume in Japanese people. JOP J Pancreas. (2014) 15:626–7. doi: 10.6092/1590-8577/2858
27. Honka H, Koffert J, Hannukainen JC, Tuulari JJ, Karlsson HK, Immonen H, et al. The effects of bariatric surgery on pancreatic lipid metabolism and blood flow. J Clin Endocrinol Metab. (2015) 100:2015–23. doi: 10.1210/jc.2014-4236
28. Umemura A, Sasaki A, Nitta H, Baba S, Ando T, Kajiwara T, et al. Pancreas volume reduction and metabolic effects in Japanese patients with severe obesity following laparoscopic sleeve gastrectomy. Endocr J. (2017) 64:487–98. doi: 10.1507/endocrj.EJ16-0321
29. Lautenbach A, Wernecke M, Riedel N, Veigel J, Yamamura J, Keller S, et al. Adaptive changes in pancreas post Roux-en-Y gastric bypass induced weight loss. Diabetes Metab Res Rev. (2018) 34:e3025. doi: 10.1002/dmrr.3025
30. Szczepaniak LS, Victor RG, Mathur R, Nelson MD, Szczepaniak EW, Tyer N, et al. Pancreatic steatosis and its relationship to β-cell dysfunction in humans: racial and ethnic variations. Diabetes Care. (2012) 35:2377–83. doi: 10.2337/dc12-0701
31. Ou H-Y, Wang C-Y, Yang Y-C, Chen M-F, Chang C-J. The association between nonalcoholic fatty pancreas disease and diabetes. PLoS ONE. (2013) 8:e62561. doi: 10.1371/journal.pone.0062561
32. Heni M, Machann J, Staiger H, Schwenzer NF, Peter A, Schick F, et al. Pancreatic fat is negatively associated with insulin secretion in individuals with impaired fasting glucose and/or impaired glucose tolerance: a nuclear magnetic resonance study. Diabetes Metab Res Rev. (2010) 26:200–5. doi: 10.1002/dmrr.1073
33. Begovatz P, Koliaki C, Weber K, Strassburger K, Nowotny B, Nowotny P, et al. Pancreatic adipose tissue infiltration, parenchymal steatosis and beta cell function in humans. Diabetologia. (2015) 58:1646–55. doi: 10.1007/s00125-015-3544-5
34. Li Y, Sang Y, Sun Y, Liu X, Geng H, Zha M, et al. Pancreatic fat is not significantly correlated with β-cell dysfunction in patients with new-onset type 2 diabetes mellitus using quantitative computed tomography. Int J Med Sci. (2020) 17:1673–82. doi: 10.7150/ijms.46395
35. Chan TT, Tse YK, Lui RN-S, Wong GL-H, Chim AM-L, Kong AP-S, et al. Fatty pancreas is independently associated with subsequent diabetes mellitus development: a 10-year prospective cohort study. Clin Gastroenterol Hepatol. (2021) doi: 10.1016/j.cgh.2021.09.027
36. Nadarajah C, Fananapazir G, Cui E, Gichoya J, Thayalan N, Asare-Sawiri M, et al. Association of pancreatic fat content with type II diabetes mellitus. Clin Radiol. (2020) 75:51–6. doi: 10.1016/j.crad.2019.05.027
37. Lee Y, Lingvay I, Szczepaniak LS, Ravazzola M, Orci L, Unger RH. Pancreatic steatosis: harbinger of type 2 diabetes in obese rodents. Int J Obes. (2010) 34:396–400. doi: 10.1038/ijo.2009.245
38. Cnop M. Fatty acids and glucolipotoxicity in the pathogenesis of type 2 diabetes. Biochem Soc Trans. (2008) 36:348–52. doi: 10.1042/BST0360348
39. Saisho Y. Pancreas volume and fat deposition in diabetes and normal physiology: consideration of the interplay between endocrine and exocrine pancreas. Rev Diabet Stud RDS. (2016) 13:132–47. doi: 10.1900/RDS.2016.13.132
40. Horii T, Fujita Y, Ishibashi C, Fukui K, Eguchi H, Kozawa J, et al. Islet inflammation is associated with pancreatic fatty infiltration and hyperglycemia in type 2 diabetes. BMJ Open Diabetes Res Care. (2020) 8:e001508. doi: 10.1136/bmjdrc-2020-001508
41. Saisho Y, Butler AE, Meier JJ, Monchamp T, Allen-Auerbach M, Rizza RA, et al. Pancreas volumes in humans from birth to age one hundred taking into account sex, obesity, and presence of type-2 diabetes. Clin Anat. (2007) 20:933–42. doi: 10.1002/ca.20543
42. Yamazaki H, Tauchi S, Wang J, Dohke M, Hanawa N, Kodama Y, et al. Longitudinal association of fatty pancreas with the incidence of type-2 diabetes in lean individuals: a 6-year computed tomography-based cohort study. J Gastroenterol. (2020) 55:712–21. doi: 10.1007/s00535-020-01683-x
43. Kühn J-P, Berthold F, Mayerle J, Völzke H, Reeder SB, Rathmann W, et al. Pancreatic steatosis demonstrated at MR imaging in the general population: clinical relevance. Radiology. (2015) 276:129–36. doi: 10.1148/radiol.15140446
44. Yamazaki H, Tsuboya T, Katanuma A, Kodama Y, Tauchi S, Dohke M, et al. Lack of Independent association between fatty pancreas and incidence of type 2 diabetes: 5-year Japanese Cohort Study. Diabetes Care. (2016) 39:1677–83. doi: 10.2337/dc16-0074
45. Wagner R, Jaghutriz BA, Gerst F, Barroso Oquendo M, Machann J, Schick F, et al. Pancreatic steatosis associates with impaired insulin secretion in genetically predisposed individuals. J Clin Endocrinol Metab. (2020) 105:dgaa435. doi: 10.1210/clinem/dgaa435
46. Gerst F, Wagner R, Kaiser G, Panse M, Heni M, Machann J, et al. Metabolic crosstalk between fatty pancreas and fatty liver: effects on local inflammation and insulin secretion. Diabetologia. (2017) 60:2240–51. doi: 10.1007/s00125-017-4385-1
47. Heber SD, Hetterich H, Lorbeer R, Bayerl C, Machann J, Auweter S, et al. Pancreatic fat content by magnetic resonance imaging in subjects with prediabetes, diabetes, and controls from a general population without cardiovascular disease. PLoS ONE. (2017) 12:e0177154. doi: 10.1371/journal.pone.0177154
48. Pieńkowska J, Brzeska B, Kaszubowski M, Kozak O, Jankowska A, Szurowska E, et al. MRI assessment of ectopic fat accumulation in pancreas, liver and skeletal muscle in patients with obesity, overweight and normal BMI in correlation with the presence of central obesity and metabolic syndrome. Diabetes Metab Syndr Obes. (2019) 12:623–36. doi: 10.2147/DMSO.S194690
49. Jiang Y, Spurny M, Schübel R, Nonnenmacher T, Schlett CL, von Stackelberg O, et al. Changes in pancreatic fat content following diet-induced weight loss. Nutrients. (2019) 11:912. doi: 10.3390/nu11040912
50. Ogilvie RF. The islands of langerhans in 19 cases of obesity. J Pathol Bacteriol. (1933) 37:473–81. doi: 10.1002/path.1700370314
51. Lee JS, Kim SH, Jun DW, Han JH, Jang EC, Park JY, et al. Clinical implications of fatty pancreas: correlations between fatty pancreas and metabolic syndrome. World J Gastroenterol. (2009) 15:1869–75. doi: 10.3748/wjg.15.1869
52. Al-Haddad M, Khashab M, Zyromski N, Pungpapong S, Wallace MB, Scolapio J, et al. Risk factors for hyperechogenic pancreas on endoscopic ultrasound: a case-control study. Pancreas. (2009) 38:672–5. doi: 10.1097/MPA.0b013e3181a9d5af
53. van der Zijl NJ, Goossens GH, Moors CCM, van Raalte DH, Muskiet MHA, Pouwels PJW, et al. Ectopic fat storage in the pancreas, liver, and abdominal fat depots: impact on β-cell function in individuals with impaired glucose metabolism. J Clin Endocrinol Metab. (2011) 96:459–67. doi: 10.1210/jc.2010-1722
54. Wagner R, Eckstein SS, Yamazaki H, Gerst F, Machann J, Jaghutriz BA, et al. Metabolic implications of pancreatic fat accumulation. Nat Rev Endocrinol. (2022) 18:43–54. doi: 10.1038/s41574-021-00573-3
55. Schwenzer NF, Machann J, Martirosian P, Stefan N, Schraml C, Fritsche A, et al. Quantification of pancreatic lipomatosis and liver steatosis by MRI: comparison of in/opposed-phase and spectral-spatial excitation techniques. Invest Radiol. (2008) 43:330–7. doi: 10.1097/RLI.0b013e31816a88c6
Keywords: metabolic surgery, pancreas, steatosis, insulin, resistance
Citation: Salman AA, Salman MA, Said M, El Sherbiny M, Elkassar H, Hassan MB, Marwan A, Morad MA, Ashoush O, Labib S, Aon MH, Awad A, Sayed M, Taha AE, Moustafa A, Shaaban HE-D, Khater A, Elewa A, Khalaf AM, Mostafa AA, Matter M and Youssef A (2022) Improvement of Pancreatic Steatosis and Indices of Insulin Resistance After Metabolic Surgery. Front. Med. 9:894465. doi: 10.3389/fmed.2022.894465
Received: 11 March 2022; Accepted: 27 April 2022;
Published: 06 June 2022.
Edited by:
Yanyong Yang, Second Military Medical University, ChinaReviewed by:
Metin Basaranoglu, Bezmiâlem Vakif Üniversitesi, TurkeyAkira Umemura, Iwate Medical University, Japan
Copyright © 2022 Salman, Salman, Said, El Sherbiny, Elkassar, Hassan, Marwan, Morad, Ashoush, Labib, Aon, Awad, Sayed, Taha, Moustafa, Shaaban, Khater, Elewa, Khalaf, Mostafa, Matter and Youssef. This is an open-access article distributed under the terms of the Creative Commons Attribution License (CC BY). The use, distribution or reproduction in other forums is permitted, provided the original author(s) and the copyright owner(s) are credited and that the original publication in this journal is cited, in accordance with accepted academic practice. No use, distribution or reproduction is permitted which does not comply with these terms.
*Correspondence: Ahmed Abdallah Salman, YXdlYTg0QGthc3JhbGFpbnkuZWR1LmVn
†ORCID: Ahmed Abdallah Salman orcid.org/0000-0003-0026-0841
Mohamed Abdalla Salman orcid.org/0000-0001-5445-6415
Mohamed H. Aon orcid.org/0000-0001-9109-1697