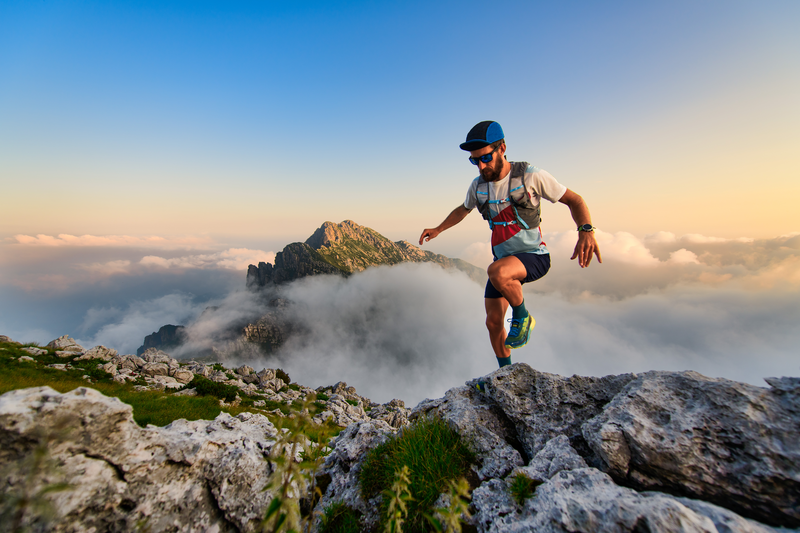
94% of researchers rate our articles as excellent or good
Learn more about the work of our research integrity team to safeguard the quality of each article we publish.
Find out more
MINI REVIEW article
Front. Med. , 11 March 2022
Sec. Infectious Diseases – Surveillance, Prevention and Treatment
Volume 9 - 2022 | https://doi.org/10.3389/fmed.2022.867147
This article is part of the Research Topic Prevention and Control of Human T Lymphotropic Viruses 1 and 2 (HTLV-1/2) View all 32 articles
Breastfeeding is recommended by the World Health Organization for at least 6 months up to 2 years of age, and breast milk protects against several diseases and infections. Intriguingly, few viruses are transmitted via breastfeeding including Human T-cell leukemia virus Type 1 (HTLV-1). HTLV-1 is a highly oncogenic yet neglected retrovirus, which primarily infects CD4+ T-cells in vivo and causes incurable diseases like HTLV-1-associated inflammatory conditions or Adult T-cell leukemia/lymphoma (ATLL) after lifelong viral persistence. Worldwide, at least 5–10 million people are HTLV-1-infected and most of them are unaware of their infection posing the risk of silent transmissions. HTLV-1 is transmitted via cell-containing body fluids such as blood products, semen, and breast milk, which constitutes the major route of mother-to-child transmission (MTCT). Risk of transmission increases with the duration of breastfeeding, however, abstinence from breastfeeding as it is recommended in some endemic countries is not an option in resource-limited settings or underrepresented areas and populations. Despite significant progress in understanding details of HTLV-1 cell-to-cell transmission, it is still not fully understood, which cells in which organs get infected via the oral route, how these cells get infected, how breast milk affects this route of infection and how to inhibit oral transmission despite breastfeeding, which is an urgent need especially in underrepresented areas of the world. Here, we review these questions and provide an outlook how future research could help to uncover prevention strategies that might ultimately allow infants to benefit from breastfeeding while reducing the risk of HTLV-1 transmission.
Human T-cell leukemia virus type 1 (HTLV-1) is a neglected highly oncogenic retrovirus infecting at least 5–10 million people worldwide, which is probably an underestimation (1). Endemic regions are located in Japan, Melanesia, Central Australia, Sub-Saharan Africa, parts of South America (e.g., Brazil), the Caribbean, and the Middle East (2). A high frequency of people infected with HTLV-1 (PHTLV) worldwide lives in resource-limited settings or belongs to social or ethnic minorities (2, 3). Problematically, many of the PHTLV are unaware of their infection since HTLV-1 is not screened routinely in every endemic country (1). Therefore, PHTLV might pass the virus to other vulnerable groups via blood products, sexual transmission and mother-to-child transmission (MTCT). Upon infection, HTLV-1 integrates into the host cell genome and PHTLV are life-long suffering from the burden of HTLV-1-infection and an increasingly recognized impairment of quality of life (4). Carriers face the risk of developing diseases with high morbidity and mortality, especially if infection has been acquired during infancy and due to the high incidence of co-infections (5). Approximately 10% of PHTLV develop incurable diseases including HTLV-1-associated inflammatory conditions like HTLV-1-associated myelopathy/tropical spastic paraparesis (HAM/TSP), or the fatal neoplasia Adult T-cell leukemia/lymphoma (ATLL). Infection upon MTCT poses an exceptional high risk for the infants to develop ATLL during their lives (6). In endemic areas of HTLV-1-infection, such as southwestern Japan, MTCT has been demonstrated to be the primary mode of transmission (7), while the main route of transmission for other countries like Brazil (ca. 0.8–2.5 million PHTLV) or Central Australia is still a matter of discussion (3, 8, 9). Although intra-uterine transmission of HTLV-1 has been described and perinatal transmission cannot be fully excluded (10, 11), the majority of HTLV-1 MTCT occurs via breastfeeding since the level of infection among babies that are exclusively formula fed is low (6, 7). In breastfed infants, MTCT occurs at rates varying from 7.4 to 32%, compared with a rate of less than 2.5–5% among bottle-fed children (6, 8, 12). Risk factors for HTLV-1 transmission via breastfeeding are (1) high proviral loads (PVL) in milk and blood, (2) low income, (3) breastfeeding over a longer period, (4) previous HTLV-1-infected offspring, (5) HLA-concordance between mother and child, (6) coinfection with the nematode Strongyloides sp., or (7) being a HAM/TSP patient (6, 13). Infection most likely results from the prolonged exposure of infants to HTLV-1 infected cells in breast milk after the loss of protective maternal antibodies (9, 14).
Overall, there is a dilemma whether the benefits from breastfeeding outweigh the risk of virus transmission (Figure 1). On the one hand, breastfeeding is recommended by WHO for the first 6 months up to 2 years of age since breast milk provides optimal nutrition to the infant and protects against severe diseases and infections, especially diarrheal infections attributed to contaminated drinking water (15). On the other hand, few viruses are transmitted via breast milk including Human Cytomegalovirus (CMV), Human Immunodeficiency Virus (HIV), HTLV-1, and the related HTLV-2 (16, 17). For arboviruses like Zika Virus, Dengue Virus, or Yellow Fever Virus, transmission via breast milk is under debate (18). However, abstinence from breastfeeding is no option in resource-limited settings due to impaired access to clean drinking water. In addition, social stigmatization of non-breastfeeding mothers, in certain cases, might serve as a hurdle to cease from breastfeeding, or impact adherence to the recommendation of avoidance of breastfeeding. Moreover, freezing of milk from HTLV-1-infected mothers has been shown to reduce risk of transmission in Japan (19), but this may not be feasible in developing countries. Therefore, this mini-review provides an overview of the oral route of HTLV-1 transmission via breastfeeding and an outlook how future research could help to uncover prevention strategies of MTCT, which proves to be one of WHO main priorities (12). These implementations should ultimately allow infants to benefit from breastfeeding while reducing the risk of HTLV-1 transmission.
Figure 1. The dilemma of breastfeeding and HTLV-1 transmission. HCMV, Human Cytomegalovirus; HIV, Human Immunodeficiency virus; HTLV, Human T-cell leukemia virus; WHO, World Health Organization. Created with BioRender.
Ingestion of breast milk constitutes the major pathway of HTLV-1 MTCT, and common marmosets, rabbits and rats can be experimentally infected with HTLV-1 via the oral route (20–23). Upon ingestion, breast milk first comes to pass the oral cavity of the infant. Due to their location, architecture and enrichment in lymphocytes, palatine tonsils represent the first possible viral entry site (Figure 2). Indeed, tonsils seem to constitute an HTLV-1 reservoir as proviral DNA could be detected in extrafollicular areas in tonsil sections from HTLV-1 seropositive patients (24). Several lines of evidence additionally suggest that labial salivary glands (LSG) represent a non-lymphoid viral reservoir since proviral DNA as well as HTLV-1 gene products have been detected in LSG tissues and acini cells of HAM/TSP patients and individuals presenting with Sjögren’s syndrome (25, 26). Moreover, in co-culture with the chronically infected T-cell line HCT-5, HTLV-1 Gag protein was rapidly transferred to salivary gland epithelial cells, indicating that this cell type might—at least in vitro—be susceptible to HTLV-1 infection (27). Yet, it remains to be experimentally determined whether these tissues are permissive for primary HTLV-1 infection, especially in the context of breast milk carrying infected cells.
Figure 2. The oral route of HTLV-1 transmission. HTLV-1 is transmitted from mother to child via breastfeeding. It remains to be determined (1) whether HTLV-1 or HTLV-1-infected cells cross epithelial barriers in the tonsils, the stomach, or the intestine of the infant in vivo, and (2) by which mechanisms the virus is transmitted in vivo. Tissues and cells displayed in this figure were drawn manually by Annika P. Schnell with Procreate® Version 5.2.4 (Hobart, Tasmania) on the Apple iPad Pro 11” 2020 (Cupertino, United States).
Having passed the suckling’s pharynx, subsequent potential HTLV-1 entry sites are located in the gut and small intestine (Figure 2). To this end, infected cells or infectious virus have to withstand the passage through the infant’s stomach. This idea is bolstered by experimental data indicating that medium pH = 3 had little influence on leukocyte survival as well as on HTLV-1 transmissibility from in vitro transformed breast milk macrophages to stimulated PBMC (28, 29). In fact, several animal models including rat, sheep and baboon, showed that colostral cells and milk leukocytes that were delivered to the newborn by lactation or oral application were able to pass the gastric epithelium and reach the neonate’s tissues and circulation (30–32).
After gastric transit, infected cells or infectious virus encounter the small intestine. Here, the gut-associated lymphoid tissue (GALT), a major secondary lymphatic organ, arises as an ideal candidate for potential HTLV-1 entry. Beneath the protective mucus film containing a multitude of antimicrobial peptides like defensins and lysozymes, the intestinal epithelial cell layer followed by the lamina propria is located (33). These structures contain many innate immune cells including macrophages and dendritic cells as well as intestinal intraepithelial lymphocytes (IEL) (34). Since most IELs at the surface mucosal compartment are partially activated T-cells it is conceivable that these cells are easily susceptible to HTLV-I infection (29). Importantly, the GALT contains accumulated lymphoid follicles clustering as Peyer’s patches (PP) in the ileum. Of note, in the murine system, maternal leukocytes that are transferred upon ingestion of breast milk preferentially localize to the offspring’s PPs (35). PPs are surrounded by the follicle-associated epithelium (FAE), which constitutes a junction between the GALT and the luminal microenvironment (36). The FAE is not only characterized by a large number of infiltrated B- and T-cells as well as macrophages and dendritic cells (DCs) but also encompasses specialized cells termed M-cells (microfold cells). Interestingly, M-cells were shown to serve as entry ports to the host for several viruses, including HIV-1, reo- and poliovirus in humans, as well as murine leukemia virus in mice (37–40). Therefore, M-cells might represent an attractive target also for HTLV-1 transmission, which remains to be addressed. General permissiveness of intestine tissues for HTLV-1 infection was demonstrated as the virus was detected in the mesenteric lymph nodes and the GALT after intravenous viral inoculation of squirrel monkeys or rabbits, respectively (41, 42).
Establishing an HTLV-1 infection via the oral route could occur in several ways, which has been nicely summarized in recent reviews (43, 44). First, HTLV-1 infected cells could readily pass a physically damaged or disrupted epithelial cell layer. Of note, in humans, intestinal permeability is very high shortly after birth but drops soon during the infant’s development (45). Since subsequent gut closure is a very gradual process this might serve as an entry point for infected cells. In fact, an in vitro study conducted by Afonso and colleagues showed that infection of cerebral endothelial cells mimicking the blood-brain-barrier led to barrier breakdown (46). On the other hand, using intestinal epithelial cell lines, no loss of epithelial cell layer integrity was observed (47), indicating cell type specific outcomes in different tissues. Interestingly, a recent study suggested that co-infection with HIV-1 and HCMV evoked a disruption of the mucosal layer, allowing viral spread to the tonsils using an in vitro model (48). Similarly, a report by Ohata and colleagues analyzing an HTLV-1/HCMV co-infected patient described a gastroenterocolitis setting, indicating that viral interplay might affect mucosal integrity (49). Interestingly, mixed feeding (powdered infant formula and breastfeeding) is associated with higher rates of HIV-1 transmission (50). This finding is probably due to altered gut mucosal lining and permeability as a result of an increased gut pH and altered microbiota, which has been observed in infants fed with formula milk or prematurely with solid foods (51–53). Therefore, it would be interesting to determine whether mixed feeding also impacts transmission of HTLV-1.
Apart from that, HTLV-1 infected macrophages, although not formally demonstrated yet, but which might be present in breast milk, could transmigrate through the intact epithelium. This mode of action was observed to be employed by HIV-1 using ex vivo organ tissue models, however, until now there is no experimental data in regard to HTLV-1 (54). Another plausible mechanism establishing HTLV-1 infection is productive infection of the epithelial cell layer. Indeed, an in vitro study observed HTLV-1 infection of enterocytes with newly generated virus being released from the basal surface (55). In contrast, Martin-Latil and colleagues could not observe productive infection in their epithelial cell line models but rather demonstrated that HTLV-1 crosses the epithelial barrier by transcytosis (47). Such transit of virions incorporated into vesicles from the apical-to-basal surface has been delineated also for HIV-1 in a monostratified epithelium in vitro model (56).
Irrespective of the mechanism, HTLV-1 is dependent on close cell-cell contacts to establish infection. A well-described specialized structure, which is formed after contact between an HTLV-1 infected and a target T-cell, is the virological synapse (VS) (57, 58). Adding to the model of virus transmission by close cell-cell contacts, in vitro data put forward the idea that clusters of virus containing components of the extracellular matrix (ECM) are transferred to target cells, so called viral biofilms (VB) (27, 59–61). However, it remains to be determined whether formation of the VS or of infectious VB occurs during the oral infection route. Moreover, it is unclear whether extracellular vesicles secreted from HTLV-1 infected cells, which enhance viral spread in vitro, also modulate primary infection via the oral route (62). Of special note, it was shown that subepithelial DCs were infected in a co-culture model after transcytosis of HTLV-1 through an intestinal epithelial cell layer (47). Plasmacytoid and myeloid DCs from the peripheral blood of HTLV-1 seropositive individuals can be infected by HTLV-1 and are able to transmit the virus to and productively infect CD4+ target T-cells (63, 64). This mode of action was demonstrated to occur not only after cis infection of DCs but also as an in trans model with HTLV-1 virions binding to DCs followed by transfer of the virus to target T-cells prior to infection of the DCs themselves (64, 65). Moreover, plasmacytoid DCs from uninfected people are susceptible to infection, can be experimentally infected with viral biofilm and pass the infection to T lymphocytes in vitro (60). These findings altogether propose DCs to be key player in primary infection of HTLV-1 permissive tissues as well as in activation of innate immunity (66). Still, the role of DCs in the context of HTLV-1 transmission by breast milk remains elusive and needs to be experimentally investigated.
Breast milk constitutes of water, probiotic bacteria, breast milk cells, and macro- and micronutrients including lipids, fats, proteins and carbohydrates. The repertoire of breast milk cells is highly heterogeneous and contains (1) breast-derived cells including luminal epithelial cells (LEC; ductal non-secretory epithelial cells and lactocytes), myoepithelial cells from the ducts, squamous epithelial cells (from skin and nipple of the breast), progenitor and stem cells, and (2) blood-derived cells including immune cells (e.g., macrophages, lymphocytes, and neutrophils), and hematopoietic progenitor and stem cells (67–69). Moreover, breast milk is also rich in biologically active components like growth hormones, immunoglobulins, microbiota, and human milk oligosaccharides (HMOs), the latter representing important nutrients in human milk, which also function in direct pathogen binding and as prebiotics during the establishment of the infant’s microbiome. Interestingly, HMOs have also antiviral properties, e.g., against HIV, rotavirus, norovirus, influenza, and respiratory syncytial virus (68, 70, 71).
In breast milk, cell free HTLV-1 virions have not been detected yet, but HTLV-1-infected mononuclear cells (72, 73). There are large numbers of macrophages present in early lactation that decrease with the maturation of the milk (68). In experimentally infected breast milk macrophages (BMM), integrated provirus as well as Gag p24 (intracellular) and Gag p19 (secreted) were detectable. Immortalized BMM retained their phagocytic activity and were able to transmit HTLV-1 to T-cells (29). Next to lymphocytes and macrophages of human breast milk, also LEC can be experimentally infected with HTLV-1 and may pass the infection to other cells (43, 74). Interestingly, productively HTLV-1-infected LEC could be kept in continuous culture and synthesized an extensive extracellular matrix containing Collagen IV, which is also part of the viral biofilm (59, 61, 75). LEC were able to infect milk and intestinal epithelial cells as well as blood and milk leukocytes, and HTLV-1-infected LEC have been detected in breast biopsies of an ATLL patient with gynecomastia. Therefore, it is possible that LEC could play a role as an HTLV-1 reservoir in vivo (75, 76). Yet, it remains to be determined whether also breast milk lymphocytes are infected with HTLV-1. Recent studies from mothers vaccinated against SARS-CoV-2 revealed that breastmilk T-cells (BMTC) contain higher concentrations of antigen-experienced effector and central memory T-cells (77). This is in agreement with an earlier study in people with HIV infection, and it may be speculated that BMTC arise from a tissue-resident population in breast tissue rather than from the peripheral blood. Thus, breast biopsies of HTLV-1-infected mothers as well as a detailed characterization of the HTLV-1-infected cells in breast milk would broaden our understanding of the initial steps of MTCT.
Next to breast milk cells, other milk components may impact HTLV-1-transmission. Testing of paired blood and milk samples from HTLV-1-infected mothers using a newly developed anti-HTLV IgG capture assay showed the presence of anti-HTLV-1/2 IgG in milk in the same proportion as blood but in lower quantity and that PVL in milk correlates with blood (14). Anti-HTLV-antibodies may protect transmission in utero, but decline after birth, and levels are low in breast milk. Therefore, prolonged breastfeeding and decline of protective antibodies may finally increase the risk of HTLV-1-transmission over time (14). Several milk components have been shown to interfere with HTLV-1 transmission, amongst them the soluble milk protein lactoferrin, transforming growth factor beta (TGF-β), and prostaglandin E2 (PGE2), which enhance HTLV-1 transmission to cord blood lymphocytes and transactivate the HTLV-1 long terminal repeat promotor (78–80). PGE2 has also been shown to enhance Gag p19 secretion, depending on viral replication (80). Interestingly, lactoferrin gene expression can also be induced by the HTLV-1 Tax transactivator, even when Tax is extracellularly administered, suggesting the existence of a paracrine loop in the lactic compartment between infected cells and lactoferrin-expressing cells of the mammary epithelium (81). Analysis with recombinant vaccinia viruses expressing HTLV-1 Env suggested that lactoferrin diminishes fusogenic activity of Env, but this has not been verified with HTLV-1-infected cells (78). Yet, a systematic evaluation of pro-and antiviral factors in breast milk and their impact on HTLV-1 MTCT is lacking.
A prerequisite for installing prevention strategies of HTLV-1 MTCT is to raise public awareness about virus transmission in endemic regions and, especially, to gain more knowledge about HTLV-1 prevalence in pregnant women worldwide. Still, there is a lack of cost-effective implementation strategies targeting HTLV-1 transmission in public health programs of many different realities around the world. An important step would be antenatal screening programs of pregnant women and education or counseling campaigns of seropositive mothers about the potential risks of viral transmission by breastfeeding, which has only been implemented for few endemic regions of HTLV-1-infection yet (6, 8). In fact, several studies showed that antenatal screening programs would not only prevent MTCT events but would even be financially beneficial to national health authorities (82, 83).
Nationwide antenatal screening programs have been installed in Japan in 2010 (84, 85), while in France, Brazil and Chile, people with certain risk factors or from endemic regions are screened (86). In addition, screening of breast milk donations is recommended in the United Kingdom and in France, at least for donors from endemic regions (12). Routine screening of milk banks originating from donors of endemic regions should be advisable. Brazil has been recommending HTLV-1/2 infected mothers to avoid breastfeeding in 2019 (3), a strategy that proved successful in Japan earlier: Abstinence from breastfeeding by PHTLV mothers reduced the prevalence of HTLV carriers from 20–25% to 4% in the Nagasaki population in Japan (8). In Japan, HTLV-1 infected mothers are advised not to breastfeed or to do so only for less than 3 months (7). These recommendations are based on studies showing that avoidance of breastfeeding and exclusive formula feeding, short-term breastfeeding up to 3–6 months, or, in few studies, the freeze-thaw method reduce MTCT (19, 87, 88).
However, these practices may not be feasible in any setting: avoidance of breastfeeding may not only be harmful to the infant due to the loss of maternal passive immunity but also due to the risk of infection-related infant mortality, e.g., as a result of limited access to clean water, or limited financial resources (89, 90). Several studies have described severe diarrheas and weight loss upon weaning (91). Next to economic problems, social stigmatization in some cultures when mothers do not breastfeed their children should not be ignored (92). On the other hand, seropositive mothers, who are aware of their infection, might be hampered to breastfeed due to their fear of transmitting the virus to their offspring or even feel guilty upon inadvertent transmission due to the lack of efficient education and MTCT prevention strategies. Taking into account the different realities around the world, it should be acknowledged that every recommendation favoring or possible obstacle precluding breastfeeding should be carefully weighed against each other. It is important to asses a seropositive mother’s particular risk of MTCT considering individual settings. Ideally, future prevention strategies should focus on inhibition of HTLV-1 MTCT despite breastfeeding to allow optimal nutrition of the infants.
But how could this be achieved? Prevention strategies could target either the infected mother or the newly infected infant. For HIV, WHO guidelines on HIV and Infant Feeding recommend “Mothers living with HIV should breastfeed for at least 12 months and may continue breastfeeding for up to 24 months or longer while being fully supported for antiretroviral therapy (ART) adherence” (93). In fact, this contrasts with recommendations provided in several high-income countries, including the United States, which advise complete cessation from breastfeeding to mothers living with HIV regardless of viral load and ART therapy (94, 95). However, unlike HIV, HTLV-1 predominantly expands by mitotic division of the infected cells rather than by new infections, thus, it remains to be determined whether ART could interfere with HTLV MTCT. Currently, clinical studies addressing the impact of ART on the PVL in breast milk of HTLV-1-infected mothers or, vice versa, assessing the use of prophylactic treatment of the suckling, using for example integrase inhibitors, are lacking but might serve as a promising and cost-effective tool to prevent MTCT. Future challenging strategies to get rid of the integrated virus within infected mothers could include excision strategies using HTLV-1-specific recombinases that have already been evolved for HIV (96, 97), zinc finger nucleases (98) or genetic editing strategies using CRISPR/Cas 9 (99). Hence, it may be easier to prevent de novo infection of the infant. From a practical point of view, mothers being aware of their infection should be equipped with easy-to-use, cheap, heat-resistant prevention tools, which can be applied while breastfeeding. These prevention tools do not exist yet and have to be developed. A specific tool to block HTLV-1 MTCT could be based on the anti-gp46 (env) neutralizing monoclonal antibody (mAb) termed LAT-27, which prevents in vivo transmission of HTLV-1 in a simple humanized mouse model of HTLV-1 infection (100). Of note, LAT-27 was not able to prevent MTCT in an orally HTLV-1 infected rat model when injected intraperitoneally, indicating that the mode of application might be decisive to inhibit MTCT (101). It remains to be determined whether MTCT could be prevented by neutralizing HTLV-1 specific antibodies in humans, which is an effective strategy to inhibit Hepatitis B Virus (HBV) transmission using Hepatitis B immune globulin (HBIG) (102). Moreover, it may also be interesting to see whether these antibodies can be applied as a spray into the oral cavity while suckling. Moreover, little is known about therapeutics that prevent entry of the infected cell, e.g., by interfering with the HTLV-1-receptor, the virus, or the infected cells, and which could ideally be applied topically on the breast of the infected mother or within the oral cavity of the suckling infant.
Overall, there is a great need to develop novel prevention strategies of HTLV MTCT with the potential to protect the most vulnerable members of our society, newborn infants, from live-long, live-threatening, and incurable infections.
SM and AT-K wrote the article. Both authors contributed to the article and approved the submitted version.
AT-K’s research was funded by the Federal Ministry of Education and Research (BMBF) of the Federal Republic of Germany (Milk-TV, 01KI2023), by Deutsche Forschungsgemeinschaft (DFG), GRK2504, project number 401821119, subprojects A2, by the DFG grant TH2166/1-1, and by the Interdisciplinary Center for Clinical Research (IZKF) at the Medical Faculty of FAU Erlangen-Nürnberg (Project A91).
The authors declare that the research was conducted in the absence of any commercial or financial relationships that could be construed as a potential conflict of interest.
All claims expressed in this article are solely those of the authors and do not necessarily represent those of their affiliated organizations, or those of the publisher, the editors and the reviewers. Any product that may be evaluated in this article, or claim that may be made by its manufacturer, is not guaranteed or endorsed by the publisher.
We thank Annika P. Schnell for illustration of Figure 2. Stefanie Heym is greatly acknowledged for critical reading of the manuscript.
1. Martin F, Tagaya Y, Gallo R. Time to eradicate HTLV-1: an open letter to WHO. Lancet. (2018) 391:1893–4. doi: 10.1016/S0140-6736(18)30974-7
2. Gessain A, Cassar O. Epidemiological aspects and world distribution of HTLV-1 infection. Front Microbiol. (2012) 3:388. doi: 10.3389/fmicb.2012.00388
3. Rosadas C, Menezes MLB, Galvao-Castro B, Assone T, Miranda AE, Aragon MG, et al. Blocking HTLV-1/2 silent transmission in Brazil: current public health policies and proposal for additional strategies. PLoS Negl Trop Dis. (2021) 15:e0009717. doi: 10.1371/journal.pntd.0009717
4. Rosadas C, Assone T, Yamashita M, Adonis A, Puccioni-Sohler M, Santos M, et al. Health state utility values in people living with HTLV-1 and in patients with HAM/TSP: the impact of a neglected disease on the quality of life. PLoS Negl Trop Dis. (2020) 14:e0008761. doi: 10.1371/journal.pntd.0008761.,
5. Rosadas C, Taylor GP. HTLV-1 and co-infections. Front Med. (2022) 9:812016. doi: 10.3389/fmed.2022.812016
6. Rosadas C, Taylor GP. Mother-to-child HTLV-1 transmission: unmet research needs. Front Microbiol. (2019) 10:999. doi: 10.3389/fmicb.2019.00999
7. Hino S. Establishment of the milk-borne transmission as a key factor for the peculiar endemicity of human T-lymphotropic virus type 1 (HTLV-1): the ATL prevention program Nagasaki. Proc Jpn Acad Ser B Phys Biol Sci. (2011) 87:152–66. doi: 10.2183/pjab.87.152
8. Carneiro-Proietti AB, Amaranto-Damasio MS, Leal-Horiguchi CF, Bastos RH, Seabra-Freitas G, Borowiak DR, et al. Mother-to-child transmission of human T-cell lymphotropic viruses-1/2: what we know, and what are the gaps in understanding and preventing this route of infection. J Pediatric Infect Dis Soc. (2014) 3(Suppl. 1):S24–9. doi: 10.1093/jpids/piu070
9. Einsiedel L, Woodman RJ, Flynn M, Wilson K, Cassar O, Gessain A. Human T-lymphotropic virus type 1 infection in an indigenous australian population: epidemiological insights from a hospital-based cohort study. BMC Public Health. (2016) 16:787. doi: 10.1186/s12889-016-3366-5
10. Fujino T, Fujiyoshi T, Yashiki S, Sonoda S, Otsuka H, Nagata Y. HTLV-I transmission from mother to fetus via placenta. Lancet. (1992) 340:1157. doi: 10.1016/0140-6736(92)93181-l
11. Tezuka K, Fuchi N, Okuma K, Tsukiyama T, Miura S, Hasegawa Y, et al. HTLV-1 targets human placental trophoblasts in seropositive pregnant women. J Clin Invest. (2020) 130:6171–86. doi: 10.1172/JCI135525
12. WHO. Human T-Lymphotropic Virus Type 1: Technical Report. [Internet]. (2021). Available online at: https://www.who.int/publications/i/item/9789240020221 (accessed January 26, 2022).
13. Paiva AM, Assone T, Haziot MEJ, Smid J, Fonseca LAM, Luiz ODC, et al. Risk factors associated with HTLV-1 vertical transmission in Brazil: longer breastfeeding, higher maternal proviral load and previous HTLV-1-infected offspring. Sci Rep. (2018) 8:7742. doi: 10.1038/s41598-018-25939-y
14. Rosadas C, Woo T, Haddow J, Rowan A, Taylor GP. Anti-HTLV-1/2 igg antibodies in the breastmilk of seropositive mothers. Microorganisms. (2021) 9:1413. doi: 10.3390/microorganisms9071413
15. WHO. Health Topics Breastfeeding. [Internet]. (2022). Available online at: https://www.who.int/health-topics/breastfeeding#tab=tab_1 (accessed January 26, 2022).
16. Prendergast AJ, Goga AE, Waitt C, Gessain A, Taylor GP, Rollins N, et al. Transmission of CMV, HTLV-1, and HIV through breastmilk. Lancet Child Adolesc Health. (2019) 3:264–73. doi: 10.1016/S2352-4642(19)30024-0
17. Ishak R, Harrington WJ Jr., Azevedo VN, Eiraku N, Ishak MO, Guerreiro JF, et al. Identification of human T cell lymphotropic virus type IIa infection in the kayapo, an indigenous population of Brazil. AIDS Res Hum Retroviruses. (1995) 11:813–21. doi: 10.1089/aid.1995.11.813
18. Desgraupes S, Hubert M, Gessain A, Ceccaldi PE, Vidy A. Mother-to-child transmission of arboviruses during breastfeeding: from epidemiology to cellular mechanisms. Viruses. (2021) 13:1312. doi: 10.3390/v13071312
19. Ando Y, Ekuni Y, Matsumoto Y, Nakano S, Saito K, Kakimoto K, et al. Long-term serological outcome of infants who received frozen-thawed milk from human T-lymphotropic virus type-I positive mothers. J Obstet Gynaecol Res. (2004) 30:436–8. doi: 10.1111/j.1447-0756.2004.00227.x
20. Yamanouchi K, Kinoshita K, Moriuchi R, Katamine S, Amagasaki T, Ikeda S, et al. Oral transmission of human T-cell leukemia virus type-I into a common marmoset (Callithrix jacchus) as an experimental model for milk-borne transmission. Jpn J Cancer Res. (1985) 76:481–7.
21. Kinoshita K, Yamanouchi K, Ikeda S, Momita S, Amagasaki T, Soda H, et al. Oral infection of a common marmoset with human T-cell leukemia virus type-I (HTLV-I) by inoculating fresh human milk of HTLV-I carrier mothers. Jpn J Cancer Res. (1985) 76:1147–53.
22. Uemura Y, Kotani S, Yoshimoto S, Fujishita M, Yano S, Ohtsuki Y, et al. Oral transmission of human T-cell leukemia virus type I in the rabbit. Jpn J Cancer Res. (1986) 77:970–3.
23. Kato H, Koya Y, Ohashi T, Hanabuchi S, Takemura F, Fujii M, et al. Oral administration of human T-cell leukemia virus type 1 induces immune unresponsiveness with persistent infection in adult rats. J Virol. (1998) 72:7289–93. doi: 10.1128/JVI.72.9.7289-7293.1998
24. Takenouchi N, Matsuoka E, Moritoyo T, Nagai M, Katsuta K, Hasui K, et al. Molecular pathologic analysis of the tonsil in HTLV-I-infected individuals. J Acquir Immune Defic Syndr. (1999) 22:200–7. doi: 10.1097/00126334-199910010-00014
25. Tangy F, Ossondo M, Vernant JC, Smadja D, Bletry O, Baglin AC, et al. Human T cell leukemia virus type I expression in salivary glands of infected patients. J Infect Dis. (1999) 179:497–502. doi: 10.1086/314588
26. Nakamura H, Hasegawa H, Sasaki D, Takatani A, Shimizu T, Kurushima S, et al. Detection of human T lymphotropic virus type-I bZIP factor and tax in the salivary glands of Sjogren’s syndrome patients. Clin Exp Rheumatol. (2018) 36(Suppl. 112):51–60.
27. Nakamura H, Shimizu T, Takatani A, Suematsu T, Nakamura T, Kawakami A. Initial human T-cell leukemia virus type 1 infection of the salivary gland epithelial cells requires a biofilm-like structure. Virus Res. (2019) 269:197643. doi: 10.1016/j.virusres.2019.197643
28. Paxson CL Jr., Cress CC. Survival of human milk leukocytes. J Pediatr. (1979) 94:61–4. doi: 10.1016/s0022-3476(79)80352-2
29. Takeuchi H, Takahashi M, Norose Y, Takeshita T, Fukunaga Y, Takahashi H. Transformation of breast milk macrophages by HTLV-I: implications for HTLV-I transmission via breastfeeding. Biomed Res. (2010) 31:53–61. doi: 10.2220/biomedres.31.53
30. Seelig LL Jr., Head JR. Uptake of lymphocytes fed to suckling rats. an autoradiographic study of the transit of labeled cells through the neonatal gastric mucosa. J Reprod Immunol. (1987) 10:285–97. doi: 10.1016/0165-0378(87)90031-3
31. Tuboly S, Bernath S, Glavits R, Kovacs A, Megyeri Z. Intestinal absorption of colostral lymphocytes in newborn lambs and their role in the development of immune status. Acta Vet Hung. (1995) 43:105–15.
32. Jain L, Vidyasagar D, Xanthou M, Ghai V, Shimada S, Blend M. In vivo distribution of human milk leucocytes after ingestion by newborn baboons. Arch Dis Child. (1989) 64:930–3. doi: 10.1136/adc.64.7_spec_no.930
33. Jakaitis BM, Denning PW. Human breast milk and the gastrointestinal innate immune system. Clin Perinatol. (2014) 41:423–35. doi: 10.1016/j.clp.2014.02.011
34. Varol C, Zigmond E, Jung S. Securing the immune tightrope: mononuclear phagocytes in the intestinal lamina propria. Nat Rev Immunol. (2010) 10:415–26. doi: 10.1038/nri2778
35. Cabinian A, Sinsimer D, Tang M, Zumba O, Mehta H, Toma A, et al. Transfer of maternal immune cells by breastfeeding: maternal cytotoxic T lymphocytes present in breast milk localize in the Peyer’s patches of the nursed infant. PLoS One. (2016) 11:e0156762. doi: 10.1371/journal.pone.0156762
36. Jung C, Hugot JP, Barreau F. Peyer’s patches: the immune sensors of the intestine. Int J Inflam. (2010) 2010:823710. doi: 10.4061/2010/823710
37. Amerongen HM, Weltzin R, Farnet CM, Michetti P, Haseltine WA, Neutra MR. Transepithelial transport of HIV-1 by intestinal M cells: a mechanism for transmission of aids. J Acquir Immune Defic Syndr (1988). (1991) 4:760–5.
38. Amerongen HM, Wilson GA, Fields BN, Neutra MR. Proteolytic processing of reovirus is required for adherence to intestinal M cells. J Virol. (1994) 68:8428–32. doi: 10.1128/JVI.68.12.8428-8432.1994
39. Sicinski P, Rowinski J, Warchol JB, Jarzabek Z, Gut W, Szczygiel B, et al. Poliovirus type 1 enters the human host through intestinal M cells. Gastroenterology. (1990) 98:56–8. doi: 10.1016/0016-5085(90)91290-m
40. Haugh KA, Ladinsky MS, Ullah I, Stone HM, Pi R, Gilardet A, et al. In vivo imaging of retrovirus infection reveals a role for siglec-1/Cd169 in multiple routes of transmission. eLife. (2021) 10:e64179. doi: 10.7554/eLife.64179
41. Kazanji M, Ureta-Vidal A, Ozden S, Tangy F, de Thoisy B, Fiette L, et al. Lymphoid organs as a major reservoir for human T-Cell leukemia virus type 1 in experimentally infected squirrel monkeys (Saimiri sciureus): provirus expression, persistence, and humoral and cellular immune responses. J Virol. (2000) 74:4860–7. doi: 10.1128/jvi.74.10.4860-4867.2000
42. Haynes RA II, Zimmerman B, Millward L, Ware E, Premanandan C, Yu L, et al. Early spatial and temporal events of human T-lymphotropic virus type 1 spread following blood-borne transmission in a rabbit model of infection. J Virol. (2010) 84:5124–30. doi: 10.1128/JVI.01537-09
43. Percher F, Jeannin P, Martin-Latil S, Gessain A, Afonso PV, Vidy-Roche A, et al. Mother-to-child transmission of HTLV-1 epidemiological aspects, mechanisms and determinants of mother-to-child transmission. Viruses. (2016) 8:E40. doi: 10.3390/v8020040
44. Carpentier A, Barez PY, Hamaidia M, Gazon H, de Brogniez A, Perike S, et al. Modes of human T cell leukemia virus type 1 transmission, replication and persistence. Viruses. (2015) 7:3603–24. doi: 10.3390/v7072793
45. Kalach N, Rocchiccioli F, de Boissieu D, Benhamou PH, Dupont C. Intestinal permeability in children: variation with age and reliability in the diagnosis of cow’s milk allergy. Acta Paediatr. (2001) 90:499–504. doi: 10.1080/08035250116842
46. Afonso PV, Ozden S, Cumont MC, Seilhean D, Cartier L, Rezaie P, et al. Alteration of blood-brain barrier integrity by retroviral infection. PLoS Pathog. (2008) 4:e1000205. doi: 10.1371/journal.ppat.1000205
47. Martin-Latil S, Gnadig NF, Mallet A, Desdouits M, Guivel-Benhassine F, Jeannin P, et al. Transcytosis of HTLV-1 across a tight human epithelial barrier and infection of subepithelial dendritic cells. Blood. (2012) 120:572–80. doi: 10.1182/blood-2011-08-374637
48. Sufiawati I, Herrera R, Mayer W, Cai X, Borkakoti J, Lin V, et al. Human immunodeficiency virus (HIV) and human cytomegalovirus (HCMC) coinfection of infant tonsil epithelium may synergistically promote both HIV-1 and HCMV spread and infection. J Virol. (2021) 95:e0092121. doi: 10.1128/JVI.00921-21
49. Ohata J, Matsuoka M, Yamashita T, Tojo A, Tani K, Asano S. Cd4/Cd8 double-positive adult T cell leukemia with preceding cytomegaloviral gastroenterocolitis. Int J Hematol. (1999) 69:92–5.
50. Blackshaw K, Valtchev P, Koolaji N, Berry N, Schindeler A, Dehghani F, et al. The risk of infectious pathogens in breast-feeding, donated human milk and breast Milk substitutes. Public Health Nutr. (2021) 24:1725–40. doi: 10.1017/S1368980020000555
51. Lee SA, Lim JY, Kim BS, Cho SJ, Kim NY, Kim OB, et al. Comparison of the gut microbiota profile in breast-fed and formula-fed Korean infants using pyrosequencing. Nutr Res Pract. (2015) 9:242–8. doi: 10.4162/nrp.2015.9.3.242
52. Walker M. Formula supplementation of the breastfed infant: assault on the gut microbiome. Clin Lact. (2014) 5:128–32. doi: 10.1891/2158-0782.5.4.128
53. Prameela KK. HIV transmission through breastmilk: the science behind the understanding of current trends and future research. Med J Malaysia. (2012) 67:644–51.
54. Tugizov SM, Herrera R, Veluppillai P, Greenspan D, Soros V, Greene WC, et al. Differential transmission of HIV traversing fetal oral/intestinal epithelia and adult oral epithelia. J Virol. (2012) 86:2556–70. doi: 10.1128/JVI.06578-11
55. Zacharopoulos VR, Perotti ME, Phillips DM. Lymphocyte-facilitated infection of epithelia by human T-cell lymphotropic virus type I. J Virol. (1992) 66:4601–5. doi: 10.1128/JVI.66.7.4601-4605.1992
56. Bomsel M. Transcytosis of infectious human immunodeficiency virus across a tight human epithelial cell line barrier. Nat Med. (1997) 3:42–7. doi: 10.1038/nm0197-42
57. Igakura T, Stinchcombe JC, Goon PK, Taylor GP, Weber JN, Griffiths GM, et al. Spread of HTLV-I between lymphocytes by virus-induced polarization of the cytoskeleton. Science. (2003) 299:1713–6. doi: 10.1126/science.1080115
58. Gross C, Thoma-Kress AK. Molecular mechanisms of HTLV-1 cell-to-cell transmission. Viruses. (2016) 8:74. doi: 10.3390/v8030074
59. Pais-Correia AM, Sachse M, Guadagnini S, Robbiati V, Lasserre R, Gessain A, et al. Biofilm-like extracellular viral assemblies mediate HTLV-1 cell-to-cell transmission at virological synapses. Nat Med. (2010) 16:83–9. doi: 10.1038/nm.2065
60. Alais S, Mahieux R, Dutartre H. Viral source-independent high susceptibility of dendritic cells to human T-cell leukemia virus type 1 infection compared to that of T lymphocytes. J Virol. (2015) 89:10580–90. doi: 10.1128/JVI.01799-15
61. Millen S, Gross C, Donhauser N, Mann MC, Peloponese JM Jr., Thoma-Kress AK. Collagen IV (COL4A1, COL4A2), a component of the viral biofilm, is induced by the HTLV-1 oncoprotein tax and impacts virus transmission. Front Microbiol. (2019) 10:2439. doi: 10.3389/fmicb.2019.02439
62. Pinto DO, Al Sharif S, Mensah G, Cowen M, Khatkar P, Erickson J, et al. Extracellular vesicles from HTLV-1 infected cells modulate target cells and viral spread. Retrovirology. (2021) 18(1):6. doi: 10.1186/s12977-021-00550-8
63. Macatonia SE, Cruickshank JK, Rudge P, Knight SC. Dendritic cells from patients with tropical spastic paraparesis are infected with HTLV-1 and stimulate autologous lymphocyte Proliferation. AIDS Res Hum Retroviruses. (1992) 8:1699–706. doi: 10.1089/aid.1992.8.1699
64. Jones KS, Petrow-Sadowski C, Huang YK, Bertolette DC, Ruscetti FW. Cell-free HTLV-1 infects dendritic cells leading to transmission and transformation of CD4+ T cells. Nat Med. (2008) 14:429–36. doi: 10.1038/nm1745
65. Jain P, Manuel SL, Khan ZK, Ahuja J, Quann K, Wigdahl B. Dc-sign mediates cell-free infection and transmission of human T-cell lymphotropic virus type 1 by dendritic cells. J Virol. (2009) 83:10908–21. doi: 10.1128/JVI.01054-09
66. Assil S, Futsch N, Decembre E, Alais S, Gessain A, Cosset FL, et al. Sensing of cell-associated HTLV by plasmacytoid dendritic cells is regulated by dense beta-galactoside glycosylation. PLoS Pathog. (2019) 15:e1007589. doi: 10.1371/journal.ppat.1007589
67. Witkowska-Zimny M, Kaminska-El-Hassan E. Cells of human breast milk. Cell Mol Biol Lett. (2017) 22:11. doi: 10.1186/s11658-017-0042-4
68. Cacho NT, Lawrence RM. Innate immunity and breast milk. Front Immunol. (2017) 8:584. doi: 10.3389/fimmu.2017.00584
69. Twigger AJ, Hepworth AR, Lai CT, Chetwynd E, Stuebe AM, Blancafort P, et al. Gene expression in breastmilk cells is associated with maternal and infant characteristics. Sci Rep. (2015) 5:12933. doi: 10.1038/srep12933
70. Moore RE, Xu LL, Townsend SD. Prospecting human milk oligosaccharides as a defense against viral infections. ACS Infect Dis. (2021) 7:254–63. doi: 10.1021/acsinfecdis.0c00807
71. Ramani S, Stewart CJ, Laucirica DR, Ajami NJ, Robertson B, Autran CA, et al. Human milk oligosaccharides, milk microbiome and infant gut microbiome modulate neonatal rotavirus infection. Nat Commun. (2018) 9:5010. doi: 10.1038/s41467-018-07476-4
72. Kinoshita K, Hino S, Amagaski T, Ikeda S, Yamada Y, Suzuyama J, et al. Demonstration of adult T-cell leukemia virus antigen in milk from three sero-positive mothers. Gan. (1984) 75:103–5.
73. Matsubara F, Haraguchi K, Harada K, Koizumi A. Screening for antibodies to human T-cell leukemia virus type I in Japanese breast milk. Biol Pharm Bull. (2012) 35:773–6. doi: 10.1248/bpb.35.773
74. LeVasseur RJ, Southern SO, Southern PJ. Mammary epithelial cells support and transfer productive human T-cell lymphotropic virus infections. J Hum Virol. (1998) 1:214–23.
75. Southern SO, Southern PJ. Persistent HTLV-I infection of breast luminal epithelial cells: a role in HTLV transmission? Virology. (1998) 241:200–14. doi: 10.1006/viro.1997.8978
76. Loureiro P, Southern SO, Southern PJ, Pombo-de-Oliveira MS. Clinicopathological studies of a patient with adult T-cell leukemia and pseudogynecomasty. Am J Hematol. (2000) 65:256–9. doi: 10.1002/1096-8652(200011)65:3
77. Armistead B, Jiang Y, Carlson M, Ford ES, Jani S, Houck J, et al. Mucosal memory T cells in breastmilk are modulated by SARS-CoV-2 mRNA vaccination. medRxiv [Preprint]. (2021). doi: 10.1101/2021.12.03.21267036
78. Moriuchi M, Moriuchi HA. Milk protein lactoferrin enhances human T cell leukemia virus type I and suppresses HIV-1 infection. J Immunol. (2001) 166:4231–6. doi: 10.4049/jimmunol.166.6.4231
79. Moriuchi M, Moriuchi H. Transforming growth factor-beta enhances human T-cell leukemia virus type I infection. J Med Virol. (2002) 67:427–30. doi: 10.1002/jmv.10074
80. Moriuchi M, Inoue H, Moriuchi H. Reciprocal interactions between human T-lymphotropic virus type 1 and prostaglandins: implications for viral transmission. J Virol. (2001) 75:192–8. doi: 10.1128/JVI.75.1.192-198.2001
81. Moriuchi M, Moriuchi H. Induction of lactoferrin gene expression in myeloid or mammary gland cells by human T-cell leukemia virus type 1 (HTLV-1) tax: implications for milk-borne transmission of HTLV-1. J Virol. (2006) 80:7118–26. doi: 10.1128/JVI.00409-06
82. Rosadas C, Caterino-de-Araujo A, Taylor GP. Specificity of HTLV screening tests and its impact on health care program costs: the perspective of antenatal screening in Brazil. Rev Soc Bras Med Trop. (2021) 54:e0853–2020. doi: 10.1590/0037-8682-0853-2020
83. Malik B, Taylor GP. Can we reduce the incidence of adult T-cell leukaemia/lymphoma? Cost-effectiveness of human T-lymphotropic virus type 1 (HTLV-1) antenatal screening in the United Kingdom. Br J Haematol. (2019) 184:1040–3. doi: 10.1111/bjh.15234
84. Nishijima T, Shimada S, Noda H, Miyake K. Towards the elimination of HTLV-1 infection in Japan. Lancet Infect Dis. (2019) 19:15–6. doi: 10.1016/S1473-3099(18)30735-7
85. Itabashi K, Miyazawa T, Sekizawa A, Tokita A, Saito S, Moriuchi H, et al. A nationwide antenatal human T-cell leukemia virus type-1 antibody screening in Japan. Front Microbiol. (2020) 11:595. doi: 10.3389/fmicb.2020.00595
86. PAHO/WHO. Webinar HTLV World Day: International Health Policy Forum for the Elimination of HTLV. [Internet]. (2021). Available online at: https://www.paho.org/en/international-health-policy-forum-elimination-htlv (accessed January 26, 2022).
87. Boostani R, Sadeghi R, Sabouri A, Ghabeli-Juibary A. Human T-lymphotropic virus type I and breastfeeding; systematic review and meta-analysis of the literature. Iran J Neurol. (2018) 17: 174–9.
88. Itabashi K, Miyazawa T. Mother-to-child transmission of human T-cell leukemia virus type 1: mechanisms and nutritional strategies for prevention. Cancers (Basel). (2021) 13:4100. doi: 10.3390/cancers13164100
89. Mosca F, Gianni ML. Human milk: composition and health benefits. Pediatr Med Chir. (2017) 39:155. doi: 10.4081/pmc.2017.155
90. Lembcke JL, Brown KH. Effect of milk-containing diets on the severity and duration of childhood diarrhea. Acta Paediatr Suppl. (1992) 381:87–92. doi: 10.1111/j.1651-2227.1992.tb12378.x
91. Santos FS, Santos FC, Santos LH, Leite AM, Mello DF. Breastfeeding and protection against diarrhea: an integrative review of literature. Einstein (Sao Paulo). (2015) 13:435–40. doi: 10.1590/S1679-45082015RW3107
92. Li KMC, Li KYC, Bick D, Chang YS. Human immunodeficiency virus-positive women’s perspectives on breastfeeding with antiretrovirals: a qualitative evidence synthesis. Matern Child Nutr. (2021) 17:e13244. doi: 10.1111/mcn.13244
93. WHO. Guideline: Updates on HIV and Infant Feeding. The Duration of Breastfeeding, and Support From Health Services to Improve Feeding Practices Among Mothers Living With HIV. [Internet]. (2016). Available online at: https://www.who.int/publications/i/item/9789241549707 (accessed January 26, 2022).
94. Committee on Pediatric AIDS. Infant feeding and transmission of human immunodeficiency virus in the United States. Pediatrics. (2013) 131:391–6. doi: 10.1542/peds.2012-3543
95. CDC. HIV and Pregnant Women, Infants, and Children [Internet]. (2022). Available online at: https://www.cdc.gov/hiv/group/gender/pregnantwomen/index.html (accessed February 16, 2022).
96. Sarkar I, Hauber I, Hauber J, Buchholz F. HIV-1 proviral DNA excision using an evolved recombinase. Science. (2007) 316:1912–5. doi: 10.1126/science.1141453
97. Karpinski J, Hauber I, Chemnitz J, Schafer C, Paszkowski-Rogacz M, Chakraborty D, et al. Directed evolution of a recombinase that excises the provirus of most HIV-1 primary isolates with high specificity. Nat Biotechnol. (2016) 34:401–9. doi: 10.1038/nbt.3467
98. Tanaka A, Takeda S, Kariya R, Matsuda K, Urano E, Okada S, et al. A novel therapeutic molecule against HTLV-1 infection targeting provirus. Leukemia. (2013) 27:1621–7. doi: 10.1038/leu.2013.46
99. Panfil AR, Green PL, Yoder KE. Crispr genome editing applied to the pathogenic retrovirus HTLV-1. Front Cell Infect Microbiol. (2020) 10:580371. doi: 10.3389/fcimb.2020.580371
100. Saito M, Tanaka R, Fujii H, Kodama A, Takahashi Y, Matsuzaki T, et al. The neutralizing function of the anti-HTLV-1 antibody is essential in preventing in vivo transmission of HTLV-1 to human T cells in nod-scid/gammacnull (Nog) mice. Retrovirology. (2014) 11:74. doi: 10.1186/s12977-014-0074-z
101. Murakami Y, Hasegawa A, Ando S, Tanaka R, Masuda T, Tanaka Y, et al. A novel mother-to-child human T-cell leukaemia virus type 1 (HTLV-1) transmission model for investigating the role of maternal anti-HTLV-1 antibodies using orally infected mother rats. J Gen Virol. (2017) 98:835–46. doi: 10.1099/jgv.0.000733
Keywords: HTLV-1, virus transmission, breastfeeding, breast milk, tonsils, small intestine, oral route
Citation: Millen S and Thoma-Kress AK (2022) Milk Transmission of HTLV-1 and the Need for Innovative Prevention Strategies. Front. Med. 9:867147. doi: 10.3389/fmed.2022.867147
Received: 31 January 2022; Accepted: 18 February 2022;
Published: 11 March 2022.
Edited by:
Ricardo Ishak, Federal University of Pará, BrazilReviewed by:
Tatiane Assone, FMUSP, BrazilCopyright © 2022 Millen and Thoma-Kress. This is an open-access article distributed under the terms of the Creative Commons Attribution License (CC BY). The use, distribution or reproduction in other forums is permitted, provided the original author(s) and the copyright owner(s) are credited and that the original publication in this journal is cited, in accordance with accepted academic practice. No use, distribution or reproduction is permitted which does not comply with these terms.
*Correspondence: Andrea K. Thoma-Kress, YW5kcmVhLnRob21hLWtyZXNzQHVrLWVybGFuZ2VuLmRl
†These authors have contributed equally to this work
Disclaimer: All claims expressed in this article are solely those of the authors and do not necessarily represent those of their affiliated organizations, or those of the publisher, the editors and the reviewers. Any product that may be evaluated in this article or claim that may be made by its manufacturer is not guaranteed or endorsed by the publisher.
Research integrity at Frontiers
Learn more about the work of our research integrity team to safeguard the quality of each article we publish.