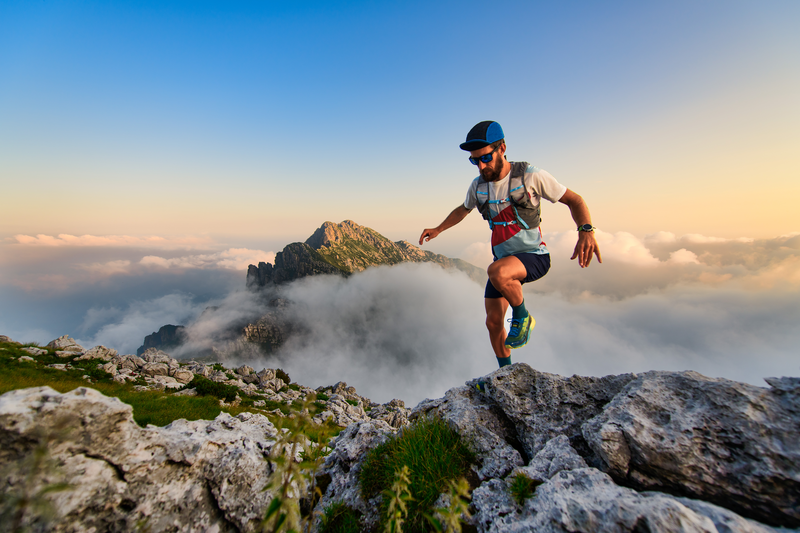
94% of researchers rate our articles as excellent or good
Learn more about the work of our research integrity team to safeguard the quality of each article we publish.
Find out more
ORIGINAL RESEARCH article
Front. Med. , 16 March 2022
Sec. Gastroenterology
Volume 9 - 2022 | https://doi.org/10.3389/fmed.2022.849677
This article is part of the Research Topic Global Excellence in Gastroenterology: Africa View all 8 articles
Background: Environmental enteropathy (EE) contributes to impaired linear growth (stunting), in millions of children worldwide. We have previously reported that confocal laser endomicroscopy (CLE) shows fluorescein leaking from blood to gut lumen in vivo in adults and children with EE. We set out to identify epithelial lesions which might explain this phenomenon in Zambian children with stunting non-responsive to nutritional support.
Methods: We performed confocal laser endomicroscopy (CLE) in 75 children and collected intestinal biopsies for histology in 91 children. CLE videos were evaluated, employing the Watson score to determine severity of leakiness. Morphometry was carried out on well-orientated mucosa and 3 biopsies were examined by electron microscopy.
Results: Confocal laser endomicroscopy demonstrated substantial leakage from circulation to gut lumen in 73 (97%) children. Histology consistently showed characteristic changes of EE: villus blunting, lamina propria and epithelial inflammation, and depletion of secretory cells (Paneth cells and goblet cells). Epithelial abnormalities included marked variability in epithelial height, disorganised and shortened microvilli, dilated intercellular spaces, pseudostratification, formation of synechiae between epithelium on adjacent villi, crypt destruction, and abundant destructive lesions which may correspond to the microerosions identified on CLE.
Conclusion: Epithelial abnormalities were almost universal in Zambian children with non-responsive stunting, including epithelial microerosions, cell-cell adhesion anomalies, and defects in secretory cells which may all contribute to impairment of mucosal barrier function and microbial translocation.
Stunting in young children is defined as length for age Z-score <-2.0 (1). Stunting is a pervasive problem in low and middle income countries (LMICs) worldwide (2), and is associated with increased mortality (3), impaired neurocognitive development (4) and blunted responses to oral vaccines (5, 6). A large body of evidence shows that nutritional supplementation does not overcome childhood stunting in LMICs (7), even when given during early pregnancy (8), possibly because of endemic environmental enteropathy (EE) in pregnant women (9, 10). It is not clear how much of the dysfunction is attributable to altered composition and function of the microbiome, but recent evidence that microbiota-directed complementary foods can improve growth (11) suggests that it may be a substantial contribution. EE is a chronic condition of the small intestine combining loss of villous architecture, reduced absorptive function, and intestinal and systemic inflammation. We have previously identified epithelial lesions as central features of enteropathy in children with severe acute malnutrition (SAM), where enteropathy drives microbial translocation and may correlate with adverse outcomes (12).
The spectrum of small intestinal disorders described as “enteropathy” has expanded recently. These enteropathies share many pathological features, and many of them lead to malnutrition and both mucosal and systemic inflammation. Coeliac disease was described by Gee in 1880, and tropical sprue was described by Manson in 1888, but older descriptions of clinical syndromes resembling these enteropathies date back centuries (13). Coeliac disease, like environmental enteropathy, may be manifest as malnutrition in childhood, through a combination of maldigestion, malabsorption, and inflammation. Infective enteropathy includes Whipple’s disease, non-tuberculous mycobacteriosis, strongyloidiasis, and other persisting infections. Persisting bacterial and protozoal infections are potent and highly prevalent causes of malnutrition in children (14). Immunological enteropathies, all rare, include graft-versus-host disease (GVHD), common variable immunodeficiency (CVID), CD55 deficiency (15), transplantation rejection enteropathy (16), and autoimmune enteropathy (17, 18). Two drug-induced enteropathies, olmesartan enteropathy (19) and immune checkpoint inhibitor enteropathy (20) are probably also immune-mediated. Radiation-induced small intestinal enteropathy is induced by DNA damage in stem cells and damage to vascular endothelium (21). The key role of prostaglandins in maintaining small intestinal mucosal integrity is emphasised by the role of non-steroidal anti-inflammatory drugs (NSAIDs) (19) and SLCO2A1 mutations (22) in inducing enteropathy. These enteropathies all share villus blunting and inflammation as prominent pathological abnormalities, and it is likely that reduced surface area plays a major role in driving the impaired nutritional state which is a common clinical manifestation.
Recent work has achieved a degree of consensus among pathologists on key histological features of environmental enteropathy (23). Villus architectural remodelling (villus blunting) is a key feature of enteropathy, as was described decades ago (24, 25). Loss of goblet cells and Paneth cells is also a prominent feature of EE, in common with autoimmune enteropathies (17). Epithelial thinning, also referred to as flattening, or cuboidal change, is also a prominent feature of autoimmune enteropathies (17). We recently reported leakage from circulation to gut lumen in adults (26) and children (27) with EE using confocal laser endomicroscopy (CLE). Here, we assess abnormalities of the epithelium in children with environmental enteropathy to identify epithelial pathology that might explain leakiness of the small intestinal mucosa, combining CLE, histology, and electron microscopy.
The BEECH study has been described in detail previously (27). In brief, children under 12 months of age in Misisi, Lusaka, were screened for evidence of growth failure. Those with weight-for-age z (WAZ) score of ≤-1 were invited to come to the local research clinic, St Augustine Clinic, for further evaluation. Those with length-for-age z (LAZ) scores of –2 or less were offered daily nutritional supplementation with corn-soy blend (28) (1.5 kg/week), an egg, and a single micronutrient sprinkle (Nutromix, Hexagon Ltd., Mumbai, India), for periods of 4–8 months. Those with no evidence of response (consistent LAZ score of ≤–2 over at least 1 month) were investigated further (29), including upper gastrointestinal endoscopy, biopsy for morphometry and confocal laser endomicroscopy (27). Ethics approval for this study was obtained from the University of Zambia Biomedical Research Ethics Committee (approval 006-02-16 dated 31 May 2016), and written consent was obtained from all the parents and guardians of children participating in the study.
We performed endoscopy with a Pentax EG-2490k gastroscope with external diameter 8mm and working channel diameter of 2.4 mm. CLE was performed before biopsies were collected, using the Cellvizio system (Mauna Kea Technologies, Paris, France) with Alveoflex probes as previously reported (27). After obtaining a stable viewing position in the second part of the duodenum, and avoiding trauma to the mucosa, 2 mL of 1% fluorescein was injected intravenously and images were collected continuously for one minute. Videos were evaluated by a single observer (VK); after initial fogging and excluding periods of non-contact or movement artefact the median duration of evaluable imaging was 50 (IQR 39–56, range 27–106) s. The Watson score (30) was used to evaluate the severity of epithelial leakiness, but as the Alveoflex has lower resolution than the Pentax EG3870CIK confocal laser endomicroscope we used previously (26) it was difficult reliably to discriminate between Watson grades 2 and 3 which requires reliable identification of microerosions.
Biopsies were collected into normal (0.9%) saline and orientated under a dissecting microscope, then placed on cellulose acetate paper (Sartorius GmbH, Gottingen, Germany) in neutral buffered formal-saline (CellPath Ltd., Newtown, Powys, United Kingdom), and processed into wax blocks. Sections of 3–4 μm were stained with haematoxylin and eosin and then scanned on an Olympus VS120 slide scanning microscope at 20× magnification, as previously described (27), in order to generate measurements of villus height (VH), crypt depth (CD), and epithelial perimeter in relation to length of muscularis mucosae (Supplementary Figure 1). All slides were reviewed for features which could contribute to an understanding of epithelial leakiness. Morphometry was carried out on well-orientated mucosa (Supplementary Figure 1). The criterion for orientation of a section or part of a section was that each crypt should be seen throughout its length. Where this criterion was satisfied, all villus and crypt units, the overlying epithelium, and the underlying muscularis mucosae, were measured. Especial care was taken to include small villus units which represent the beginning and end of a ridge-like villus. Epithelial and brush border heights were also measured in the same sections. One biopsy each from three participating children selected at random were placed without orientation into glutaraldehyde buffer (Sigma, Poole, United Kingdom) for electron microscopy and transported to the Washington University Center for Cellular Imaging (St. Louis, MO, United States) where they were further rinsed in 0.15 M cacodylate buffer with 2 mM calcium chloride three times for 10 min each and subjected to a secondary fixation for 1 h in 1% osmium tetroxide/1.5% potassium ferrocyanide in cacodylate buffer. Following this, samples were rinsed in ultrapure water three times for 10 min each and incubated in an aqueous solution of 2% uranyl acetate overnight at 4°C. The samples were then rinsed in ultrapure water 3 times for 10 minutes each, dehydrated in a graded ethanol series (30%, 50%, 70%, 90%, 100% 3x) for 10 min in each step, and infiltrated with microwave assistance (Pelco BioWave Pro, Redding, CA, United States) into Epon resin. Samples were embedded and cured in an oven at 60°C for 72 h. Post resin curing, samples were sectioned and 70 nm thick sections were stained with aqueous 2% uranyl acetate and Reynold’s lead prior to imaging on a transmission electron microscope (TEM JEOL JEM-1400 Plus) at 120 kV.
Intestinal biopsies of adequate quality for assessment were obtained from 91 children with stunting, and CLE videos were obtained in 75 procedures [Table 1 and reference (27)]. Biopsies from 27 children were unsatisfactory for mucosal evaluation. The images show consistent evidence of epithelial leakiness, demonstrated by plumes of fluorescein observed flowing from systemic circulation, into which the fluorescein was injected, into intestinal lumen (Figure 1). Images from 73 (97%) children had Watson scores of 2 or 3 and only two (2.7%) had a score of 1. CLE showed fluorescein in intercellular spaces between epithelial cells (Figure 1) in 46 (62%) of videos, although this was usually patchy and variable in extent. Occasional microerosions were also recorded (Figure 1D). Light microscopy revealed a pathogen (Giardia intestinalis) in one biopsy, which had not been detected on stool examination.
Table 1. Clinical and demographic characteristics of children who had biopsies and the subset who had confocal laser endomicroscopy.
Figure 1. Confocal laser endomicroscopy images of environmental enteropathy in Zambian children with stunting. (A) Normal image showing fluorescein, which was injected intravenously, in villus epithelium and lamina propria and no signal in the lumen which therefore appears dark (star). (B) Fluorescein leakage from epithelium as plumes (white hollow arrows). (C) Fluorescein highlighting intercellular spaces (small arrows) and two microerosions (black hollow arrows). (D) Fluorescein highlighting intercellular spaces and leaking into gut lumen (small arrows). (E) Fluorescein plume (white hollow arrow) originating from capillaries, the escaping through defect in epithelium and into lumen.
Biopsies showed a wide range of appearances, from mild inflammation to severe villus atrophy (Figure 2 and Table 2). On the whole VH was reduced compared to a UK reference range (Table 2), and 80 (88%) of 91 children had villus blunting, defined as below the United Kingdom reference range. Lymphoid aggregates and penetration of the mucosa by Brunner’s glands were also observed (Supplementary Figures 2, 3). Three of the biopsies demonstrated ballooning of the villi due to expansion of the lamina propria (Figure 2).
Figure 2. Mucosal features of environmental enteropathy. (A) Marked villus blunting in a well-orientated section (confirmed by crypts imaged in full longitudinal section). (B) Ballooning of villus tips caused by expansion of translucent material or oedema marked by *. (C) Pronounced inflammatory infiltrate in lamina propria. (D) Extensive villus fusion, in which fused villus structures overlie several crypts. (E) Loss of epithelial integrity at villus tips representing microerosions.
In 89 biopsies from 91 children the epithelium contained expanded intercellular spaces, and in 18 of these it was severe (Figure 3); this appeared consistent with the intercellular fluorescein accumulation seen in CLE videos (Figures 1D,E). This was corroborated by electron microscopy which demonstrated wide gaps in between epithelial cells (Figure 3).
Figure 3. Light and electron micrographs showing dilated intercellular spaces. (A) Light micrograph of epithelium in a biopsy from a child with stunting; black arrows indicate dilated intercellular spaces. (B) Electron micrograph from the same child as panel (B). Solid white arrows indicate dilated intercellular spaces. (C) Light micrograph of crypts in a biopsy from a different child showing the same dilated intercellular spaces; the dotted black arrow indicates an enteroendocrine cell. (D) Further magnification of the are in panel (C) represented by the white box showing the dilated intercellular spaces more clearly.
Epithelial height displayed variation between biopsies. There was also marked irregularity in epithelial cell height (Figure 4 and Table 2), which in some cases resembled tufting (Figure 4C). Pseudostratification was also observed (Figure 4D). Destructive lesions and microerosions which appear consistent with epithelial defects seen on CLE (Figure 1D).
Figure 4. Enterocyte morphological abnormalities. (A) Variable epithelial height, ranging from full epithelial height to severe flattening (black arrows); at the villus tip there is also scalloping (white hollow arrow) which frequently resembled tufting. (B,C) Villus tip epithelial defect (solid black arrow) allowing extrusion of lamina propria contents (white arrows) into lumen. (D) Pseudostratification, the misalignment of nuclei into layers (range illustrated by brackets) which normally occupy a uniformly basal position in the enterocyte.
Characteristic features of environmental enteropathy were observed, including goblet cell depletion, Paneth cell degranulation, and intra-epithelial lymphocytosis (23) (Figure 5). Enteroendocrine cells (Figure 5) were seen occasionally. In contrast, healthy biopsies from individuals without EE show plump eosinophilic Paneth cell granules and abundant goblet cells (Supplementary Figure 4).
Figure 5. Secretory cell abnormalities in environmental enteropathy. (A–C) Paneth cell depletion; the expected location of Paneth cells is shown, normally recognisable by brightly eosinophilic granules. (D,E) Electron micrographs of goblet cells with depleted granules.
The microvillus brush border (BB) was markedly irregular (Table 2 and Figure 6). Using a cut-off of 1.3μm to define shortened microvilli (33), 42 (46%) of biopsies showed BB thinning. VH correlated with BB (ρ = 0.24; P = 0.02) and epithelial height (ρ = 0.24; P = 0.02). Electron micrographs confirmed this irregularity of the brush border (Figure 6) but no pathogens were identified in sections imaged by EM as potential explanations for BB damage.
Figure 6. Brush border disruption in environmental enteropathy, ranging from minor loss of uniformity of microvilli (A,C) to marked loss of microvilli (B,D).
In a small number of biopsies, severe lamina propria inflammation was associated with crypt architectural abnormalities (Supplementary Figure 5) or loss of crypts (Supplementary Figure 6). Synechiae were observed in CLE images and corresponding structures were observed in histological sections (Figure 7), closely mirroring previously reported synechiae in a range of enteropathies (34).
Figure 7. Synechiae (epithelial bridges, marked with arrows) progressing to villus fusion. These are shown by confocal laser endomicroscopy (A,B) and histology (C–E).
Epithelial leakiness in environmental enteropathy is a central pathophysiological feature, associated with microbial translocation and previously visualised in vivo in adults and children using confocal laser endomicroscopy (26, 27). Here we report visual evidence of epithelial damage which appears to underlie this leakiness, and which is also likely to be a feature of several enteropathic conditions. The remarkable feature of the severe abnormalities described in this paper is that these biopsies were taken from ambulant, stunted children with no specific symptoms. We have identified dilated intercellular spaces, epithelial irregularity (with pseudostratification and occasional similarities to tufting enteropathy), brush border damage, and loss of secretory cells in the epithelium. We also identified crypt destruction in some biopsies, and we speculate that this may help explain the reduction in proliferating cells we have observed in adults using single cell transcriptomic analysis (35). Epithelial flattening has long been recognised as a feature of coeliac disease (36), and variable flattening of epithelial cells was clearly evident in this study of environmental enteropathy, closely resembling the epithelial flattening observed long ago in “PEM” (protein energy malnutrition; an outmoded term which describes syndromes now called “Severe Acute Malnutrition”)(37). Pseudostratification (Figure 4D) was observed in a large proportion of biopsies, contrasting clearly with normal biopsies which show nuclei in an orderly basal position in the epithelial cell (38). Both epithelial flattening and pseudostratification are features of coeliac disease, and synechiae are visible in biopsies from coeliac disease (39). The significance of these morphological changes is uncertain, but pseudostratification is a prominent feature of intestinal morphogenesis during fetal, or foetal development (40, 41). It may therefore represent a more proliferative epithelium, or possibly dedifferentiation. The principal limitation of this study is the lack of normal biopsies from children without EE. It is difficult to justify obtaining normal tissue from healthy children, and healthy controls from the same population may also have degrees of enteropathy given the high carriage rates of potentially pathogenic microbes. Thus identifying suitable controls is difficult, and it may be that outlier groups from temperate countries may be needed. Anyway, it would be highly desirable to have control tissue to conclude definitely that the epithelial abnormalities reported here are exclusive to environmental enteropathy.
Confocal laser endomicroscopy in young children is inevitably constrained by the lower resolution of the endoscopes than we can achieve in adults, because of size limits on instruments that can be used. In adults we used a Pentax EG3870CIK confocal laser endomicroscope, which has a resolution of 1 μm but an external diameter of 18mm which is too large for use in young children. In the children reported here and in our previous publication, we used Alveoflex probes which have lower resolution, insufficient to image cellular detail consistently, but the visualisation of the leak of fluorescein is still very clear and reliable and some cellular imaging is possible. Consequently, we could not discriminate reliably between Watson grades 2 and 3 in all cases. Our data do, however, show clearly that young children with stunting in Zambia have surprisingly severe environmental enteropathy with pronounced epithelial leakiness. Only 2 out of 75 children had Watson scores of 1, which contrasts sharply with grade 1 scores in 80% of German adults sampled from the same part of the duodenum (42). We are not aware of similar interrogations of the small bowel in children in industrialised countries using CLE.
Many of the key findings in this study recapitulate earlier studies using electron microscopy in children with PEM (37) or marasmus (43). Given the severity of the clinical malnutrition in those earlier studies, which would now be termed severe acute malnutrition (SAM), this is surprising as the children reported in the present study were all ambulant and apparently healthy, though significantly stunted. Children with SAM in the earlier studies were all hospitalised. Both Brunser (43) and Shiner (37) reported reduced size and morphological abnormalities in the brush border, and Shiner’s report (37) mentioned reduced epithelial height which improved on nutritional rehabilitation. In our samples there was a great deal of variability in epithelial height, which often showed marked irregularity resembling tufting enteropathy. Tufting enteropathy is a severe congenital childhood enteropathy which has now been reproduced in vitro in an EpCAM mutation model (44). It would be of interest to determine if disturbances of EpCAM expression play a role in environmental enteropathy. There are some important features of EE which help distinguish it from, for example, autoimmune enteropathy (AIE), which shares loss of goblet and Paneth cells but does not display increased densities of IELs (45).
The physiological consequences of dilated intercellular spaces, if any, remain uncertain and any relationship to tight junction malfunction needs to be clarified. Paracellular flow through dilated intercellular spaces may permit passive transexudation of plasma proteins and other plasma constituents, and is likely to contribute to permeation of molecules such as lactulose (46–48). It may also allow for passive uptake of malabsorbed nutrients [e.g., zinc (49)] following oral administration, in the immediate post-prandial phase when luminal concentrations are higher than plasma concentrations.
The disruption of the brush border observed in our study would be expected to have consequences for digestion and absorption. We have previously reported reduced gene expression of a range of digestive enzymes including enterokinase, aminopeptidases A and N, two trypsinogens, diprolyl peptidase 4, folate hydrolase, angiotensin converting enzyme, alkaline phosphatase, maltase-glucoamylase, sucrose-isomaltase, lactase, and trehalase in children with malnutrition and environmental enteropathy (50). A range of nutrient transporters (SLC genes) were also reduced (50). Taken together with the evidence of brush border damage reported here, and older evidence of the link between brush border health and disaccharide malabsorption (51), this strongly suggests that damage to the microvilli contributes to malabsorption which may underlie environmental enteric dysfunction (9, 10, 52–55).
Depletion of secretory cells appears to be a consistent feature of EE (23). As goblet cells secrete the mucus layer, and Paneth cells synthesise a variety of antimicrobial peptides into it (56), such defects would be expected to lead to impaired barrier function. It seems likely that impaired secretory function is responsible for the intense microbial translocation which we and others have reported in EE (12, 27). The mechanism of secretory cell depletion is not understood, but it was not observed in experimental riboflavin deficiency in animals (57). Whether other specific nutritional deficiencies or pathobiont colonisation (11, 27) are responsible for the epithelial damage in EE is not clear, but a clear understanding of mechanism is clearly urgently needed.
The original contributions presented in the study are included in the article/Supplementary Material, further inquiries can be directed to the corresponding author.
The studies involving human participants were reviewed and approved by University of Zambia Biomedical Research Ethics Committee. Written informed consent to participate in this study was provided by the participants’ legal guardian/next of kin.
CM, JF, VM and PK: study conceptualisation. CM, KC, BA, and PK: sample collection and processing. VM: biopsy scoring. VK: video scoring. SS and JF: electron microscopy. PK: first draft of the manuscript. All authors contributed to the manuscript.
Bill & Melinda Gates Foundation grant (OPP1066118) to PK.
The authors declare that the research was conducted in the absence of any commercial or financial relationships that could be construed as a potential conflict of interest.
All claims expressed in this article are solely those of the authors and do not necessarily represent those of their affiliated organizations, or those of the publisher, the editors and the reviewers. Any product that may be evaluated in this article, or claim that may be made by its manufacturer, is not guaranteed or endorsed by the publisher.
We are grateful for expert nursing to Rosemary Banda, Joyce Hamusonde, Likando Munalula, Mulima Mwiinga, Rosemary Soko, and to our community workers John Mbewe, Mora Minyoi, and Richard Mulaka.
The Supplementary Material for this article can be found online at: https://www.frontiersin.org/articles/10.3389/fmed.2022.849677/full#supplementary-material
1. de Onis M, Branca F. Childhood stunting: a global perspective. Matern Child Nutr. (2016) 12(Suppl. 1):12–26. doi: 10.1111/mcn.12231
2. Black RE, Victora CG, Walker SP, Bhutta ZA, Christian P, de Onis M, et al. Maternal and child undernutrition and overweight in low-income and middle-income countries. Lancet. (2013) 382:427–51. doi: 10.1016/S0140-6736(13)60937-X
3. Olofin I, McDonald CM, Ezzati M, Flaxman S, Black RE, Fawzi WW, et al. Associations of suboptimal growth with all-cause and cause-specific mortality in children under five years: a pooled analysis of ten prospective studies. PLoS One. (2013) 8:e64636. doi: 10.1371/journal.pone.0064636
4. Prendergast AJ, Humphrey JH. The stunting syndrome in developing countries. Paediatr Int Child Health. (2014) 34:250–65. doi: 10.1179/2046905514Y.0000000158
5. Church JA, Parker EP, Kosek MN, Kang G, Grassly NC, Kelly P, et al. Exploring the relationship between environmental enteric dysfunction and oral vaccine responses. Future Microbiol. (2018) 13:1055–70. doi: 10.2217/fmb-2018-0016
6. Marie C, Ali A, Chandwe K, Petri WA, Kelly P. Pathophysiology of environmental enteric dysfunction and its impact on oral vaccine efficacy. Mucosal Immunol. (2018) 11:1290–8. doi: 10.1038/s41385-018-0036-1
7. Panjwani A, Heidkamp R. Complementary feeding interventions have a small but significant impact on linear and ponderal growth of children in low- and middle-income countries: a systematic review and meta-analysis. J Nutr. (2017) 147:2169S–78S. doi: 10.3945/jn.116.243857
8. Hambidge KM, Westcott JE, Garces A, Figueroa L, Goudar SS, Dhaded SM, et al. A multicountry randomized controlled trial of comprehensive maternal nutrition supplementation initiated before conception: the women first trial. Am J Clin Nutr. (2019) 109:457–69. doi: 10.1093/ajcn/nqy228
9. Owino V, Ahmed T, Freemark M, Kelly P, Loy A, Manary M, et al. Environmental enteric dysfunction and growth failure/stunting in global child health. Pediatrics. (2016) 138:e20160641. doi: 10.1542/peds.2016-0641
10. Crane RJ, Jones KD, Berkley JA. Environmental enteric dysfunction: an overview. Food Nutr Bull. (2015) 36(1 Suppl.):S76–87. doi: 10.1177/15648265150361S113
11. Chen RY, Mostafa I, Hibberd MC, Das S, Mahfuz M, Naila NN, et al. A microbiota-directed food intervention for undernourished children. N Engl J Med. (2021) 384:1517–28. doi: 10.1056/NEJMoa2023294
12. Amadi B, Besa E, Zyambo K, Kaonga P, Louis-Auguste J, Chandwe K, et al. Impaired barrier function and autoantibody generation in malnutrition enteropathy in Zambia. EBioMedicine. (2017) 22:191–9. doi: 10.1016/j.ebiom.2017.07.017
13. Cook GC. Malabsorption in the tropics. In: Cook GC, editors. Tropical Gastroenterology. Oxford: OUP (1980). p. 271–324.
14. Salazar-Lindo E, Allen S, Brewster DR, Elliott EJ, Fasano A, Phillips AD, et al. Latin American society for pediatric gastroenterology, hepatology and nutrition. intestinal infections and environmental enteropathy: working group report of the second world congress of pediatric gastroenterology, hepatology, and nutrition. J Pediatr Gastroenterol Nutr. (2004) 39(Suppl. 2):S662–9. doi: 10.1097/00005176-200406002-00013
15. Ozen A, Comrie WA, Ardy RC, Domínguez Conde C, Dalgic B, Beser ÖF, et al. CD55 deficiency, early-onset protein-losing enteropathy, and thrombosis. N Engl J Med. (2017) 377:52–61. doi: 10.1056/NEJMoa1615887
16. Lee RG, Nakamura K, Tsamandaas AC, Abu-Elmagd K, Furukawa H, Hutson WR, et al. Pathology of human intestinal transplantation. Gastroenterology. (1996) 110:1820–34. doi: 10.1053/gast.1996.v110.pm8964408
17. Umetsu SE, Brown I, Langner C, Lauwers GY. Autoimmune enteropathies. Virchows Arch. (2018) 472:55–66. doi: 10.1007/s00428-017-2243-7
18. van Wanrooij RLJ, Bontkes HJ, Neefjes-Borst EA, Mulder CJ, Bouma G. Immune-mediated enteropathies: from bench to bedside. J Autoimmun. (2021) 118:102609. doi: 10.1016/j.jaut.2021.102609
19. Scarpignato C, Bjarnason I. Drug-induced small bowel injury: a challenging and often forgotten clinical condition. Curr Gastroenterol Rep. (2019) 21:55. doi: 10.1007/s11894-019-0726-1
20. Duval L, Habes S, Chatellier T, Guerzider P, Bossard C, Masliah C, et al. Nivolumab-induced celiac-like enteropathy in patient with metastatic renal cell carcinoma: case report and review of the literature. Clin Case Rep. (2019) 7:1689–93. doi: 10.1002/ccr3.2342
21. Lu L, Li W, Chen L, Su Q, Wang Y, Guo Z, et al. Radiation-induced intestinal damage: latest molecular and clinical developments. Future Oncol. (2019) 15:4105–18. doi: 10.2217/fon-2019-0416
22. Yamaguchi S, Yanai S, Nakamura S, Kawasaki K, Eizuka M, Uesugi N, et al. Immunohistochemical differentiation between chronic enteropathy associated with SLCO2A1 gene and other inflammatory bowel diseases. Intest Res. (2018) 16:393–9. doi: 10.5217/ir.2018.16.3.393
23. Liu T-C, VanBuskirk K, Ali A, Kelly P, Holtz LR, Yilmaz OH, et al. A novel histological index for evaluation of environmental enteric dysfunction identifies geographic-specific features of enteropathy among children with suboptimal growth. PLoS Negl Trop Dis. (2020) 14:e0007975. doi: 10.1371/journal.pntd.0007975
24. Ford RP, Menzies IS, Phillips AD, Walker-Smith JA, Turner MW. Intestinal sugar permeability: relationship to diarrhoeal disease and small bowel morphology. J Pediatr Gastroenterol Nutr. (1985) 4:568–74.
25. Baker SJ. Subclinical intestinal malabsorption in developing countries. Bull World Health Organ. (1976) 54:485–94.
26. Kelly P, Besa E, Zyambo K, Louis-Auguste J, Lees J, Banda T, et al. Endomicroscopic and transcriptomic analysis of impaired barrier function and malabsorption in environmental enteropathy. PLoS Negl Trop Dis. (2016) 10:e0004600. doi: 10.1371/journal.pntd.0004600
27. Amadi B, Zyambo K, Chandwe K, Besa E, Mulenga C, Mwakamui S, et al. Adaptation of the small intestine to microbial enteropathogens in Zambian children with stunting. Nat Microbiol. (2021) 6:445–54. doi: 10.1038/s41564-020-00849-w
28. Jansen GR, O’Deen L, Tribelhorn RE, Harper JM. The calorie densities of gruels made from extruded corn-soy blends. Food Nutr Bull. (1981) 3:1–6.
29. Chandwe K, Amadi B, Chipunza M, Zyambo M, Kelly P. Safety and ethics in endoscopic studies in children: evidence from the BEECH study in Zambia. J Trop Pediatr. (2021) 67:fmaa074. doi: 10.1093/tropej/fmaa074
30. Kiesslich R, Duckworth CA, Moussata D, Gloeckner A, Lim LG, Goetz M, et al. Local barrier dysfunction identified by confocal laser endomicroscopy predicts relapse in inflammatory bowel disease. Gut. (2012) 61:1146–53. doi: 10.1136/gutjnl-2011-300695
31. Penna FJ, Hill ID, Kingston D, Robertson K, Slavin G, Shiner M. Jejunal mucosal morphometry in children with and without gut symptoms and in normal adults. J Clin Path. (1981) 34:386–92. doi: 10.1136/jcp.34.4.386
32. Campbell DI, Murch SH, Elia M, Sullivan PB, Sanyang MS, Jobarteh B, et al. Chronic T cell-mediated enteropathy in rural west African children: relationship with nutritional status and small bowel function. Pediatr Res. (2003) 54:306–11. doi: 10.1203/01.PDR.0000076666.16021.5E
33. VanDussen KL, Stojmirović A, Li K, Liu TC, Kimes PK, Muegge BD, et al. Abnormal small intestinal epithelial microvilli in patients with Crohn’s disease. Gastroenterology. (2018) 155:815–28. doi: 10.1053/j.gastro.2018.05.028
34. Schenk EA, Samloff IM, Klipstein FA. Pathogenesis of jejunal mucosal alterations: synechia formation. Am J Pathol. (1967) 50:523–31.
35. Kummerlowe C, Wallach T, Mwakamui S, Hughes TK, Mulugeta N, Mudenda V, et al. Single-cell profiling of environmental enteropathy reveals signatures of epithelial remodeling and immune activation in severe disease. bioRxiv [Preprint]. (2021). doi: 10.1101/2021.04.11.439202
36. Crowe PT, Marsh MN. Morphometric analysis of small intestinal mucosa IV. Determining cell volumes. Virchows Archiv A Pathol Anat. (1993) 422:459–66. doi: 10.1007/BF01606454
37. Shiner M, Redmond AOB, Hansen JDL. The jejunal mucosa in protein-energy malnutrition: a clinical, histological and ultrastructural study. Exp Molec Pathol. (1973) 19:61–78. doi: 10.1016/0014-4800(73)90041-5
38. Schenk EA, Samloff IM, Klipstein FA. Morphology of small bowel biopsies. Am J Clin Nutr. (1968) 21:944–61. doi: 10.1093/ajcn/21.9.944
39. Dickson BC, Streutker CJ, Chetty R. Coeliac disease: an update for pathologists. J Clin Path. (2006) 59:1008–16. doi: 10.1136/jcp.2005.035345
40. Grosse AS, Pressprich MF, Curley LB, Hamilton KL, Margolis B, Hildebrand JD, et al. Cell dynamics in fetal intestinal epithelium: implications for intestinal growth and morphogenesis. Development. (2011) 138:4423–32. doi: 10.1242/dev.065789
41. Walton KD, Mishkind D, Riddle MR, Tabin CJ, Gumucio DL. Blueprint for an intestinal villus: species-specific assembly required. Wiley Interdiscip Rev Dev Biol. (2018) 7:e317. doi: 10.1002/wdev.317
42. Lim LG, Neumann J, Hansen T, Goetz M, Hoffman A, Neurath MF, et al. Confocal endomicroscopy identifies loss of local barrier function in the duodenum of patients with Crohn’s disease and ulcerative colitis. Inflamm Bowel Dis. (2014) 20:892–900. doi: 10.1097/MIB.0000000000000027
43. Brunser O, Castillo C, Araya M. Fine structure of the small intestinal mucosa in infantile marasmic malnutrition. Gastroenterology. (1976) 70:495–507.
44. Das B, Okamoto K, Rabalais J, Young JA, Barrett KE, Sivagnanam M. Aberrant epithelial differentiation contributes to pathogenesis in a murine model of congenital tufting enteropathy. Cell Mol Gastroenterol Hepatol. (2021) 12:1353–71. doi: 10.1016/j.jcmgh.2021.06.015
45. Greenson JK. The biopsy pathology of non-coeliac enteropathy. Histopathology. (2015) 66:29–36. doi: 10.1111/his.12522
46. Menzies IS, Zuckerman MJ, Nukajam WS, Somasundaram SG, Murphy B, Jenkins AP, et al. Geography of intestinal permeability and absorption. Gut. (1999) 44:483–9. doi: 10.1136/gut.44.4.483
47. Kosek MN, Mal-Ed Network Investigators. Causal pathways from enteropathogens to environmental enteropathy: findings from the MAL-ED birth cohort study. EBiomedicine. (2017) 18:109–17. doi: 10.1016/j.ebiom.2017.02.024
48. Denno DM, VanBuskirk K, Nelson ZC, Musser CA, Hay Burgess DC, Tarr PI. Use of the lactulose to mannitol ratio to evaluate childhood environmental enteric dysfunction: a systematic review. Clin Infect Dis. (2014) 59(Suppl. 4):S213–9. doi: 10.1093/cid/ciu541
49. Zyambo K, Hodges P, Chandwe K, Mweetwa M, Westcott J, Krebs N, et al. Reduced fractional absorption of zinc in children with environmental enteropathy in Zambia. J Ped Gastroenterol Nutr. (2022) 74:277–83. doi: 10.1097/MPG.0000000000003292
50. Kelly P, Amadi B, Chandwe K, Besa E, Zyambo K, Chama M, et al. Gene expression profiles compared in environmental and malnutrition enteropathy in Zambian children and adults. EBiomedicine. (2021) 70:103509. doi: 10.1016/j.ebiom.2021.103509
51. Phillips AD, Avigad S, Sacks J, Rice SJ, France NE, Walker-smith J, et al. Microvillous surface area in secondary disaccharidase deficiency. Gut. (1980) 21:44–8. doi: 10.1136/gut.21.1.44
52. Keusch GT, Duggan C, Guerrant RL, Lavery JV, Tarr PI, Ward HD, et al. Implications of acquired environmental enteric dysfunction for growth and stunting in infants and children living in low- and middle-income countries. Food Nutr Bull. (2013) 34:357–64. doi: 10.1177/156482651303400308
53. Guerrant RL, DeBoer MD, Moore SR, Scharf RJ, Lima AAM. The impoverished gut—a triple burden of diarrhoea, stunting and chronic disease. Nat Rev Gastroenterol Hepatol. (2013) 10:220–9. doi: 10.1038/nrgastro.2012.239
54. Prendergast A, Kelly P. Enteropathies in the developing world; neglected effects on global health. Am J Trop Med Hyg. (2012) 86:756–63. doi: 10.4269/ajtmh.2012.11-0743
55. Zambruni M, Cabada MM, Morales ML, Mitreva M, Rosa BA, Acosta GJ, et al. Stunting is preceded by intestinal mucosal damage and microbiome changes and is associated with systemic inflammation in a cohort of Peruvian infants. Am J Trop Med Hyg. (2019) 101:1009–17. doi: 10.4269/ajtmh.18-0975
56. Bevins CL, Salzman NH. Paneth cells, antimicrobial peptides and maintenance of intestinal homeostasis. Nat Rev Microbiol. (2011) 9:356–68. doi: 10.1038/nrmicro2546
Keywords: environmental enteropathy, environmental enteric dysfunction (EED), microvilli, Africa, malnutrition
Citation: Mulenga C, Sviben S, Chandwe K, Amadi B, Kayamba V, Fitzpatrick JAJ, Mudenda V and Kelly P (2022) Epithelial Abnormalities in the Small Intestine of Zambian Children With Stunting. Front. Med. 9:849677. doi: 10.3389/fmed.2022.849677
Received: 06 January 2022; Accepted: 22 February 2022;
Published: 16 March 2022.
Edited by:
Sean Spencer, Stanford University, United StatesReviewed by:
Jeffrey Donowitz, Children’s Hospital of Richmond at VCU, United StatesCopyright © 2022 Mulenga, Sviben, Chandwe, Amadi, Kayamba, Fitzpatrick, Mudenda and Kelly. This is an open-access article distributed under the terms of the Creative Commons Attribution License (CC BY). The use, distribution or reproduction in other forums is permitted, provided the original author(s) and the copyright owner(s) are credited and that the original publication in this journal is cited, in accordance with accepted academic practice. No use, distribution or reproduction is permitted which does not comply with these terms.
*Correspondence: Paul Kelly, bS5wLmtlbGx5QHFtdWwuYWMudWs=
Disclaimer: All claims expressed in this article are solely those of the authors and do not necessarily represent those of their affiliated organizations, or those of the publisher, the editors and the reviewers. Any product that may be evaluated in this article or claim that may be made by its manufacturer is not guaranteed or endorsed by the publisher.
Research integrity at Frontiers
Learn more about the work of our research integrity team to safeguard the quality of each article we publish.