- 1Department of Laboratory Medicine, The First Affiliated Hospital of Chongqing Medical University, Chongqing, China
- 2Department of Burn and Plastic Surgery, The First Affiliated Hospital of Chongqing Medical University, Chongqing, China
- 3Chongqing Testing and lnspection Center for Medical Devices, Chongqing, China
- 4Division of Allergy and Clinical Immunology, Brigham and Women's Hospital and Harvard Medical School, Boston, MA, United States
- 5Department of Laboratory Medicine, The Affiliated Children's Hospital of Chongqing Medical University, Chongqing, China
The dissemination of carbapenemase-producing Enterobacterales (CPE) is worrisome given their scarce treatment options. CPE bloodstream infections (BSIs) had a high mortality rate in adults, and there was little data on pediatric CPE-BSIs around the world. We comprehensively explored the differences in the clinical and microbiological characteristics between pediatric and adult CPE-BSIs. Forty-eight pediatric and 78 adult CPE-BSIs cases were collected. All-cause 30 day-mortality in children with CPE-BSIs (14.6%, 7/48) was significantly lower than that in adult patients (42.3%, 33/78, p = 0.001). The subgroup in adults empirically treated with tigecycline as an active drug displayed a significantly higher 30-days crude mortality (63.3%, 19/30) than the subgroup treated without tigecycline (29.2%, 14/48, p = 0.003). K. pneumoniae was the most prevalent species in both the pediatric (45.8%, 22/48) and adult populations (64.1%, 50/78), with discrepant carbapenemase genes in each population: 95.4% (21/22) of the pediatric K. pneumoniae isolates carried blaNDM, while 82.0% (41/50) of the adult strains harbored blaKPC. The ratio of E. coli in children (37.5%) was significantly higher than that in adults (12.8%, p = 0.002). In both populations, the majority of E. coli expressed blaNDM, particularly blaNDM−5. With statistical significance, blaNDM was much more common in children (95.8%, 46/48) than in adults (34.6%, 27/78). The rate of multiple-heteroresistance phenotypes in children was as high as 87.5%, which was much lower in adults (57.1%). Agar dilution checkboard experiment against one pediatric carbapenemase-producing E. coli isolates showed that the combination of amikacin and fosfomycin yielded an additive effect. Overall, K. pneumoniae was the most common CPE-BSIs pathogen in both populations, with NDM-producing K. pneumoniae and KPC-producing ST11 K. pneumoniae being the most prevalent species in children and adults, respectively. E. coli was more prevalent in children than in adults, yet blaNDM−5 was the most common carbapenem-resistant mechanism in E. coli in both populations. The wide range of multiple-heteroresistance combination traits found in different pathogen species from different host populations should provide a good foundation for future combination therapy design. Further investigations from more CPE isolates of various species are needed to evaluate the possible in vitro partial synergy of the amikacin and fosfomycin combination.
Introduction
Carbapenemase-producing Enterobacterales (CPE), a predominant member of carbapenem-resistant Enterobacterales (CRE), pose a great threat to global health (1, 2). Bloodstream infections (BSIs) due to CPE (CPE-BSIs) tend to be persistent or recurrent; furthermore, the mortality of such infections can even reach 50% in children and 65% in adults (3–5). However, global CPE epidemics demonstrated important regional differences concerning patients' baseline characteristics, clinical outcomes, and bacterial characteristics. Research findings from one region might not be generalisable to other regions. In addition, compared to adults, little is known about the treatment strategies used by children with CPE-BSIs.
Various carbapenemases along with other resistance mechanisms contribute to the deleterious effect on CPE management. Typical carbapenemases include Klebsiella pneumoniae carbapenemase (KPC), Metallo-β-lactamases (MBLs), and Oxacillinase-48 (OXA-48), as representatives of Ambler class A, Ambler class B, and Ambler class D, respectively (6, 7). There are currently no active MBLs inhibitors in clinics, particularly for the subtype New-Delhi Metallo-lactamase (NDM) found in many Enterobacterales species (8, 9).
Timely active antibiotic treatment can improve the outcomes (10, 11), but there are few treatment options for CPE-BSIs. Antibiotic therapy now rely mostly on medications like colistin, tigecycline, fosfomycin, and aminoglycosides, which are all threatened by developing resistance and plagued by considerable side effects (12, 13). Moreover, both colistin and tigecycline are known as last-resort antibiotics used mainly to treat MDR infections. New antibiotics show superior bactericidal effects, for instance, ceftazidime-avibactam (CAZ-AVI) targeting KPC and OXA-48 producers (14), and aztreonam-avibactam (ATM-AVI) active against KPC, MBLs, AmpC, and OXA-48 producers (15), yet resistance to these drugs is still inevitable (16, 17). Combination therapy, pathogen virulence-targeting method, host immunity modulation strategy, and phage therapy are some of the most promising therapeutic strategies for combating antibacterial resistance (6, 18), among which combination therapy is strongly recommended to fight against highly resistant infections as it can not only slow down the acquisition of resistance but also improve bactericidal efficacy. Thus, efficient combination therapy is recommended to combat CPE-BSIs, especially in children for whom anti-infection treatments are mostly extrapolated from adults, and most last-resort drugs such as aminoglycosides are not recommended (19). Overall, a thorough understanding of the mechanistic principles governing antibacterial drug resistance is fundamental for the development of novel combination therapeutics to combat current and emerging bacterial threats. However, as most of the combination therapies were clinical experience-directed or observation-directed, with controversial results and few systematic experiments and clinical trials, combination therapy is still lacking solid ground (10). As drug combinations targeting multiple-heteroresistance mechanisms exhibited excellent activities (20), multiple-heteroresistance may be a critical foundation for antibiotic combination therapy. However, the effect of combination therapy is still sporadic with such a theoretical basis. Notably, combination therapy involves the drug repurposing and regrouping of the existing antimicrobial agents to provide a synergistic approach for management of infectious diseases by obtaining broad-spectrum coverage and minimizing resistance development, thus the rationale behind the choice of combination therapy is that the antimicrobials will have a synergistic effect when given together. Hence, it is not that any two or three antibiotics showing multiple-heteroresistance can be used in combination. Most medications are previously known to kill bacteria, and antimicrobials are generally classed as bacteriostatic or bactericidal agents. Combining a bacteriostatic (e.g., doxycycline) and a bactericidal (e.g., β-lactam) medicine might theoretically limit the growth required for the latter's mechanism of action. Therefore, one step in developing multiple-heteroresistance-based new combinations includes theoretical scrutiny and subsequent in vitro testing for synergy through the dilution checkerboard method.
Though dissemination of CPE in adults and related antibiotic treatments for CPE-BSIs are well-explored, there is still a knowledge gap in children with CPE-BSIs. Understanding the distinctions between children and adult CPE-BSIs would not only help to complete a full research of the mechanisms involved in CPE dissemination in children, but it will also help to enhance treatment options in both groups.
Here, we explored the differences between children and adults with CPE-BSIs mainly from two aspects: (1) clinical characteristics, and (2) microbiological characteristics. Besides, we investigated the heteroresistance phenotypes in all the CPE-BSIs isolates from both populations and evaluated the inspiration on the optimization of the treatment strategies by exploiting multiple-heteroresistance.
Methods
Patients and Strains
CRE is defined as Enterobacterales that are resistant to at least one carbapenem antibiotic (ertapenem, meropenem, doripenem, or imipenem) or produce a carbapenemase, according to the Centers for Disease Control and Prevention in the USA (https://www.cdc.gov/hai/organisms/cre/technical-info.html#Definition). Both adult and pediatric patients with mono-microbial CRE-BSIs from three tertiary teaching hospitals in the medical center of Chongqing Medical University from 2015 to 2020 were collected. Both the laboratory information system and the hospital information system were used to collect all relevant data, including demographics, underlying diseases, antimicrobial therapeutics, and microbiological data. Then the CRE-BSIs isolates carrying any of the tested carbapenemase genes (blaKPC, blaNDM, blaVIM, blaIMP, blaOXA−48−like) as confirmed by polymerase chain reaction (PCR) and DNA sequencing were recognized as CPE-BSIs strains, and patients with CPE-BSIs were included in this study.
Pediatric patients and corresponding strains were from the Affiliated Children's Hospital of Chongqing Medical University, while adult patients and related strains were from both the First Affiliated and the Second Affiliated Hospital of Chongqing Medical University. The above-mentioned three hospitals were large tertiary teaching hospitals from one medical center in Chongqing, China.
The VITEK MS (BioMerieux) was used to identify all CRE strains isolated from blood, which were then stored at −80°C in the laboratory.
Detection of Carbapenemase-Encoding Genes and Extended-Spectrum β-Lactamases
Total DNA was extracted by the boiling centrifugation method. Briefly, single colonies were picked from an overnight culture of each isolate, resuspended in 200 ul of sterile distilled water, and boiled at 100°C for 10 min. After centrifugation at 15,000 × g for 15 min, supernatants were collected and stored at −20°C. The potential presence of both the carbapenemase genes (blaKPC, blaNDM, blaVIM, blaIMP, blaOXA−48−like) and the ESBLs genes (blaSHV, blaTEM, blaCTXM) was detected by PCR as described previously (21), and were further confirmed by DNA sequencing. The carbapenemase genes' primers were designed to amplify as many variants as possible. For example, the primers for blaNDM covered blaNDM−1~blaNDM−31 and blaNDM−34~blaNDM−41; the primers for blaKPC covered blaKPC−2~blaKPC−8, blaKPC−10~blaKPC−70, blaKPC−72~blaKPC−75, blaKPC−78~blaKPC−82, blaKPC−84~blaKPC−88, blaKPC−90~blaKPC−91, blaKPC−94~blaKPC−98, blaKPC−102~blaKPC−108. Therefore, blaNDM−1~blaNDM−31, blaNDM−34~blaNDM−41, blaKPC−2~blaKPC−8, blaKPC−10~blaKPC−70, blaKPC−72~blaKPC−75, blaKPC−78~blaKPC−82, blaKPC−84~blaKPC−88, blaKPC−90~blaKPC−91, blaKPC−94~blaKPC−98, and blaKPC−102~blaKPC−108 were screened.
Multi-Locus Sequence Typing
MLST analyses were performed on Klebsiella pneumoniae, Escherichia coli, Enterobacter cloacae and Citrobacter freudii, according to the protocols established by BIGSdb-Pasteur (https://bigsdb.pasteur.fr/index.html) and PubMLST (https://pubmlst.org/).
Broth Microdilution Methods
Minimum inhibitory concentrations (MICs) of ertapenem, meropenem, imipenem, ceftazidime, ceftazidime-avibactam, aztreonam, aztreonam-avibactam, polymyxin B, and tigecycline were established by broth microdilution methods recommended by the Clinical and Laboratory Standards Institute (CLSI) (22). E. coli ATCC25922 was employed as the control strain. The MIC breakpoints of tigecycline and polymyxin B were from EUCAST (23), and the others were from CLSI.
Disk Diffusion Methods
According to CLSI (22), overnight cultures on Columbia blood agar were suspended in 0.9% saline to achieve 0.5 McFarland units (~1.5 × 108 CFU/ml), then the inoculums were spread onto Mueller-Hinton (MH) plates by sterile cotton swabs, and disks were applied by tweezers or dispensers. The category of the disks (Oxoid) used was as follows: ceftriaxone (CRO, 30 μg), cefepime (FEP, 30 μg), ceftazidime (CAZ, 30 μg), piperacillin/tazobactam (TZP, 100 ug/10 μg), ceftazidime-avibactam (CAZ-AVI, 30 μg/20 μg), aztreonam (ATM, 30 μg), ertapenem (ETP, 10 μg), imipenem (IPM, 10 μg), meropenem (MEM, 10 μg), tobramycin (TOB, 10 μg), amikacin (AK, 30 μg), tetracycline (TE, 30 μg), minocycline (MH, 30 μg), ciprofloxacin (CIP, 5 μg), fosfomycin (FOS, 200 μg), tigecycline (TGC, 15 μg), polymyxin B (PB, 300 μg), colistin (CT, 10 μg). Plates were then incubated at 37°C for 48 h, during which time the diameters of the inhibition zones and heteroresistance phenotypes were measured and recorded.
Dual disks diffusion tests were carried out according to the same protocol, whereas the distance of the two disks placed was half of the sum of diameters of the inhibition zones of the two disks.
Population Analysis Profile
PAP analyses were conducted as described previously (24). Two or three colonies from the overnight cultures on Columbia blood agar were suspended in 1 ml MH broth respectively, and incubated overnight at 37°C. After that, each culture was diluted 1:1000 in PBS buffer, and 1 μl of each was inoculated into a vial with 1 ml of MH broth. After overnight growth, 10−1 to 10−4 dilutions in PBS buffer were prepared. Five microlitre from each suspension (0~10−4) was spread onto the freshly prepared MH agar plates with or without increasing amounts of antibiotics (FOS and AK). The concentration of antibiotics was from ¼ × MIC to 8 × MIC (2 fold increments). All plates were incubated overnight at 37°C and colonies were counted to determine the frequency of bacteria growing at each antibiotic concentration. Strains with colonies which appeared on 4 × MIC or 8 × MIC plates at a frequency of 10−7 or higher were recognized as heteroresistant strains (20).
E-Test
Overnight cultures on Columbia blood agar were suspended in 0.9% saline to achieve 0.5 McFarland units (~1.5 × 108 CFU/ml), and then the inoculum was spread onto MH plates by sterile cotton swabs, and equivalent fosfomycin (including 25 μg/ml G-6-P) and amikacin MIC Test Strips (Liofilchem) were applied by tweezers. Plates were subsequently incubated for 24 h at 37°C, and MICs were read after 24 h and 48 h. Synergy tests were carried out according to the protocol of the MTSTM Synergy Applicator System of Liofilchem (25).
Agar Dilution Checkboard Assay
A series of agar plates (containing 25 mg/L G-6-P) with or without mixed antibiotics (fosfomycin and amikacin) were prepared in the form of 8 × 8 matrix, and the concentrations of FOS from right to left and AK from top to bottom ranged from 1/16 × MIC to 4 × MIC (2-fold increments). Overnight cultures at 0.5 McFarland units were diluted 1:10 in 0.9% saline, and then 2 μl of the suspensions were inoculated onto the plates. All plates were incubated at 37°C for 16–20 h. New MICs of each isolate to each drug alone or drug combinations were determined. The Fraction Inhibitory Concentration Index (FICI) values were calculated according to the following formula: FICI = (MIC drug A with drug B/MIC drug A alone) + (MIC drug B with drug A/MIC drug B alone). The FICI results were interpreted as synergistic (≤ 0.5), additive (>0.5 to ≤ 1), indifferent (>1 to <4) or antagonism (≥ 4).
Statistical Analysis
The age of adults was presented as average, and the rest variables in the study were presented as absolute frequencies or percentages. The clinic-related categorical variables in both populations were compared using χ2 test or Fisher's exact test, as appropriate. The percentages of various species distribution and resistance mechanisms in both populations were compared by Fisher's exact test. The Kaplan-Meier analysis was performed for the survival analysis. A two-tailed P-value of ≤ 0.05 was considered statistically significant. All statistical analysis was finished by SPSS25.0 (IBM).
Results
Clinical Characteristics of Pediatric and Adult Patients With CPE-BSIs
Baseline information (Table 1) 48 CRE isolates carrying any of the tested carbapenemase genes were identified as CPE among 52 cases of children with CRE-BSIs collected, and 48 cases of pediatric patients with CPE-BSIs were thus included in the study. Forty-two (87.5%) pediatric patients with CPE-BSIs were under 1 year old (neonates), and 30 (62.5%) children were male. Meanwhile, 39 (81.3%) pediatric patients were with nosocomial infections.
In the meantime, the study included 78 adult patients with CPE-BSIs. The mean age of the patients was 58 years old, with a minimum age of 18 and a maximum age of 88. Fifty-five (70.5%) were male, and 61 (78.2%) were with hospital-acquired infections.
Treatment and outcomes: All-cause mortality within 30 days from the onset of CPE-BSIs of children was 14.6% (7/48), which was significantly lower than that of the adults (42.3%, 33/78, p = 0.001) (Figure 1A).
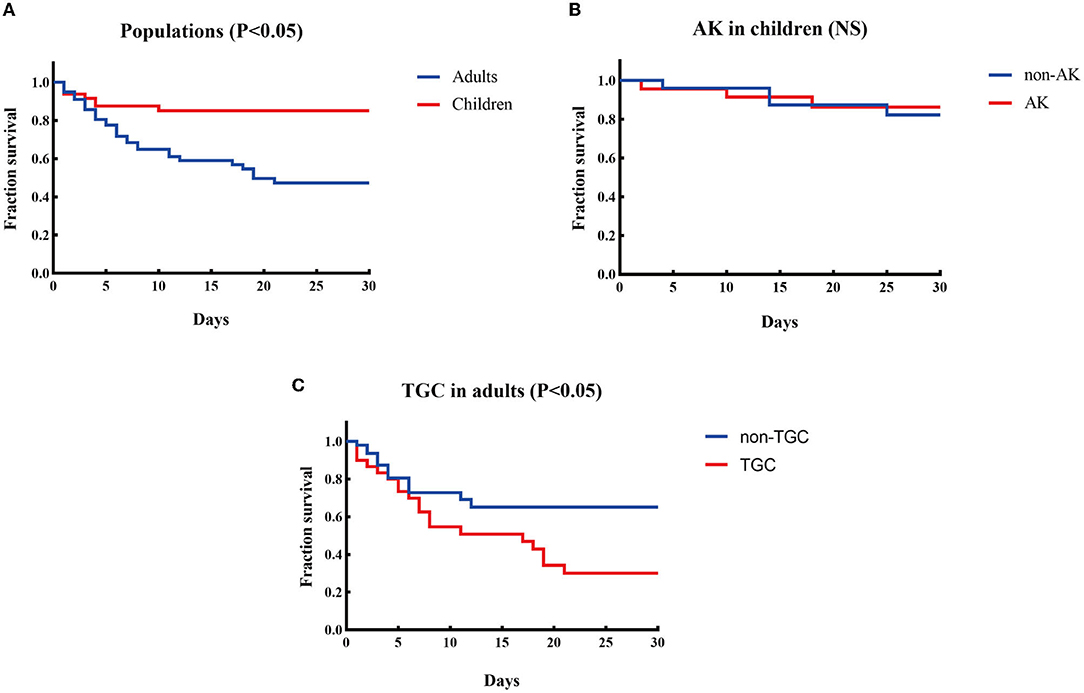
Figure 1. Kaplan-Meier curves of different factors and survival at 30 days in patients with CPE-BSIs. (A) The difference of 30-day crude mortality between adults and children. (B) The difference in crude mortality after 30 days between children who received AK as an active drug empirically and those who did not. AK, amikacin. (C) The difference of 30-day crude mortality between the adults who received TGC as an active drug empirically and the adults who didn't. TGC, tigecycline; NS, non-significant.
Amikacin was the most commonly prescribed drug in children's treatment (45.8%, 22/48), with 19 monotherapy and 3 combination therapy. Besides, 22 children received amikacin as the active drug. There was no significant difference in the unadjusted 30-day-crude mortality between the subgroup who received amikacin and the subgroup who did not (Figure 1B).
On the contrary, tigecycline was the most frequently prescribed drug in adults (38.5%, 30/78), and tigecycline was active in all the corresponding strains in vitro. The mainstay of combination therapies was tigecycline, but the other drug was not always fixed. Surprisingly, the subgroup given tigecycline as an active drug had a higher 30-day crude mortality rate (63.3%, 19/30) than the subgroup given no tigecycline (29.2%, 14/48, P = 0.003) (Figure 1C).
Microbiological Characteristics of Pediatric and Adult Patients With CPE-BSIs
K. pneumoniae was the most prevalent species in both populations, with discrepant carbapenemase genes in each population. K. pneumoniae was discovered to be the most common species in both patient groups, accounting for 45.8% (22/48) and 64.1% (50/78) of CPE-BSI isolates in the pediatric and adult populations, respectively, nevertheless with discrepant carbapenemase genes in each population: In children, 95.4% (21/22) of the K. pneumoniae isolates carried blaNDM, with 11 being blaNDM−1 and the other 10 being blaNDM−5; By contrast, 82.0% (41/50) of the K. pneumoniae strains in adults carried blaKPC, with all being subtype blaKPC−2 (Figures 2C,D).
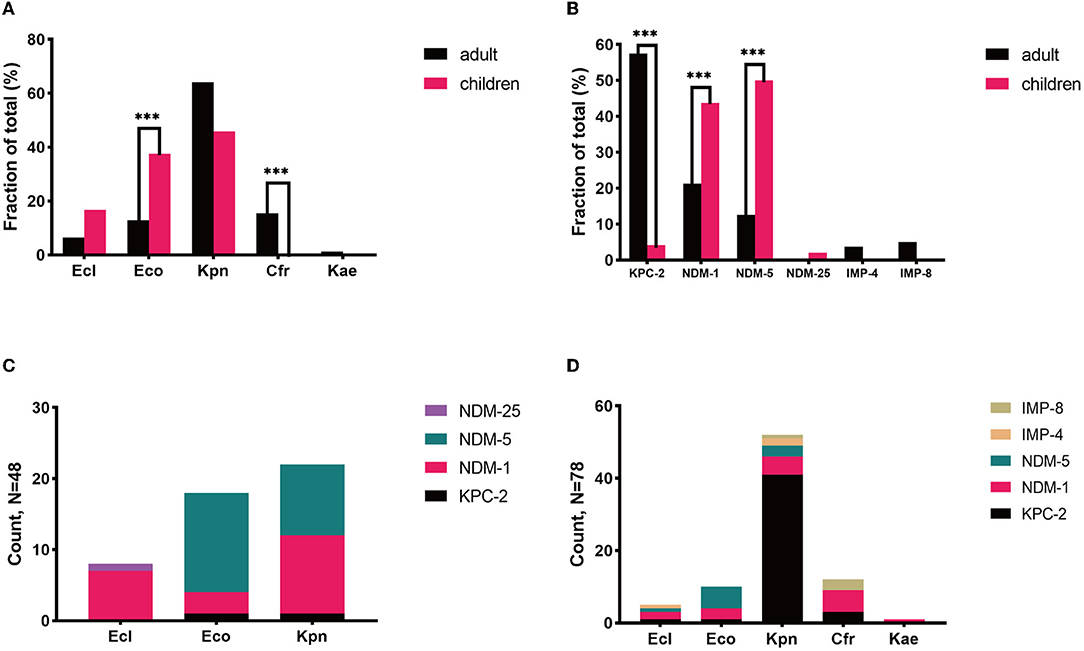
Figure 2. Microbiological characteristics of pediatric and adult CPE-BSIs patients. (A) The differences of species distribution in both populations. (B) The differences of carbapenemase-encoding genes distribution in both populations. (C) The carbapenemase-encoding genes distribution in the species from pediatric patients. (D) The carbapenemase-encoding genes distribution in the species from adult patients. ***P < 0.05. Kpn, K. pneumoniae; Eco, E. coli; Ecl, E. cloacae; Cfr, C. freudii; Kae, Klebsiella aerogenes.
Besides, most E. cloacae isolates in both populations carried MBLs genes, and most C. freudii strains, exclusively identified in adults, also carried MBLs.
Of note, ESBLs were prevalent in both populations. 93.7% of the CPE isolates in children co-expressed ESBLs, while 84.6% of the isolates in adults co-expressed ESBLs.
E. coli and blaNDM were of high proportion in children. While K. pneumoniae was the most prevalent species in both populations, E. cloacae were found in both pediatric and adult populations, yet C. freudii and Enterobacter aerogenes were only identified in adults. Notably, the proportion of E. coli in children (37.5%) was significantly higher than that in adults with CPE-BSIs (12.8%, p = 0.002) (Figure 2A).
While blaKPC−2 (46/78, 59.0%) was the most common carbapenemase-encoding gene in adults, blaNDM, including blaNDM−5 (24/48. 50.0%), blaNDM−1 (21/48, 43.8%), and blaNDM−25 (1/48, 2.1%), was the predominant one in children (46/48, 95.8%). Furthermore, blaNDM was much more prevalent in children (95.8%, 46/48) than in adults (34.6%, 27/78) with statistical significance (p < 0.001) (Figure 2B).
Most E. coli expressed blaNDM in both populations, especially blaNDM−5. In children, 94.4% (17/18) of the E. coli isolates carried blaNDM, with 82.4% (14/17) of blaNDM being the subtype blaNDM−5. In adults, 90.0% (9/10) of the E coli strains carried blaNDM, and 66.7% (6/9) of blaNDM was the blaNDM−5 subtype (Figures 2C,D).
MLST in Strains With blaNDM Was Complex
We further explored the possible roles sequence types (STs) of different species played during the dissemination of CPE in both populations. As shown in Figure 3, regardless of species, various STs were demonstrated to carry blaNDM. In children, 7 out of 8 ST2 E. coli and 4 out of 5 ST692 E. coli expressed blaNDM−5, while there were no superior STs in E. coli isolates harboring blaNDM in adults. In E. cloacae, similar findings have been found. Seven out of 8 ST145 E. cloacae isolates in children carried blaNDM−1, whilst no superior ST was found in E. cloacae isolates in adults. The MLSTs of blaNDM-harboring K. pneumoniae isolates in children were also varied, with no ST showing evident superiority. While 33 out of the 41 (80.5%) blaKPC−2-positive K. pneumoniae isolates in adults belonged to ST11, 7 out of the 21 (33.3%) pediatric blaNDM-carrying K. pneumoniae strains belonged to ST2407.
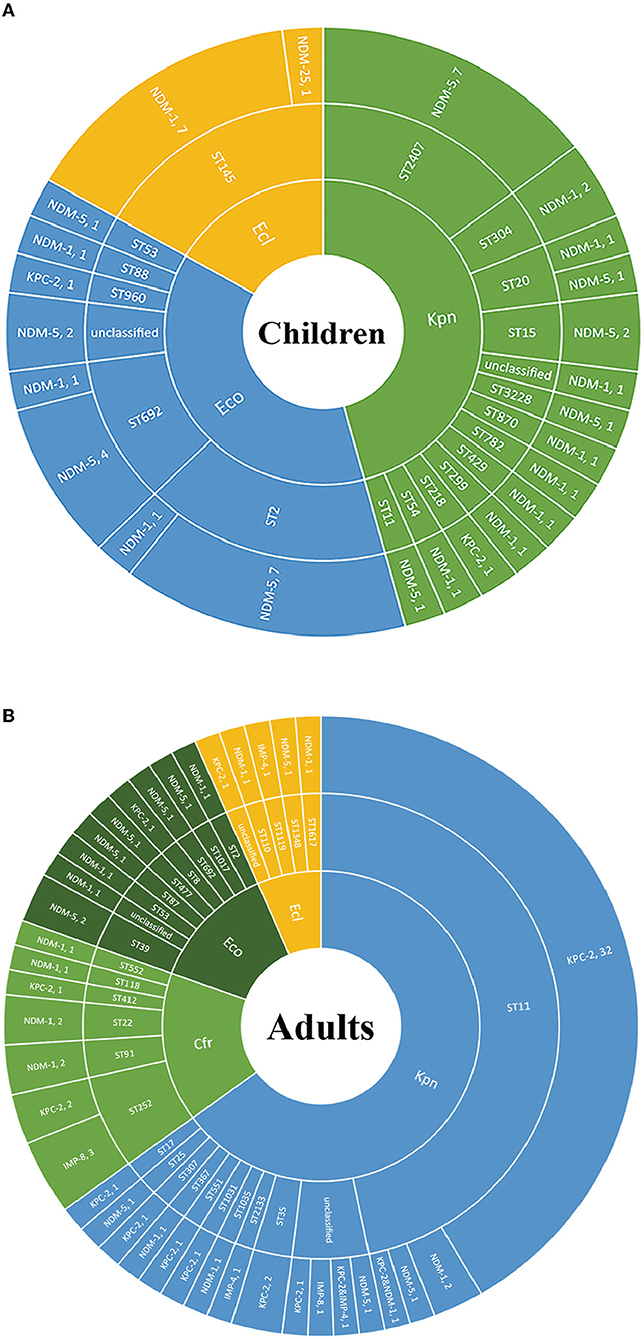
Figure 3. The Sunburst chart of the relationships among populations, species, STs, and carbapenemase distributions. The smallest circle indicates the species. The second-largest circle indicates the STs distributions in different species. The largest circle indicates the carbapenemase distributions in different STs in diverse species. The text before the comma indicates the carbapenemase's name, and the number after the comma indicates the number of the isolates with the defined carbapenemase, vice versa. (A) Children. (B) Adults. Kpn, K. pneumoniae; Eco, E. coli; Ecl, E. cloacae; Cfr, C. freudii.
ATM-AVI Exhibited Excellent in vitro Antibacterial Activity Against CPE-BSIs Isolates From Both Populations
Most of the isolates in both populations were resistant to all the three carbapenem antibiotics, including IPM, MEM, and ETP. Of note, the resistance rate of MEM was the lowest among the three. While none of the adult CPE-BSIs isolates were tigecycline-resistant, 12.5% of the pediatric strains were resistant to tigecycline. CPE-BSI isolates from children, on the other hand, had a much lower polymyxin B resistance rate (4.2%) than those from adults (14.3%). The susceptibility rates of ATM-AVI were 100.0% in children and 98.7% in adults, with the non-susceptible adult isolate being intermediate resistant. As for CAZ-AVI, while 85.4% of the pediatric isolates showed resistance, only 37.7% of isolates from adults were resistant (Table 2).
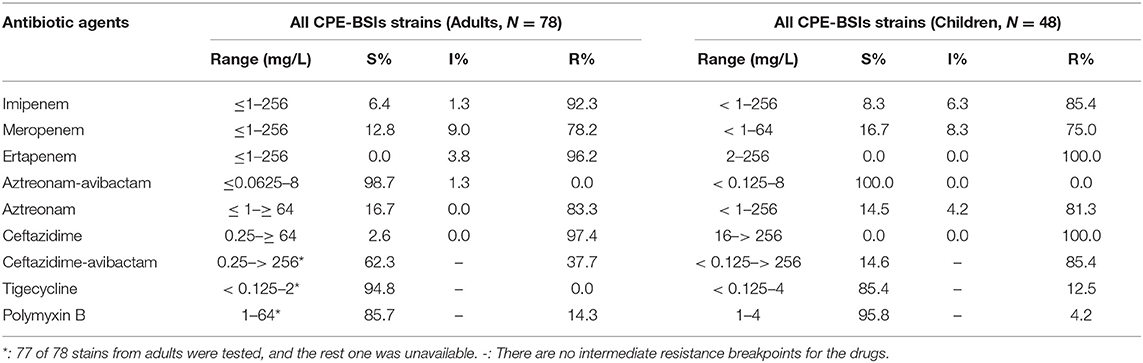
Table 2. MICs (mg/L) of the classical antibiotics against CPE-BSI isolates from children and adults.
Common Multiple-Heteroresistance Phenotype: A Possible Way to Design the Combination Therapy Strategy
We next explored the antimicrobial heteroresistance phenotypes in CPE-BSIs isolates to find the possible ways to design a combination therapy strategy.
Heteroresistance phenotypes were respectively observed for 83.3% (15/18) and 88.9% (16/18) of the tested antibiotics in pediatric and adult CPE-BSIs isolates. In children, the proportion of isolates with heteroresistance ranged from 93.8% for FOS to 2.1% for CT, while in adults, it was 74.0% for FOS and 1.3% for TE (Table 3). Furthermore, multiple-heteroresistance phenotypes were frequently observed in both populations (Figure 4).
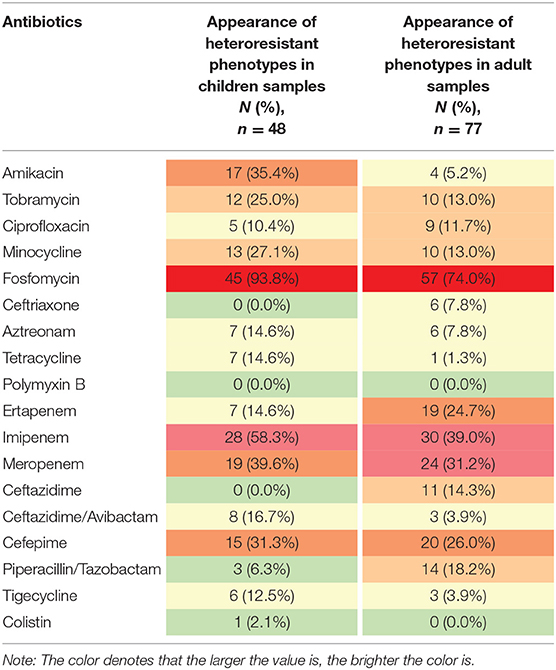
Table 3. The frequencies of heteroresistance phenotypes to different antibiotics in both populations.
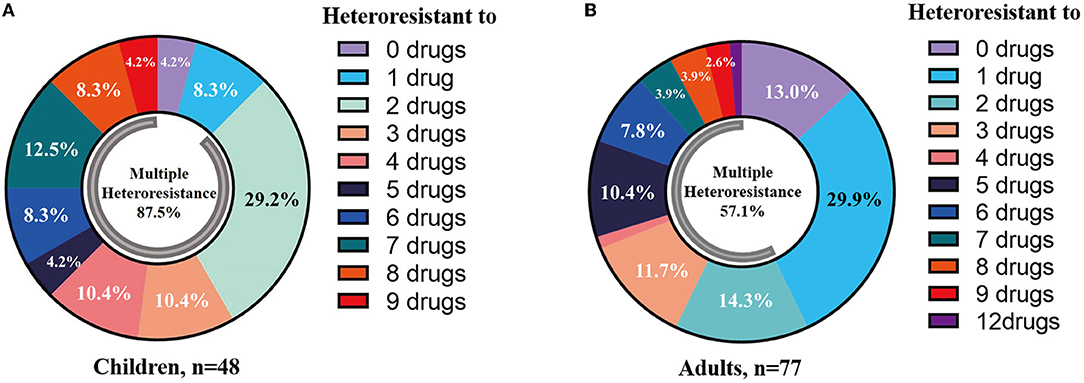
Figure 4. Multiple-heteroresistance phenotypes were common in both populations. (A) Multiple-heteroresistance phenotypes in children. (B) Multiple-heteroresistance phenotypes in adults.
The proportion of different species exhibiting heteroresistance to different drug combinations was various. Surprisingly, Table 4 shows that some common multiple-heteroresistance phenotypes cover a significant percentage of some species. Different species were given specific combination recipes based on their multiple-heteroresistance phenotype.
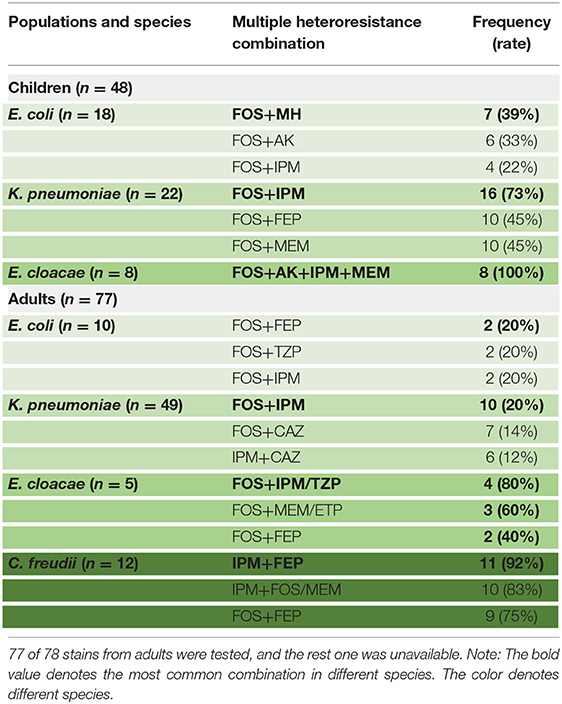
Table 4. The frequencies of common multiple-heteroresistance combinations in isolates of different species from children.
According to Table 4, a typical pediatric E coli strain, #22 strain, was demonstrated to be heteroresistant to both FOS and AK by dual K-B tests and gold standard PAP (Figure 5). We further evaluated the antimicrobial effect of the combination therapy with FOS and AK on the FOS- and AK-co-heteroresistant strain. In dual K-B tests, there was no colony growing at the interface of the two disks, showing a potential additive effect (Figure 6A), which was further supported by E-tests result with a FICI of 1 (Figures 6B,C). We finally used agar dilution checkboard assay, the gold standard method for synergy testing. As expected, the FICI (0.75) of the gold standard further indicated that the effect of the combination was additive (Figure 6D).
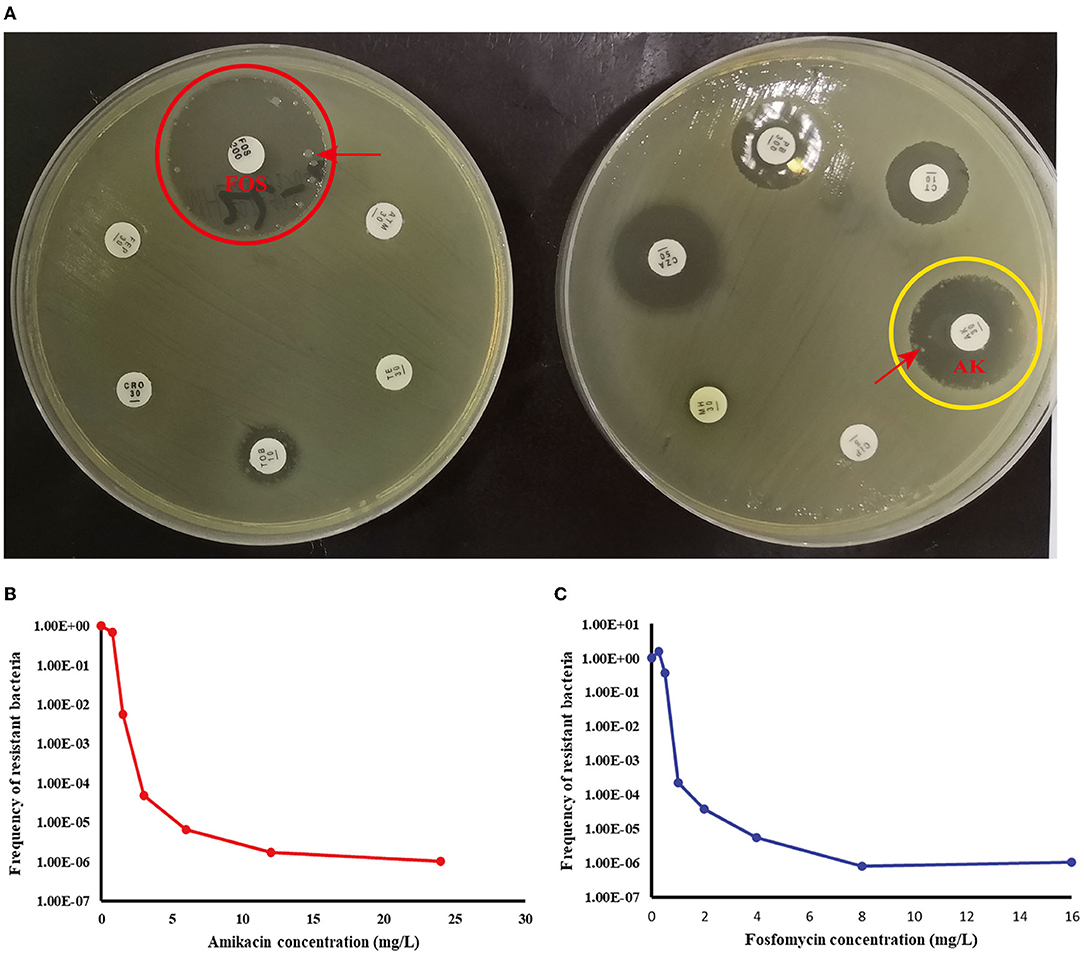
Figure 5. Children #22 strain (E. coli) showed FOS- and AK-co-heteroresistance. (A) K-B tests: the heteroresistance phenotypes of the strain to the two drugs. (B) The PAP curve of amikacin. (C) The PAP curve of fosfomycin. Each value was the average of the results of three repeated experiments. The highest drug concentrations on the plates (FOS and AK) were 4 times the MIC for each drug, and the heteroresistant frequencies of both drugs were >1× 10−7. Red arrows indicated heteroresistant colonies.
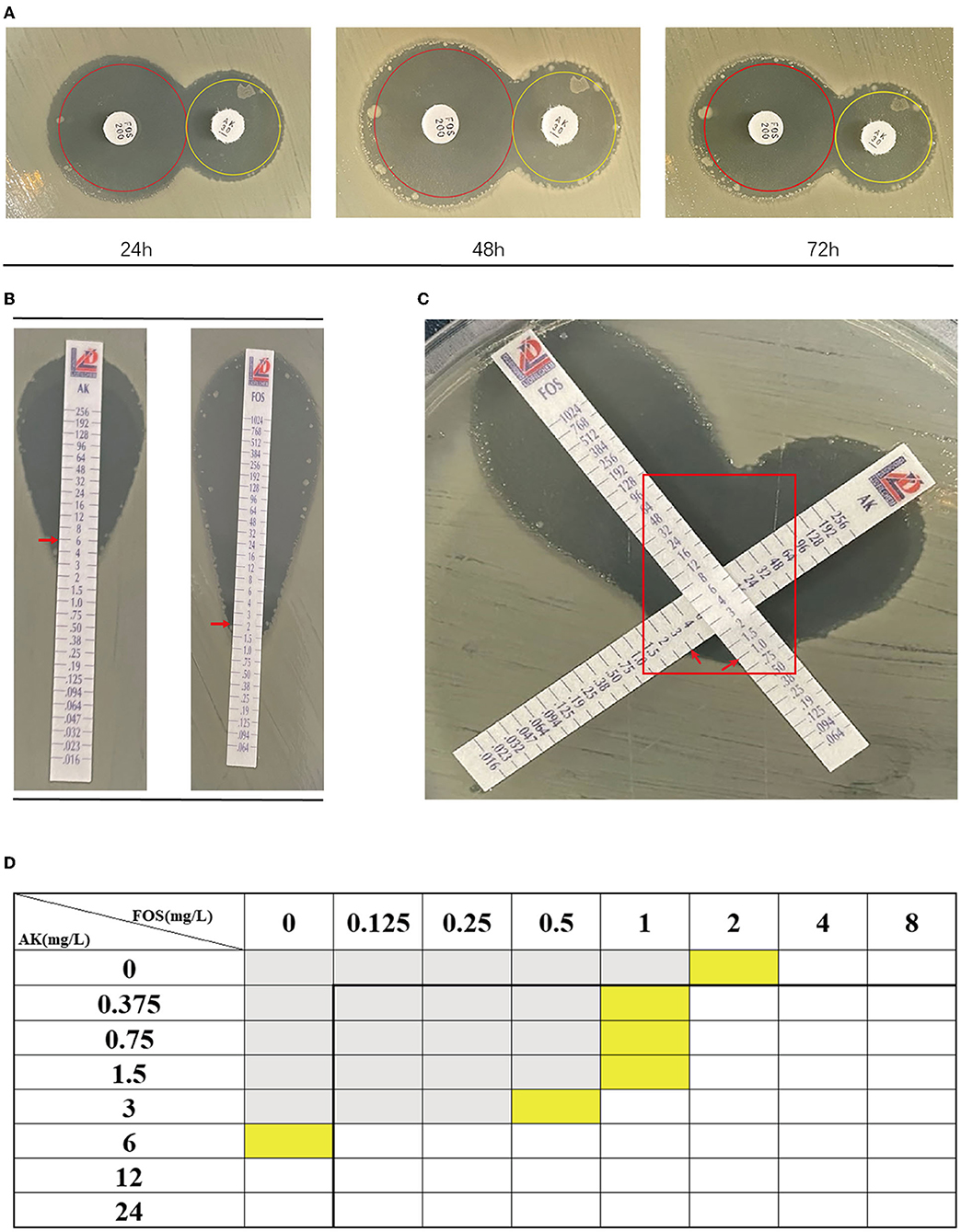
Figure 6. The combination of FOS and AK showed an additive effect on Children #22 strain. (A) Dual K-B tests. The results showed that the effect of the combination of FOS and AK on the Children #22 strain was additive. The pictures from right to left were taken at 24, 48, and 72 h, respectively. There was no colony at the interface of the two antibiotic disks. (B) The E-test results of Children #22 strain to AK and FOS. Red rectangle: there was no colony at the interface of the two antibiotic strips. (C) The modified E-test showed that the combination of FOS and AK on Children #22 strain was additive (FICI = 1) according to the interpretation by Liofilchem. Red arrows on the pictures point to MICs. (D) A schematic of the checkboard assay: the FICI of the assay was 0.75, indicating additive effect. Gray grids showed that bacteria were growing, yellow grids indicated MIC, and white grids showed that no bacteria were growing.
Discussion
Antibiotic treatment for CPE-BSIs is concerning, and the spread of resistance cannot be determined solely based on plasmids or chromosomal lineages (26). Our work comprehensively compared both the clinical and microbiological characteristics of CPE-BSIs in children and adults. We also explored the possibility of designing a combination therapy strategy based on multiple-heteroresistance phenotypes. The research not only filled a knowledge gap in children with CPE-BSIs, but it also discovered the principle behind resistance spread and an effective way to design antibiotic combination therapy.
From the perspective of the clinic, children had a significantly lower 30-day crude mortality when compared with that of adults. We further analyzed the antibiotics administered in both populations. 45.8% of the children were given amikacin as an active drug on an empirical basis, and there was no difference in mortality between those who received amikacin and those who did not. Although aminoglycoside is not recommended in children for its ototoxicity and nephrotoxicity (27), amikacin might be outstanding for its excellent bactericidal activity, thus being the choice of the clinicians in cases where no other effective antibiotics were available. While 38.4 percent of adults were given tigecycline as an active drug on an empirical basis, the tigecycline-treated subgroup had significantly higher 30-day crude mortality. Tigecycline is recommended for CPE infections by many researchers (28–30). In Chinese consensus (29), most combination therapies to XDR Enterobacteriaceae are based on tigecycline, and researches showed that the combination including tigecycline was active to CRE infections, which was contrary to our results. It may be due to 3 reasons: (1) the subject of our study was confined to CPE; (2) severe side-effect of tigecycline; (3) we did not consider confounders, such as the severity of the illness. Anyway, our results indicated that the utility of tigecycline for CPE infections should be reconsidered and limited, rather than taking it as a panacea. However, depending on the underlying conditions and microbiological characteristics, amikacin may be suitable for neonates regardless of toxicity. Notably, nosocomial CPE-BSIs accounted respectively for 81.3% (39/48) and 78.2% (61/78) in the pediatric and adult populations. Some of the explanations for the higher distribution of CPE in nosocomial settings might be the promoted clonal spread and horizontal gene transfer under potent antimicrobial pressure in the hospital environment. CPE transmission in nosocomial settings may also have been aided by frequent invasive procedures and a lack of control measures in healthcare settings aimed at CPE carriers. From the view of microbiology, E. coli isolates tended to carry blaNDM, especially blaNDM−5, whilst E. cloacae strains tended to express MBLs, especially NDM-1, no matter in children or adults. Diverse STs in all species tested tended to carry blaNDM without preferences. The low fitness cost of blaNDM may account for the phenomena, regardless of the plasmids or clonal lineages. Carolina and her colleagues have proved that NDM is of a unique structure and located at the membrane, and these features confer its lowest fitness cost among a series of classical MBLs (8, 31). Furthermore, while it has been reported that plasmids carrying blaNDM often co-harbor the resistance genes to aminoglycosides (32, 33), we discovered that the majority of blaNDM-positive isolates from children were amikacin-susceptible.
Consistent with some previous studies (34, 35), ST11 blaKPC−2-producing K. pneumoniae (42.3%, 33/78) was the mainstay in adults, while in some other previous studies, the successful clonal lineage of blaKPC is ST258/512 (26). Interestingly, ST258 was recognized as a product of the recombination event between ST11 and ST442 (36). There may be some advantages of these STs that contribute to transmission. However, this cannot explain the predominance of blaNDM-producing K. pneumoniae in children. Host immunity may be one of the determinants that affect the strategy that K. pneumoniae is taken to attack, since in our study, E. coli seems to be more readily to infect children instead of adults and the immunity of both populations is of great difference (37).
Because of their efficient carbapenemase activity against practically all β-lactam antibiotics except ATM, potent horizontal transfer, and lack of therapeutic inhibitors, the spread of NDM in pediatric CPE-BSI isolates should be regarded as the most urgent concern. Therapy of CPE-BSIs due to NDM-producing Enterobacterales is extremely challenging, as most blaNDM-encoding plasmids co-harbored multiple resistance determinants, including serine-β-lactamase (SBLs) for ATM, 16S RNA methylases for aminoglycosides, rifampin-modifying enzymes for rifampin, chloramphenicol acetyltransferase for chloramphenicol, and esterase for macrolides, thus expanding to multi-drug resistance (MDR), extreme-drug resistance (XDR), and pan-drug resistance (PDR) (38). Avibactam (AVI) is a non-β-lactam-β-lactamase inhibitor which can inhibit most SBLs, including class A (KPC), class C (AmpC), and certain class D (OXA-48) enzymes, but can neither inhibit MBLs nor protect β-lactams from MBLs hydrolysis (39). The addition of AVI to ATM can theoretically protect ATM from the hydrolysis by SBLs and therefore can expand the spectrum of ATM to cover Enterobacterales with class A, B, C, and some D β-lactamases. As expected, the ATM-AVI combination showed robust in vitro action against KPC producers, NDM-positive isolates, and even KPC and NDM co-producers in our study, with a susceptible rate of 100.0% in pediatric isolates and 98.7% in adult strains, respectively (Table 2). Nevertheless, the possible clinical development of ATM-AVI resistance is of particular concern. In fact, decreased susceptibilities and resistance to ATM-AVI have been recently witnessed in clinical E. coli strains from India (40), China (41), Europe, Africa, Asia, and Australia (42), due to PBP3 insertions and CMY-42 production. Of note, our present study has also demonstrated decreased susceptibilities to ATM-AVI (MIC = 8 mg/L) in one NDM-5-producing adult E. coli isolate from the First Affiliated Hospital of Chongqing Medical University (Table 2). The observation that PBP3 insertions and CMY-42 production in E. coli could adversely impact ATM-AVI activity against MBLs-producing Enterobacteriaceae (MPE) reveals a clinically significant spectrum gap in this MBL-targeted therapy. Such vulnerability of ATM-AVI could also compromise the combined effectiveness of CAZ-AVI and ATM (both targeting PBP3), being viewed as a “rescue therapy” for serious MBL-producer infections (43, 44).
CAZ-AVI, on the other hand, has provided opportunities for safe and effective therapies for serious infections caused by some of the most threatening pathogens producing ESBLs, KPC, AmpC, and OXA-48, but it showed no in vitro activity against MPE (45), as evidenced by our AST results, which showed a low CAZ-AVI-susceptible rate of 14.6% in pediatric isolates (most of them are MPE) and a much higher 62.3% susceptible rate in the adult isolates (most of them are KPC-producers) (Table 2).
Multiple-heteroresistance may help to improve the efficacy of antimicrobial combination therapy design. Traditionally, combination therapy was decided on the basis of experience rather than principles, and it lacked pertinence. We strive to use multiple-heteroresistance phenotypes to tackle the problems: combining the antibiotics showing multiple-heteroresistance phenotypes had a synergistic effect (20), and we discovered that the multiple-heteroresistance profiles of different species were different (Table 4). We finally identified a combination of FOS and AK showing the additive effect on a FOS- and AK-co-heteroresistant E coli strain by this way, and such a combination has been reported recently to be of efficiency without a theoretical basis (46). There were concerns about the toxicity of the two drugs, but reducing doses of the two drugs when combined together could decrease the toxicity of each drug (27, 47, 48). Our study proved the feasibility of the way to design combination therapy based on multiple-heteroresistance, and in this way, we can not only reduce the workload in finding efficient combination but also confer the features of experimental evidence and theoretical basis to the combination therapy. Nevertheless, further investigations from more CPE isolates of various species are needed to evaluate the possible in vitro synergy of the other antimicrobial combinations based on the multiple-heteroresistance phenotypes. Furthermore, because most in vitro test methods are unable to predict clinical success rates, prospective clinical studies based on in vitro synergy testing results are required to improve clinical outcomes.
There is only one limitation of this study is that it's a retrospective study with a relatively small sample size, and a larger prospective cohort study is still needed. Nevertheless, as the test level was kept constant (α = 0.05), and the test efficiency of our study can reach ≥80% (1-β ≥ 80%), the indicators with detected significant differences in our study were authentic.
Overall, we discovered much more detailed characteristics about the dissemination of CPE in different host populations by comparing children and adults with CPE-BSIs, and we proved that the multiple-heteroresistance profile in different species exhibited some specificity, which helped us design combination therapy.
Data Availability Statement
The original contributions presented in the study are included in the article/supplementary material, further inquiries can be directed to the corresponding author/s.
Ethics Statement
The study was approved by the Institutional Ethics Committee of the First Affiliated Hospital of Chongqing Medical University (Number: 2021-524).
Author Contributions
SH, QZ, and SN designed the study. HY, DM, BL, QL, and RY performed the experimental work. DM, BL, and SY collected the data. HY and XJ analyzed the data. HY and SH wrote the article. All authors read and approved the final article.
Funding
This work was sponsored by the National Natural Science Foundation of China (Grant Nos. 82072346 and 82072349), and the Natural Science Foundation of Chongqing, China (No. cstc2020jcyj-msxmX0519).
Conflict of Interest
The authors declare that the research was conducted in the absence of any commercial or financial relationships that could be construed as a potential conflict of interest.
Publisher's Note
All claims expressed in this article are solely those of the authors and do not necessarily represent those of their affiliated organizations, or those of the publisher, the editors and the reviewers. Any product that may be evaluated in this article, or claim that may be made by its manufacturer, is not guaranteed or endorsed by the publisher.
References
1. Tamma PD, Goodman KE, Harris AD, Tekle T, Roberts A, Taiwo A, et al. Comparing the outcomes of patients with carbapenemase-producing and non-carbapenemase-producing carbapenem-resistant Enterobacteriaceae bacteremia. Clin Infect Dis. (2017) 64:257–64. doi: 10.1093/cid/ciw741
2. Livermore DM, Nicolau DP, Hopkins KL, Meunier D. Carbapenem-resistant enterobacterales, carbapenem resistant organisms, carbapenemase-producing enterobacterales, and carbapenemase-producing organisms: terminology past its “Sell-By Date” in an era of new antibiotics and regional carbapenemase epidemiology. Clin Infect Dis. (2020) 71:1776–82. doi: 10.1093/cid/ciaa122
3. Li X, Ye H. Clinical and mortality risk factors in bloodstream infections with carbapenem-resistant Enterobacteriaceae. Can J Infect Dis Med Microbiol. (2017) 2017:6212910. doi: 10.1155/2017/6212910
4. Nabarro LEB, Shankar C, Pragasam AK, Mathew G, Jeyaseelan V, Veeraraghavan B, et al. Clinical and bacterial risk factors for mortality in children with carbapenem-resistant Enterobacteriaceae bloodstream infections in India. Pediatr Infect Dis J. (2017) 36:e161–6. doi: 10.1097/INF.0000000000001499
5. Stewardson AJ, Marimuthu K, Sengupta S, Allignol A, El-Bouseary M, Carvalho MJ, et al. Effect of carbapenem resistance on outcomes of bloodstream infection caused by Enterobacteriaceae in low-income and middle-income countries (PANORAMA): a multinational prospective cohort study. Lancet Infect Dis. (2019) 19:601–10. doi: 10.1016/S1473-3099(18)30792-8
6. Potter RF, D'Souza AW, Dantas G. The rapid spread of carbapenem-resistant Enterobacteriaceae. Drug Resist Updat. (2016) 29:30–46. doi: 10.1016/j.drup.2016.09.002
7. Durante-Mangoni E, Andini R, Zampino R. Management of carbapenem-resistant Enterobacteriaceae infections. Clin Microbiol Infect. (2019) 25:943–50. doi: 10.1016/j.cmi.2019.04.013
8. González LJ, Bahr G, Nakashige TG, Nolan EM, Bonomo RA, Vila AJ. Membrane anchoring stabilizes and favors secretion of New Delhi metallo-β-lactamase. Nat Chem Biol. (2016) 12:516–22. doi: 10.1038/nchembio.2083
9. Wu W, Feng Y, Tang G, Qiao F, McNally A, Zong Z, et al. Metallo-β-Lactamases and Their Bacterial Producers in Health Care Settings. Clin Microbiol Rev. (2019) 32:45. doi: 10.1128/CMR.00115-18
10. Gutiérrez-Gutiérrez B, Salamanca E, de Cueto M, Hsueh P-R, Viale P, Paño-Pardo JR, et al. Effect of appropriate combination therapy on mortality of patients with bloodstream infections due to carbapenemase-producing Enterobacteriaceae (INCREMENT): a retrospective cohort study. Lancet Infect Dis. (2017) 17:726–34. doi: 10.1016/S1473-3099(17)30522-4
11. Wang X, Wang Q, Cao B, Sun S, Zhang Y, Gu B, et al. Retrospective observational study from a chinese network of the impact of combination therapy versus monotherapy on mortality from carbapenem-resistant Enterobacteriaceae bacteremia. Antimicrob Agents Chemother. (2018) 63:e01511–18. doi: 10.1128/AAC.01511-18
12. Laxminarayan R, Matsoso P, Pant S, Brower C, Røttingen J-A, Klugman K, et al. Access to effective antimicrobials: a worldwide challenge. Lancet. (2016) 387:168–75. doi: 10.1016/S0140-6736(15)00474-2
13. van Duin D, Doi Y. The global epidemiology of carbapenemase-producing Enterobacteriaceae. Virulence. (2017) 8:460–9. doi: 10.1080/21505594.2016.1222343
14. Falcone M, Paterson D. Spotlight on ceftazidime/avibactam: a new option for MDR gram-negative infections. J Antimicrob Chemother. (2016) 71:2713–22. doi: 10.1093/jac/dkw239
15. Biedenbach DJ, Kazmierczak K, Bouchillon SK, Sahm DF, Bradford PA. In vitro activity of aztreonam-avibactam against a global collection of Gram-negative pathogens from 2012 and 2013. Antimicrob Agents Chemother. (2015) 59:4239–48. doi: 10.1128/AAC.00206-15
16. Periasamy H, Joshi P, Palwe S, Shrivastava R, Bhagwat S, Patel M. High prevalence of Escherichia coli clinical isolates in India harbouring four amino acid inserts in PBP3 adversely impacting activity of aztreonam/avibactam. J Antimicrob Chemother. (2020) 75:1650–1. doi: 10.1093/jac/dkaa021
17. Zhang P, Shi Q, Hu H, Hong B, Wu X, Du X, et al. Emergence of ceftazidime/avibactam resistance in carbapenem-resistant Klebsiella pneumoniae in China. Clin Microbiol Infect. (2020) 26:124.e1–4. doi: 10.1016/j.cmi.2019.08.020
18. Manohar P, Loh B, Athira S, Nachimuthu R, Hua X, Welburn SC, et al. Secondary bacterial infections during pulmonary viral disease: phage therapeutics as alternatives to antibiotics? Front Microbiol. (2020) 11:1434. doi: 10.3389/fmicb.2020.01434
19. Chiotos K, Hayes M, Gerber JS, Tamma PD. Treatment of carbapenem-resistant Enterobacteriaceae infections in children. J Pediatric Infect Dis Soc. (2020) 9:56–66. doi: 10.1093/jpids/piz085
20. Band VI, Hufnagel DA, Jaggavarapu S, Sherman EX, Wozniak JE, Satola SW, et al. Antibiotic combinations that exploit heteroresistance to multiple drugs effectively control infection. Nat Microbiol. (2019) 4:1627–35. doi: 10.1038/s41564-019-0480-z
21. Tian X, Sun S, Jia X, Zou H, Li S, Zhang L. Epidemiology of and risk factors for infection with extended-spectrum β-lactamase-producing carbapenem-resistant Enterobacteriaceae: results of a double case-control study. Infect Drug Resist. (2018) 11:1339–46. doi: 10.2147/IDR.S173456
22. Patel J, Weinstein M, Eliopoulos G, Jenkins S, Lewis J, Limbago B. M100 Performance standards for antimicrobial susceptibility testing. United State: Clinical and Laboratory Standards Institute (2017).
23. Testing TECoAS. Breakpoint tables for interpretation of MICs and zone diameters. version 5.0. Copenhagen: The European Committee on Antimicrobial Susceptibility Testing (2015).
24. Nicoloff H, Hjort K, Levin BR, Andersson DI. The high prevalence of antibiotic heteroresistance in pathogenic bacteria is mainly caused by gene amplification. Nat Microbiol. (2019) 4:504–14. doi: 10.1038/s41564-018-0342-0
25. Iannaccone M, Boattini M, Bianco G, Cavallo R, Costa C. Meropenem/vaborbactam-based combinations against KPC-producing Klebsiella pneumoniae and multidrug-resistant Pseudomonas aeruginosa. Int J Antimicrob Agents. (2020) 56:106066. doi: 10.1016/j.ijantimicag.2020.106066
26. David S, Cohen V, Reuter S, Sheppard AE, Giani T, Parkhill J, et al. Integrated chromosomal and plasmid sequence analyses reveal diverse modes of carbapenemase gene spread among Klebsiella pneumoniae. Proc Natl Acad Sci. (2020) 117:25043–54. doi: 10.1073/pnas.2003407117
27. Kent A, Turner MA, Sharland M, Heath PT. Aminoglycoside toxicity in neonates: something to worry about? Expert Rev Anti Infect Ther. (2014) 12:319–31. doi: 10.1586/14787210.2014.878648
28. Hsu AJ, Tamma PD. Treatment of multidrug-resistant gram-negative infections in children. Clin Infect Dis. (2014) 58:1439–48. doi: 10.1093/cid/ciu069
29. Guan X, He L, Hu B, Hu J, Huang X, Lai G, et al. Laboratory diagnosis, clinical management and infection control of the infections caused by extensively drug-resistant gram-negative bacilli: a Chinese consensus statement. Clin Microbiol Infect. (2016) 22:S15–25. doi: 10.1016/j.cmi.2015.11.004
30. Sheu C-C, Chang Y-T, Lin S-Y, Chen YH, Hsueh PR. Infections caused by carbapenem-resistant Enterobacteriaceae: an update on therapeutic options. Front Microbiol. (2019) 10:80. doi: 10.3389/fmicb.2019.00080
31. López C, Ayala JA, Bonomo RA, González LJ, Vila AJ. Protein determinants of dissemination and host specificity of metallo-β-lactamases. Nat Commun. (2019) 10:3617. doi: 10.1038/s41467-019-11615-w
32. Taylor E, Sriskandan S, Woodford N, Hopkins KL. High prevalence of 16S rRNA methyltransferases among carbapenemase-producing Enterobacteriaceae in the UK and Ireland. Int J Antimicrob Agents. (2018) 52:278–82. doi: 10.1016/j.ijantimicag.2018.03.016
33. Findlay J, Poirel L, Kessler J, Kronenberg A, Nordmann P. New Delhi metallo-β-lactamase-producing enterobacterales bacteria, Switzerland, 2019–2020. Emerg Infect Dis. (2021) 27:2628–37. doi: 10.3201/eid2710.211265
34. Gu D, Dong N, Zheng Z, Lin D, Huang M, Wang L, et al. A fatal outbreak of ST11 carbapenem-resistant hypervirulent Klebsiella pneumoniae in a Chinese hospital: a molecular epidemiological study. Lancet Infect Dis. (2018) 18:37–46. doi: 10.1016/S1473-3099(17)30489-9
35. David S, Reuter S, Harris SR, Glasner C, Feltwell T, Argimon S, et al. Epidemic of carbapenem-resistant Klebsiella pneumoniae in Europe is driven by nosocomial spread. Nature Microbiology. (2019) 4:1919–29. doi: 10.1038/s41564-019-0492-8
36. Pitout JD, Nordmann P, Poirel L. Carbapenemase-producing Klebsiella pneumoniae, a key pathogen set for global nosocomial dominance. Antimicrob Agents Chemother. (2015) 59:5873–84. doi: 10.1128/AAC.01019-15
37. Dasari P, Zola H, Nicholson IC. Expression of Toll-like receptors by neonatal leukocytes. Pediatr Allergy Immunol. (2011) 22:221–8. doi: 10.1111/j.1399-3038.2010.01091.x
38. Bush K, Bradford PA. Epidemiology of β-lactamase-producing pathogens. Clin Microbiol Rev. (2020) 33:e00047–19. doi: 10.1128/CMR.00047-19
39. Abboud MI, Damblon C, Brem J, Smargiasso N, Mercuri P, Gilbert B, et al. Interaction of avibactam with class B metallo-β-lactamases. Antimicrob Agents Chemother. (2016) 60:5655–62. doi: 10.1128/AAC.00897-16
40. Alm RA, Johnstone MR, Lahiri SD. Characterization of Escherichia coli NDM isolates with decreased susceptibility to aztreonam/avibactam: role of a novel insertion in PBP3. J Antimicrob Chemother. (2015) 70:1420–8. doi: 10.1093/jac/dku568
41. Ma K, Feng Y, McNally A, Zong Z. Struggle to survive: the choir of target alteration, hydrolyzing enzyme, and plasmid expression as a novel aztreonam-avibactam resistance mechanism. mSystems. (2020) 5:e00821-20. doi: 10.1128/mSystems.00821-20
42. Sadek M, Juhas M, Poirel L, Nordmann P. Genetic features leading to reduced susceptibility to aztreonam-avibactam among metallo-β-lactamase-producing Escherichia coli isolates. Antimicrob Agents Chemother. (2020) 64:e01659-20. doi: 10.1128/AAC.01659-20
43. Sieswerda E, van den Brand M, van den Berg RB, Sträter J, Schouls L, van Dijk K, et al. Successful rescue treatment of sepsis due to a pandrug-resistant, NDM-producing Klebsiella pneumoniae using aztreonam powder for nebulizer solution as intravenous therapy in combination with ceftazidime/avibactam. J Antimicrob Chemother. (2020) 75:773–5. doi: 10.1093/jac/dkz495
44. Falcone M, Daikos GL, Tiseo G, Bassoulis D, Giordano C, Galfo V, et al. Efficacy of ceftazidime-avibactam plus aztreonam in patients with bloodstream infections caused by metallo-β-lactamase-producing enterobacterales. Clin Infect Dis. (2021) 72:1871–8. doi: 10.1093/cid/ciaa586
45. Yahav D, Giske CG, Grāmatniece A, Abodakpi H, Tam VH, Leibovici L. New β-lactam-β-lactamase inhibitor combinations. Clin Microbiol Rev. (2020) 34:e00115–20. doi: 10.1128/CMR.00115-20
46. Darlow CA, Docobo-Perez F, Farrington N, Johnson A, McEntee L, Unsworth J, et al. Amikacin combined with fosfomycin for treatment of neonatal sepsis in the setting of highly prevalent antimicrobial resistance. Antimicrob Agents Chemother. (2021) 65:e0029321. doi: 10.1128/AAC.00293-21
47. Falagas ME, Vouloumanou EK, Samonis G, Vardakas KZ. Fosfomycin. Clin Microbiol Rev. (2016) 29:321–47. doi: 10.1128/CMR.00068-15
Keywords: carbapenemase-producing Enterobacterales, bloodstream infections, antibiotic treatment, blaNDM, blaKPC, heteroresistance, combination therapy, children
Citation: Yu H, Ma D, Liu B, Yang S, Lin Q, Yu R, Jia X, Niu S, Zhang Q and Huang S (2022) Differences in the Distribution of Species, Carbapenemases, Sequence Types, Antimicrobial Heteroresistance and Mortality Rates Between Pediatric and Adult Carbapenemase-Producing Enterobacterales in Bloodstream Infections. Front. Med. 9:827474. doi: 10.3389/fmed.2022.827474
Received: 02 December 2021; Accepted: 14 February 2022;
Published: 14 March 2022.
Edited by:
Qazi Mohd Rizwanul Haq, Jamia Millia Islamia, IndiaReviewed by:
Prasanth Manohar, Zhejiang University-University of Edinburgh Institute, ChinaEman Aly ELGhareeb Hamza, Cairo University, Egypt
Copyright © 2022 Yu, Ma, Liu, Yang, Lin, Yu, Jia, Niu, Zhang and Huang. This is an open-access article distributed under the terms of the Creative Commons Attribution License (CC BY). The use, distribution or reproduction in other forums is permitted, provided the original author(s) and the copyright owner(s) are credited and that the original publication in this journal is cited, in accordance with accepted academic practice. No use, distribution or reproduction is permitted which does not comply with these terms.
*Correspondence: Siqiang Niu, c2lxaWFuZ25pdSYjeDAwMDQwO2NxbXUuZWR1LmNu; Qun Zhang, emhhbmdxdW4xOTgxNjYmI3gwMDA0MDsxNjMuY29t; Shifeng Huang, c2ZodWFuZyYjeDAwMDQwO2hvc3BpdGFsLmNxbXUuZWR1LmNu
†These authors have contributed equally to this work and share first authorship