- 1Eye Center of the Second Affiliated Hospital, School of Medicine, Zhejiang University, Hangzhou, China
- 2Zhejiang Provincial Key Lab of Ophthalmology, Hangzhou, China
Purpose: This study aimed to evaluate the features of corneal nerve with in vivo confocal microscopy (IVCM) among patients with non-neurological autoimmune (NNAI) diseases.
Methods: We systematically searched PubMed, Web of Science, and Cochrane Central Register of Controlled Trials for studies published until May 2021. The weighted mean differences (WMDs) of corneal nerve fiber length (CNFL), corneal nerve fiber density (CNFD), corneal nerve branch density (CNBD), tortuosity, reflectivity, and beadings per 100 μm with a 95% CI between NNAI and control group were analyzed using a random-effects model.
Results: The results showed 37 studies involving collective totals of 1,423 patients and 1,059 healthy controls were ultimately included in this meta-analysis. The pooled results manifested significantly decreased CNFL (WMD: −3.94, 95% CI: −4.77–−3.12), CNFD (WMD: −6.62, 95% CI: −8.4–−4.85), and CNBD (WMD: −9.89, 95% CI: −14–−5.79) in NNAI patients. In addition, the NNAI group showed more tortuous corneal nerve (WMD: 1.19, 95% CI:0.57–1.81). The comparison between NNAI patients and healthy controls in beadings per 100 μm corneal nerve length was inconsistent. No significant difference was found in the corneal nerve fiber reflectivity between NNAI and the control group (WMD: −0.21, 95% CI: −0.65–0.24, P = 0.361).
Conclusions: The parameters and morphology of corneal nerves observed by IVCM proved to be different in NNAI patients from healthy controls, suggesting that IVCM may be a non-invasive technique for identification and surveillance of NNAI diseases.
Introduction
Autoimmune diseases are a range of diseases characterized by increased activity of the immune system which results in organ damage or dysfunction (1). According to research, autoimmune diseases affect approximately 7.6–9.4% of the general population and impose huge burdens not only on patients themselves but also on the whole society (2). Genetic, microbial, environmental, lifestyle, and psychological factors are thought as contributing elements to autoimmune diseases although the underlying etiology remains to be explored (3). Despite impressive advances in the management of autoimmune diseases, they are still impossible to cure. A definitive diagnosis as early as possible can increase the efficiency and efficacy of the treatment strategy and also help to avoid complications (4, 5). In this case, an early diagnosis can play a decisive role in improving the patient's quality of life as well as life expectancies.
The cornea is a transparent part covering the front portion of the eyewall and is regarded as the most densely innervated tissue in the human body. With a density of approximately 7,000 epithelial-free nerve endings per square millimeter, the cornea is about 300–600 times more sensitive than skin (6). A review has concluded that changes in corneal innervation can occur for many reasons, including keratitis, corneal dystrophies, corneal degenerations, corneal ecstasies, glaucoma, medical treatment, etc (7). Corneal nerve alternation is not only a window to observe some ocular diseases, but also a potential window to observe systemic diseases. In this article, we focus on non-neurological autoimmune (NNAI) diseases which exclude autoimmune diseases that affect the central nervous system mostly or present obvious psychiatric manifestations. This is a range of autoimmune diseases admitted by the American Autoimmune and Related Diseases Association and excluded from the list of known neurological disorders by the American Academy of Neurology. Some of the NNAI diseases have been discovered to be associated with the human cornea and peripheral neurological manifestations as early as the 1980's. Keratitis was found may be a presenting sign of rheumatoid arthritis or sarcoidosis (8); immune deposits in the cornea were found in patients with systemic lupus erythematosus by immunopathological staining (9). People with NNAI diseases are at high risk of innervation alternation and have a high incidence of various kinds of neuropathy. For instance, it is reported that up to 86% of patients with sarcoidosis present with typical small-fiber neuropathy symptoms (10), over 60% of patients with Sjögren's syndrome suffer from peripheral neuropathy (11, 12), higher prevalence of NNAI diseases including rheumatoid arthritis, systemic lupus erythematosus, Sjögren's syndrome suffer from fibromyalgia and so on (13). Innervation alternation may be progressing soon after the onset of NNAI because of the high sensitivity of the nerves. The corneal nerve may have undergone a long time when observable changes appear, but no symptoms or discomfort are perceived by the patient. For this reason, corneal signs may be the first manifestation of autoimmune diseases. Alteration of corneal nerve parameters is of great significance beyond ocular diseases, it can provide clinicians with thought-provoking insight into the clinical diagnosis or management of many diseases like type 1 diabetes, Parkinson's disease, Friedreich ataxia before organ damages is manifested (14–16). Many researchers showed significant associations between the reduction in corneal innervation and increasing disease severity in neurological autoimmune diseases like multiple sclerosis (17, 18). However, studies present conflicting results on the effect of NNAI diseases on corneal innervation. Moreover, previous studies focus on histopathology results rather than non-invasive analysis. In vivo confocal microscopy (IVCM), with its ease of clinical set-up and a 800-fold magnification of cellular level, is becoming a promising as well as a non-invasive tool to view and quantify corneal nerve parameters directly (19). In this way, IVCM may provide a non-invasive potential biomarker for NNAI. Hence, we collected data from different studies about the corneal nerve parameters measured by IVCM in various NNAI diseases and conduct a meta-analysis to evaluate the potential application of this technique as an indicator of NNAI diseases.
Methods
Search Strategy
A systematic literature search was conducted in PubMed, Web of Science, and Cochrane Central Register of Controlled Trials (updated to May 2021). No constraints were applied regarding the language or the publication time of works of literature. Search terms included confocal microscopy or IVCM or cornea* nerve with a combination of autoimmune diseases or autoimmune diseases or XXX, the last-mentioned representing 36 individual NNAI diseases (Figure 1). The selection of NNAI diseases referred to Alexis E. Cullen's study (20). All autoimmune diseases searched were selected a priori from the American Autoimmune and Related Diseases Association and were cross-checked against known neurological disorders, as listed by the American Academy of Neurology. We excluded uveitis for it is essentially a type of eye disease and would, to some extent, affects corneal structure and function. Neither did we adopt data among type 1 diabetes peripheral neuropathy and type 1 diabetic retinopathy, for they had been proved to be related to corneal nerve changes (21–23).
Inclusion and Exclusion Criteria
We included studies that met the following criteria: (1) at least 10 adults with a definite diagnosis of NNAI diseases in the test group; (2) a healthy population as the control group; (3) reporting at least corneal nerve fiber density (CNFD) or corneal nerve fiber length (CNFL). Exclusion Criteria were as follows: (1) inappropriate types of articles, such as reviews, case reports, editorials, conference papers and abstracts, short surveys, or letters; (2) studies which subjects with NNAI diseases were divided into irrelevant subgroups, for instance, dividing patients with type 1 diabetes by erectile dysfunction; (3) studies assessing only animals; (4) studied based or partially based on the same population (studies with the most sufficient data were selected); (5) articles without sufficient data (i.e., mean and SD).
Data Extraction
All publications searched were exported to Endnote (version X9.3; The Thomson Corporation Corp, Stanford, CT, USA). Then, duplicate publications were collated and removed. Two researchers (YG and XL) assessed the titles and abstracts independently for potential eligibility, and the full-text articles were retrieved which appeared relevant. Final eligibility was performed by assessing full-text articles and disagreements on eligibility were resolved via discussion and, if necessary, by consulting a third researcher (XY). Studies that were in accord with the inclusion/exclusion criteria were read, and the following information was extracted from the eligible articles: study details (such as the first author's name, year of acceptance, type of IVCM, and software used to measure corneal nerve parameters) and subjects' information (such as mean age, subjects' sex, duration of NNAI diseases, type of diseases, and corneal nerve parameters). The screening process and protocol are summarized and described in the flow diagram.
Assessments of Article Quality
The Newcastle-Ottawa Scale, covering three methodological domains (selection criteria, comparability, and measurement of exposure and/or outcome), was used to rate article quality. With a maximum score of 9, we defined the article as low quality if the numeric score was 0–3, moderate quality if the score was 4–6, and high quality if the score was 7–9. Low-quality articles were excluded.
Statistical Analysis
This meta-analysis was conducted using the Stata (version 15.1; StataCorp LLC, College Station, TX, USA), a p-value of <0.05 was considered statistically significant. We extracted the mean, standard deviation, and sample size for continuous corneal nerve parameters, and the Random-effects model was applied to calculate the weighted mean difference (WMD) with 95% CI. In order to facilitate comparison, we defined the total length of the corneal nerve fibers as CNFL, the total number of corneal nerve fibers per mm2 as CNFD, and the number of branches originating from major nerve trunks per mm2 as corneal nerve branch density (CNBD). Nerve length or nerve density was divided by image area, if necessary, in order to unify the units of corneal nerve parameters. Besides the parameters above, we also recorded nerve tortuosity, reflectivity, and beadings. Nerve tortuosity and nerve reflectivity were presented as four grades according to previously validated grading scales (24). Beadings were defined as the number of bead-like formations in 100 μm of the nerve fiber. It should be mentioned that some works of research evaluated corneal nerve tortuosity with tortuosity coefficient, which is not adopted in this meta-analysis for a reliable comparison. We performed a sensitivity analysis by omitting one study at a time and calculating a pooled estimate for the remaining studies to evaluate the contribution of each individual study to the results. The I2 statistic was used to estimate heterogeneity among the studies. To explore the potential confounding factors, we performed subgroup analysis by age, type of IVCM, software used to measure corneal nerve parameters, and types of NNAI diseases. Publication bias was estimated by funnel plot, as well as Egger's linear regression test and Begg's rank association test with significance set to P < 0.1 (25, 26).
Results
Search Process
The selection of studies is shown in Figure 2. Potential references were screened from PubMed (n = 4,159), Web of Science (n = 6,571) and Cochrane Library (n = 82). After duplicate publications were removed, the titles and abstracts of 7,020 remaining studies were assessed for potential eligibility. For final eligibility, a total of 139 full-text articles were screened thoroughly and 102 studies were excluded due to reasons listed in Figure 2. No articles were excluded because of low quality. Quality rating scores ranged from 5 to 9 (mean: 7.24, SD: 1.04). Thus, a total of 37 studies were eligible for the final meta-analysis which included 1,423 patients and 1,059 healthy controls (16, 27–61).
Study Characteristics
Among the 37 included studies, 18 were related to type 1 diabetes, 13 were related to Sjögren's Syndrome, 1 was related to Bechet's disease, 1 was related to coeliac disease, 1 was related to Graves' disease, 1 was related to hypothyroidism, 1 was related to mucous membrane pemphigoid, and 1 was related to rheumatoid arthritis. As shown in Table 1, different studies reported different corneal nerve parameters. Most of the studies used laser scanning confocal microscopy (LSCM) or slit scanning confocal microscopy (SSCM) as IVCM appliances, except for tandem scanning confocal microscopy (TSCM) in one study and unspecified appliance in another. As for IVCM image analysis software, CCMetrics, ACCMetrics, and Image J were commonly used. Other characteristics of the included studies such as demographics, research groups, disease durations are also summarized in Table 1. Representative IVCM images of the cornea in patients with healthy controls and patients with NNAI diseases are listed in Figure 3.
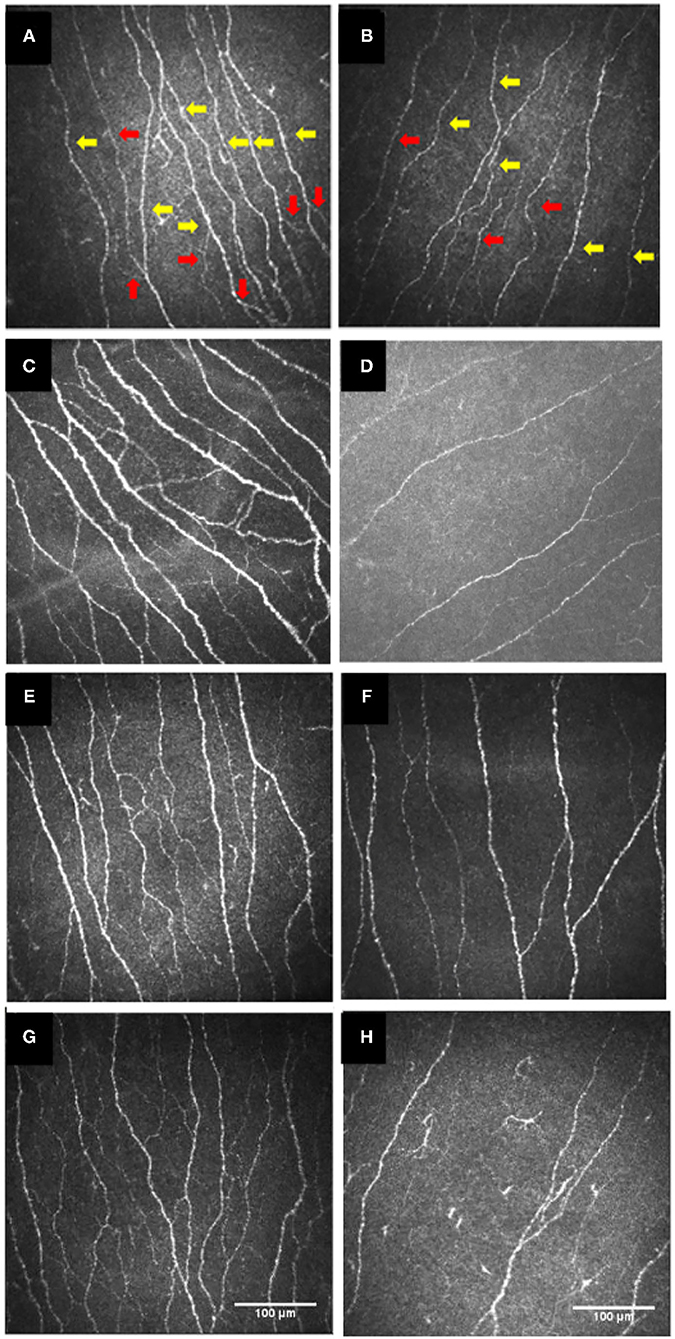
Figure 3. IVCM images of the cornea in the healthy controls (A,C,E,G) and patients with type 1 diabetes (B), or with Sjögren's syndrome (D), or with celiac disease (F), or with Behçet's disease (H). Red arrows show corneal nerve branches and yellow arrows show corneal nerve fibers. (A,B) were re-organized with permission from (45), copyright 2017, Public Library of Science. (C,D) were re-organized with permission from (62), copyright 2021, BioMed Central. (E,F) were re-organized with permission from (33), copyright 2020, Public Library of Science. (G,H) were re-organized with permission from (40), copyright 2018, Frontiers. IVCM, in vivo confocal microscopy.
Corneal Nerve Parameters (CNFL, CNFD, CNBD)
Including 2,335 participants (1,337 in the NNAI group and 998 in the control group), thirty-two studies reported on CNFL. The WMD in CNFL between NNAI and control groups was−3.94 (95% CI: −4.77–−3.12, P < 0), with significant heterogeneity across studies (I2 = 93.2%, Figure 4). The results showed CNFL (mm/mm2) was obviously lower in the NNAI group.
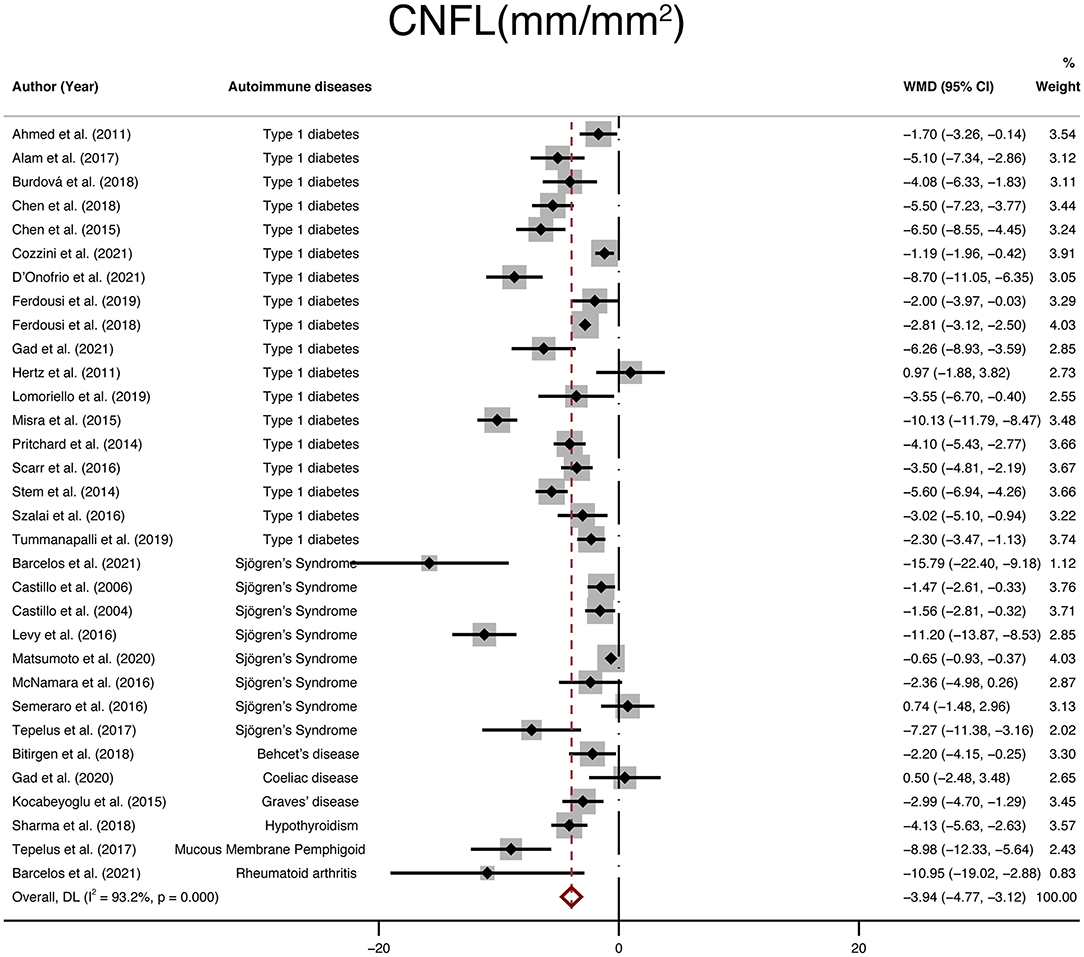
Figure 4. Forest plot of the WMD of CNFL between the NNAI group and the control group. WMD, weighted mean difference; CI, confidence interval; CNFL, corneal nerve fiber length; NNAI, non-neurological autoimmune (diseases).
Furthermore, twenty-eight studies with a total of 1,696 participants (946 in the NNAI group and 750 in the control group) reported on CNFD. The weighted mean difference was−6.62 (95% CI: −8.4–−4.85, P < 0), with significant heterogeneity across studies (I2 = 90.6%, Figure 5), showing that CNFD (no./mm2) of the NNAI group was significantly lower than that of the control group.
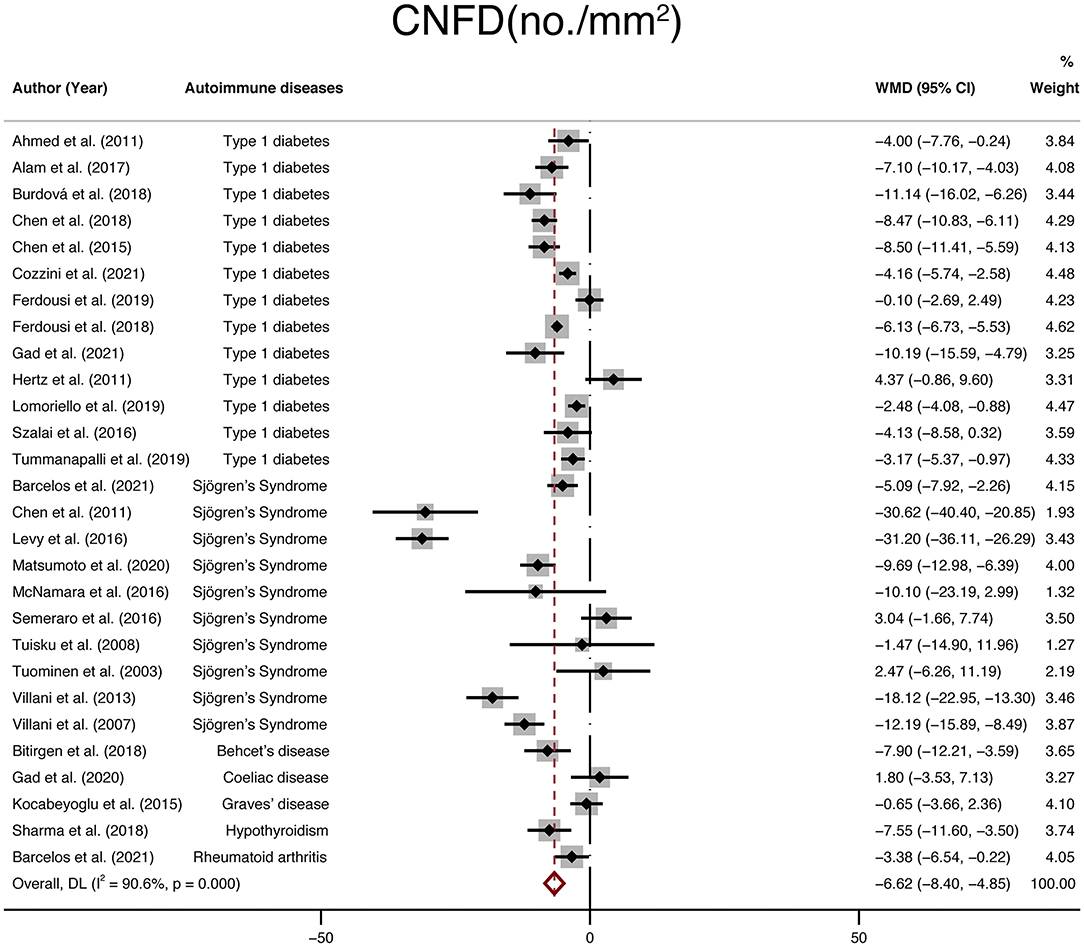
Figure 5. Forest plot of the WMD of CNFD between the NNAI group and the control group. WMD, weighted mean difference; CI, confidence interval; CNFD, corneal nerve fiber density; NNAI, non-neurological autoimmune (diseases).
Finally, twenty-two studies with a total of 1,699 participants (924 in the NNAI group and 775 in the control group) reported on CNBD. The weighted mean difference was −9.89 (95% CI: −14–−5.79, P < 0), with significant heterogeneity across studies (I2 = 88.4%, Figure 6). Consistently, CNBD (no./mm2) of NNAI patients was significantly lower than that of healthy controls.
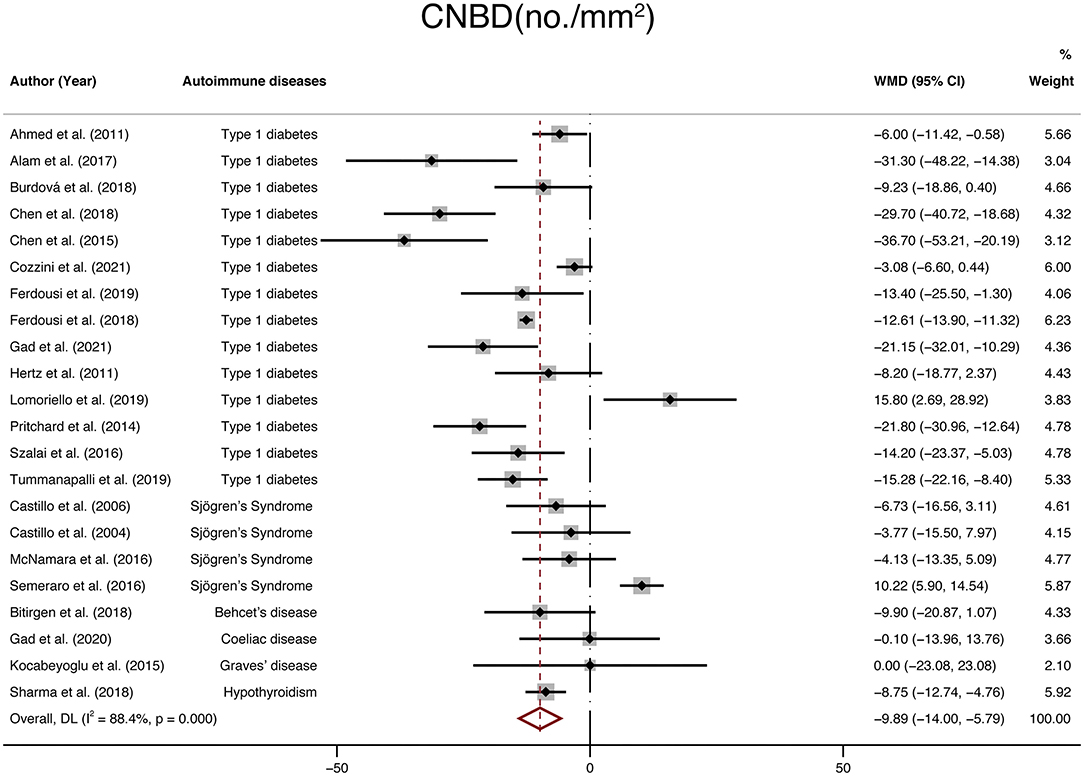
Figure 6. Forest plot of the WMD of CNBD between the NNAI group and the control group. WMD, weighted mean difference; CI, confidence interval; CNBD, corneal nerve branch density; NNAI, non-neurological autoimmune (diseases).
Publication Bias
The publication bias of the studies was shown by funnel plots (Figure 7). The symmetrical funnel plot showed no significant publication bias in the publications reported on CNFD and CNBD. However, the results revealed that studies reported CNFL was mild asymmetry visually, suggesting a publication bias. In addition, Egger linear regression tests and the Begg's rank association tests were performed (Table 2). All other results demonstrated no evidence of significant publication bias except for Egger's test on CNFL. After recalculating the WMD on CNFL using the trim and fill methods, the pooled results were similar to the original results, which means the observed publication bias did not influence the overall results.
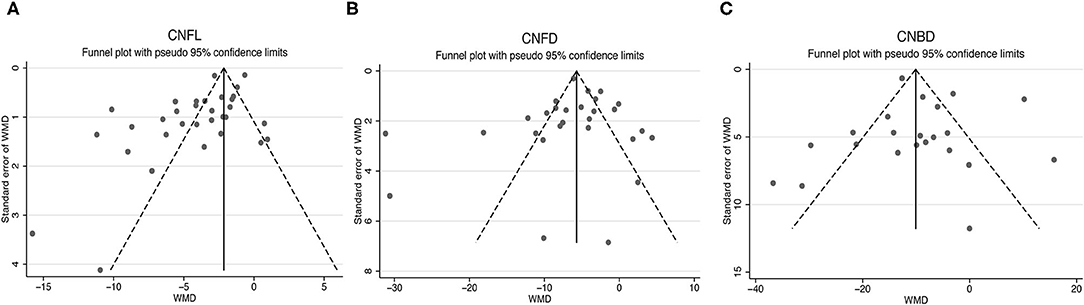
Figure 7. Funnel plots for studies included reported CNFL (A), CNFD (B), and CNBD (C). CNFL, corneal nerve fiber length; CNFD, corneal nerve fiber density; CNBD, corneal nerve branch density; WMD, weighted mean difference.
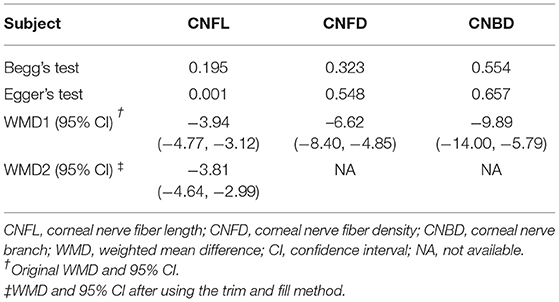
Table 2. Publication bias measured by Begg's and Egger's tests, WMD (95% CI) recalculated with trim and fill method.
Sensitivity Analysis and Subgroup Analysis
To explore the source of heterogeneity, sensitivity analysis was performed. The results revealed that no individual study had an excessive influence on the above-mentioned pooled effect (Figure 8).
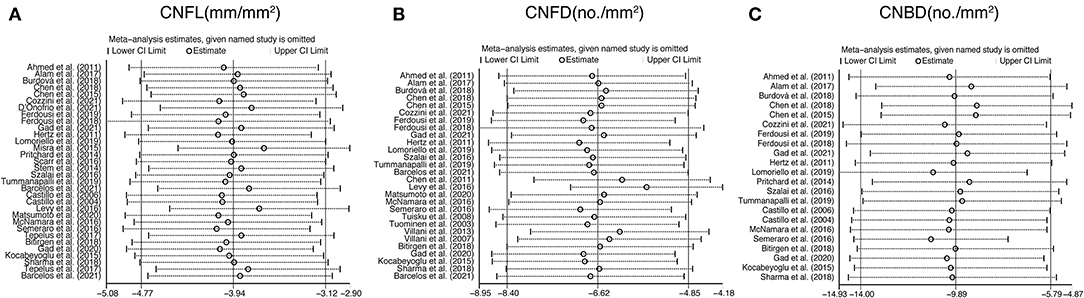
Figure 8. Sensitivity analysis data for studies included reported CNFL (A), CNFD (B), and CNBD (C). CNFL, corneal nerve fiber length; CNFD, corneal nerve fiber density; CNBD, corneal nerve branch density.
Stratifications by age, type of IVCM, software used to measure corneal nerve parameters, and type of NNAI diseases were analyzed due to high heterogeneity. Among studies that reported CNFL, subgroup analysis demonstrated that heterogeneity was reduced for studies grouped by type of IVCM only when using SSCM to record CNFL (I2 = 26.1%). Among studies that reported CNFD, subgroup analysis demonstrated that heterogeneity was reduced for studies grouped by the software used only when using built-in software to assess CNFD (I2 = 43.1%). And among studies that reported CNBD, heterogeneity was significantly reduced for studies grouped by the software used only when using Image J to assess CNBD (I2 = 0%). The detailed results of subgroup analysis are depicted in Table 3.
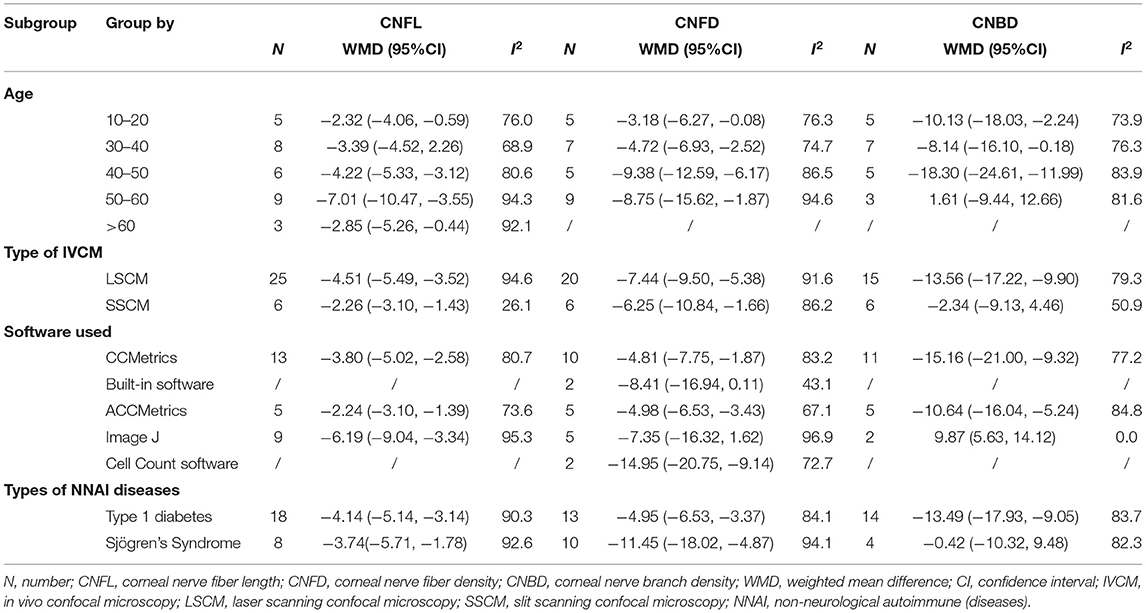
Table 3. Subgroup analysis of CNFL, CNFD, and CNBD by age, type of IVCM, software used, and types of NNAI diseases.
Tortuosity, Reflectivity, and Beadings
In addition, IVCM enabled en-face examination of corneal nerves. Therefore, tortuosity, reflectivity, and beadings are also widely used to quantify corneal nerve morphology. We collected 11 studies that reported tortuosity, 6 studies that reported reflectivity, 6 studies that reported beadings and performed a meta-analysis. The results demonstrated that the differences in tortuosity (WMD: 1.19, 95% CI:0.57–1.81) and beadings (WMD: 19.91, 95% CI: 11.92–27.9) between the NNAI group and the control group were statistically significant, while the reflectivity (WMD: −0.21, 95% CI: −0.65–0.24) of NNAI patients showed no statistical difference from healthy controls (Figure 9).
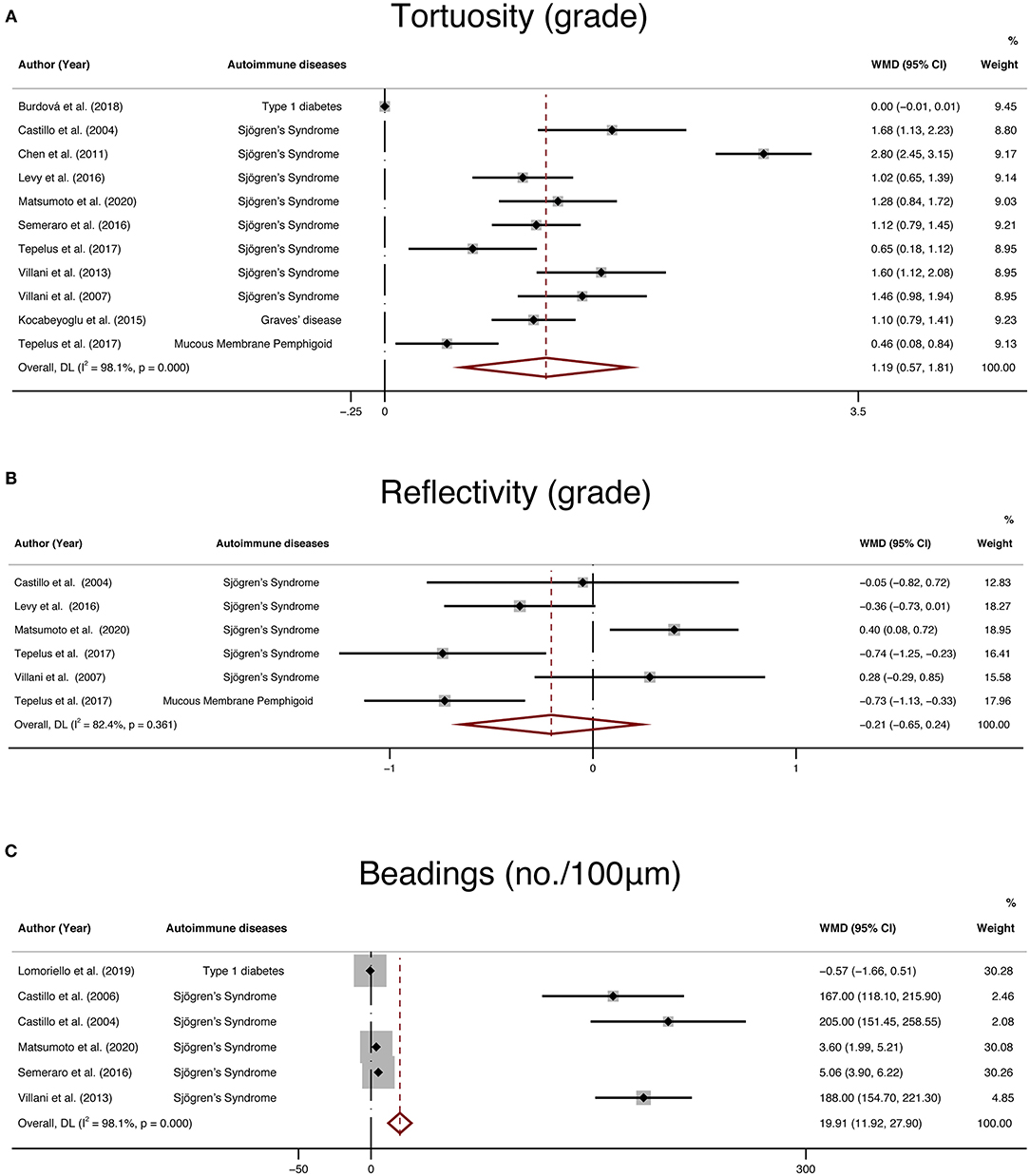
Figure 9. Forest plot of the WMD of tortuosity (A), reflectivity (B), and beadings (C) between the NNAI group and the control group. WMD, weighted mean difference; CI, confidence interval; NNAI, non-neurological autoimmune (diseases).
To further assess the reliability of our results, we also performed funnel plots (Figure 10), as well as Egger's linear regression tests and Begg's rank association tests (Table 4) to estimated publication bias. Sensitivity analysis of studies that reported beadings of corneal nerve per 100 μm showed that four out of six studies may have excessive influence on the above-mentioned pooled effect, and results of tortuosity and reflectivity showed that no individual study had an excessive influence on the above-mentioned pooled effect (Figure 11). Funnel plots of tortuosity and beadings were visually asymmetric, suggesting possible publication bias. Egger's test also showed that there may be a publication bias on studies reported on tortuosity and beadings. After using the trim and fill methods, the pooled result of tortuosity was not changed while that of beadings was quite different from the original results. According to our study, the publication bias did not influence the overall results of tortuosity but did interfere with the overall result of beadings.
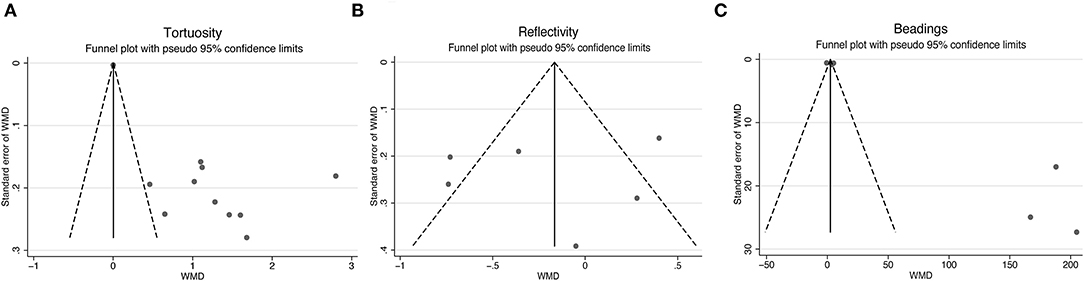
Figure 10. Funnel plots for studies included reported tortuosity (A), reflectivity (B), and beadings (C). WMD, weighted mean difference.
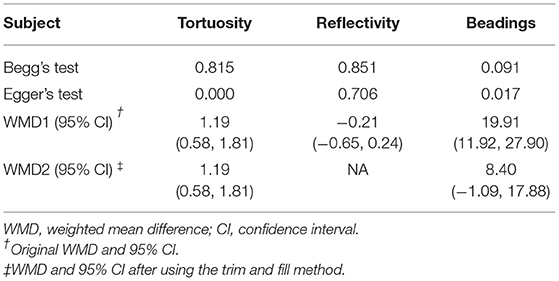
Table 4. Publication bias measured by Begg's and Egger's test, WMD (95% CI) recalculated with trim and fill method.
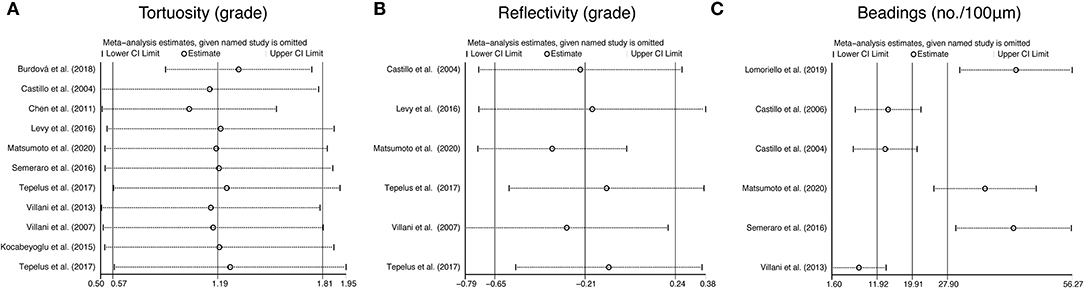
Figure 11. Sensitivity analysis data for studies included reported tortuosity (A), reflectivity (B), and beadings (C).
Discussion
The cornea, as the front portion of the ocular surface, plays an important role in the visual system. Its integrity is crucial for the health and normal function of the eye, and its delicate mucosal immune system was extremely vulnerable to autoimmune dysregulation so that the cornea is able to detect and repair the damage promptly. It was reported that assessment of corneal nerve parameters has become one of the most common clinical tests to evaluate ocular surface symptoms in many kinds of diseases (63). IVCM provides a direct and non-invasive tool to observe corneal nerve morphology and assess corneal nerve parameters. NNAI diseases, to our knowledge, are a range of diseases with abnormal autoimmune reactions including varied manifestations on the ocular surface. Many researchers reported that the involvement of the cornea may be an initial manifestation of some of the autoimmune diseases and may be sight-threatening if not well treated (64–66). As one of the most densely innervated parts of the human body, the corneal nerve may serve as a marker of some diseases with its morphological alternation.
In the pathology of NNAI diseases, the exact etiological and pathophysiological mechanisms are often unknown. However, many researchers found that elevating inflammatory mediators, such as IL1-beta, IL6, IL8, and TNF-alpha might play an important role in autoimmune patients with small fiber neuropathy. Reducing mechanical nociceptive thresholds and dysesthesias were also found to be associated with higher IL1-beta and TNF-alpha concentrations (67–69). The corneal nerves, as one kind of small nerve fibers, may share the same mechanisms to some extent. Patients with Sjögren's syndrome, systemic lupus erythematosus, or rheumatoid arthritis, for instance, were found to have local increasing lymphocytes in the cornea which implied inflammatory infiltration in corneal nerve fibers (70). Recently, researchers using Mouse models of type 1 diabetes found that decreasing neutrophil infiltration and reducing expression of IL1-beta and TNF-alpha could prevent corneal nerve loss (71, 72). In this way, we speculate that inflammatory mediators may be one reason why a similar pattern of corneal nerve loss occurs in NNAI diseases. Other mechanisms such as metabolic, infectious, and genetic factors may also take part, but the exact pathophysiological mechanisms would need future explorations.
Various works of research had proved that corneal IVCM could be a sensitive evaluation tool in early diabetic peripheral neuropathy and might be clinically useful to diagnosis and surveillance of other neuropathies (48, 73, 74). It is plausible that the alteration of the corneal nerve under IVCM may be a tool to identify NNAI diseases. The other way around, the effect of NNAI diseases on the corneal nerve might be the reason why ocular symptoms were commonly presented among NNAI patients. It is well acknowledged that the corneal nerve helps maintain a well-lubricated and smooth eye surface not only by inducing tear production but also by stimulating the blinking reflex through an elaborate interaction between the corneal surface and lacrimal glands (75). Therefore, damage of the corneal nerve may be associated with the ocular sicca symptoms usually seen and more severe in many NNAI diseases (76–78).
However, many of the previous studies are limited in sample size and their results were contradictory. There is a lack of analytical summary to evaluate the change of corneal nerves in a certain spectrum of NNAI diseases. In this case, a meta-analysis is a powerful tool to summarize results from different studies by providing a more objective evaluation of the major effect with enhanced accuracy and to explain the heterogeneity between different studies. To the best of our knowledge, this is the first meta-analysis to investigate the corneal nerve parameters using IVCM in patients with NNAI and control groups. Our analysis showed significantly decreased CNFL (WMD: −3.94, 95% CI: −4.77–−3.12), CNFD (WMD: −6.62, 95% CI: −8.4–−4.85), CNBD (WMD: −9.89, 95% CI: −14–−5.79) in NNAI groups. However, there was significant heterogeneity of three sets of parameters mentioned above among the studies included. Sensitivity analysis, creation of funnel plots, Egger's test, Begg's test, and the trim and fill methods were performed to confirm the reliability of the results. And the analysis stratified by age, type of IVCM, software used, and types of NNAI diseases, were performed to assess between-study heterogeneity. However, subgroup results showed no potential source of heterogeneity. In the article of Roszkowska et al., it was concluded that corneal nerve changes in diabetes examined by IVCM are related to HbA1c level, diabetes duration, the progress of diabetic retinopathy, and race (79). It is possible that factors such as the severity or duration of NNAI, racial differences in participants, male-female distribution, the acquisition mode with IVCM, or the number of images analyzed per participant, might cause heterogeneity. Due to the incomparability and incompleteness of data, the effect of these above-mentioned potential factors on between-study heterogeneity could not be further examined. All in all, our meta-analysis included thirty-seven studies and with analysis of a large sample size, had shown a significant decrease in CNFL, CNFD, and CNBD among NNAI patients.
In addition, it is interesting that results showed patients with Sjögren's syndrome had a greater reduction in CNFD and minimal impact on CNBD, and consequent comparable reduction in CNFL. In many diseases affecting corneal nerves, CNBD was found to be elevated rather than reduced as subconsciously assumed. For instance, the pattern of corneal nerves appeared to be unique in Parkinson's disease with reduced CNFD and a markedly increased CNBD (80, 81). Similarly, a study demonstrated enhanced CNBD and reduced CNFD and CNFL in patients with painful diabetic neuropathy (82). In addition, increased CNBD was also found to be the first sign to indicate regeneration after simultaneous pancreas and kidney transplantation or continuous subcutaneous insulin infusion in type 1 diabetes (83, 84). All studies mentioned above supported the hypothesis that enhanced CNBD signified some preserved susceptibility of corneal nerve fibers toward regeneration and attempts to repair, but the attempts as yet appeared insufficient to culminate in an increased CNFD or CNFL. Consequently, we consider that CNBD's attempt at regeneration may to some extent compensate for the reduced CNBD by injury. As for CNFL and CNFD, to our knowledge, it is proved that CNFL has been shown to have the best reproducibility and consistency compared to CNFD and CNBD for detecting early preclinical small fiber damage (54, 79, 85, 86). This may indicate that CNFL is most susceptible to damage from various diseases, but our results presented contradictory. It is strange and hard to explain, and maybe knowing the exact pathology of Sjögren's syndrome would help explain it. However, at present, studies on corneal nerve alternation of patients are mainly in vivo studies, which means that we can basically only carry out some non-invasive detections like corneal confocal, corneal sensation, biological fluid detection, etc. Although these detections prove to be very promising ways to an early small fiber neuropathy diagnosis (87), the exact pathophysiology and signaling pathways activated in diseases remain unknown due to invasive procedures that cannot be performed in vivo. Moving forward, more future research is needed for a deeper understanding.
Besides CNFL, CNFD, and CNBD, tortuosity, reflectivity, and beadings are also important parameters to describe corneal nerve morphology. According to our analysis, the corneal nerve of the NNAI group presented more beadings per 100 μm (WMD: 19.91, 95% CI: 11.92–27.9) and was more tortuous (WMD: 1.19, 95% CI:0.57–1.81) than that of the control group, while there seemed to be no statistical difference on corneal nerve reflectivity (WMD: −0.21, 95% CI: −0.65–0.24, P = 0.361) between two groups. However, the results of subjective parameters like tortuosity and beadings in our analysis seem to be less convincing according to publication bias analysis. There could be due to many reasons. One of the reasons may be that these subjective parameters are infrequently reported in the included literature, resulting in a small sample size of data. Another reason may be that measurement of subjective parameters is not uniform across studies. For instance, some studies reported corneal nerve tortuosity according to previously validated grading scales, while others used tortuosity coefficient (47). Besides, the interpretations of the results by these subjective parameters rely a lot on researchers' subjective judgment and observers' experience, which made the results less comparable.
Nevertheless, we can't deny the promising function of subjective parameters in predicting corneal nerve neuropathy. Indeed, according to research examining corneal nerves in patients with type 2 diabetes (88), the size and number of beadings had the best sensitivity and specificity to predict the dysfunctions of the peripheral neuropathy compared with CNFD, CNFL. Similarly, a previous study among glaucoma patients showed that tortuosity and beadings directly correlated with corneal nerve function (89). In recent years, software and methods have been developed to obtain more objective and reproducible evaluations of tortuosity (90, 91). For example, a study proposed an automatic algorithm that was able to correctly trace more than 80% of the recognizable nerve fibers in the images and proved its clinical validity regarding tortuosity measure (92). We believe that in the future, more accurate software will help make these subjective parameters more comparable among various studies and more practical in clinical performance.
The present study has some limitations that should be considered. Firstly, the types of NNAI included in our study were mostly typed 1 diabetes and Sjögren's Syndrome, which might not be representative of NNAI in general. Many other NNAI diseases were reported presenting ocular manifestation as the initial manifestation like rheumatoid arthritis and systemic lupus erythematosus. It is reasonable to infer that alteration in corneal innervation also occurs in these diseases, but it is a pity that we did not find qualified studies for every NNAI disease that could be included in the meta-analysis. And we look forward to more research about morphological alternation of the corneal nerve of NNAI so we may draw a more reliable conclusion. Secondly, although IVCM has already been widely used in clinical practice, there is still a lack of a gold standard for corneal nerve parameters. For example, the majority of studies have defined CNFL as the total length of nerves visible within a defined area in mm/mm2 while some only measured nerve branches longer than 50 μm or analyzed the total length of nerves within a frame (93–97). Other factors contributing to the non-uniform assessment may include. (1) Each image captured by IVCM represents only approximately 0.2% of the average corneal surface which might give out non-representative images and result in misleading inferences (7, 98). (2) A possible correlation between myopic refractive error and CNFL might be neglected among our included articles that assess corneal nerves (99). (3) According to instrument design, IVCM can be generally divided into tandem scanning confocal microscopy, laser scanning confocal microscopy, and slit scanning confocal microscopy (100). Different kinds of confocal microscopy are equipped with different field brightness and contrast which may affect the apparent thickness of corneal nerves, particularly when they approach the limit of resolution, thus influencing the uniformity among different studies (97, 101). (4) IVCM image processing could be performed by different methods, including manual tracing, ImageJ, the CCMetrics system, the ACCMetrics system, etc (102, 103). The inconsistency of image-processing methods and the subjectivity during the image-analyzing procedure among different studies may also result in significant discrepancy and heterogeneity.
In conclusion, this meta-analysis suggested that corneal nerve parameters (CNFL, CNFD, CNBD) might be clinical markers for NNAI diseases, while our analysis of other morphology indicators (tortuosity, reflectivity, beadings) lack reliable conclusion from the included studies. Future longitudinal studies could delve into the role of IVCM as a promising way to diagnose and evaluate NNAI diseases.
Data Availability Statement
The original contributions presented in the study are included in the article/supplementary material, further inquiries can be directed to the corresponding authors.
Author Contributions
YG, XL, and XY conceived of the study, carried out the literature search, extracted the data, and performed the statistical analysis. NY and WK conducted the quality assessment. YG, XL, and QQ were involved in revising and modification of the manuscript. KW and MC directed the project, reviewed, and revised the manuscript. All authors have contributed significantly and agree with the content of the manuscript. All authors read and approved the final manuscript.
Funding
This study was supported by the Zhejiang Provincial Natural Science Foundation of China (Nos. LGF20H120003 and LY19H120006) and the National Natural Science Foundation of China (No. 82171045).
Conflict of Interest
The authors declare that the research was conducted in the absence of any commercial or financial relationships that could be construed as a potential conflict of interest.
Publisher's Note
All claims expressed in this article are solely those of the authors and do not necessarily represent those of their affiliated organizations, or those of the publisher, the editors and the reviewers. Any product that may be evaluated in this article, or claim that may be made by its manufacturer, is not guaranteed or endorsed by the publisher.
References
1. Karagianni P, Tzioufas AG. Epigenetic perspectives on systemic autoimmune disease. J Autoimmun. (2019) 104:102315. doi: 10.1016/j.jaut.2019.102315
2. Cooper GS, Bynum ML, Somers EC. Recent insights in the epidemiology of autoimmune diseases: improved prevalence estimates and understanding of clustering of diseases. J Autoimmun. (2009) 33:197–207. doi: 10.1016/j.jaut.2009.09.008
3. Stojanovich L, Marisavljevich D. Stress as a trigger of autoimmune disease. Autoimmun Rev. (2008) 7:209–13. doi: 10.1016/j.autrev.2007.11.007
4. Saccucci M, Di Carlo G, Bossù M, Giovarruscio F, Salucci A, Polimeni A. Autoimmune diseases and their manifestations on oral cavity: diagnosis and clinical management. J Immunol Res. (2018) 2018:6061825. doi: 10.1155/2018/6061825
5. Bodolay E, Dérfalvi B, Gergely P, Poór G. When does an autoimmune disease begin? Importance of the early diagnosis. Orv Hetil. (2007) 148 Suppl 1:25–30. doi: 10.1556/oh.2007.28031
6. Yang AY, Chow J, Liu J. Corneal Innervation and Sensation: the eye and beyond. Yale J Biol Med. (2018) 91:13–21.
7. Kokot J, Wylegała A, Wowra B, Wójcik Ł, Dobrowolski D, Wylegała E. Corneal confocal sub-basal nerve plexus evaluation: a review. Acta Ophthalmol. (2018) 96:232–42. doi: 10.1111/aos.13518
8. Freissler KA, Lang GE. The cornea and systemic diseases. Curr Opin Ophthalmol. (1996) 7:22–7. doi: 10.1097/00055735-199608000-00005
9. Karpik AG, Schwartz MM, Dickey LE, Streeten BW, Roberts JL. Ocular immune reactants in patients dying with systemic lupus erythematosus. Clin Immunol Immunopathol. (1985) 35:295–312. doi: 10.1016/0090-1229(85)90091-1
10. Basantsova NY, Starshinova AA, Dori A, Zinchenko YS, Yablonskiy PK, Shoenfeld Y. Small-fiber neuropathy definition, diagnosis, and treatment. Neurol Sci. (2019) 40:1343–50. doi: 10.1007/s10072-019-03871-x
11. Harboe E, Tjensvoll AB, Maroni S, Gøransson LG, Greve OJ, Beyer MK, et al. Neuropsychiatric syndromes in patients with systemic lupus erythematosus and primary Sjögren syndrome: a comparative population-based study. Ann Rheum Dis. (2009) 68:1541–6. doi: 10.1136/ard.2008.098301
12. Lopate G, Pestronk A, Al-Lozi M, Lynch T, Florence J, Miller T, et al. Peripheral neuropathy in an outpatient cohort of patients with Sjögren's syndrome. Muscle Nerve. (2006) 33:672–6. doi: 10.1002/mus.20514
13. Ryabkova VA, Churilov LP, Shoenfeld Y. Neuroimmunology: What Role for Autoimmunity, Neuroinflammation, and Small Fiber Neuropathy in Fibromyalgia, Chronic Fatigue Syndrome, and Adverse Events after Human Papillomavirus Vaccination? Int J Mol Sci. (2019) 20:5164. doi: 10.3390/ijms20205164
14. Lim SH, Ferdousi M, Kalteniece A, Kass-Iliyya L, Petropoulos IN, Malik RA, et al. Corneal confocal microscopy detects small fibre neurodegeneration in Parkinson's disease using automated analysis. Sci Rep. (2020) 10:20147. doi: 10.1038/s41598-020-76768-x
15. Pagovich OE, Vo ML, Zhao ZZ, Petropoulos IN, Yuan M, Lertsuwanroj B, et al. Corneal confocal microscopy: Neurologic disease biomarker in Friedreich ataxia. Ann Neurol. (2018) 84:893–904. doi: 10.1002/ana.25355
16. Szalai E, Deák E, Módis L Jr, Németh G, Berta A, Nagy A, et al. Early Corneal Cellular and Nerve Fiber Pathology in Young Patients With Type 1 Diabetes Mellitus Identified Using Corneal Confocal Microscopy. Invest Ophthalmol Vis Sci. (2016) 57:853–8. doi: 10.1167/iovs.15-18735
17. Bitirgen G, Akpinar Z, Uca AU, Ozkagnici A, Petropoulos IN, Malik RA. Progressive loss of corneal and retinal nerve fibers in patients with multiple sclerosis: a 2-year follow-up study. Transl Vis Sci Technol. (2020) 9:37. doi: 10.1167/tvst.9.13.37
18. Bitirgen G, Akpinar Z, Malik RA, Ozkagnici A. Use of corneal confocal microscopy to detect corneal nerve loss and increased dendritic cells in patients with multiple sclerosis. JAMA Ophthalmol. (2017) 135:777–82. doi: 10.1001/jamaophthalmol.2017.1590
19. Cruzat A, Qazi Y, Hamrah P. In Vivo Confocal Microscopy of Corneal Nerves in Health and Disease. Ocul Surf. (2017) 15:15–47. doi: 10.1016/j.jtos.2016.09.004
20. Cullen AE, Holmes S, Pollak TA, Blackman G, Joyce DW, Kempton MJ, et al. Associations between non-neurological autoimmune disorders and psychosis: a meta-analysis. Biol Psychiatry. (2019) 85:35–48. doi: 10.1016/j.biopsych.2018.06.016
21. Tavakoli M, Quattrini C, Abbott C, Kallinikos P, Marshall A, Finnigan J, et al. Corneal confocal microscopy: a novel noninvasive test to diagnose and stratify the severity of human diabetic neuropathy. Diabetes Care. (2010) 33:1792–7. doi: 10.2337/dc10-0253
22. Messmer EM, Schmid-Tannwald C, Zapp D, Kampik A. In vivo confocal microscopy of corneal small fiber damage in diabetes mellitus. Graefes Arch Clin Exp Ophthalmol. (2010) 248:1307–12. doi: 10.1007/s00417-010-1396-8
23. Chang PY, Carrel H, Huang JS, Wang IJ, Hou YC, Chen WL, et al. Decreased density of corneal basal epithelium and subbasal corneal nerve bundle changes in patients with diabetic retinopathy. Am J Ophthalmol. (2006) 142:488–90. doi: 10.1016/j.ajo.2006.04.033
24. Oliveira-Soto L, Efron N. Morphology of corneal nerves using confocal microscopy. Cornea. (2001) 20:374–84. doi: 10.1097/00003226-200105000-00008
25. Egger M, Davey Smith G, Schneider M, Minder C. Bias in meta-analysis detected by a simple, graphical test. BMJ. (1997) 315:629–34. doi: 10.1136/bmj.315.7109.629
26. Begg CB, Mazumdar M. Operating characteristics of a rank correlation test for publication bias. Biometrics. (1994) 50:1088–101. doi: 10.2307/2533446
27. Gad H, Al-Jarrah B, Saraswathi S, Mohamed S, Kalteniece A, Petropoulos IN, et al. Corneal confocal microscopy identifies a reduction in corneal keratocyte density and sub-basal nerves in children with type 1 diabetes mellitus. Br J Ophthalmol. (2021). doi: 10.1136/bjophthalmol-2021-319057
28. D'Onofrio L, Kalteniece A, Ferdousi M, Azmi S, Petropoulos IN, Ponirakis G, et al. Small Nerve Fiber Damage and Langerhans Cells in Type 1 and Type 2 Diabetes and LADA Measured by Corneal Confocal Microscopy. Invest Ophthalmol Vis Sci. (2021) 62:5. doi: 10.1167/iovs.62.6.5
29. Cozzini T, Piona C, Marchini G, Merz T, Brighenti T, Bonetto J, et al. In vivo confocal microscopy study of corneal nerve alterations in children and youths with Type 1 diabetes. Pediatr Diabetes. (2021) 22:780–6. doi: 10.1111/pedi.13219
30. Barcelos F, Hipólito-Fernandes D, Martins C, Ângelo-Dias M, Cardigos J, Monteiro R, et al. Corneal sub-basal nerve plexus assessment and its association with phenotypic features and lymphocyte subsets in Sjögren's Syndrome. Acta Ophthalmol. (2021) 99:e1315. doi: 10.1111/aos.14811
31. Tummanapalli SS, Issar T, Kwai N, Poynten A, Krishnan AV, Willcox M, et al. Association of corneal nerve loss with markers of axonal ion channel dysfunction in type 1 diabetes. Clin Neurophysiol. (2020) 131:145–54. doi: 10.1016/j.clinph.2019.09.029
32. Matsumoto Y, Ibrahim OMA, Kojima T, Dogru M, Shimazaki J, Tsubota K. Corneal In Vivo Laser-Scanning Confocal Microscopy Findings in Dry Eye Patients with Sjögren's Syndrome. Diagnostics (Basel). (2020) 10:497. doi: 10.3390/diagnostics10070497
33. Gad H, Saraswathi S, Al-Jarrah B, Petropoulos IN, Ponirakis G, Khan A, et al. Corneal confocal microscopy demonstrates minimal evidence of distal neuropathy in children with celiac disease. PLoS ONE. (2020) 15:e0238859. doi: 10.1371/journal.pone.0238859
34. Schiano Lomoriello D, Abicca I, Parravano M, Giannini D, Russo B, Frontoni S, et al. Early Alterations of Corneal Subbasal Plexus in Uncomplicated Type 1 Diabetes Patients. J Ophthalmol. (2019) 2019:9818217. doi: 10.1155/2019/9818217
35. Ferdousi M, Romanchuk K, Mah JK, Virtanen H, Millar C, Malik RA, et al. Early corneal nerve fibre damage and increased Langerhans cell density in children with type 1 diabetes mellitus. Sci Rep. (2019) 9:8758. doi: 10.1038/s41598-019-45116-z
36. Sharma S, Tobin V, Vas PRJ, Rayman G. The LDIFLARE and CCM methods demonstrate early nerve fiber abnormalities in untreated hypothyroidism: a prospective study. J Clin Endocrinol Metab. (2018) 103:3094–102. doi: 10.1210/jc.2018-00671
37. Ferdousi M, Petropoulos IN, Kalteniece A, Azmi S, Ponirakis G, Efron N, et al. No Relation between the severity of corneal nerve, epithelial, and keratocyte cell morphology with measures of dry eye disease in type 1 diabetes. Invest Ophthalmol Vis Sci. (2018) 59:5525–30. doi: 10.1167/iovs.18-25321
38. Chen X, Graham J, Petropoulos IN, Ponirakis G, Asghar O, Alam U, et al. Corneal nerve fractal dimension: a novel corneal nerve metric for the diagnosis of diabetic sensorimotor polyneuropathy. Invest Ophthalmol Vis Sci. (2018) 59:1113–8. doi: 10.1167/iovs.17-23342
39. Ceská Burdová M, Kulich M, Dotrelová D, Mahelková G. Effect of diabetes mellitus type 1 diagnosis on the corneal cell densities and nerve fibers. Physiol Res. (2018) 67:963–74. doi: 10.33549/physiolres.933899
40. Bitirgen G, Tinkir Kayitmazbatir E, Satirtav G, Malik RA, Ozkagnici A. In Vivo Confocal Microscopic Evaluation of Corneal Nerve Fibers and Dendritic Cells in Patients With Behçet's Disease. Front Neurol. (2018) 9:204. doi: 10.3389/fneur.2018.00204
41. Tepelus TC, Huang J, Sadda SR, Lee OL. Characterization of Corneal Involvement in Eyes With Mucous Membrane Pemphigoid by In Vivo Confocal Microscopy. Cornea. (2017) 36:933–41. doi: 10.1097/ICO.0000000000001201
42. Tepelus TC, Chiu GB, Huang J, Huang P, Sadda SR, Irvine J, et al. Correlation between corneal innervation and inflammation evaluated with confocal microscopy and symptomatology in patients with dry eye syndromes: a preliminary study. Graefes Arch Clin Exp Ophthalmol. (2017) 255:1771–8. doi: 10.1007/s00417-017-3680-3
43. Scarr D, Lovblom LE, Ostrovski I, Kelly D, Wu T, Farooqi MA, et al. Agreement between automated and manual quantification of corneal nerve fiber length: Implications for diabetic neuropathy research. J Diabetes Complications. (2017) 31:1066–73. doi: 10.1016/j.jdiacomp.2016.07.024
44. Levy O, Labbé A, Borderie V, Hamiche T, Dupas B, Laroche L, et al. Increased corneal sub-basal nerve density in patients with Sjögren syndrome treated with topical cyclosporine A. Clin Exp Ophthalmol. (2017) 45:455–63. doi: 10.1111/ceo.12898
45. Alam U, Jeziorska M, Petropoulos IN, Asghar O, Fadavi H, Ponirakis G, et al. Diagnostic utility of corneal confocal microscopy and intra-epidermal nerve fibre density in diabetic neuropathy. PLoS ONE. (2017) 12:e0180175. doi: 10.1371/journal.pone.0180175
46. Semeraro F, Forbice E, Nascimbeni G, Taglietti M, Romano V, Guerra G, et al. Effect of Autologous Serum Eye Drops in Patients with Sjögren Syndrome-related Dry Eye: Clinical and In Vivo Confocal Microscopy Evaluation of the Ocular Surface. In Vivo. (2016) 30:931–8. doi: 10.21873/invivo.11016
47. McNamara NA, Ge S, Lee SM, Enghauser AM, Kuehl L, Chen FY, et al. Reduced Levels of Tear Lacritin Are Associated With Corneal Neuropathy in Patients With the Ocular Component of Sjögren's Syndrome. Invest Ophthalmol Vis Sci. (2016) 57:5237–43. doi: 10.1167/iovs.16-19309
48. Misra SL, Craig JP, Patel DV, McGhee CN, Pradhan M, Ellyett K, et al. In vivo confocal microscopy of corneal nerves: an ocular biomarker for peripheral and cardiac autonomic neuropathy in type 1 diabetes mellitus. Invest Ophthalmol Vis Sci. (2015) 56:5060–5. doi: 10.1167/iovs.15-16711
49. Kocabeyoglu S, Mocan MC, Cevik Y, Irkec M. Ocular Surface Alterations and In Vivo Confocal Microscopic Features of Corneas in Patients With Newly Diagnosed Graves' Disease. Cornea. (2015) 34:745–9. doi: 10.1097/ICO.0000000000000426
50. Chen X, Graham J, Dabbah MA, Petropoulos IN, Ponirakis G, Asghar O, et al. Small nerve fiber quantification in the diagnosis of diabetic sensorimotor polyneuropathy: comparing corneal confocal microscopy with intraepidermal nerve fiber density. Diabetes Care. (2015) 38:1138–44. doi: 10.2337/dc14-2422
51. Stem MS, Hussain M, Lentz SI, Raval N, Gardner TW, Pop-Busui R, et al. Differential reduction in corneal nerve fiber length in patients with type 1 or type 2 diabetes mellitus. J Diabetes Complications. (2014) 28:658–61. doi: 10.1016/j.jdiacomp.2014.06.007
52. Pritchard N, Edwards K, Dehghani C, Fadavi H, Jeziorska M, Marshall A, et al. Longitudinal assessment of neuropathy in type 1 diabetes using novel ophthalmic markers (LANDMark): study design and baseline characteristics. Diabetes Res Clin Pract. (2014) 104:248–56. doi: 10.1016/j.diabres.2014.02.011
53. Villani E, Magnani F, Viola F, Santaniello A, Scorza R, Nucci P, et al. In vivo confocal evaluation of the ocular surface morpho-functional unit in dry eye. Optom Vis Sci. (2013) 90:576–86. doi: 10.1097/OPX.0b013e318294c184
54. Ahmed A, Bril V, Orszag A, Paulson J, Yeung E, Ngo M, et al. Detection of diabetic sensorimotor polyneuropathy by corneal confocal microscopy in type 1 diabetes: a concurrent validity study. Diabetes Care. (2012) 35:821–8. doi: 10.2337/dc11-1396
55. Hertz P, Bril V, Orszag A, Ahmed A, Ng E, Nwe P, et al. Reproducibility of in vivo corneal confocal microscopy as a novel screening test for early diabetic sensorimotor polyneuropathy. Diabet Med. (2011) 28:1253–60. doi: 10.1111/j.1464-5491.2011.03299.x
56. Chen Q, Zhang X, Cui L, Huang Q, Chen W, Ma H, et al. Upper and lower tear menisci in Sjögren's syndrome dry eye. Invest Ophthalmol Vis Sci. (2011) 52:9373–8. doi: 10.1167/iovs.11-7431
57. Tuisku IS, Konttinen YT, Konttinen LM, Tervo TM. Alterations in corneal sensitivity and nerve morphology in patients with primary Sjögren's syndrome. Exp Eye Res. (2008) 86:879–85. doi: 10.1016/j.exer.2008.03.002
58. Villani E, Galimberti D, Viola F, Mapelli C, Ratiglia R. The cornea in Sjogren's syndrome: an in vivo confocal study. Invest Ophthalmol Vis Sci. (2007) 48:2017–22. doi: 10.1167/iovs.06-1129
59. Benítez-Del-Castillo JM, Acosta MC, Wassfi MA, Díaz-Valle D, Gegúndez JA, Fernandez C, et al. Relation between corneal innervation with confocal microscopy and corneal sensitivity with noncontact esthesiometry in patients with dry eye. Invest Ophthalmol Vis Sci. (2007) 48:173–81. doi: 10.1167/iovs.06-0127
60. Benítez del Castillo JM, Wasfy MA, Fernandez C, Garcia-Sanchez J. An in vivo confocal masked study on corneal epithelium and subbasal nerves in patients with dry eye. Invest Ophthalmol Vis Sci. (2004) 45:3030–5. doi: 10.1167/iovs.04-0251
61. Tuominen IS, Konttinen YT, Vesaluoma MH, Moilanen JA, Helintö M, Tervo TM. Corneal innervation and morphology in primary Sjögren's syndrome. Invest Ophthalmol Vis Sci. (2003) 44:2545–9. doi: 10.1167/iovs.02-1260
62. Li F, Zhang Q, Ying X, He J, Jin Y, Xu H, et al. Corneal nerve structure in patients with primary Sjögren's syndrome in China. BMC Ophthalmol. (2021) 21:211. doi: 10.1186/s12886-021-01967-7
63. Palomar APD, Montolío A, Cegoñino J, Dhanda SK, Lio CT, Bose T. The Innate Immune Cell Profile of the Cornea Predicts the Onset of Ocular Surface Inflammatory Disorders. J Clin Med. (2019) 8:2110. doi: 10.3390/jcm8122110
64. McCluskey P, Powell RJ. The eye in systemic inflammatory diseases. Lancet. (2004) 364:2125–33. doi: 10.1016/S0140-6736(04)17554-5
65. Ladas JG, Mondino BJ. Systemic disorders associated with peripheral corneal ulceration. Curr Opin Ophthalmol. (2000) 11:468–71. doi: 10.1097/00055735-200012000-00014
66. Messmer EM, Foster CS. Vasculitic peripheral ulcerative keratitis. Surv Ophthalmol. (1999) 43:379–96. doi: 10.1016/S0039-6257(98)00051-4
67. Uçeyler N, Kafke W, Riediger N, He L, Necula G, Toyka KV, et al. Elevated proinflammatory cytokine expression in affected skin in small fiber neuropathy. Neurology. (2010) 74:1806–13. doi: 10.1212/WNL.0b013e3181e0f7b3
68. Ferreira SH, Lorenzetti BB, Bristow AF, Poole S. Interleukin-1 beta as a potent hyperalgesic agent antagonized by a tripeptide analogue. Nature. (1988) 334:698–700. doi: 10.1038/334698a0
69. Cunha FQ, Poole S, Lorenzetti BB, Ferreira SH. The pivotal role of tumour necrosis factor alpha in the development of inflammatory hyperalgesia. Br J Pharmacol. (1992) 107:660–4. doi: 10.1111/j.1476-5381.1992.tb14503.x
70. Wang H, Wang PB, Chen T, Zou J, Li YJ, Ran XF, et al. Analysis of clinical characteristics of immune-related dry eye. J Ophthalmol. (2017) 2017:8532397. doi: 10.1155/2017/8532397
71. Zhang Z, Hu X, Qi X, Di G, Zhang Y, Wang Q, et al. Resolvin D1 promotes corneal epithelial wound healing and restoration of mechanical sensation in diabetic mice. Mol Vis. (2018) 24:274–85.
72. Hou Y, Xin M, Li Q, Wu X. Glycyrrhizin micelle as a genistein nanocarrier: Synergistically promoting corneal epithelial wound healing through blockage of the HMGB1 signaling pathway in diabetic mice. Exp Eye Res. (2021) 204:108454. doi: 10.1016/j.exer.2021.108454
73. Tavakoli M, Petropoulos IN, Malik RA. Corneal confocal microscopy to assess diabetic neuropathy: an eye on the foot. J Diabetes Sci Technol. (2013) 7:1179–89. doi: 10.1177/193229681300700509
74. Petropoulos IN, Green P, Chan AW, Alam U, Fadavi H, Marshall A, et al. Corneal confocal microscopy detects neuropathy in patients with type 1 diabetes without retinopathy or microalbuminuria. PLoS ONE. (2015) 10:e0123517. doi: 10.1371/journal.pone.0123517
75. Stern ME, Gao J, Siemasko KF, Beuerman RW, Pflugfelder SC. The role of the lacrimal functional unit in the pathophysiology of dry eye. Exp Eye Res. (2004) 78:409–16. doi: 10.1016/j.exer.2003.09.003
76. Turk MA, Hayworth JL, Nevskaya T, Pope JE. Ocular manifestations in rheumatoid arthritis, connective tissue disease, and vasculitis: a systematic review and metaanalysis. J Rheumatol. (2021) 48:25–34. doi: 10.3899/jrheum.190768
77. Palejwala NV, Walia HS, Yeh S. Ocular manifestations of systemic lupus erythematosus: a review of the literature. Autoimmune Dis. (2012) 2012:290898. doi: 10.1155/2012/290898
78. Marsovszky L, Resch MD, Németh J, Toldi G, Medgyesi E, Kovács L, et al. In vivo confocal microscopic evaluation of corneal Langerhans cell density, and distribution and evaluation of dry eye in rheumatoid arthritis. Innate Immun. (2013) 19:348–54. doi: 10.1177/1753425912461677
79. Roszkowska AM, Licitra C, Tumminello G, Postorino EI, Colonna MR, Aragona P. Corneal nerves in diabetes-The role of the in vivo corneal confocal microscopy of the subbasal nerve plexus in the assessment of peripheral small fiber neuropathy. Surv Ophthalmol. (2021) 66:493–513. doi: 10.1016/j.survophthal.2020.09.003
80. Nolano M, Provitera V, Estraneo A, Selim MM, Caporaso G, Stancanelli A, et al. Sensory deficit in Parkinson's disease: evidence of a cutaneous denervation. Brain. (2008) 131(Pt 7):1903–11. doi: 10.1093/brain/awn102
81. Kass-Iliyya L, Javed S, Gosal D, Kobylecki C, Marshall A, Petropoulos IN, et al. Small fiber neuropathy in Parkinson's disease: a clinical, pathological and corneal confocal microscopy study. Parkinsonism Relat Disord. (2015) 21:1454–60. doi: 10.1016/j.parkreldis.2015.10.019
82. Püttgen S, Bönhof GJ, Strom A, Müssig K, Szendroedi J, Roden M, et al. Augmented corneal nerve fiber branching in painful compared with painless diabetic neuropathy. J Clin Endocrinol Metab. (2019) 104:6220–8. doi: 10.1210/jc.2019-01072
83. Tavakoli M, Mitu-Pretorian M, Petropoulos IN, Fadavi H, Asghar O, Alam U, et al. Corneal confocal microscopy detects early nerve regeneration in diabetic neuropathy after simultaneous pancreas and kidney transplantation. Diabetes. (2013) 62:254–60. doi: 10.2337/db12-0574
84. Azmi S, Ferdousi M, Petropoulos IN, Ponirakis G, Fadavi H, Tavakoli M, et al. Corneal confocal microscopy shows an improvement in small-fiber neuropathy in subjects with type 1 diabetes on continuous subcutaneous insulin infusion compared with multiple daily injection. Diabetes Care. (2015) 38:e3–4. doi: 10.2337/dc14-1698
85. Petropoulos IN, Manzoor T, Morgan P, Fadavi H, Asghar O, Alam U, et al. Repeatability of in vivo corneal confocal microscopy to quantify corneal nerve morphology. Cornea. (2013) 32:e83–9. doi: 10.1097/ICO.0b013e3182749419
86. Edwards K, Pritchard N, Vagenas D, Russell A, Malik RA, Efron N. Utility of corneal confocal microscopy for assessing mild diabetic neuropathy: baseline findings of the LANDMark study. Clin Exp Optom. (2012) 95:348–54. doi: 10.1111/j.1444-0938.2012.00740.x
87. Terkelsen AJ, Karlsson P, Lauria G, Freeman R, Finnerup NB, Jensen TS. The diagnostic challenge of small fibre neuropathy: clinical presentations, evaluations, and causes. Lancet Neurol. (2017) 16:934–44. doi: 10.1016/S1474-4422(17)30329-0
88. Ishibashi F, Kojima R, Taniguchi M, Kosaka A, Uetake H, Tavakoli M. The Expanded Bead Size of Corneal C-Nerve Fibers Visualized by Corneal Confocal Microscopy Is Associated with Slow Conduction Velocity of the Peripheral Nerves in Patients with Type 2 Diabetes Mellitus. J Diabetes Res. (2016) 2016:3653459. doi: 10.1155/2016/3653459
89. Martone G, Frezzotti P, Tosi GM, Traversi C, Mittica V, Malandrini A, et al. An in vivo confocal microscopy analysis of effects of topical antiglaucoma therapy with preservative on corneal innervation and morphology. Am J Ophthalmol. (2009) 147:725–35.e1. doi: 10.1016/j.ajo.2008.10.019
90. Scarpa F, Colonna A, Ruggeri A. Multiple-image deep learning analysis for neuropathy detection in corneal nerve images. Cornea. (2020) 39:342–7. doi: 10.1097/ICO.0000000000002181
91. Kim J, Markoulli M. Automatic analysis of corneal nerves imaged using in vivo confocal microscopy. Clin Exp Optom. (2018) 101:147–61. doi: 10.1111/cxo.12640
92. Scarpa F, Zheng X, Ohashi Y, Ruggeri A. Automatic evaluation of corneal nerve tortuosity in images from in vivo confocal microscopy. Invest Ophthalmol Vis Sci. (2011) 52:6404–8. doi: 10.1167/iovs.11-7529
93. Zhang M, Chen J, Luo L, Xiao Q, Sun M, Liu Z. Altered corneal nerves in aqueous tear deficiency viewed by in vivo confocal microscopy. Cornea. (2005) 24:818–24. doi: 10.1097/01.ico.0000154402.01710.95
94. Erie JC, McLaren JW, Hodge DO, Bourne WM. The effect of age on the corneal subbasal nerve plexus. Cornea. (2005) 24:705–9. doi: 10.1097/01.ico.0000154387.51355.39
95. Patel DV, McGhee CN. In vivo confocal microscopy of human corneal nerves in health, in ocular and systemic disease, and following corneal surgery: a review. Br J Ophthalmol. (2009) 93:853–60. doi: 10.1136/bjo.2008.150615
96. Patel DV, Ku JY, Johnson R, McGhee CN. Laser scanning in vivo confocal microscopy and quantitative aesthesiometry reveal decreased corneal innervation and sensation in keratoconus. Eye (Lond). (2009) 23:586–92. doi: 10.1038/eye.2008.52
97. Patel DV, McGhee CN. Quantitative analysis of in vivo confocal microscopy images: a review. Surv Ophthalmol. (2013) 58:466–75. doi: 10.1016/j.survophthal.2012.12.003
98. Vagenas D, Pritchard N, Edwards K, Shahidi AM, Sampson GP, Russell AW, et al. Optimal image sample size for corneal nerve morphometry. Optom Vis Sci. (2012) 89:812–7. doi: 10.1097/OPX.0b013e31824ee8c9
99. Roszkowska AM, Wylegała A, Gargano R, Spinella R, Inferrera L, Orzechowska-Wylegała B, et al. Impact of corneal parameters, refractive error and age on density and morphology of the subbasal nerve plexus fibers in healthy adults. Sci Rep. (2021) 11:6076. doi: 10.1038/s41598-021-85597-5
100. Guthoff RF, Zhivov A, Stachs O. In vivo confocal microscopy, an inner vision of the cornea - a major review. Clin Exp Ophthalmol. (2009) 37:100–17. doi: 10.1111/j.1442-9071.2009.02016.x
101. Erie EA, McLaren JW, Kittleson KM, Patel SV, Erie JC, Bourne WM. Corneal subbasal nerve density: a comparison of two confocal microscopes. Eye Contact Lens. (2008) 34:322–5. doi: 10.1097/ICL.0b013e31818b74f4
102. Baltrusch S. Confocal microscope examination of the corneal nerve plexus as biomarker for systemic diseases : View from the corneal nerve plexus on diabetes mellitus disease. Ophthalmologe. (2017) 114:592–600. doi: 10.1007/s00347-017-0480-4
Keywords: corneal nerve, confocal microscopy, non-neurological autoimmune diseases, type 1 diabetes, Sjögren's syndrome
Citation: Gu Y, Liu X, Yu X, Qin Q, Yu N, Ke W, Wang K and Chen M (2022) Corneal in vivo Confocal Microscopy for Assessment of Non-Neurological Autoimmune Diseases: A Meta-Analysis. Front. Med. 9:809164. doi: 10.3389/fmed.2022.809164
Received: 04 November 2021; Accepted: 07 February 2022;
Published: 09 March 2022.
Edited by:
Anna Maria Roszkowska, University of Messina, ItalyReviewed by:
Rayaz A. Malik, Weill Cornell Medicine, QatarAdam Wylegala, Śląski Uniwersytet Medyczny, Poland
Copyright © 2022 Gu, Liu, Yu, Qin, Yu, Ke, Wang and Chen. This is an open-access article distributed under the terms of the Creative Commons Attribution License (CC BY). The use, distribution or reproduction in other forums is permitted, provided the original author(s) and the copyright owner(s) are credited and that the original publication in this journal is cited, in accordance with accepted academic practice. No use, distribution or reproduction is permitted which does not comply with these terms.
*Correspondence: Min Chen, chenmineye@zju.edu.cn; Kaijun Wang, ze_wkj@zju.edu.cn
†These authors have contributed equally to this work and share first authorship
‡These authors have contributed equally to this work and share last authorship