- 1Department of Intestine and Hernia Surgery, The First Affiliated Hospital of Kunming Medical University, Kunming, China
- 2NHC Key Laboratory of Drug Addiction Medicine, Kunming Medical University, Kunming, China
- 3Yunnan Institute of Digestive Disease, The First Affiliated Hospital of Kunming Medical University, Kunming, China
Methamphetamine (METH) is an illegal drug widely abused in many countries. Methamphetamine abuse is a major health and social problem all over the world. However, the effects of METH on the digestive system have rarely been reported. Previous studies and clinical cases have shown that METH use can lead to the impaired intestinal barrier function and severe digestive diseases. METH can cause multiple organ dysfunction, especially in the central nervous system (CNS). The gut microbiota are involved in the development of various CNS-related diseases via the gut-brain axis (GBA). Here, we describe the related effects of METH on the intestinal barrier via cytokines and the underlying mechanisms by which METH may occur in the brain-gut axis.
Introduction
Methamphetamine (METH) abuse is a major public health and social problem throughout the world. METH is a widely abused illegal drug and is a highly addictive psychostimulant that can easily cross the blood–brain barrier (BBB) and cause severe brain damage (1), leading to neurological abnormalities and eventually mental disorders (2–4). Some of the symptoms of METH neurotoxicity include oxidative stress, excitatory toxicity, mitochondrial dysfunction, and microglial cell proliferation. METH can also cause acute, subacute, and chronic damage to the nervous system (5), the cardiovascular system, the respiratory system, the digestive system, and the teeth (6). At the same time, the adverse reactions of METH include hypertension, tachycardia, arrhythmia (7), psychosis (4), coronary artery and peripheral vascular spasm (7), insomnia (8), tremor, gastrointestinal disorder (9), and drug addiction.
Methamphetamine has different pharmacokinetic characteristics in different ways of use. Generally, it can enter the body by swallowing, injection, snorting, and smoking. METH is taken orally by the majority of people (10). The upper and lower digestive tracts are the first tissues to contact METH, so the research on the damage caused by METH to the digestive system is increasing day by day. It is one reason for writing this review.
According to previous studies and clinical cases, METH use can contribute to the formation and function of the digestive system. Vasoconstriction of the gastrointestinal tract is the most common physical change. It also includes abdominal pain or stomach pain, severe constipation or diarrhea, or alternating between constipation and diarrhea (9). In some cases, a significant reduction in gastrointestinal blood flow can contribute to serious complications, such as paralytic ileus, which if left untreated can lead to severe infections, gangrene, perforation of the intestinal wall, and water and electrolyte disturbances. In severe cases, intestinal infarction may develop into septic shock with multiple organ failures. Multiple studies have shown that damage to the intestinal mucosa and epithelial barrier resulting in increased intestinal permeability plays an important role in the pathophysiology of anxiety, stress, depression, cognitive decline, chronic fatigue, and eating and sleep disorders, all of which are clinically common among METH users (9). It can also be seen that the intestinal barrier plays an important role in the intestinal injury caused by METH. Recently, studies on METH and the intestinal barrier have been increasing and gaining attention. However, there is still no systematic treatment for intestinal barrier repair. Treatment of the intestinal barrier is currently limited to the disease itself.
Intestinal Barrier
Intestinal Barrier Composition
In this article, the intestinal barrier is defined as a dynamic, permeable physical and immune defense barrier composed of a variety of proteins and intestinal epithelial cells as well as epithelial cell secretions and a large number of immune cells below the intestinal epithelium. On the one hand, the intestinal barrier allows nutrients and certain fluids to be absorbed into the body to some extent; on the other hand, it also prevents toxins and bacteria from entering the body through the intestinal epithelium (11). The stability of the epithelial barrier function is a necessary condition to maintain the stability of the internal mucosal environment. In the subepithelial lamina propria, the balance between pro-inflammatory factors and anti-inflammatory factors plays a certain role in the maintenance of intestinal epithelial barrier function. The inflammatory microenvironment alters the structure and function of junctions between epithelial cells through both direct and indirect mechanisms, affecting epithelial barrier permeability.
Here, we divide the intestinal barrier into three interconnected and interrelated layers (Figure 1). (1) The external mucus layer is symbiotic with the intestinal flora and consists of antimicrobial proteins (AMPs) and secreted immunoglobulin A (sIgA), food antigens, mucinous proteins, digestive enzymes, etc. (2) A central single-celled layer with specialized epithelial cells is a single columnar epithelium that separates the body from the lumen environment. Epithelial cells are made up of several different cell types, such as Paneth cells and goblet cells, and these cells and their different functions form a tight barrier to protect the intestinal environment. The types of these cells and their roles in the intestinal barrier have been described in other reviews (12). (3) Lamina propria: home to innate and adaptive immune cells, such as T cells, B cells, macrophages, and dendritic cells. This layer is the body’s largest reservoir of immune cells and is responsible for clearing bacteria and viruses as well as dead cells, secreting inflammatory cytokines, and maintaining intestinal homeostasis.
Intestinal epithelial cells are connected by intercellular molecular connection complexes, such as tight junctions, adhesion junctions, desmosomes, and gap junctions. Molecular linkage complexes between intestinal epithelial cells play an important role in the entry of certain substances into the lamina propria or portal vein circulation.
In other reviews, the effects of various factors [such as dietary factors, alcohol, medication (NSAIDs and PPIs), smoking, and stress] on the intestinal barrier function (such as increased intestinal barrier permeability) (13), as well as methods and experiments for detecting intestinal barrier function are described in detail (13, 14).
Tight Junction
The tight junction is a multi-protein composite [e.g., Occludin, Tricellulin, MarvelD3, Claudin protein family (15), JAM, ZO-1, ZO-2, ZO-3, cingulin, and symplekin (16, 17)] that connects the top end between epithelial cells and plays an important role in the intestinal barrier by allowing certain solutes and molecules to be transported but blocking the proteins, lipids, and microbial peptides (18, 19), strictly limiting the transportation of various harmful molecules (20). Therefore, any change in the tight junction structure may be harmful to the body. Disruption and/or degradation of TJ contribute to increased intestinal barrier permeability. Disorder of TJ protein expression/positioning and reduced intestinal barrier permeability lead to intestinal leakages and pathological state of the systemic diseases, such as inflammatory bowel disease (IBD), celiac disease, gastrointestinal tract infection, and chronic liver disease, autoimmune arthritis, multiple sclerosis, type 1 diabetes, and Parkinson’s disease. Therefore, barrier defects were significantly associated with abnormal systemic immune responses.
Meth and Genomics of Gut
Transcriptome studies have been conducted to investigate changes in gene expression in intestinal tissues caused by METH. A total of 326 differentially expressed genes (DEGs) were identified in the transcriptome study of the meth-induced IBD mouse model (Table 1). Among them, 120 genes were upregulated and 206 genes were downregulated, suggesting that METH significantly affected the intestinal transcriptome of mice (21). DEGs obtained from the METH-induced IBD mouse model and bioinformatics analysis showed that METH may cause a specific type of IBD. These results provide new insights into the relationship between METH abuse and IBD. A new molecule or pathway may play a role when METH causes IBD, but whether it has practical significance for the clinical treatment of such patients and the treatment of IBD remains to be further studied.
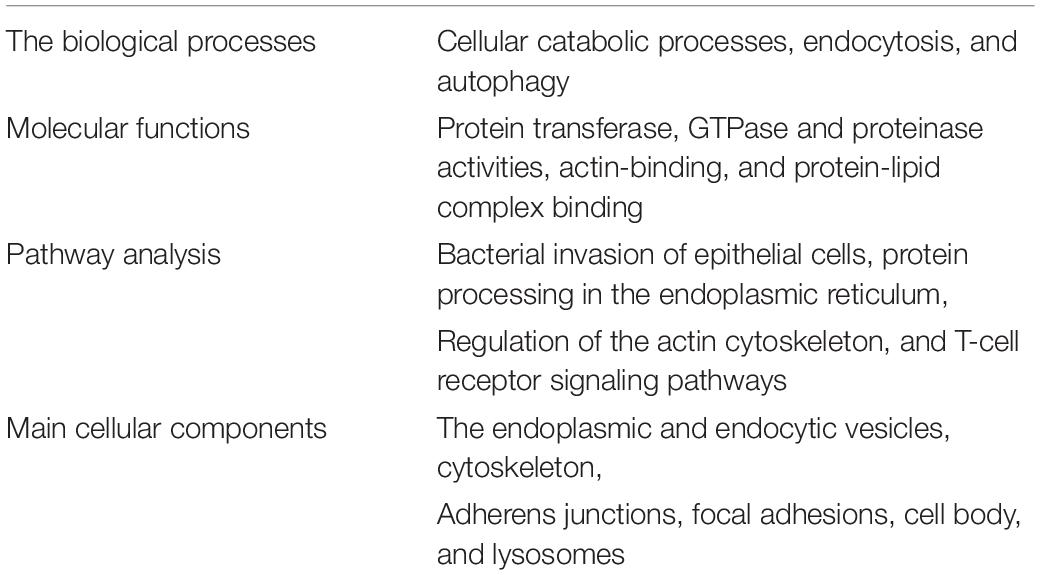
Table 1. Bioinformatics analysis of differentially expressed genes in methamphetamine-induced inflammatory bowel disease mouse models.
Meth Contributes to Intestinal Barrier Damage Through Cytokines
Cytokine-mediated alterations in paracellular permeability in a variety of clinical and pathological conditions such as IBD, airway inflammation (22, 23), cystic fibrosis in asthma, and blood-brain barrier disorders (24). TNFα, IFN-γ, and interleukin play important roles in regulating tight junction integrity (25). Cytokine-mediated signaling interferes with tight junctions and increases intestinal barrier permeability, increasing tissue exposure to antigens in the gastrointestinal tract. When intestinal barrier function is disrupted, endotoxin (lipopolysaccharide LPS) is more likely to enter the circulatory system. In in vitro microglial cell studies, METH reduced the distribution and expression of toll-like receptor 4 (TLR4) and the production of pro-inflammatory factors after LPS action (26). This is different from the effects of alone METH on the TLR4 of microglial cell. In macrophages in vitro experiments [the phenotype or abundance of macrophages in the gut wall contribute to the development of the intestinal epithelium and the ability to sample gut antigens (27)], METH and LPS can significantly increase TNF-α, IL-1β, and IL-8 levels (signal transduction paths in NF-κ B, MAPK, and PI3-Akt are involved in mediated increased inflammatory responses) (28). The increase in cytokines or chemokines is usually greater than the increase in LPS treatment alone.
Long-term methamphetamine use can cause changes in cytokine levels. Previous studies have reported that (29) serum levels of TNF-α, IL-6, and IL-18 were measured in 78 hospitalized long-term METH users. The TNF-α, IL-6, and IL-18 levels increased significantly (Figure 2A). Persistent inflammation in abusers further induces nerve damage, it is usually characterized by glial proliferation and increased cytokine levels (30). METH leads to BBB dysfunction directly or indirectly through the release of TNF-α and subsequent activation of NF-κB pathway (31). TNF-α not only disrupted the tight junctions between cells and led to an increase in intestinal barrier permeability, but also induced apoptosis of epithelial cells. Apoptosis accounts for approximately half (56%) of the TNF-α-induced increase in permeability, while the other half is caused by the degradation of the tight junctions (32). Many researchers believe that TNF-α is an important inflammatory mechanism leading to Crohn’s disease and other IBDs.
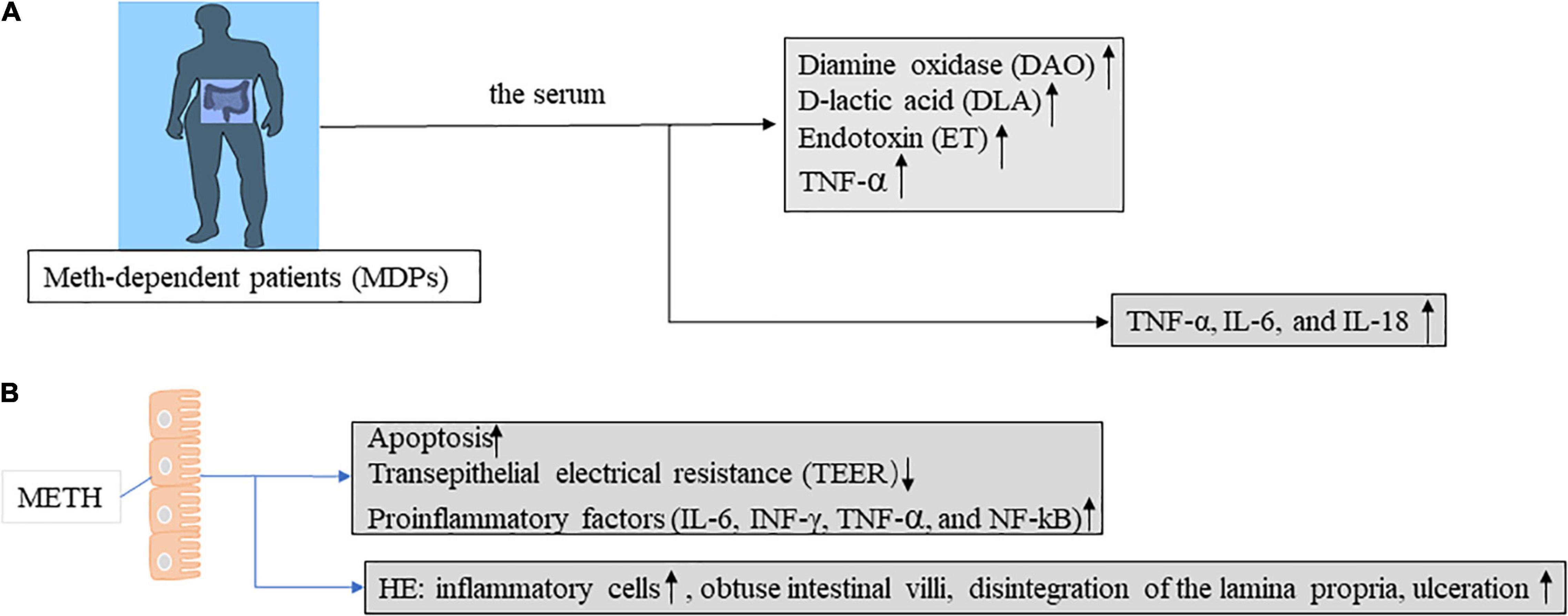
Figure 2. Intestinal barrier function was evaluated in mice and humans in the context of METH treatment. (A) Intestinal mucosal injury, intestinal wall permeability, and bacterial translocation are assessed by determining the serum levels of diamine oxidase (DAO), D-lactic acid (DLA), and endotoxin (ET) in Meth-dependent patients (MDPs). Abnormal levels of all these indicators reflect intestinal barrier dysfunction. (B) Intestinal epithelial cells of methamphetamine-treated mice are evaluated for intestinal barrier function by apoptosis, TEER, proinflammatory factors, and HE staining. Abnormal levels of all these indicators reflect intestinal barrier dysfunction.
In animal models of METH, METH can cause significant intestinal barrier damage, increase pro-inflammatory factors (such as, IL-6, INF-γ, TNF-α, and NF-κB) (33), and induce intestinal tissue injury in mice (Figure 2B). The IFN-γ signaling pathway in intestinal glial cells is critical for intestinal homeostasis, and the IFNγ -EGC-CXCL10 axis plays a key role in immune response and tissue repair after infection. METH treatment increases IL-8 expression in vitro through the nuclear factor-κB pathway. Meanwhile, IL-8 activation of CXCR1 (C-X-C motif chemokine receptor 1) induces an increase in METH-related neuronal apoptosis in vivo and in vitro (34). Increased IL-8 was also observed in mouse intestinal epithelial cells cultured in vitro (33). In addition, cytokines (IFN-γ, IL-1β, and IL-8) in epithelial cells were increased in the METH in vitro model. In mouse models (Figure 3A), METH-induced spatial learning was impaired, and this effect was associated with reduced hippocampal IL-1β levels. Studies have shown that the loss of METH-related cognitive decline is linked to IL-1 (35). It provides a potential new therapeutic approach for the treatment of cognitive changes in individuals with METH abuse.
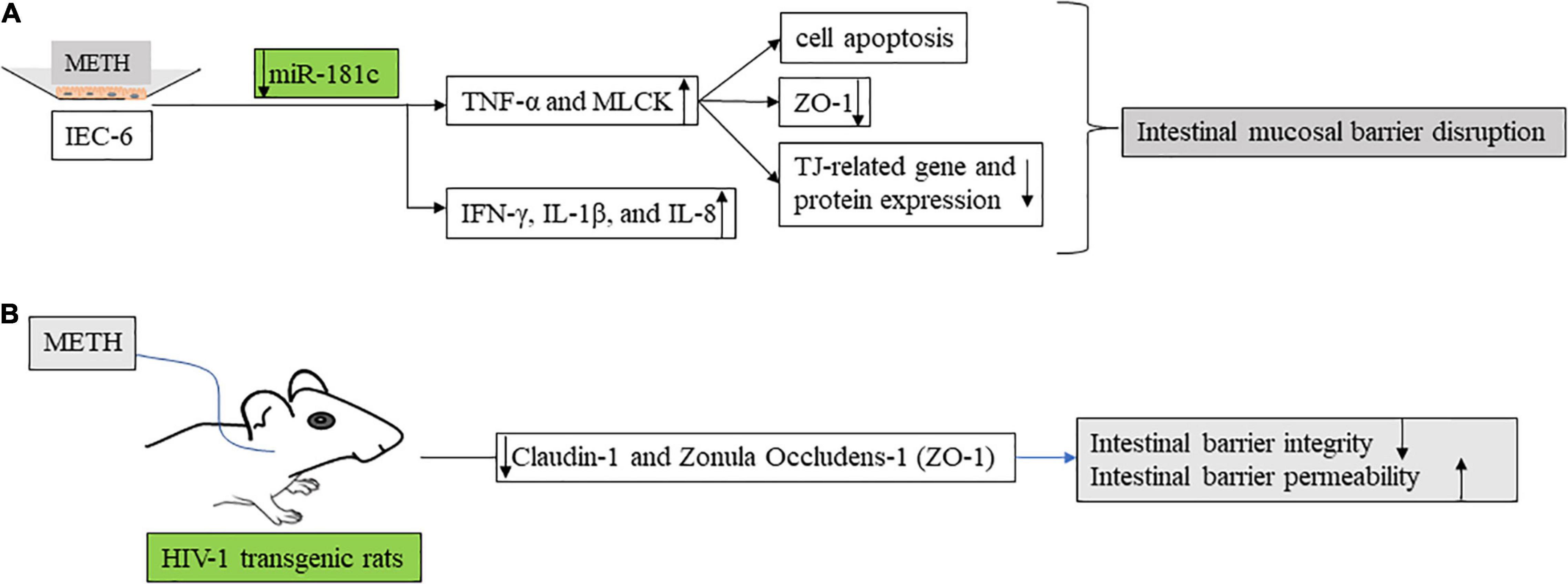
Figure 3. The effects of methamphetamine on intestinal barrier function. (A) In in vitro model, methamphetamine regulates Mir-181c. Anti-mir-181c promotes the secretion of TNF-α and MLCK, resulting in increased intestinal barrier permeability (decreased tight junction related protein) and epithelial cell apoptosis. IEC-6 is a well-established rat cell line with characteristics similar to those of intestinal epithelial cells and is extensively used as a surrogate for intestinal epithelial cells, for in vitro studies. MLCK, myosin light chain kinase. (B) Self-administered methamphetamine resulted in decreased colonic barrier function [Claudin-1 and Zonula occludens-1 (ZO-1)] and impaired colonic integrity in HIV-1 transgenic rats.
MicroRNAs have been shown to regulate epithelial barrier homeostasis by mediating the production of TNF-α and to participate in a variety of biological processes, such as cell proliferation, apoptosis, invasion, and metastasis, and stem cell differentiation, forming complex networks with important functions (36). In in vivo models, human diamine oxidase (DAO), D-lactic acid (DLA), and endotoxin (ET) levels are used to assess intestinal mechanical barrier function (Figure 2A). In an in vitro model, METH inhibits Mir-181c (miRNAs that regulate TNF-α) and then promotes TNF-α (TNF-α activates the MLCK promoter through the NF-κB pathway, and then promotes the increase of MLCK transcription, expression, and activity (37), and promotes the downregulation of ZO-1 protein expression and changes in junction location) (38) secretion, thereby increasing intestinal barrier permeability and epithelial cell apoptosis (33) (Figure 3A). This provides a new direction for the treatment of intestinal damage caused by METH, and microRNA regulation has a greater application prospect in future therapeutic strategies to prevent intestinal barrier dysfunction compared with available targeted protein drugs.
Previous studies in a model of chronic METH abuse in rhesus monkeys have shown that METH can cause damage to the intestinal mucosal barrier, resulting in a high risk of intestinal infection. In addition, METH enhanced the expression of chemokine and chemokine receptor-related genes and promoted the apoptosis of tissue cells and the pro-inflammatory pathway (39). Recent studies have shown that METH promotes the overexpression of NLRP3 inflammasome and induces intestinal inflammatory injury (40) (Figure 4), NLRP3 is widely expressed in the gastrointestinal tract and can be found in epithelial cells at mucosal sites, which are important for maintaining environmental balance in the gut (41). Blocking NLRP3 inflammasome activation partially prevents inflammatory damage.

Figure 4. Methamphetamine (METH) promotes the overexpression of NLRP3 inflammasome and induces intestinal inflammatory injury.
When it comes to METH abuse, it is necessary to mention the special group of people infected with HIV. Amanda L. Persons reported (42) reduced colonic barrier function and impaired colonic integrity in HIV-1 transgenic rats as a result of self-medicated METH (Figure 3B). METH abuse is a common situation among HIV-infected people. HIV infection itself causes certain damage to the intestine. In the self-drug model of HIV transgenic rats, the intestinal barrier permeability and endotoxin level of the colon of rats increased after METH addiction (42). At the same time, METH use and sexual behavior contribute to chronic HIV-1 infection and intestinal disorders in young men who have sex with men (43, 44). METH abuse in men infected with HIV is associated with a microbiological imbalance of pro-inflammatory bacteria, including some with neuroactive bacteria and bacteria thought to be associated with adverse HIV outcomes (44).
Numerous studies have shown that multiple pro-inflammatory factors increase in METH users, suggesting that METH-aggravated inflammation may be a common feature of infectious disease in METH abusers. Inflammatory factors have also been shown to disrupt intestinal barrier function in a variety of ways (tight junctions or apoptosis of intestinal epithelial cells). Anti-inflammatory factor drugs may play a role in protecting the intestinal barrier. The contribution of METH to inflammatory factors is not only limited to intestinal barrier injury but also affects diseases related to intestinal barrier function, although it has not been further confirmed. However, understanding the molecular and barrier function roles of disrupting and stabilizing intestinal epithelial TJs and understanding the development of inflammation may help to treat patients with intestinal barrier disorders associated with inflammation, or to prevent the development of inflammation. Therefore, bowel barrier therapy has good prospects in terms of improving a variety of diseases and cytokine regulation.
The Effects of Meth on the Gut-Brain Axis
Methamphetamine can enhance the action of monoamines because METH has a similar chemical structure to monoamines and can directly bind to and activate the receptors of these compounds (45). Different doses of METH promote the release or enhancement of monoamines in different ways. In addition, METH attenuated monoamine metabolism. There is evidence that METH blocks the reuptake of catecholamines leading to an increase in the effects of norepinephrine. Most of the peripheral effects of norepinephrine released by METH are caused by the rapid and sustained release of catecholamines and stimulation of α and β adrenergic receptors (α 1 receptors cause peripheral vascular contraction, leading to hypertension and visceral ischemia). METH is known to have a sympathetic-like effect, leading to constriction of blood vessels in the visceral circulation. The rapid and sustained release of norepinephrine after METH use causes arterial constriction. Effects are also seen in the intestinal mesenteric blood vessels, leading to acute intestinal ischemia.
The most common harmful effects of METH are seen in neurological and circulatory damage or disease. However, one case of paralytic ileus caused by METH was diagnosed (46). The mechanism may be that METH promotes the release of norepinephrine and dopamine, and the activation of the dopamine-1 receptor leads to a significant decrease in intestinal contractility and motor capacity. In addition, norepinephrine alters the intestinal nervous system, leading to a decrease in intestinal muscle tone. Intestinal obstruction is associated with intestinal and vascular barriers (47), oxidative stress, and nitrifying stress. METH-mediated neurotransmitter release also promotes the production of oxidative stress molecules [reactive nitrogen species (ROS) and reactive nitrogen species (RNS)], which can lead to the injury and death of intestinal neurons and intestinal barrier dysfunction. Intestinal mucosal barrier dysfunction increases intestinal permeability and plays an important role in the pathophysiology of anxiety (48), stress (49), depression (50), cognitive decline (51), and eating and sleep disorders (52) (Figure 5).
Disruption of the intestinal barrier (damage of intestinal epithelial cells and disruption of tight junctions) results in the leakage of inflammatory factors, gut microbiota, and gut microbiota products from the lumen into the circulatory and lymphatic systems (53). Gut microbes, as well as their metabolites, are produced in the vicinity of intestinal epithelial cells and therefore have a significant impact on intestinal barrier function and immune response (12). The human gastrointestinal tract contains countless types of microbes, and the relationship between the microbes and the host is very close. At the same time, METH use increases the permeability of the BBB (31), and these intestinal microbial components have the ability to enter the brain (3). Gut bacteria collaborate with their animal hosts to regulate immune, metabolic, and nervous system development and function through dynamic two-way communication along the “gut-brain axis (54).” Besides, the intestinal flora may play an important role in the communication between the gut and the brain, for example, the gut microbiome plays an important role in anxiety and depression (55, 56). In animal models of phenethylamine-induced hyperthermia (PIH), certain changes in gut bacteria promote the bidirectional regulation between the brain and gut (57) (PIH). In METH treated mouse models, METH increased the relative abundance of pathogenic bacteria (58), promoted intestinal inflammation, and reduced intestinal TJ protein expression, but decreased the relative abundance of probiotics and changed fecal metabolites. METH exposure enhances the intestinal autophagy flora, promotes the accumulation of fecal metabolites in the autophagy pathway, and induces autophagy in the central nervous system (59).
Methamphetamine abusers have increased intestinal inflammatory biomarkers, including inflammatory cytokines (already mentioned above), which have been shown to enter the brain and interact with areas of pathophysiology related to depression (60), such as neurotransmitter metabolism, neuroendocrine function, and neuroplasticity.
Summary and Future Directions
There has been little progress in the treatment of common gastrointestinal symptoms, such as gastrointestinal pain, diarrhea, and bloating. As a result, there is a growing interest in gut barrier research. The role of intestinal barrier function is believed to be important, but there are still many unresolved questions because there is no clear gold standard for barrier function testing and the clinical significance and relevance of the existing multiple methods for measuring intestinal barrier function is unclear.
The effect of METH on the intestinal barrier does exist (the exact mechanism is still unclear). METH use has been shown to cause changes in intestinal barrier function and permeability (such as intestinal epithelial cell apoptosis and intercellular TJ damage), as well as cause some abdominal complications. The digestive system can be thought of as the body’s second “brain.” A number of cumulative observations suggest that a number of neurological disorders, such as METH-related changes, may be associated with increased intestinal barrier permeability, and that these relatively minor disturbances can be reversed by counteracting the associated inflammatory pathways and protecting the intestinal barrier. It is important to study the characteristics of the intestinal barrier and the pathways between intestinal epithelial cells to solve the problems related to the brain-gut axis, which provides a basis for the detection of parenteral diseases. In addition, part of the gut-brain axis includes the effects of gut flora on the brain, behavior, and health. For example, taking probiotics can treat certain aspects of depressive and anxious behavior (61–65). Some probiotics may improve intestinal barrier dysfunction, but it is unclear whether intestinal barrier therapy is associated with anxiety and depression. Thus, gut microbiota and/or metabolites may affect the health and neurological function of the host. Moreover, gastrointestinal dysfunction is more common in the early stages of Parkinson’s disease than other systemic symptoms. In Hye-young Sung’s questionnaire, 88.9% of 54 selected patients reported intestinal symptoms before the early motor symptoms of Parkinson’s disease (66). This suggests that intestinal alterations may help in the early diagnosis of neurological diseases.
Increased intestinal epithelial permeability is due to increased paracellular transport, apoptosis, or transcellular permeability. Any inflammatory process can compromise barrier integrity; for example, anti-TNF-α therapy reduces mucosal inflammation and restores intestinal permeability in patients with IBD. Cells at the gut barrier are exposed to microbial and METH factors that may promote the production of inflammatory factors either by directly damaging cells or by overactivating inflammatory processes. Cytokines and chemokines are neuroimmune factors expressed in neurons, astrocytes, and microglia, which show promising application prospects in clinical neuroinflammation, neuronal injury, and behavioral disorders. Inflammatory factors may serve as potential therapeutic targets for METH use disorders in the future.
Impaired intestinal barrier function is associated with many intestinal and systemic disease states. Unfortunately, most current clinical data are correlated, making it difficult to distinguish cause from effect when interpreting the significance of barrier loss. The effect of restoring barrier function on improving clinical manifestations of local gastrointestinal disease or systemic disease has also not been demonstrated. There is currently no treatment that targets the epithelial barrier. To achieve this goal, the regulatory mechanisms of barriers should be better understood. Clinicians should be aware of the possibility of barrier dysfunction in gastrointestinal diseases and as a target for future treatment.
Author Contributions
YL, KB, HL, and DK conceived and designed the manuscript and prepared the manuscript. YL, HL, DK, and KB performed the literature search.YL and DK did the picture making. KB, YL, DK, and HL revised the manuscript. All authors read and approve the final version of the manuscript.
Funding
This work was supported by the Science and Technology Department of Yunnan Province (202001AS070004), Yunnan Province Intelligent Talent Platform (RLMY20200019), National Natural Science Foundation of China (82060525), and Yunnan Provincial Health and Health Commission (202005AF150090).
Conflict of Interest
The authors declare that the research was conducted in the absence of any commercial or financial relationships that could be construed as a potential conflict of interest.
Publisher’s Note
All claims expressed in this article are solely those of the authors and do not necessarily represent those of their affiliated organizations, or those of the publisher, the editors and the reviewers. Any product that may be evaluated in this article, or claim that may be made by its manufacturer, is not guaranteed or endorsed by the publisher.
References
1. Davidson C, Gow AJ, Lee TH, Ellinwood EH. Methamphetamine neurotoxicity: necrotic and apoptotic mechanisms and relevance to human abuse and treatment. Brain Res Brain Res Rev. (2001) 36:1–22. doi: 10.1016/s0165-0173(01)00054-6
2. Mizoguchi H, Yamada K. Methamphetamine use causes cognitive impairment and altered decision-making. Neurochem Int. (2019) 124:106–13. doi: 10.1016/j.neuint.2018.12.019
3. Northrop NA, Yamamoto BK. Methamphetamine effects on blood-brain barrier structure and function. Front Neurosci. (2015) 9:69. doi: 10.3389/fnins.2015.00069
4. Shin EJ, Dang DK, Tran TV, Tran HQ, Jeong JH, Nah SY, et al. Current understanding of methamphetamine-associated dopaminergic neurodegeneration and psychotoxic behaviors. Arch Pharmacal Res. (2017) 40:403–28. doi: 10.1007/s12272-017-0897-y
5. Frost DO, Cadet JL. Effects of methamphetamine-induced neurotoxicity on the development of neural circuitry: a hypothesis. Brain Res Brain Res Rev. (2000) 34:103–18. doi: 10.1016/s0165-0173(00)00042-4
6. Lineberry TW, Bostwick JM. Methamphetamine abuse: a perfect storm of complications. Mayo Clin Proc. (2006) 81:77–84. doi: 10.4065/81.1.77
7. Kevil CG, Goeders NE, Woolard MD, Bhuiyan MS, Dominic P, Kolluru GK, et al. Methamphetamine use and cardiovascular disease. Arterioscler Thromb Vasc Biol. (2019) 39:1739–46.
8. Vrajová M, Šlamberová R, Hoschl C, Ovsepian SV. Methamphetamine and sleep impairments: neurobehavioral correlates and molecular mechanisms. Sleep. (2021) 44:zsab001. doi: 10.1093/sleep/zsab001
9. Prakash MD, Tangalakis K, Antonipillai J, Stojanovska L, Nurgali K, Apostolopoulos V. Methamphetamine: effects on the brain, gut and immune system. Pharmacol Res. (2017) 120:60–7. doi: 10.1016/j.phrs.2017.03.009
10. Chomchai C, Chomchai S. Global patterns of methamphetamine use. Curr Opin Psychiatry. (2015) 28:269–74. doi: 10.1097/YCO.0000000000000168
11. Furness JB, Kunze WA, Clerc N. Nutrient tasting and signaling mechanisms in the gut. II. The intestine as a sensory organ: neural, endocrine, and immune responses. Am J Physiol. (1999) 277:G922–8. doi: 10.1152/ajpgi.1999.277.5.G922
12. Ghosh S, Whitley CS, Haribabu B, Jala VR. Regulation of intestinal barrier function by microbial metabolites. Cell Mol Gastroenterol Hepatol. (2021) 11:1463–82. doi: 10.1016/j.jcmgh.2021.02.007
13. Vancamelbeke M, Vermeire S. The intestinal barrier: a fundamental role in health and disease. Exp Rev Gastroenterol Hepatol. (2017) 11:821–34. doi: 10.1080/17474124.2017.1343143
14. Schoultz I, Keita ÅV. The intestinal barrier and current techniques for the assessment of gut permeability. Cells. (2020) 9:1909. doi: 10.3390/cells9081909
15. Spalinger MR, Sayoc-Becerra A, Santos AN, Shawki A, Canale V, Krishnan M, et al. PTPN2 regulates interactions between macrophages and intestinal epithelial cells to promote intestinal barrier function. Gastroenterology. (2020) 159:1763–77.e14. doi: 10.1053/j.gastro.2020.07.004
16. Günzel D, Yu AS. Claudins and the modulation of tight junction permeability. Physiol Rev. (2013) 93:525–69. doi: 10.1152/physrev.00019.2012
17. Mitic LL, Van Itallie CM, Anderson JM. Molecular physiology and pathophysiology of tight junctions I. Tight junction structure and function: lessons from mutant animals and proteins. Am J Physiol Gastrointest Liver Physiol. (2000) 279:G250–4. doi: 10.1152/ajpgi.2000.279.2.G250
18. Diamond JM. Twenty-first Bowditch lecture. The epithelial junction: bridge, gate, and fence. Physiologist. (1977) 20:10–8.
19. Berkes J, Viswanathan VK, Savkovic SD, Hecht G. Intestinal epithelial responses to enteric pathogens: effects on the tight junction barrier, ion transport, and inflammation. Gut. (2003) 52:439–51. doi: 10.1136/gut.52.3.439
20. Chelakkot C, Ghim J, Ryu SH. Mechanisms regulating intestinal barrier integrity and its pathological implications. Exp Mol Med. (2018) 50:1–9. doi: 10.1038/s12276-018-0126-x
21. Sun J, Chen F, Chen C, Zhang Z, Zhang Z, Tian W, et al. Intestinal mRNA expression profile and bioinformatics analysis in a methamphetamine-induced mouse model of inflammatory bowel disease. Ann Transl Med. (2020) 8:1669. doi: 10.21037/atm-20-7741
22. Holgate ST. Epithelium dysfunction in asthma. J Allergy Clin Immun. (2007) 120:1233–44;quiz1245–6.
23. Coyne CB, Vanhook MK, Gambling TM, Carson JL, Boucher RC, Johnson LG. Regulation of airway tight junctions by proinflammatory cytokines. Mol Biol Cell. (2002) 13:3218–34. doi: 10.1091/mbc.e02-03-0134
24. Persidsky Y, Ramirez SH, Haorah J, Kanmogne GD. Blood-brain barrier: structural components and function under physiologic and pathologic conditions. J Neuroimmune Pharmacol. (2006) 1:223–36. doi: 10.1007/s11481-006-9025-3
25. Capaldo CT, Nusrat A. Cytokine regulation of tight junctions. Biochim Biophys Acta (BBA) Biomembr. (2009) 1788:864–71. doi: 10.1016/j.bbamem.2008.08.027
26. Vargas AM, Rivera-Rodriguez DE, Martinez LR. Methamphetamine alters the TLR4 signaling pathway, NF-κB activation, and pro-inflammatory cytokine production in LPS-challenged NR-9460 microglia-like cells. Mol Immunol. (2020) 121:159–66. doi: 10.1016/j.molimm.2020.03.013
27. Sehgal A, Donaldson DS, Pridans C, Sauter KA, Hume DA, Mabbott NA. The role of CSF1R-dependent macrophages in control of the intestinal stem-cell niche. Nat Commun. (2018) 9:1272. doi: 10.1038/s41467-018-03638-6
28. Liu X, Silverstein PS, Singh V, Shah A, Qureshi N, Kumar A. Methamphetamine increases LPS-mediated expression of IL-8, TNF-α and IL-1β in human macrophages through common signaling pathways. PloS One. (2012) 7:e33822. doi: 10.1371/journal.pone.0033822
29. Luo Y, He H, Ou Y, Zhou Y, Fan N. Elevated serum levels of TNF-α, IL-6, and IL-18 in chronic methamphetamine users. Human Psychopharmacol. (2021) 37:e2810. doi: 10.1002/hup.2810
30. Coelho-Santos V, Gonçalves J, Fontes-Ribeiro C, Silva AP. Prevention of methamphetamine-induced microglial cell death by TNF-α and IL-6 through activation of the JAK-STAT pathway. J Neuroinflamm. (2012) 9:103. doi: 10.1186/1742-2094-9-103
31. Coelho-Santos V, Leitão RA, Cardoso FL, Palmela I, Rito M, Barbosa M, et al. The TNF-α/NF-κB signaling pathway has a key role in methamphetamine-induced blood-brain barrier dysfunction. J Cereb Blood Flow Metab. (2015) 35:1260–71. doi: 10.1038/jcbfm.2015.59
32. Gitter AH, Bendfeldt K, Schulzke JD, Fromm M. Leaks in the epithelial barrier caused by spontaneous and TNF-alpha-induced single-cell apoptosis. FASEB J. (2000) 14:1749–53. doi: 10.1096/fj.99-0898com
33. Shen S, Zhao J, Dai Y, Chen F, Zhang Z, Yu J, et al. Methamphetamine-induced alterations in intestinal mucosal barrier function occur via the microRNA-181c/TNF-α/tight junction axis. Toxicol Lett. (2020) 321:73–82. doi: 10.1016/j.toxlet.2019.12.020
34. Du SH, Zhang W, Yue X, Luo XQ, Tan XH, Liu C, et al. Role of CXCR1 and Interleukin-8 in Methamphetamine-Induced Neuronal Apoptosis. Front Cell Neurosci. (2018) 12:230. doi: 10.3389/fncel.2018.00230
35. Liśkiewicz A, Przybyła M, Park M, Liśkiewicz D, Nowacka-Chmielewska M, Małecki A, et al. Methamphetamine-associated cognitive decline is attenuated by neutralizing IL-1 signaling. Brain Behav Immun. (2019) 80:247–54. doi: 10.1016/j.bbi.2019.03.016
36. Zou L, Xiong X, Wang K, Yin Y. MicroRNAs in the intestine: role in renewal, homeostasis, and inflammation. Curr Mol Med. (2018) 18:190–8. doi: 10.2174/1566524018666180907163638
37. Ye D, Ma I, Ma TY. Molecular mechanism of tumor necrosis factor-alpha modulation of intestinal epithelial tight junction barrier. Am J Physiol Gastrointest Liver Physiol. (2006) 290:G496–504. doi: 10.1152/ajpgi.00318.2005
38. Ma TY, Iwamoto GK, Hoa NT, Akotia V, Pedram A, Boivin MA, et al. TNF-alpha-induced increase in intestinal epithelial tight junction permeability requires NF-kappa B activation. Am J Physiol Gastrointest Liver Physiol. (2004) 286:G367–76. doi: 10.1152/ajpgi.00173.2003
39. Najera JA, Bustamante EA, Bortell N, Morsey B, Fox HS, Ravasi T, et al. Methamphetamine abuse affects gene expression in brain-derived microglia of SIV-infected macaques to enhance inflammation and promote virus targets. BMC Immunol. (2016) 17:7. doi: 10.1186/s12865-016-0145-0
40. Zhao J, Shen S, Dai Y, Chen F, Wang K. Methamphetamine induces intestinal inflammatory injury via nod-like receptor 3 protein (NLRP3) inflammasome overexpression in vitro and in vivo. Med Sci Monitor. (2019) 25:8515–26. doi: 10.12659/MSM.920190
41. Kummer JA, Broekhuizen R, Everett H, Agostini L, Kuijk L, Martinon F, et al. Inflammasome components NALP 1 and 3 show distinct but separate expression profiles in human tissues suggesting a site-specific role in the inflammatory response. J Histochem Cytochem. (2007) 55:443–52. doi: 10.1369/jhc.6A7101.2006
42. Persons AL, Bradaric BD, Dodiya HB, Ohene-Nyako M, Forsyth CB, Keshavarzian A, et al. Colon dysregulation in methamphetamine self-administering HIV-1 transgenic rats. PloS One (2018) 13:e0190078. doi: 10.1371/journal.pone.0190078
43. Fulcher JA, Hussain SK, Cook R, Li F, Tobin NH, Ragsdale A, et al. Effects of substance use and sex practices on the intestinal microbiome during HIV-1 infection. J Infect Dis. (2018) 218:1560–70. doi: 10.1093/infdis/jiy349
44. Cook RR, Fulcher JA, Tobin NH, Li F, Lee DJ, Woodward C, et al. Alterations to the gastrointestinal microbiome associated with methamphetamine use among young men who have sex with men. Sci Rep. (2019) 9:14840. doi: 10.1038/s41598-019-51142-8
45. Cruickshank CC, Dyer KR. A review of the clinical pharmacology of methamphetamine. Addiction (Abingdon, England). (2009) 104:1085–99. doi: 10.1111/j.1360-0443.2009.02564.x
46. Carlson TL, Plackett TP, Gagliano RA Jr., Smith RR. Methamphetamine-induced paralytic ileus. Hawaii J Med Pub Health. (2012) 71:44–5.
47. Boschetti E, Accarino A, Malagelada C, Malagelada JR, Cogliandro RF, Gori A, et al. Gut epithelial and vascular barrier abnormalities in patients with chronic intestinal pseudo-obstruction. Neurogastroenterol Motil. (2019) 31:e13652. doi: 10.1111/nmo.13652
48. Stevens BR, Goel R, Seungbum K, Richards EM, Holbert RC, Pepine CJ, et al. Increased human intestinal barrier permeability plasma biomarkers zonulin and FABP2 correlated with plasma LPS and altered gut microbiome in anxiety or depression. Gut. (2018) 67:1555–7. doi: 10.1136/gutjnl-2017-314759
49. Camilleri M. Leaky gut: mechanisms, measurement and clinical implications in humans. Gut. (2019) 68:1516–26. doi: 10.1136/gutjnl-2019-318427
50. Trzeciak P, Herbet M. Role of the intestinal microbiome, intestinal barrier and psychobiotics in depression. Nutrients. (2021) 13:927. doi: 10.3390/nu13030927
51. Wang Y, An Y, Ma W, Yu H, Lu Y, Zhang X, et al. 27-Hydroxycholesterol contributes to cognitive deficits in APP/PS1 transgenic mice through microbiota dysbiosis and intestinal barrier dysfunction. J Neuroinflamm. (2020) 17:199. doi: 10.1186/s12974-020-01873-7
52. Gao T, Wang Z, Dong Y, Cao J, Lin R, Wang X, et al. Role of melatonin in sleep deprivation-induced intestinal barrier dysfunction in mice. J Pineal Res. (2019) 67:e12574. doi: 10.1111/jpi.12574
53. Suzuki T. Regulation of intestinal epithelial permeability by tight junctions. Cell Mol Life Sci. (2013) 70:631–59. doi: 10.1007/s00018-012-1070-x
54. Morais LH, Schreiber H. L. t, Mazmanian SK. The gut microbiota-brain axis in behaviour and brain disorders. Nat Rev Microbiol. (2021) 19:241–55. doi: 10.1038/s41579-020-00460-0
55. Lach G, Schellekens H, Dinan TG, Cryan JF. Anxiety, depression, and the microbiome: a role for gut peptides. Neurother. (2018) 15:36–59. doi: 10.1007/s13311-017-0585-0
56. Forouzan S, Hoffman KL, Kosten TA. Methamphetamine exposure and its cessation alter gut microbiota and induce depressive-like behavioral effects on rats. Psychopharmacol. (2021) 238:281–92. doi: 10.1007/s00213-020-05681-y
57. Aburahma A, Pachhain S, Choudhury SR, Rana S, Phuntumart V, Larsen R, et al. Potential contribution of the intestinal microbiome to phenethylamine-induced hyperthermia. Brain Behav Evol. (2020) 95:256–71. doi: 10.1159/000512098
58. Ning T, Gong X, Xie L, Ma B. Gut microbiota analysis in rats with methamphetamine-induced conditioned place preference. Front Microbiol. (2017) 8:1620. doi: 10.3389/fmicb.2017.01620
59. Chen LJ, Zhi X, Zhang KK, Wang LB, Li JH, Liu JL, et al. Escalating dose-multiple binge methamphetamine treatment elicits neurotoxicity, altering gut microbiota and fecal metabolites in mice. Food Chem Toxicol. (2021) 148:111946. doi: 10.1016/j.fct.2020.111946
60. Miller AH, Maletic V, Raison CL. Inflammation and its discontents: the role of cytokines in the pathophysiology of major depression. Biol Psychiatry. (2009) 65:732–41. doi: 10.1016/j.biopsych.2008.11.029
61. Desbonnet L, Garrett L, Clarke G, Kiely B, Cryan JF, Dinan TG. Effects of the probiotic Bifidobacterium infantis in the maternal separation model of depression. Neuroscience. (2010) 170:1179–88. doi: 10.1016/j.neuroscience.2010.08.005
62. Bravo JA, Forsythe P, Chew MV, Escaravage E, Savignac HM, Dinan TG, et al. Ingestion of Lactobacillus strain regulates emotional behavior and central GABA receptor expression in a mouse via the vagus nerve. Proc Natl Acad Sci USA. (2011) 108:16050–5. doi: 10.1073/pnas.1102999108
63. Arseneault-Bréard J, Rondeau I, Gilbert K, Girard SA, Tompkins TA, Godbout R, et al. Combination of Lactobacillus helveticus R0052 and Bifidobacterium longum R0175 reduces post-myocardial infarction depression symptoms and restores intestinal permeability in a rat model. Br J Nutr. (2012) 107:1793–9.
64. Yarandi SS, Peterson DA, Treisman GJ, Moran TH, Pasricha PJ. Modulatory effects of gut microbiota on the central nervous system: how gut could play a role in neuropsychiatric health and diseases. J Neurogastroenterol Motil. (2016) 22:201–12. doi: 10.5056/jnm15146
65. Kelly JR, Clarke G, Cryan JF, Dinan TG. Brain-gut-microbiota axis: challenges for translation in psychiatry. Ann Epidemiol. (2016) 26:366–72. doi: 10.1016/j.annepidem.2016.02.008
Keywords: methamphetamine, intestinal barrier, tight junction (TJ), cytokines, the brain-gut axis
Citation: Li Y, Kong D, Bi K and Luo H (2022) Related Effects of Methamphetamine on the Intestinal Barrier via Cytokines, and Potential Mechanisms by Which Methamphetamine May Occur on the Brain-Gut Axis. Front. Med. 9:783121. doi: 10.3389/fmed.2022.783121
Received: 25 September 2021; Accepted: 23 March 2022;
Published: 10 May 2022.
Edited by:
Kulmira Nurgali, Victoria University, AustraliaReviewed by:
Andreas Büttner, University Hospital Rostock, GermanyKeisaku Sato, Indiana University, United States
Copyright © 2022 Li, Kong, Bi and Luo. This is an open-access article distributed under the terms of the Creative Commons Attribution License (CC BY). The use, distribution or reproduction in other forums is permitted, provided the original author(s) and the copyright owner(s) are credited and that the original publication in this journal is cited, in accordance with accepted academic practice. No use, distribution or reproduction is permitted which does not comply with these terms.
*Correspondence: Huayou Luo, a20tbGh5QHFxLmNvbQ==