- 1Department of BioSciences, Rice University, Houston, TX, United States
- 2Department of Nutritional Sciences, Texas Christian University, Fort Worth, TX, United States
Obesity is considered an epidemic by the World Health Organization. In particular, maternal obesity can affect the development of obesity and other related metabolic disorders in infants. Recently, both animal and human studies have pointed to the importance of the gut microbiome in facilitating the transmission of the obesity phenotype from mother to offspring. The gut microbiome changes significantly during the progression of pregnancy, and the microbiota of the amniotic fluid and placenta have recently been shown to colonize the infant gut in utero. Microbial composition, diversity, and richness are significantly altered by maternal obesity, which in turn affects the infant’s acquisition of the gut microbiome and their risk to develop metabolic disorders. C-section has also been shown to affect the colonization of the infant gut and offspring metabolic and immune health. This narrative review seeks to discuss the role of the gut microbiome in the transmission of the obesity phenotype from mother to child, as well as how birth delivery, breastfeeding, and probiotic interventions may modulate this relationship.
1 Introduction
Approximately 40% of women in the US had obesity (1). Maternal obesity can increase the risk of childhood obesity by >2 times (2, 3) which is alarming due to the high obesity levels worldwide (4). Both human and animal models have demonstrated that maternal obesity can contribute to many obesity-linked metabolic and immune disorders in offspring, including increased risk of hypertension, insulin resistance, and systemic inflammation (5, 6). There have been many mechanisms hypothesized to contribute to the intergenerational transmission of obesity, including the previously recognized genetic and environmental factors and the newly identified gut microbial composition.
The gut microbiome of an infant develops significantly during the first year of life and recent animal and human research is trying to explore the role of the gut microbiome in the development of diseases (7, 8). Many human studies have shown that the diversity and composition of the gut microbiome are significantly altered in pregnant women with obesity, which in turn can play a factor in the development of obesity in children (9, 10). For instance, pregnant women with obesity tend to have higher levels of Bacteroides and Staphylococcus in their third trimester when compared to pregnant women of normal weight (9). Recently, research has established that the maternal gut microbiota exists in the placenta of pregnant women, which strongly supports that the maternal microbiome may be transferred to the infant prior to birth, playing an important role in establishing the infant gut microbiome (11, 12). Thus, there is a need to assess how obesity can affect this transmission and in turn lead to the development of other transgenerational metabolic disorders.
The study of the transmission of the maternal gut microbiota to infants, however, can be complicated by a host of other factors including the method of birth delivery, breastfeeding, and the use of antibiotic and probiotic interventions at an early age (7, 13). Cesarean delivery, or C-section, is a surgical procedure by which an infant is delivered through an incision in the mother’s abdomen, often in the case that vaginal delivery may put the mother or infant at risk. C-section has been shown to alter the gut microbiome in the first year of life, with infants born through C-section having lower Bifidobacterium, Streptococcus, and Lactobacillus genera (2). However, the simultaneous effect of maternal obesity during pregnancy and C-section has not been studied which is represents a key gap in knowledge as mothers with overweight or obesity are more likely to give birth by C-section (14, 15).
Probiotics have been shown to have beneficial effects on the gut microbiota of infants born through C-section, helping it to more closely resemble that of infants born through vaginal delivery (16). However, it is unclear how this effect may differ between babies born to mothers with normal weight versus those born to mothers with obesity.
This review aims to evaluate how the gut microbiome is potentially involved in mother-to-infant obesity phenotype transmission and how this relationship may be modified by the method of birth delivery, breastfeeding, and the use of probiotic treatments. Furthermore, the impact of maternal obesity on their child’s metabolic and immune systems by potentially acting through the passage of gut microbial composition will also be discussed.
2 Maternal obesity and pregnancy
2.1 Changes in the gut microbiome during pregnancy
To support the development of a growing fetus, the body undergoes many changes that often resemble changes associated with metabolic disorders, including decreased insulin sensitivity and higher levels of inflammatory cytokines (17–19). However, unlike metabolic syndrome, changes in insulin sensitivity and increased adiposity are protective in pregnancy and generally increase nutrient intake for the growing fetus (18, 20). Analysis of the gut microbial composition of pregnant women has shown a significant reduction of phylogenetic diversity from the first to the third trimester (21). Levels of inflammatory cytokines such as IL-2, IL-6, TNF-α, and IFN-γ were also significantly elevated in the stool samples of the third trimester (21). Furthermore, transferring stool samples from women in their third trimester to germ-free mice induced weight gain, decreased insulin sensitivity, and increased inflammation (21). As Proteobacteria have previously been associated with inflammation and dysbiosis in human studies, the gut microbiome provides a possible mechanism by which metabolism and immunity are altered during pregnancy (22, 23). Other studies have similarly suggested that the gut microbiome diversity significantly changes throughout pregnancy; however, conflicting evidence exists (24–26). Therefore, more investigation must be completed to elucidate the modifications in the maternal gut microbiome during pregnancy and how these contribute to immune and metabolic changes in both mother and infant.
2.2 Effects of maternal obesity on pregnancy-related gut microbiome changes
Pregnant women with obesity have significantly higher levels of Bacteroides, Staphylococcus, and Clostridium (9). Bacteroides species have been previously reported to harvest energy more efficiently than other microbes in both human and animal models (9, 27). A significant increase in the relative abundance of Staphylococcus and Enterobacteriaceae species as well as Escherichia coli have also been detected in pregnant women with obesity when compared to pregnant women with normal weight. However, unlike Collado et al., they found a significantly lower level of Bacteroides (28). Although the precise relationship between gut microbial composition and weight gain during pregnancy remains to be clarified, it is clear that significant differences in the gut microbial composition of pregnant women with obesity versus pregnant women with a normal BMI do exist.
Short-chain fatty acids (SCFAs) are products of bacterial metabolism in the gut and include acetic acid, butyric acid, and propionic acid. During pregnancy, changes in the gut microbiome result in altered levels of SCFAs. Studies on microbial populations in pregnant women found that women with obesity have significantly reduced levels of butyrate-producing species such as those in the genus Clostridiales and Lachnospiraceae, and therefore, much lower levels of circulating butyrate (29). Animal models have shown that butyric acid contributes to anti-inflammatory activity by activating regulatory T (Treg) cells and inhibiting IL-17 release (30). Indeed, administration of butyrate to pregnant mice has been demonstrated to reduce levels of pro-inflammatory factors including TNFα and IL1-β (31). Similarly, propionic acid, which is produced by Bacteroides and Firmicutes species, is reported to have beneficial health effects in both rodents and humans, although the mechanisms of these effects are still relatively unknown (32). On the contrary, propionic acid is positively correlated with HbA1c and plasma glucose levels in pregnant women with obesity (33). Therefore, there is emerging evidence suggesting that changes in the gut microbiome contribute to increased inflammation during pregnancy for women with obesity due to differences in SCFA abundance.
SCFAs have also been reported to have relations with hypertension and type 2 diabetes mellitus (T2DM), metabolic disorders associated with obesity. Bacteroides (SCFA producers) are reported to have a negative correlation with blood pressure while rats with hypertension were found to have decreased quantity of SCFA-producing bacteria (34, 35). While similar observations have been made for pregnant women, it is still unclear how exactly SCFAs contribute to hypertension throughout pregnancy. Similarly, patients with T2DM have decreased concentration of butyrate-producing bacterial species (36). Since butyrate and other SCFAs have been reported to increase insulin sensitivity and glucagon-like-peptide-1 (GLP1) release in animal studies, reduced SCFA levels in pregnant women, and especially pregnant women with obesity, may be contributing factors for the insulin resistance commonly observed during pregnancy (37, 38). Altogether, these studies suggest that the gut microbiota and its byproducts contribute to the metabolic and immune system between women of normal weight and women with obesity.
3 Maternal obesity and the infant gut microbiome
3.1 Establishment of the infant gut microbiome
The sterile womb hypothesis holds that human infants are born in a sterile environment facilitated by the placental barrier (39). Human studies conducted by Theodore Escherich and Burrage seemed to confirm that neither the amniotic fluid nor placenta contained any bacteria during pregnancy (40). Even recently, researchers confirmed in human studies that the meconium, or the first stool passed by infants, was largely free from any bacteria (41). However, the use of techniques such as quantitative reverse transcription PCR (RT-qPCR) and 16S-rRNA sequencing in human research beginning in the late 20th and early 21st centuries has elucidated findings that challenge the sterile womb hypothesis (42–44). Recent research suggests that in humans, both the fetal lungs and placenta have distinct microbiome profiles as early as the first trimester of pregnancy, and other studies have confirmed that bacterial DNA is present in the human placenta (11, 45). Likewise, the placenta and amniotic fluid have distinct microbiota profiles that share features with the bacteria present in infant meconium, which is characterized by low richness and diversity and high abundance of Proteobacteria. Similar findings have been confirmed in animal models, in which mice fetal intestinal bacteria overlaps with the microbiome profile of the placenta (46). In a study of 21 healthy human neonates, researchers found that bacteria of the genera Enterococcus and Staphylococcus were most prevalent in meconium samples (47). Meconial bacterial composition isolated from the stool of neonates born to healthy mothers both vaginally and by C-section has also been found to differ significantly from the stool of healthy adults, with higher abundance of Proteobacteria and lower abundance of Bacteroidetes (48). Together, these findings further challenge the sterile womb hypothesis (49). However, the exact route of transmission from the mother’s gut to the placenta and the developing fetus has yet to be clarified.
3.2 Effects of maternal obesity on the establishment of the infant gut microbiome
Maternal obesity can significantly alter the composition of the offspring gut microbiome during pregnancy and therefore impact the establishment of the infant’s gut microbiome. Human infants born vaginally to mothers with overweight or obesity had significantly higher levels of Bacteroides compared to infants born vaginally to mothers of normal weight (2). Higher species richness within the Firmicutes phylum, as well as lower abundance of the Proteobacteria families Enterobacteriaceae and Pasteurellaceae and a higher abundance of the Firmicutes family Lachnospiraceae, have been reported in infants born to mothers with overweight or obesity (14). These infants were also approximately three times more likely to develop overweight or obesity between 1 and 3 years of age (14). The Lachnospiraceae family has been found to be associated with the development of diabetes and adiposity in obese mice (50, 51) however, in humans, Lachnospiraceae has shown to be significantly lower in pregnant women with obesity (29). This contradiction illustrates the difficulty of translating animal to human gut microbiota research. Animal studies have demonstrated that high fat diets (HFD) can induce gut dysbiosis, and this obesity-associated gut microbial composition can significantly impact the gut microbial signature of the offspring (52). Fecal transplants from mice fed HFDs cause a reduction in beta-diversity and reduced abundance of Firmicutes species when compared to those fed a control diet (52). Female offspring born to the dams that received the stool transplant from HFD mice also had significantly higher body weight and body fat composition 9 weeks after birth when compared to those born to control diet dams, while male offspring had significantly increased anxious and compulsive behavior (52). Firmicutes species produce the SCFA butyrate, which has been shown to affect inflammation, behavioral and neurological function, and the maintenance of the gut intestinal barrier in rodents (52–54). It is hypothesized that decreased levels of circulating butyrate may increase the permeability of the intestine and facilitate microbial transfer from the intestinal lumen into the circulation, which could disrupt brain signaling pathways in both humans and rodents (53, 55). Studies in animals have demonstrated that butyrate increases insulin sensitivity while reducing inflammation and food intake; a decrease in butyrate concentration due to both pregnancy and obesity can further influence the maternal and thus the infant’s gut metabolism and immune system (30, 37, 56).
Studies in humans estimate that the odds of developing childhood obesity for infants born to mothers with obesity are between 1.5 and 4 times higher when compared to infants born to mothers of normal weight (2, 14, 57). Research has shown that offspring born to women with obesity had lower levels of fecal butyrate as well as reduced abundance of SCFA-producing bacteria (58). Surprisingly, and in an opposite direction of what we would have expected, researchers also found that the microbiome of infants born to mothers with obesity had greater alpha-diversity at 12 months when compared to infants born to mothers of normal weight, and this alpha-diversity moderately predicted greater adiposity at 12 months of age (58). Thus, it seems that the maternal obesity-associated development of the infant gut microbiome may contribute to the intergenerational transmission of the obesity phenotype (Table 1).
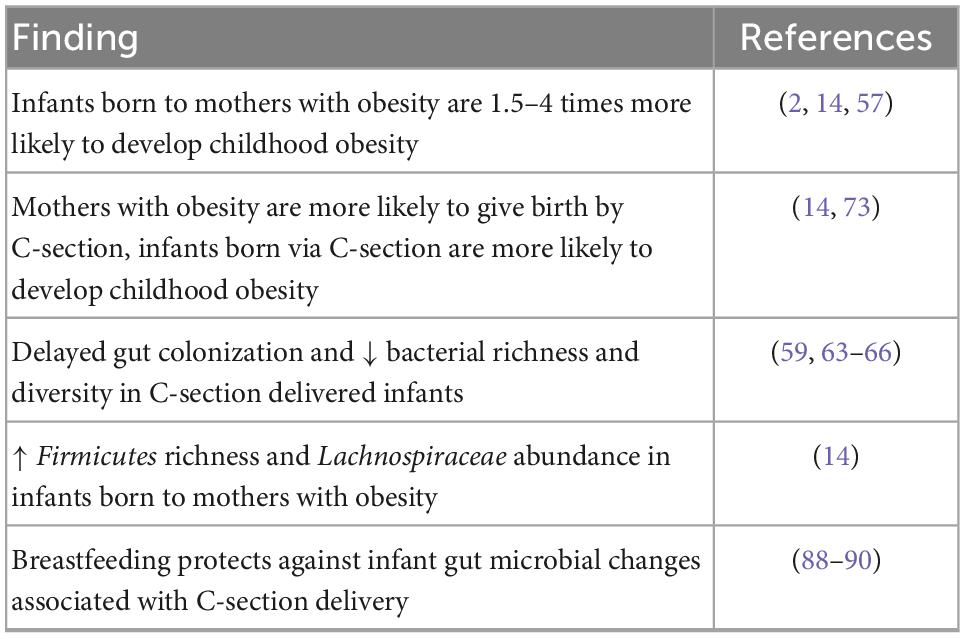
Table 1. Summary of the impact of human maternal obesity on offspring obesity and gut microbiome colonization.
4 Birth delivery methods and the infant gut microbiome
4.1 Effects of C-section on the establishment of the infant gut microbiome
In addition to exposure to microbiota in the uterine environment, the colonization of the human infant gut microbiome is also affected by the method of delivery. During vaginal delivery, species of the vagina also contribute to the establishment of the infant intestinal bacteria, and this process has been reported to be disturbed during C-section (59). While a C-section may be critical in reducing infant and maternal mortality in many cases, the differences in gut microbial composition when comparing infants delivered through C-section versus those born vaginally, may have long-term consequences on the offspring’s immune system and metabolism.
Studies in humans have shown that the gut microbiome of infants born via C-section closely resembles that found on the skin (60). This may indicate that unlike infants who are born vaginally, infants born through C-section acquire microbiota through the operating room environment due to the lack of perineal and vaginal contact during delivery (61, 62). Delayed colonization of the gut by species including Bacteroides, Lactobacillus, Bifidobacterium, and Bacteroidetes in infants born by Csection has been demonstrated by multiple human studies (63–66). Reyman et al. confirmed that at 1 week, infants born vaginally have significantly higher levels of Bifidobacterium, which has shown to be associated with positive health outcomes (67). In a study conducted with 82 newborns, infants born by C-section had significantly higher levels of Staphylococcus and Streptococcus genera when compared to infants born vaginally, with Staphylococcus species remaining significantly greater after 1 month (63). Infants born by C-section were also found to have significantly lower bacterial richness and diversity than infants born vaginally, as well as high levels of Firmicutes, especially Clostridium and Enterococcus (59, 65, 68). Furthermore, researchers have found that bacterial richness, diversity, and composition differ significantly between elective and emergency C-sections (59, 69). Therefore, the mechanism by which these different procedures contribute to the development of the infant gut microbiome needs to be further clarified.
4.2 Association between birth delivery and maternal obesity in the transmission of the obesity phenotype
Staphylococcus levels are significantly elevated in human infants born by C-section (63). This genus has been shown to be positively associated with obesity and increased energy intake, and as such, it is possible that infants born by C-section may also be at risk for obesity (70–73). Similarly, infants born through C-section have lower α-diversity which has been associated with higher risk to develop obesity and type 2 diabetes (13, 27, 74). Infants born by C-section were between 1.4 and 1.7 times more likely to develop childhood overweight or obesity after adjusting for potential confounders (2, 73, 75, 76).
It should also be noted that maternal overweight and obesity are associated with 1.5 times greater odds of giving birth by C-section, which could in turn mediate the association between maternal and child obesity and thus should be controlled in studies analyzing the gut microbiota (14).
5 Discussion
Several factors can be employed to prevent or ameliorate the negative effects brought by maternal obesity and C-section. Probiotic administration during pregnancy, has shown promising results. Particularly, it has shown to increase Bifidobacterium abundance in infants (77), and in pre-term infants it has shown to improve intestinal colonization, intestinal barrier, and bacterial diversity, with a reduction of inflammatory cytokines, which would have otherwise contributed to necrotizing enterocolitis, highly common in preterm infants (78, 79). Importantly, however, changes in the microbiome seem to stop after probiotic cessation (80). Thus, the effects of probiotics seem to be short lived.
Human breast milk contains many bacterial species that support infant metabolic and immune development (81). Human milk increases microbial diversity, stimulates the growth of beneficial species such as Bifidobacterium, and decreases the risk for infections (81–83). Bifidobacterium longum in particular, is elevated in human infants that are breastfed, and has been shown to reduce gut permeability (84, 85). Breastfeeding also seems to protect the gut microbiota of babies born through C-section helping them to more closely resemble the gut microbiome of infants born vaginally (86–88). Furthermore, breastfeeding has been shown to decrease the risk of developing obesity and diabetes in childhood and adolescence (89, 90). Unfortunately, pregnant women with obesity, who are more likely to undergo C-section, also show poor breastfeeding practices. Therefore, these practices can individually and in combination reinforce the intergenerational transmission of obesity (Figure 1).
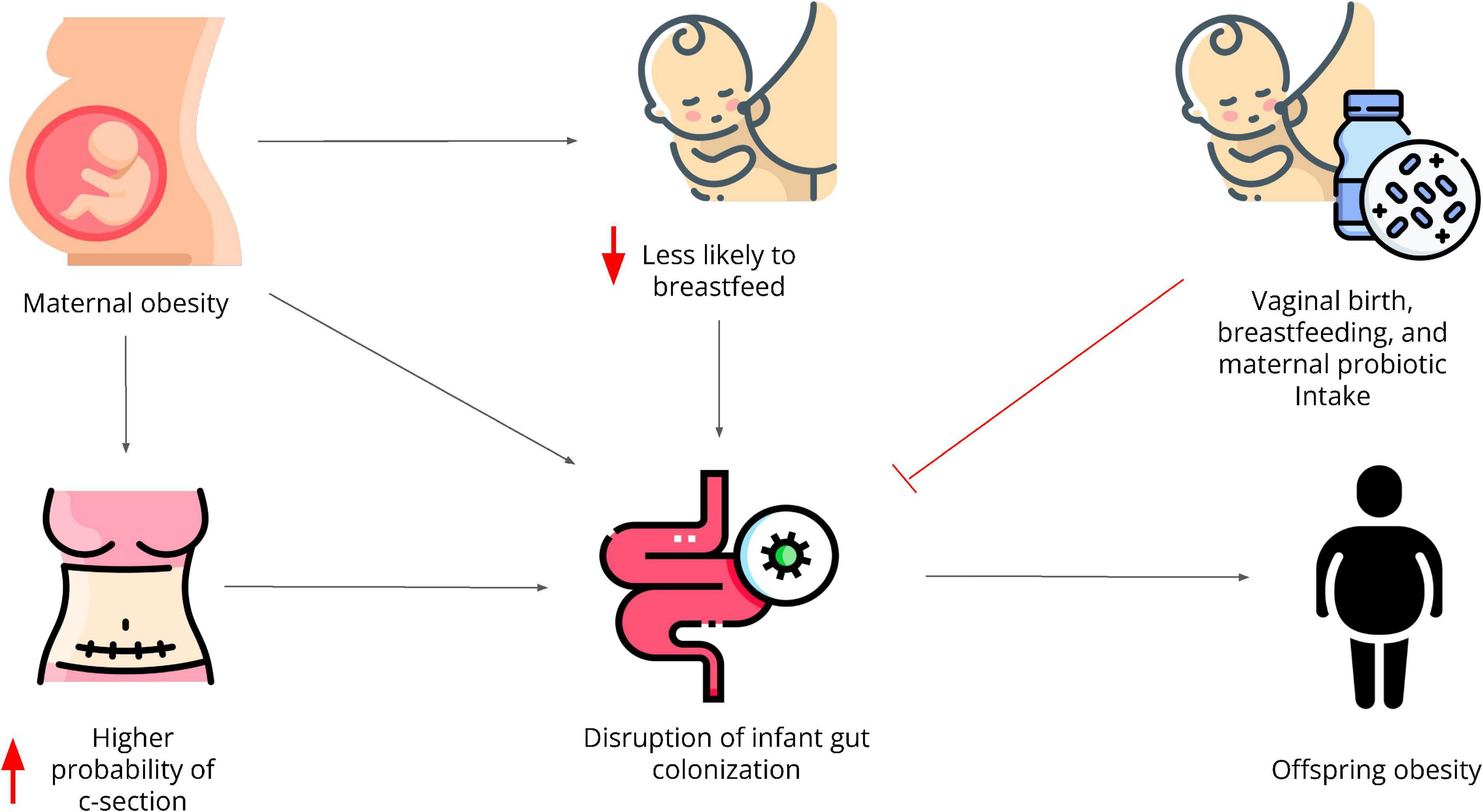
Figure 1. Maternal obesity directly and indirectly contributes to the intergenerational transmission of obesity through disruptions in the gut microbiota. It increases the likelihood to undergoing C-section and it decreases the likelihood of breastfeeding, both of which have independently shown to negatively affect the offspring’s gut microbiome. Infants with an altered gut microbiota are at higher risk to develop obesity amongst many other comorbidities. Vaginal birth, breastfeeding, and probiotic consumption during pregnancy have shown to protect the infant’s gut microbial diversity and composition.
In summary, the gut microbiome may be a key factor in the intergenerational transmission of obesity. Obesity alters a pregnant woman’s gut microbiome’s richness, diversity, composition, and thus derived metabolites, and this microbiota is transferred in utero to the infant, which seems to increase their future risks of developing obesity. In addition to maternal obesity, C-section also puts infants at greater risk for developing obesity which is concerning as mothers with obesity are more likely to undergo C-sections and less likely to breastfeed, activity that could help to ameliorate the negative impact of C-section.
To this day, however, identification of the specific gut bacterial species related to the intergenerational transmission of obesity has not been possible. Determining the gut bacterial species and gut-derived metabolites associated with obesity in humans has been difficult due to the high variation in analytical procedures. We encourage future research to study, in addition to gut bacterial composition, the gut bacteria-derived metabolome as the association between these two components can help to better understand the profiles associated with obesity development and transmission. Understanding the gut bacterial composition can help us to identify the direct interactions with the host, whereas analyzing the metabolome can contribute to detect indirect interactions through metabolite production.
Author contributions
MT conceived the presented idea and wrote the initial draft of the manuscript. EM guided, supervised, and provided critical feedback that helped shape the review to its final form. Both authors contributed to the article and approved the submitted version.
Acknowledgments
EM was a postdoc at the University of Texas Health Science Center at Houston (UT-Health) during the initial stages of the project and thus the authors would like to thank UT-Health for providing the platform for MT and EM to meet. The authors would also like to thank Dr. Sarah Messiah, from UT-Health, for establishing the link between them to work together.
Conflict of interest
The authors declare that the research was conducted in the absence of any commercial or financial relationships that could be construed as a potential conflict of interest.
Publisher’s note
All claims expressed in this article are solely those of the authors and do not necessarily represent those of their affiliated organizations, or those of the publisher, the editors and the reviewers. Any product that may be evaluated in this article, or claim that may be made by its manufacturer, is not guaranteed or endorsed by the publisher.
References
1. Centers for Disease Control and Prevention [CDC],. Products - data briefs - number 360 - February 2020 [Internet]. (2020). Available online at: https://www.cdc.gov/nchs/products/databriefs/db360.htm (accessed July, 2022).
2. Mueller NT, Shin H, Pizoni A, Werlang IC, Matte U, Goldani MZ, et al. Birth mode-dependent association between pre-pregnancy maternal weight status and the neonatal intestinal microbiome. Sci Rep. (2016) 6:23133. doi: 10.1038/srep23133
3. Wang Y, Lim H. The global childhood obesity epidemic and the association between socioeconomic status and childhood obesity. Int Rev Psychiatry. (2012) 24:176–88. doi: 10.3109/09540261.2012.688195
4. World Health Organization [WHO],. Obesity and overweight [Internet]. (2021). Available online at: https://www.who.int/news-room/fact-sheets/detail/obesity-and-overweight (accessed July, 2022).
5. Wilson RM, Messaoudi I. The impact of maternal obesity during pregnancy on offspring immunity. Mol Cell Endocrinol. (2015) 418:134–42. doi: 10.1016/j.mce.2015.07.028
6. O’Reilly JR, Reynolds RM. The risk of maternal obesity to the long-term health of the offspring. Clin Endocrinol. (2013) 78:9–16. doi: 10.1111/cen.12055
7. Zhou L, Xiao X. The role of gut microbiota in the effects of maternal obesity during pregnancy on offspring metabolism. Biosci Rep. (2018) 38:BSR20171234. doi: 10.1042/BSR20171234
8. Heiss CN, Olofsson LE. Gut microbiota-dependent modulation of energy metabolism. J Innate Immun. (2018) 10:163–71. doi: 10.1159/000481519
9. Collado MC, Isolauri E, Laitinen K, Salminen S. Distinct composition of gut microbiota during pregnancy in overweight and normal-weight women. Am J Clin Nutr. (2008) 88:894–9. doi: 10.1093/ajcn/88.4.894
10. Singh SB, Madan J, Coker M, Hoen A, Baker ER, Karagas MR, et al. Does birth mode modify associations of maternal pre-pregnancy BMI and gestational weight gain with the infant gut microbiome? Int J Obes. (2020) 44:23–32. doi: 10.1038/s41366-018-0273-0
11. Al Alam D, Danopoulos S, Grubbs B, Ali NABM, MacAogain M, Chotirmall SH, et al. Human fetal lungs harbor a microbiome signature. Am J Respir Crit Care Med. (2020) 201:1002–6. doi: 10.1164/rccm.201911-2127LE
12. Sola-Leyva A, Andrés-León E, Molina NM, Terron-Camero LC, Plaza-Díaz J, Sáez-Lara MJ, et al. Mapping the entire functionally active endometrial microbiota. Hum Reprod. (2021) 36:1021–31. doi: 10.1093/humrep/deaa372
13. Bokulich NA, Chung J, Battaglia T, Henderson N, Jay M, Li H, et al. Antibiotics, birth mode, and diet shape microbiome maturation during early life. Sci Transl Med. (2016) 8:343ra82. doi: 10.1126/scitranslmed.aad7121
14. Tun HM, Bridgman SL, Chari R, Field CJ, Guttman DS, Becker AB, et al. Roles of birth mode and infant gut microbiota in intergenerational transmission of overweight and obesity from mother to offspring. JAMA Pediatr. (2018) 172:368–77. doi: 10.1001/jamapediatrics.2017.5535
15. Chu SY, Kim SY, Schmid CH, Dietz PM, Callaghan WM, Lau J, et al. Maternal obesity and risk of cesarean delivery: a meta-analysis. Obes Rev. (2007) 8:385–94. doi: 10.1111/j.1467-789X.2007.00397.x
16. Korpela K, Salonen A, Vepsäläinen O, Suomalainen M, Kolmeder C, Varjosalo M, et al. Probiotic supplementation restores normal microbiota composition and function in antibiotic-treated and in caesarean-born infants. Microbiome. (2018) 6:182. doi: 10.1186/s40168-018-0567-4
17. Barbour LA, McCurdy CE, Hernandez TL, Kirwan JP, Catalano PM, Friedman JE. Cellular mechanisms for insulin resistance in normal pregnancy and gestational diabetes. Diabetes Care. (2007) 30(Suppl. 2):S112–9. doi: 10.2337/dc07-s202
18. Catalano PM, Tyzbir ED, Roman NM, Amini SB, Sims EAH. Longitudinal changes in insulin release and insulin resistance in nonobese pregnant women. Am J Obstet Gynecol. (1991) 165:1667–72. doi: 10.1016/0002-9378(91)90012-G
19. Kirwan JP, Hauguel-De Mouzon S, Lepercq J, Challier JC, Huston-Presley L, Friedman JE, et al. TNF-α is a predictor of insulin resistance in human pregnancy. Diabetes. (2002) 51:2207–13. doi: 10.2337/diabetes.51.7.2207
20. von Versen-Hoeynck FM, Powers RW. Maternal-fetal metabolism in normal pregnancy and preeclampsia. Front Biosci. (2007) 12:2457–70. doi: 10.2741/2247
21. Koren O, Goodrich JK, Cullender TC, Spor A, Laitinen K, Kling Bäckhed H, et al. Host remodeling of the gut microbiome and metabolic changes during pregnancy. Cell. (2012) 150:470–80. doi: 10.1016/j.cell.2012.07.008
22. Morgan XC, Tickle TL, Sokol H, Gevers D, Devaney KL, Ward DV, et al. Dysfunction of the intestinal microbiome in inflammatory bowel disease and treatment. Genome Biol. (2012) 13:R79. doi: 10.1186/gb-2012-13-9-r79
23. Shin NR, Whon TW, Bae JW. Proteobacteria: microbial signature of dysbiosis in gut microbiota. Trends Biotechnol. (2015) 33:496–503. doi: 10.1016/j.tibtech.2015.06.011
24. Smid MC, Ricks NM, Panzer A, Mccoy AN, Azcarate-Peril MA, Keku TO, et al. Maternal gut microbiome biodiversity in pregnancy. Am J Perinatol. (2018) 35:24–30. doi: 10.1055/s-0037-1604412
25. Nuriel-Ohayon M, Neuman H, Koren O. Microbial changes during pregnancy, birth, and infancy. Front Microbiol. (2016) 7:1031. doi: 10.3389/fmicb.2016.01031
26. DiGiulio DB, Callahan BJ, McMurdie PJ, Costello EK, Lyell DJ, Robaczewska A, et al. Temporal and spatial variation of the human microbiota during pregnancy. Proc Natl Acad Sci USA. (2015) 112:11060–5. doi: 10.1073/pnas.1502875112
27. Turnbaugh PJ, Ley RE, Mahowald MA, Magrini V, Mardis ER, Gordon JI. An obesity-associated gut microbiome with increased capacity for energy harvest. Nature. (2006) 444:1027–31. doi: 10.1038/nature05414
28. Santacruz A, Collado MC, García-Valdés L, Segura MT, Martín-Lagos JA, Anjos T, et al. Gut microbiota composition is associated with body weight, weight gain and biochemical parameters in pregnant women. Br J Nutr. (2010) 104:83–92. doi: 10.1017/S0007114510000176
29. Wallace JG, Bellissimo CJ, Yeo E, Fei Xia Y, Petrik JJ, Surette MG, et al. Obesity during pregnancy results in maternal intestinal inflammation, placental hypoxia, and alters fetal glucose metabolism at mid-gestation. Sci Rep. (2019) 9:17621. doi: 10.1038/s41598-019-54098-x
30. Zhang M, Zhou Q, Dorfman RG, Huang X, Fan T, Zhang H, et al. Butyrate inhibits interleukin-17 and generates Tregs to ameliorate colorectal colitis in rats. BMC Gastroenterol. (2016) 16:84. doi: 10.1186/s12876-016-0500-x
31. Li HP, Chen X, Li MQ. Butyrate alleviates metabolic impairments and protects pancreatic β cell function in pregnant mice with obesity. Int J Clin Exp Pathol. (2013) 6:1574–84.
32. Ziętek M, Celewicz Z, Szczuko M. Short-chain fatty acids, maternal microbiota and metabolism in pregnancy. Nutrients. (2021) 13:1244. doi: 10.3390/nu13041244
33. Szczuko M, Kikut J, Maciejewska D, Kulpa D, Celewicz Z, Ziętek M. The associations of SCFA with anthropometric parameters and carbohydrate metabolism in pregnant women. Int J Mol Sci. (2020) 21:E9212. doi: 10.3390/ijms21239212
34. Soderborg TK, Carpenter CM, Janssen RC, Weir TL, Robertson CE, Ir D, et al. Gestational diabetes is uniquely associated with altered early seeding of the infant gut microbiota. Front Endocrinol. (2020) 11:603021. doi: 10.3389/fendo.2020.603021
35. Yang T, Santisteban MM, Rodriguez V, Li E, Ahmari N, Carvajal JM, et al. Gut microbiota dysbiosis is linked to hypertension. Hypertension. (2015) 65:1331–40. doi: 10.1161/HYPERTENSIONAHA.115.05315
36. Qin J, Li Y, Cai Z, Li S, Zhu J, Zhang F, et al. A metagenome-wide association study of gut microbiota in type 2 diabetes. Nature. (2012) 490:55–60. doi: 10.1038/nature11450
37. Tolhurst G, Heffron H, Lam YS, Parker HE, Habib AM, Diakogiannaki E, et al. Short-chain fatty acids stimulate glucagon-like peptide-1 secretion via the g-protein–coupled receptor FFAR2. Diabetes. (2012) 61:364–71. doi: 10.2337/db11-1019
38. den Besten G, Bleeker A, Gerding A, van Eunen K, Havinga R, van Dijk TH, et al. Short-chain fatty acids protect against high-fat diet–induced obesity via a PPARγ-dependent switch from lipogenesis to fat oxidation. Diabetes. (2015) 64:2398–408. doi: 10.2337/db14-1213
39. Funkhouser LJ, Bordenstein SR. Mom knows best: the universality of maternal microbial transmission. PLoS Biol. (2013) 11:e1001631. doi: 10.1371/journal.pbio.1001631
40. Escherich T. The intestinal bacteria of the neonate and breast-fed infant. 1885. Rev Infect Dis. (1989) 11:352–6. doi: 10.1093/clinids/11.2.352
41. Hansen R, Scott KP, Khan S, Martin JC, Berry SH, Stevenson M, et al. First-pass meconium samples from healthy term vaginally-delivered neonates: an analysis of the microbiota. PLoS One. (2015) 10:e0133320. doi: 10.1371/journal.pone.0133320
42. Dong XD, Li XR, Luan JJ, Liu XF, Peng J, Luo YY, et al. Bacterial communities in neonatal feces are similar to mothers’ placentae. Can J Infect Dis Med Microbiol. (2015) 26:90–4. doi: 10.1155/2015/737294
43. Nagpal R, Tsuji H, Takahashi T, Kawashima K, Nagata S, Nomoto K, et al. Sensitive quantitative analysis of the meconium bacterial microbiota in healthy term infants born vaginally or by cesarean section. Front Microbiol. (2016) 7:1997. doi: 10.3389/fmicb.2016.01997
44. Aagaard K, Ma J, Antony KM, Ganu R, Petrosino J, Versalovic J. The placenta harbors a unique microbiome. Sci Transl Med. (2014) 6:237ra65. doi: 10.1126/scitranslmed.3008599
45. Satokari R, Grönroos T, Laitinen K, Salminen S, Isolauri E. Bifidobacterium and Lactobacillus DNA in the human placenta. Lett Appl Microbiol. (2009) 48:8–12. doi: 10.1111/j.1472-765X.2008.02475.x
46. Martinez KA, Romano-Keeler J, Zackular JP, Moore DJ, Brucker RM, Hooper C, et al. Bacterial DNA is present in the fetal intestine and overlaps with that in the placenta in mice. PLoS One. (2018) 13:e0197439. doi: 10.1371/journal.pone.0197439
47. Jiménez E, Marín ML, Martín R, Odriozola JM, Olivares M, Xaus J, et al. Is meconium from healthy newborns actually sterile? Res Microbiol. (2008) 159:187–93. doi: 10.1016/j.resmic.2007.12.007
48. Hu J, Nomura Y, Bashir A, Fernandez-Hernandez H, Itzkowitz S, Pei Z, et al. Diversified microbiota of meconium is affected by maternal diabetes status. PLoS One. (2013) 8:e78257. doi: 10.1371/journal.pone.0078257
49. Perez-Muñoz ME, Arrieta MC, Ramer-Tait AE, Walter J. A critical assessment of the “sterile womb” and “in utero colonization” hypotheses: implications for research on the pioneer infant microbiome. Microbiome. (2017) 5:48. doi: 10.1186/s40168-017-0268-4
50. Kameyama K, Itoh K. Intestinal colonization by a Lachnospiraceae bacterium contributes to the development of diabetes in obese mice. Microbes Environ. (2014) 29:427–30. doi: 10.1264/jsme2.ME14054
51. Ravussin Y, Koren O, Spor A, LeDuc C, Gutman R, Stombaugh J, et al. Responses of gut microbiota to diet composition and weight loss in lean and obese mice. Obesity. (2012) 20:738–47. doi: 10.1038/oby.2011.111
52. Bruce-Keller AJ, Fernandez-Kim SO, Townsend RL, Kruger C, Carmouche R, Newman S, et al. Maternal obese-type gut microbiota differentially impact cognition, anxiety and compulsive behavior in male and female offspring in mice. PLoS One. (2017) 12:e0175577. doi: 10.1371/journal.pone.0175577
53. Stilling RM, van de Wouw M, Clarke G, Stanton C, Dinan TG, Cryan JF. The neuropharmacology of butyrate: the bread and butter of the microbiota-gut-brain axis? Neurochem Int. (2016) 99:110–32. doi: 10.1016/j.neuint.2016.06.011
54. Peng L, Li ZR, Green RS, Holzman IR, Lin J. Butyrate enhances the intestinal barrier by facilitating tight junction assembly via activation of AMP-activated protein kinase in Caco-2 cell monolayers. J Nutr. (2009) 139:1619–25. doi: 10.3945/jn.109.104638
55. Lewis K, Lutgendorff F, Phan V, Söderholm JD, Sherman PM, McKay DM. Enhanced translocation of bacteria across metabolically stressed epithelia is reduced by butyrate†. Inflamm Bowel Dis. (2010) 16:1138–48. doi: 10.1002/ibd.21177
56. Li Z, Yi CX, Katiraei S, Kooijman S, Zhou E, Chung CK, et al. Butyrate reduces appetite and activates brown adipose tissue via the gut-brain neural circuit. Gut. (2018) 67:1269–79. doi: 10.1136/gutjnl-2017-314050
57. Portela DS, Vieira TO, Matos SM, de Oliveira NF, Vieira GO. Maternal obesity, environmental factors, cesarean delivery and breastfeeding as determinants of overweight and obesity in children: results from a cohort. BMC Pregnancy Childbirth. (2015) 15:94. doi: 10.1186/s12884-015-0518-z
58. Gilley SP, Ruebel ML, Sims C, Zhong Y, Turner D, Lan RS, et al. Associations between maternal obesity and offspring gut microbiome in the first year of life. Pediatr Obes. (2022) 17:e12921. doi: 10.1111/ijpo.12921
59. Azad MB, Konya T, Maughan H, Guttman DS, Field CJ, Chari RS, et al. Gut microbiota of healthy Canadian infants: profiles by mode of delivery and infant diet at 4 months. CMAJ. (2013) 185:385–94. doi: 10.1503/cmaj.121189
60. Chu DM, Ma J, Prince AL, Antony KM, Seferovic MD, Aagaard KM. Maturation of the infant microbiome community structure and function across multiple body sites and in relation to mode of delivery. Nat Med. (2017) 23:314–26. doi: 10.1038/nm.4272
61. Shin H, Pei Z, Martinez KA, Rivera-Vinas JI, Mendez K, Cavallin H, et al. The first microbial environment of infants born by C-section: the operating room microbes. Microbiome. (2015) 3:59. doi: 10.1186/s40168-015-0126-1
62. Shao Y, Forster SC, Tsaliki E, Vervier K, Strang A, Simpson N, et al. Stunted microbiota and opportunistic pathogen colonization in caesarean-section birth. Nature. (2019) 574:117–21. doi: 10.1038/s41586-019-1560-1
63. Pan K, Zhang C, Tian J. The effects of different modes of delivery on the structure and predicted function of intestinal microbiota in neonates and early infants. Pol J Microbiol. (2021) 70:45–55. doi: 10.33073/pjm-2021-002
64. Dogra S, Sakwinska O, Soh SE, Ngom-Bru C, Brück WM, Berger B, et al. Dynamics of infant gut microbiota are influenced by delivery mode and gestational duration and are associated with subsequent adiposity. mBio. (2015) 6:e02419-14. doi: 10.1128/mBio.02419-14
65. Jakobsson HE, Abrahamsson TR, Jenmalm MC, Harris K, Quince C, Jernberg C, et al. Decreased gut microbiota diversity, delayed Bacteroidetes colonisation and reduced Th1 responses in infants delivered by Caesarean section. Gut. (2014) 63:559–66. doi: 10.1136/gutjnl-2012-303249
66. Biasucci G, Rubini M, Riboni S, Morelli L, Bessi E, Retetangos C. Mode of delivery affects the bacterial community in the newborn gut. Early Hum Dev. (2010) 86:13–5. doi: 10.1016/j.earlhumdev.2010.01.004
67. Reyman M, van Houten MA, van Baarle D, Bosch AATM, Man WH, Chu MLJN, et al. Impact of delivery mode-associated gut microbiota dynamics on health in the first year of life. Nat Commun. (2019) 10:4997. doi: 10.1038/s41467-019-13373-1
68. Murata C, Gutiérrez-Castrellón P, Pérez-Villatoro F, García-Torres I, Enríquez-Flores S, de la Mora-de la Mora I, et al. Delivery mode-associated gut microbiota in the first 3 months of life in a country with high obesity rates: a descriptive study. Medicine. (2020) 99:e22442. doi: 10.1097/MD.0000000000022442
69. Hoang DM, Levy EI, Vandenplas Y. The impact of Caesarean section on the infant gut microbiome. Acta Paediatr. (2021) 110:60–7. doi: 10.1111/apa.15501
70. Collado MC, Isolauri E, Laitinen K, Salminen S. Effect of mother’s weight on infant’s microbiota acquisition, composition, and activity during early infancy: a prospective follow-up study initiated in early pregnancy. Am J Clin Nutr. (2010) 92:1023–30. doi: 10.3945/ajcn.2010.29877
71. Bervoets L, Van Hoorenbeeck K, Kortleven I, Van Noten C, Hens N, Vael C, et al. Differences in gut microbiota composition between obese and lean children: a cross-sectional study. Gut Pathog. (2013) 5:10. doi: 10.1186/1757-4749-5-10
72. Gomes AC, Hoffmann C, Mota JF. The human gut microbiota: metabolism and perspective in obesity. Gut Microbes. (2018) 9:308–25. doi: 10.1080/19490976.2018.1465157
73. Sitarik AR, Havstad SL, Johnson CC, Jones K, Levin AM, Lynch SV, et al. Association between cesarean delivery types and obesity in preadolescence. Int J Obes. (2020) 44:2023–34. doi: 10.1038/s41366-020-00663-8
74. Kostic AD, Gevers D, Siljander H, Vatanen T, Hyötyläinen T, Hämäläinen AM, et al. The dynamics of the human infant gut microbiome in development and in progression toward type 1 diabetes. Cell Host Microbe. (2015) 17:260–73. doi: 10.1016/j.chom.2015.01.001
75. Li HT, Zhou YB, Liu JM. The impact of cesarean section on offspring overweight and obesity: a systematic review and meta-analysis. Int J Obes. (2013) 37:893–9. doi: 10.1038/ijo.2012.195
76. Pei Z, Heinrich J, Fuertes E, Flexeder C, Hoffmann B, Lehmann I, et al. Cesarean delivery and risk of childhood obesity. J Pediatr. (2014) 164:1068–73.e2. doi: 10.1016/j.jpeds.2013.12.044
77. Gueimonde M, Sakata S, Kalliomäki M, Isolauri E, Benno Y, Salminen S. Effect of maternal consumption of lactobacillus GG on transfer and establishment of fecal bifidobacterial microbiota in neonates. J Pediatr Gastroenterol Nutr. (2006) 42:166–70.
78. Kapourchali FR, Cresci GAM. Early-life gut microbiome—the importance of maternal and infant factors in its establishment. Nutr Clin Pract. (2020) 35:386–405. doi: 10.1002/ncp.10490
79. Wang Q, Dong J, Zhu Y. Probiotic supplement reduces risk of necrotizing enterocolitis and mortality in preterm very low-birth-weight infants: an updated meta-analysis of 20 randomized, controlled trials. J 1Pediatr Surg. (2012) 47:241–8. doi: 10.1016/j.jpedsurg.2011.09.064
80. Martín-Peláez S, Cano-Ibáñez N, Pinto-Gallardo M, Amezcua-Prieto C. The impact of probiotics, prebiotics, and synbiotics during pregnancy or lactation on the intestinal microbiota of children born by cesarean section: a systematic review. Nutrients. (2022) 14:341. doi: 10.3390/nu14020341
81. Lyons KE, Ryan CA, Dempsey EM, Ross RP, Stanton C. Breast milk, a source of beneficial microbes and associated benefits for infant health. Nutrients. (2020) 12:E1039. doi: 10.3390/nu12041039
82. Morozov V, Hansman G, Hanisch FG, Schroten H, Kunz C. Human milk oligosaccharides as promising antivirals. Mol Nutr Food Res. (2018) 62:1700679. doi: 10.1002/mnfr.201700679
83. Lee SA, Lim JY, Kim BS, Cho SJ, Kim NY, Kim OB, et al. Comparison of the gut microbiota profile in breast-fed and formula-fed Korean infants using pyrosequencing. Nutr Res Pract. (2015) 9:242–8. doi: 10.4162/nrp.2015.9.3.242
84. Chichlowski M, De Lartigue G, German JB, Raybould HE, Mills DA. Bifidobacteria isolated from infants and cultured on human milk oligosaccharides affect intestinal epithelial function. J Pediatr Gastroenterol Nutr. (2012) 55:321–7. doi: 10.1097/MPG.0b013e31824fb899
85. Wickramasinghe S, Pacheco AR, Lemay DG, Mills DA. Bifidobacteria grown on human milk oligosaccharides downregulate the expression of inflammation-related genes in Caco-2 cells. BMC Microbiol. (2015) 15:172. doi: 10.1186/s12866-015-0508-3
86. Princisval L, Rebelo F, Williams BL, Coimbra AC, Crovesy L, Ferreira AL, et al. Association between the mode of delivery and infant gut microbiota composition up to 6 months of age: a systematic literature review considering the role of breastfeeding. Nutr Rev. (2021) 80:113–27. doi: 10.1093/nutrit/nuab008
87. Guo C, Zhou Q, Li M, Zhou L, Xu L, Zhang Y, et al. Breastfeeding restored the gut microbiota in caesarean section infants and lowered the infection risk in early life. BMC Pediatr. (2020) 20:532. doi: 10.1186/s12887-020-02433-x
88. Liu Y, Qin S, Song Y, Feng Y, Lv N, Xue Y, et al. The perturbation of infant gut microbiota caused by cesarean delivery is partially restored by exclusive breastfeeding. Front Microbiol. (2019) 10:598. doi: 10.3389/fmicb.2019.00598
89. Ma J, Qiao Y, Zhao P, Li W, Katzmarzyk PT, Chaput J, et al. Breastfeeding and childhood obesity: a 12-country study. Matern Child Nutr. (2020) 16:e12984. doi: 10.1111/mcn.12984
Keywords: gut microbiota, maternal obesity, pregnancy, cesarean delivery, breastfeeding, probiotic
Citation: Tang M and Marroquin E (2022) The role of the gut microbiome in the intergenerational transmission of the obesity phenotype: A narrative review. Front. Med. 9:1057424. doi: 10.3389/fmed.2022.1057424
Received: 29 September 2022; Accepted: 07 December 2022;
Published: 22 December 2022.
Edited by:
Maria Teresa Rocchetti, University of Foggia, ItalyReviewed by:
Sukirth M. Ganesan, The University of Iowa, United StatesCopyright © 2022 Tang and Marroquin. This is an open-access article distributed under the terms of the Creative Commons Attribution License (CC BY). The use, distribution or reproduction in other forums is permitted, provided the original author(s) and the copyright owner(s) are credited and that the original publication in this journal is cited, in accordance with accepted academic practice. No use, distribution or reproduction is permitted which does not comply with these terms.
*Correspondence: Elisa Marroquin, ✉ RS5NYXJyb3F1aW5AdGN1LmVkdQ==