- 1Department of Pulmonary and Critical Care Medicine, The First Affiliated Hospital of Chongqing Medical University, Chongqing, China
- 2Department of Thoracic Surgery, The Third Affiliated Hospital of Chongqing Medical University, Chongqing, China
Background: Extubation failure is common in critically ill patients, especially those with high-risk factors, and is associated with poor prognosis. Prophylactic use of oxygen therapy after extubation has been gradually introduced. However, the best respiratory support method is still unclear.
Purpose: This study aimed to evaluate the efficacy of four post-extubation respiratory support approaches in reducing reintubation and respiratory failure in patients at high-risk of extubation failure.
Methods: A comprehensive search was performed in Cochrane Central Register of Controlled Trials, PubMed, EMBASE, and Web of Science from inception to June 2022. Randomized controlled trials (RCTs) comparing post-extubation preventive use of respiratory management strategies, including conventional oxygen therapy (COT), non-invasive ventilation (NIV), and high-flow nasal catheter (HFNC) in high-risk patients with extubation failure were reviewed. Primary outcomes were reintubation rate and respiratory failure. Secondary outcomes included intensive care unit (ICU) mortality, ICU stay and length of hospital stay (LOS).
Results: Seventeen RCTs comprising 2813 participants were enrolled. Compared with COT, the three respiratory support methods (NIV, HFNC, NIV + HFNC) were all effective in preventing reintubation [odds ratio (OR) 0.46, 95% confidence interval (CI) 0.32–0.67; OR 0.26, 95% CI 0.14–0.48; OR 0.62, 95% CI 0.39–0.97, respectively] and respiratory failure (OR 0.23, 95% CI 0.10–0.52; OR 0.15, 95% CI 0.04–0.60; OR 0.26, 95% CI 0.10–0.72, respectively). NIV and NIV + HFNC also reduced ICU mortality (OR 0.40, 95% CI 0.22–0.74; OR 0.32, 95% CI 0.12–0.85). NIV + HFNC ranked best in terms of reintubation rate, respiratory failure and ICU mortality based on the surface under the cumulative ranking curve (SUCRA) (99.3, 87.1, 88.2, respectively). Although there was no significant difference in shortening ICU stay and LOS among the four methods, HFNC ranked first based on the SUCRA.
Conclusion: Preventive use of NIV + HFNC after scheduled extubation is probably the most effective respiratory support method for preventing reintubation, respiratory failure and ICU death in high-risk patients with extubation failure. HFNC alone seems to be the best method to shorten ICU stay and LOS.
Systematic review registration: [https://www.crd.york.ac.uk/prospero/], identifier [CRD42022340623].
Introduction
Extubation failure still occurs in 10–20% of patients who pass a spontaneous breathing trial (SBT) and undergo planned extubation, and is associated with poor outcomes such as reintubation, prolonged duration of intensive care unit (ICU) stay and hospital stay, and increased mortality (1, 2). For patients at high-risk of extubation failure, such as those older than 65 years and those with underlying cardiopulmonary disease, the rate of reintubation can be as high as 48% (3). And the need to reintubation is related to an increased ICU mortality of 26–50% (4). In addition to the personal challenges on patients and their families, the intensive care related resources these patients receive place a significant burden on the public health system (5). Therefore, it is essential to receive prophylactic respiratory support for post-extubated patients, especially those with high risk factors.
Various respiratory management strategies have been proposed to alleviate extubation failure and reintubation. Conventional oxygen therapy (COT) is the most frequently administered respiratory support method to improve post-extubation hypoxemia. However, the delivered fraction of inspired oxygen (FiO2) of COT such as nasal cannulas and facemasks with reservoirs is unstable (6). And for Venturi masks, one of the COT, oxygen is passively heated and humidified (7). NIV has been recommended for patients at high-risk of reintubation, particularly those with hypercapnia (8). Nevertheless, NIV is prone to aspiration pneumonia, interface intolerance, and patient discomfort (9). High-flow nasal cannula (HFNC) is a novel device that delivers high-concentration humidified oxygen through nasal cannulas, and generates a low level of positive end-expiratory pressure (PEEP) in the upper airways, facilitating alveolar recruitment (10, 11). Moreover, HFNC improves respiratory secretions management and decreases the anatomical dead space ventilation and therefore the CO2 rebreathing (12). But its ability to unload respiratory muscles in high-risk patients with extubation failure may be lower than that provided by NIV (13–15).
Previous meta-analyses have shown that HFNC was superior to COT but non-inferior to NIV in reducing reintubation rates in patients with acute respiratory failure (16, 17). However, the comprehensive effectiveness of these three oxygen therapies for high-risk patients with extubation failure, such as those over 65 years old and those with underlying cardiopulmonary disease, remains unclear. In addition, the use of HFNC during NIV breaks has been introduced recently, and this sequential alternate protocols (NIV + HFNC) could prevent reintubation compared with HFNC alone (18). While the efficacy on reducing mortality in patients at high-risk of extubation failure is controversial (18, 19). Therefore, we performed this network meta-analysis (NMA) to evaluate the comprehensive efficacy of prophylactic use of various oxygen therapies (COT, NIV, HFNC, and NIV + HFNC) on reducing reintubation rate and respiratory failure after planned extubation in patients at high-risk of extubation failure.
Methods
This NMA was reported according to the Preferred Reporting Items for Systematic Reviews and Meta-Analyses (PRISMA) extension statements for reviews incorporating network meta-analyses (Supplementary Table 1) (20). The study protocol was registered on PROSPERO (CRD42022340623).
Search strategy
The search strategy included controlled vocabulary (i.e., Medical Subject Headings) and free-text words for three basic concepts: (1) extubation, (2) high-risk patients with extubation failure, and (3) oxygen therapy, non-invasive ventilation, and high-flow therapy. Two researchers (XZ and RW) independently searched relevant literature in PubMed, Cochrane Central Register of Controlled Trials, Web of Science, and Embase from inception to June 2022, with no language restrictions. The detailed search strategy is presented in Supplementary Table 2. In addition, reference lists of included articles were reviewed. We also tried to contact authors of conference proceedings to obtain unpublished data.
Eligibility criteria
The inclusion criteria showed as following: (1) participants: adult patients (age ≥ 18 years) admitted to the ICU who received invasive mechanical ventilation (IMV) > 12 h, successfully passed the SBT and were ready for extubation, while were at high-risk of extubation failure (4, 13, 21); (2) interventions and comparisons: compared two of the four available devices: COT, NIV, HFNC, and NIV + HFNC. All of these methods were used for preventive purposes; (3) outcomes: the primary outcomes were reintubation rate and respiratory failure, and the second outcomes included ICU mortality, ICU stay and length of hospital stay (LOS). Studies reporting on at least one of the above outcomes were included; and (4) study design: prospective randomized controlled trials (RCTs).
The exclusion criteria included the following: (1) non-RCTs, including reviews, retrospective studies, cohort studies, and crossover studies; (2) more than half of the subjects were post-operative patients; (3) language not in English; (4) studies in which respiratory support was used for therapeutic purpose; (5) abstracts without full-text manuscripts.
According to the previous studies (4, 13, 21), “high risk” of extubation failure was defined as the presence of at least one of the following factors: (1) age > 65 years; (2) underlying cardiopulmonary disease; (3) APACHE II score > 12 at extubation; (4) body mass index > 30 kg/m2; (5) upper airway obstruction with stridor; (6) weak cough; (7) more than one comorbidity; (8) more than one SBT failure; (9) PaCO2 > 45 mmHg after extubation; and (10) duration of IMV > 7 days.
Study selection
After filtering duplicate records, two researchers (XZ and RW) independently selected and evaluated the titles and abstracts of the retrieved literature, and then the shortlisted studies were screened again to assess their adherence to the eligibility criteria. A third reviewer (JD) participated in the discussion to adjudicate disagreements. Language was limited to English during selection.
Data extraction
Data from eligible studies were extracted by two researchers (MM and MG) independently and combined to form a specific data collection sheet. The abstracted data included the name of the first author, publication year, number and locations of study centers, sample size, interventions and comparators, definition of high-risk patients, study outcomes, complications, main reason for intubation, and duration of mechanical ventilation before inclusion. Moreover, age, sex, acute physiology and chronic health evaluation (APACHE) II score on admission, atrial partial pressure of carbon dioxide (PaCO2) at the end of SBT, and oxygenation index at the end of SBT were also recorded. The disagreement was resolved by a joint review of the full text to reach consensus.
Quality assessment
Two researchers (JD and MG) independently assessed the risk of bias for primary outcomes in eligible studies using the Cochrane Risk of Bias tool (22). Each trial was judged as low, unclear, or high risk with respect to adequate sequence generation, allocation concealment, blinding of participants and personnel, blinding of outcome assessment, incomplete outcome data, selective reporting, and other bias. We resolved disagreements by a discussion with a third reviewer (SG) to reach consensus.
Statistical analysis
Direct comparison meta-analysis
A conventional pairwise meta-analysis was performed using RevMan 5.3 (RevMan 2014). Effect sizes from the forest plots were expressed as odds ratios (ORs) and mean differences (MDs), both with 95% confidence intervals (CIs), for categorical and continuous data, respectively. Outcome measures were pooled using a random effect model. A two-sided p-value < 0.05 was considered significant. To evaluate heterogeneity across studies within each direct comparison, we visually inspected the forest plots and quantified using the Q test and the I2 statistic. When heterogeneity was identified (I2 > 50%), we quantified it using the Chi-square test (p value). We planned to use a funnel plot for the possibility of publication bias, if ≥ 10 studies were available.
Geometry of the network
Network plots were constructed to determine the number of studies included in this NMA. We demonstrated the network geometry that presented the nodes as interventions and each head-to-head direct comparison as lines connecting these nodes. The size of the node was proportional to the number of trials that included in each method. The thickness of the connecting line was proportional to the number of direct comparisons.
Network comparison meta-analysis
A random effect NMA was performed using a frequentist framework to calculate ORs for categorical outcomes and MDs for continuous outcomes, with corresponding 95% CIs. The statistical analysis was performed using the Netmeta package in Stata/SE 16.0 (Stata-Corp, College Station, TX, USA). A two-sided p-value < 0.05 was considered statistically significant.
Assessment of the risk of bias across studies followed considerations on pairwise meta-analysis. The indirectness of each study included in the NMA was evaluated according to the relevance to study population, interventions, outcomes, and study setting. The approach to imprecision comprised a comparison of the range of treatment effects included in the 95% CI with the range of equivalence. We assessed the imprecision of treatment effects for a clinically important ORs of <0.8 or >1.25 in the CIs. To evaluate the heterogeneity, we compared the posterior distribution of the estimated heterogeneity variance with its predictive distribution. The concordance between assessments based on CI and prediction intervals, which do and do not capture heterogeneity, respectively, was used to assess the importance of heterogeneity. Inconsistency between direct and indirect estimates in the entire network for each outcome was assessed locally with a loop-specific approach and globally with design-by treatment interaction model (23). And publication bias was assessed visually using a funnel plot (24).
We also ranked the preventive effectiveness of each strategy according to the probability of achieving the best results through the surface under the cumulative ranking curve (SUCRA) (25). The higher the SUCRA value, which ranges from 0 to 100%, the more likely this respiratory support method is to be ranked as best.
Grading the quality of evidence
We evaluated the quality of evidence for each outcome using the modified Grading of Recommendation, Assessment, Development and Evaluation (GRADE) tool for NMA (26). The weight contribution matrix was constructed to assess the information contribution of direct evidence to entire NMA estimates (27). The quality of evidence in NMA would be degraded because of the risk of bias, indirectness, imprecision, publication bias, and incoherence (27).
Sensitive analysis
Given that small sample size and hypercapnia (PaCO2 > 45 mmHg) at the end of SBT might affect the relative effectiveness of respiratory support methods, two sensitivity analyses were performed to assess the robustness of NMA results to the primary outcomes by excluding studies with sample size < 50 or those involving patients with hypercapnia at the end of SBT.
Results
Study selection
The comprehensive database search yielded 5257 records. After excluding 1319 duplicates and 3844 irrelevant citations, we reviewed the full text of the remaining 94 records. Finally, a total of 17 eligible RCTs (3, 13, 15, 18, 19, 28–39), representing 2813 patients, were included in this NMA. A flowchart describing the detailed retrieval strategy is presented in Figure 1.
Study characteristics
The characteristics of each study included in this NMA are summarized in Table 1 and Supplementary Table 3. All the selected studies were published between 2005 and 2022, and the sample size ranged from 29 to 641. Of the 17 included RCTs, 8 (47%) were multicenter (3, 13, 18, 19, 28–30, 33) and 9 (53%) were single-center (15, 31, 32, 34–39). Four trials (23.5%) recruited patients from Spain (3, 13, 28, 29), 4 (23.5%) from China (17, 34, 35, 38), and 3 (17.6%) from France (18, 19, 33). The definition of high-risk factors varied from study to study. Respiratory disease was the most common complication in these high-risk patients. The main reasons for intubation were chronic obstructive pulmonary disease with acute exacerbation (AECOPD) and pulmonary infection. Most patients among the trials were older than 65, with a higher mean proportion of male than female. The APACHE II score on admission was greater than 12 in 9 of 10 (90%) trials (3, 13, 28, 29, 32, 34, 37–39). The PaCO2 at the end of SBT was greater than 45 mmHg in 2 of 12 (16.7%) trials (3, 35). Oxygenation index at the end of SBT was mostly greater than 200 mmHg among the included studies.
Quality assessment
The risk of bias within eligible studies is shown in Figure 2. All trials were assessed as low or unclear risk of bias with respect to random sequence generation and allocation concealment, except for one (39) in which participants were grouped by the admission number. All studies were judged as having a high risk of performance bias because of the inability to blind caregivers to ventilation device. There were seven unclear detection bias due to the unknown definition of reintubation (3, 28, 30, 31, 33, 35, 37). Additionally, one trial (15) had a high risk of other bias associated with the imbalanced baseline.
Pairwise meta-analysis
Compared with COT, NIV was more effective in preventing reintubation, respiratory failure, and ICU mortality. NIV + HFNC reduced the rate of reintubation and respiratory failure compared with HFNC alone. HFNC shortened the ICU stay compared with NIV (Supplementary Figures 1–5).
Network meta-analysis
The included trials evaluated four interventions, including five head-to-head comparisons for reintubation and four head-to-head comparisons for respiratory failure (Supplementary Figure 6). There were two loops in the reintubation network plot (COT-NIV-HFNC; COT-HFNC- NIV + HFNC) (Supplementary Figure 6A). Supplementary Figure 6B showed only one loop in the network plot of respiratory failure (COT-NIV-HFNC). The weight contribution matrix and league table are shown in Supplementary Figures 7–11 and Supplementary Table 4.
Reintubation
Sixteen studies were included in the analysis of reintubation (3, 13, 15, 18, 19, 28–37, 39). All the three methods (NIV, HFNC, NIV + HFNC) were superior to COT in reintubation (OR 0.46, 95% CI 0.32–0.67; OR 0.62, 95% CI 0.39–0.97; OR 0.26, 95% CI 0.14–0.48, respectively) (Figure 3A). HFNC was comparable to NIV in reducing reintubation rate (OR 1.33, 95% CI 0.94–1.90). Compared to NIV and HFNC, NIV + HFNC prevented reintubation with significant differences (OR 0.57, 95% CI 0.33–0.98; OR 0.43, 95% CI 0.28–0.65, respectively). Figure 4A showed the treatment rankings, which revealed that the hierarchy for efficacy in reducing reintubation was NIV + HFNC (SUCRA 99.3) > NIV (SUCRA 65.5) > HFNC (SUCRA 34.6) > COT (SUCRA 0.6).
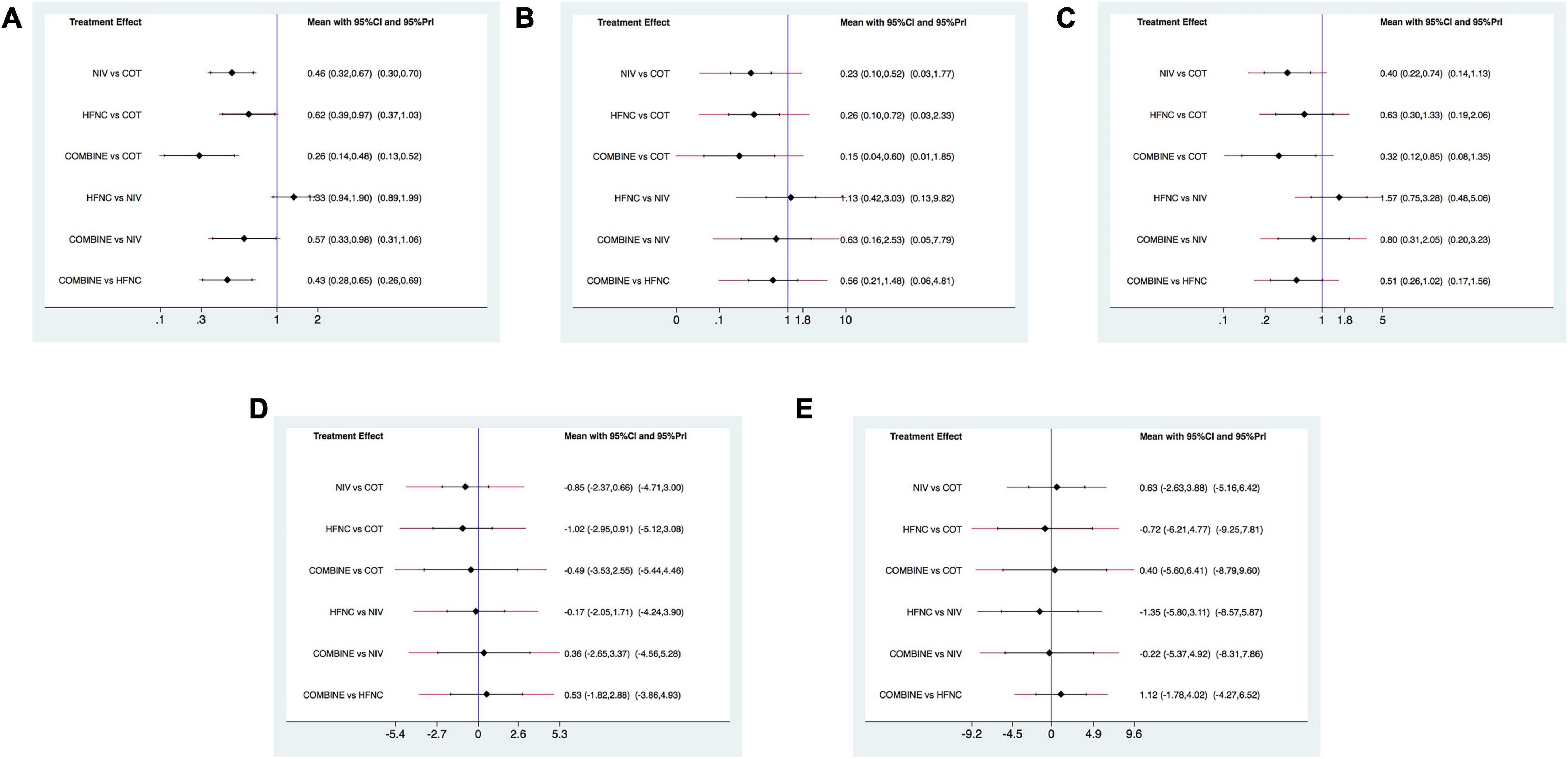
Figure 3. Forest plots for reintubation rate, respiratory failure, ICU mortality, ICU stay, and LOS. (A) Reintubation rate; (B) respiratory failure; (C) ICU mortality; (D) ICU stay; (E) LOS. ICU, intensive care unit; LOS, length of stay; NIV, non-invasive ventilation; COT, conventional oxygen therapy; HFNC, high-flow nasal cannula; CI, confidence interval; Prl, prediction interval.
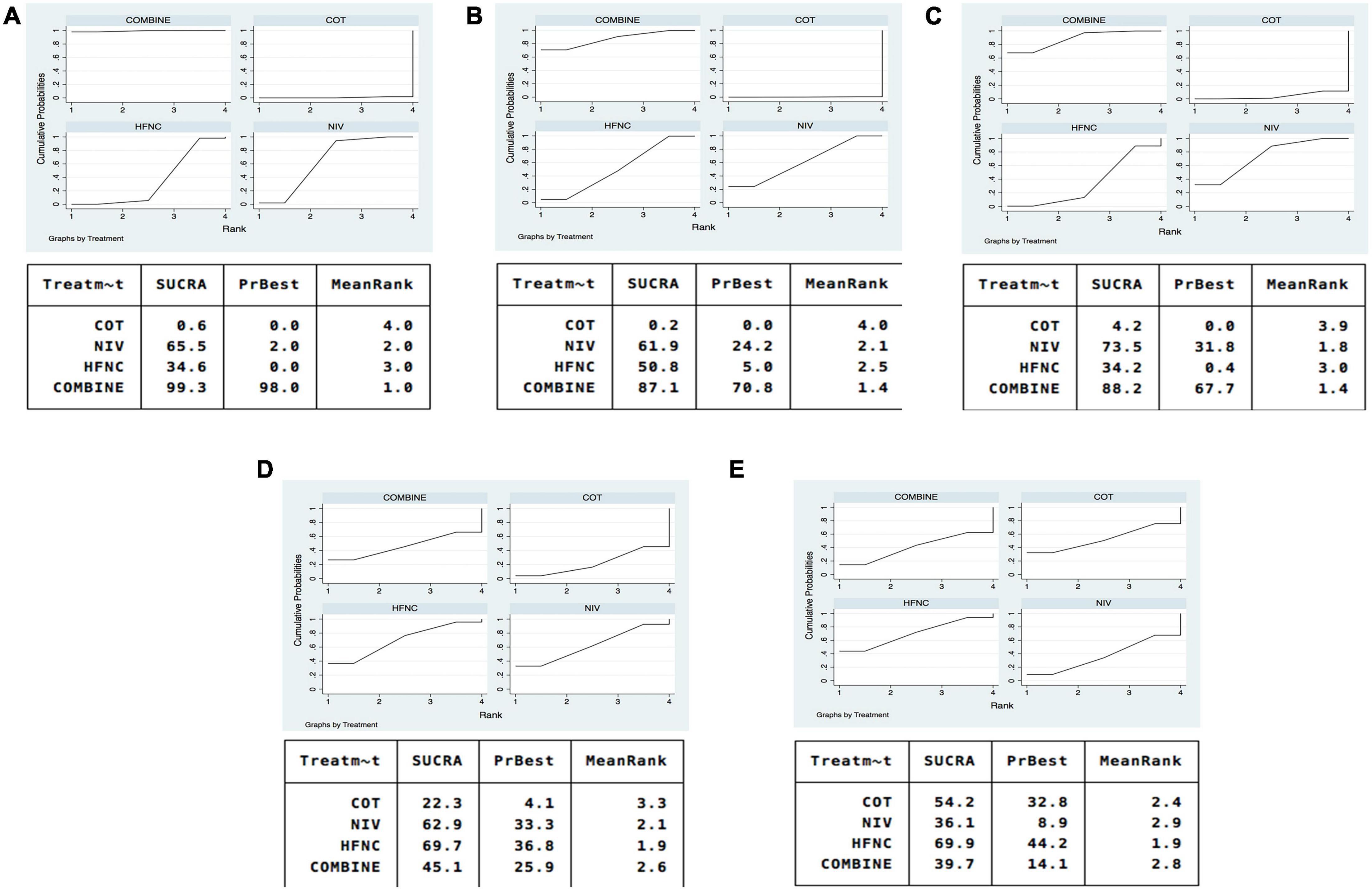
Figure 4. SUCRA of oxygen therapies for reintubation rate, respiratory failure, ICU mortality, ICU stay, and LOS. (A) Reintubation; (B) respiratory failure; (C) ICU mortality; (D) ICU stay; (E) LOS. NIV, non-invasive ventilation; COT, conventional oxygen therapy; HFNC, high-flow nasal cannula; SUCRA, surface under cumulative ranking curve.
The quality of evidence for reintubation estimated by NMA was rated as low to moderate (Table 2A). The study limitation was detected for all the comparisons because of a high risk of performance bias (Figure 2). The funnel plot suggested no publication bias (Supplementary Figure 12). The imprecision of two direct comparisons (HFNC vs. COT and NIV + HFNC vs. COT) resulted in “some concern” because 95% CIs included values favoring either treatment. Quality of evidence for indirect estimates downgraded by one level for serious heterogeneity due to I2 in three comparisons (NIV vs. COT, HFNC vs. COT, and NIV + HFNC vs. COT). And heterogeneity was observed in one network comparison due to the predictive interval (HFNC vs. COT) (Figure 3A). There was no significant difference between direct and indirect comparisons (Supplementary Figures 13, 14), indicating the consistency of different studies.
Respiratory failure
Respiratory failure was reported in 10 trials (3, 13, 18, 19, 28, 29, 33, 35, 38, 39). The network estimates suggested that NIV, HFNC and NIV + HFNC were associated with a lower risk of respiratory failure compared with COT (OR 0.23, 95% CI 0.10–0.52; OR 0.26, 95% CI 0.10–0.72; OR 0.15, 95% CI 0.04–0.60, respectively) (Figure 3B). We found no significant difference in respiratory failure among NIV, HFNC, and NIV + HFNC (OR 1.13, 95% CI 0.42–3.03; OR 0.63, 95% CI 0.16–2.53; OR 0.56, 95% CI 0.21–1.48, respectively). Figure 4B showed that NIV + HFNC ranked first in reducing respiratory failure (SUCRA 87.1).
The quality of evidence for respiratory failure assessed by NMA was rated as low (Table 2B). There was still a high risk of performance bias in studies involving respiratory failure (Figure 2). Supplementary Figure 15 indicated no significant publication bias. Two network comparisons were heterogeneous due to the predictive interval (NIV vs. COT, and HFNC vs. COT) and the other two were imprecise due to the 95% CIs (HFNC vs. NIV, and NIV + HFNC vs. HFNC). The inconsistency test at the global and local levels showed no significant difference between direct and indirect comparisons (Supplementary Figures 16, 17).
Intensive care unit mortality
Ten trials reported ICU mortality (3, 13, 15, 18, 19, 28–30, 33, 37). Compared with COT, NIV and NIV + HFNC reduced ICU mortality, with significant differences (OR 0.40, 95% CI 0.22–0.74; OR 0.32, 95% CI 0.12–0.85, respectively) (Figure 3C). HFNC was comparable to COT in reducing ICU mortality (OR 0.63, 95% CI 0.30–1.33). There were no significant differences in ICU mortality among NIV, HFNC, and NIV + HFNC. Figure 4C showed the treatment rankings, revealing that NIV + HFNC (SUCRA 88.2) was the best to alleviate ICU death. Radar map indicated that NIV + HFNC was the most effective method to prevent reintubation, respiratory failure, and ICU death (Supplementary Figure 18). No significant publication bias was detected (Supplementary Figure 19). The imprecision of two network comparisons (HFNC vs. COT and HFNC vs. NIV) resulted in “some concern” (Table 2C). And heterogeneity was observed in one comparison of NMA estimates (NIV vs. COT) (Figure 3C). There was no significant inconsistency in the global and local levels tests (Supplementary Figures 20, 21). The network geometry for ICU mortality is shown in Supplementary Figure 22.
Intensive care unit stay
Thirteen trials reported the length of ICU stay (3, 13, 15, 18, 19, 28–30, 32, 33, 35, 37, 38). The network plot is shown in Supplementary Figure 23. There was no evidence for the superiority of one particular respiratory support method because all the CIs contained the null value (Figure 3D). HFNC ranked best among the four methods (SUCRA 69.7) (Figure 4D). The quality of evidence for ICU stay assessed by NMA was low (Table 2D). There was no significant difference in publication bias (Supplementary Figure 24). All the network comparisons were imprecise (Figure 3D). The inconsistency test at the global and local levels indicated no significant difference (Supplementary Figures 25, 26).
Length of hospital stay
Length of hospital stay was reported in nine trials (3, 13, 15, 18, 19, 28–31). The network geometry is shown in Supplementary Figure 27. The network estimates provided low-quality evidence of no difference in LOS among the four methods (Figure 3E). Figure 4E suggested that HFNC was the most effective method to shorten LOS (SUCRA 69.9). No significant publication bias was detected (Supplementary Figure 28). All the network comparisons were subject to imprecision (Table 2E). There was no significant inconsistency in the test at global and local levels (Supplementary Figures 29, 30).
Sensitivity analysis
Two sensitivity analyses were performed for the primary outcomes, exclusively including 13 trials with sample size ≥ 50 and 15 trails with PaCO2 ≤ 45 mmHg at the end of SBT. The results revealed that the relative effectiveness of various therapies remained similar (Supplementary Table 5), and the SUCRA rankings were comparable to those of the preliminary analysis (Supplementary Figures 31, 32).
Discussion
In this study, NIV as well as HFNC, and NIV + HFNC significantly reduced reintubation rate and respiratory failure compared to COT. NIV and NIV + HFNC also lowered the risk of ICU death. Treatment rankings showed that NIV + HFNC scored highest in alleviating reintubation, respiratory failure, and ICU mortality. While HFNC ranked best in shortening ICU stay and LOS.
A multicenter RCT demonstrated that NIV + HFNC was effective in preventing reintubation compared with HFNC alone (18). NIV interspaced with HFNC breaks between NIV sessions is a strategy that combines the benefits of both methods: NIV for sustainable pressure support effect (32) and HFNC for increased comfort and easier clearance of secretions (13). As a result, NIV + HFNC can further improve gas exchange and decrease the work of breathing (WOB) (40). In this study, NIV + HFNC was found to be the best strategy for reducing reintubation rate, respiratory failure, and ICU mortality, which was consistent with the recommendation from the latest guidelines (41). In the ERS clinical practice guidelines, HFNC was recommended during NIV breaks in patients with acute hypoxemic respiratory failure to limit the need for prolonged NIV by maintaining adequate oxygenation and to increase patient comfort (41). However, a relevant NMA indicated that NIV + HFNC exhibited the potential to increase short-term mortality (42). The different conclusion may be related to the inclusion criteria. In the study by Zhou et al. (42), only part of the studies recruited patients at risk of extubation failure, and substantial heterogeneity was identified across the eligible trials. In addition, only one RCT (18) directly compared NIV + HFNC with HFNC in Zhou’s study, and the insufficient sample size may lead to the inconsistency between direct and indirect estimation.
In this study, NIV was superior to COT in terms of reintubation and respiratory failure. The high success rate may be attributed to the early application of NIV, immediately after programmed extubation, which kept the upper airway open and improved ventilation and oxygenation, thus preventing overload of respiratory muscles, the development of atelectasis, and respiratory distress (32). However, a recent meta-analysis concluded that NIV had no effect on reducing reintubation rate (43). In the above study (43), NIV was used as a treatment strategy for unplanned extubation patients in addition to a preventive strategy after scheduled extubation. And different from conventional pairwise meta-analyses that only include head-to-head comparisons, NMA can compare multiple treatments simultaneously by combining direct and indirect evidence and inform the relative effect of indirect comparison treatments, within a higher quality (44).
According to the latest ERS guidelines (41), NIV was recommended over HFNC after extubation for patients at high risk of extubation failure unless relative or absolute contraindications to NIV. In the current NMA, although HFNC was non-inferior to NIV in terms of reintubation and respiratory failure, NIV was beneficial to lower the risk of ICU death. It may be explained by the following: first, even though both methods can generate PEEP, the flow of HFNC only produces about 5–6 cmH2O PEEP throughout the respiratory cycle (45, 46). While NIV can offer different levels of PEEP according to patient’s needs. Therefore, the support effect of NIV is greater than that of HFNC. In addition, we focused on high-risk patients in the current study, such as those with underlying cardiopulmonary disease. NIV has been reported to have the greatest benefits in patients with hypercapnic respiratory failure caused by chronic obstructive pulmonary disease (COPD) (6), followed by congestive heart failure (CHF) (47). Positive pressure during inspiration reduces the WOB, and compensates for increased airway resistance. Positive pressure during expiration relieves venous return and prevents respiratory failure in patients with CHF (48). All of these effects may translate into a lower mortality among patients receiving NIV protocol. This may be another reason for the difference in mortality between the two methods.
In the current NMA, HFNC ranked first in shortening ICU stay and LOS among these oxygen treatments. This may be benefit from the fact that HFNC is more comfortable and better tolerated than oronasal mask (9). In a recent multicenter RCT by Maggiore et al., HFNC reduced the incidence of tachypnea and respiratory fatigue compared with Venturi mask, improving patient comfort (11). Although the ICU stay and LOS were comparable between the two groups in that study (11), this may be due to the use of therapeutic NIV rather than reintubation in patients with respiratory distress. In addition, patients with HFNC are not restricted by respiratory support in eating, drinking, and communication. And HFNC has a smaller contact area and well-humidified oxygen delivery, which is conducive to easy clearance of secretions and low risk of adverse effects (45). The high flow also irrigates the nasopharyngeal dead space, thus alleviating CO2 re-breathing. However, NMA estimates suggested that the 95% CI contained the null effect and these findings should be interpreted with caution.
Although early weaning from IMV after a successful SBT improves prognosis, reintubation is inevitable and significantly increases mortality (3). Therefore, it is important to choose an appropriate strategy to prevent reintubation, especially for high-risk patients. In clinical practice, NIV + HFNC could be used prophylactically after planned extubation to reduce the risk of reintubation and respiratory failure in high-risk patients. Once the patient’s vital signs are stable, HFNC alone should be applied as early as possible to shorten ICU stay and LOS.
The results of this study are useful for selecting an appropriate non-invasive oxygen therapy for post-extubation patients. There are still several limitations. First, the definition of high-risk patients lacks consistency. And the severity of the participants in each study is unknown, which may affect the certainty of NMA results. Second, we performed two sensitivity analyses to assess the robustness of NMA results. However, there were other effect modifiers, including the cause of intubation and duration of IMV. Unfortunately, no other sensitivity analyses were conducted given the limited information in the included studies. Third, only two RCTs directly compared HFNC to NIV, and the NMA effect size was mainly estimated by indirect evidence, which may lead to inaccurate evaluation of treatment effect. More studies are needed to provide a higher certainty of evidence. Finally, due to limited data, we didn’t consider the safety and economic benefits of each methods.
Conclusion
In conclusion, prophylactic use of NIV + HFNC after scheduled extubation is probably the most effective respiratory support method to prevent reintubation, respiratory failure and ICU death in high-risk patients with extubation failure. Among these strategies, HFNC performed a beneficial effect on shortening ICU stay and LOS. Considering few direct comparison studies, more relevant high-quality RCTs are needed in the future.
Data availability statement
The original contributions presented in this study are included in the article/Supplementary material, further inquiries can be directed to the corresponding author.
Author contributions
XZ participated in designing the study, performing statistical analyses, and drafting the manuscript. RW participated in designing the study, study search, and study selection. MG contributed to data extraction and quality assessment. JD participated in quality assessment and the interpretation of data. MM contributed to data extraction. SG contributed to conception, design, manuscript revision for critical intellectual content, and supervision of the study. All authors read and approved the final manuscript.
Funding
This study was supported by talent program for famous masters of Chongqing (SG).
Conflict of interest
The authors declare that the research was conducted in the absence of any commercial or financial relationships that could be construed as a potential conflict of interest.
Publisher’s note
All claims expressed in this article are solely those of the authors and do not necessarily represent those of their affiliated organizations, or those of the publisher, the editors and the reviewers. Any product that may be evaluated in this article, or claim that may be made by its manufacturer, is not guaranteed or endorsed by the publisher.
Supplementary material
The Supplementary Material for this article can be found online at: https://www.frontiersin.org/articles/10.3389/fmed.2022.1026234/full#supplementary-material
Abbreviations
AECOPD, chronic obstructive pulmonary disease with acute exacerbation; APACHE, acute physiology and chronic health evaluation; CIs, confidence intervals; COT, conventional oxygen therapy; FiO2, fraction of inspired oxygen; GRADE, grading of recommendation, assessment, development and evaluation; HFNC, high-flow nasal cannula; ICU, intensive care unit; IMV, invasive mechanical ventilation; LOS, length of stay; MDs, mean differences; NIV, non-invasive mechanical ventilation; NMA, network meta-analysis; ORs, odds ratios; PaCO2, atrial partial pressure of carbon dioxide; PRISMA, Preferred Reporting Items for Systematic Reviews and Meta-Analyses; RCT, randomized controlled trial; SBT, spontaneous breathing trial; SUCRA, surface under the cumulative ranking curve; WOB, work of breathing.
References
1. Menon N, Joffe AM, Deem S, Yanez ND, Grabinsky A, Dagal AH, et al. Occurrence and complications of tracheal reintubation in critically Ill adults. Respir Care. (2012) 57:1555–63. doi: 10.4187/respcare.01617
2. Epstein SK, Ciubotaru RL. Independent effects of etiology of failure and time to reintubation on outcome for patients failing extubation. Am J Respir Crit Care Med. (1998) 158:489–93. doi: 10.1164/ajrccm.158.2.9711045
3. Ferrer M, Sellarés J, Valencia M, Carrillo A, Gonzalez G, Badia JR, et al. Non-invasive ventilation after extubation in hypercapnic patients with chronic respiratory disorders: randomised controlled trial. Lancet. (2009) 374:1082–8. doi: 10.1016/s0140-6736(09)61038-2
4. Thille AW, Richard JC, Brochard L. The decision to extubate in the intensive care unit. Am J Respir Crit Care Med. (2013) 187:1294–302. doi: 10.1164/rccm.201208-1523CI
5. Köhler D. [Tremendous increase of home care in ventilated and tracheostomized patients – reasons, consequences, solutions]. Dtsch Med Wochenschr. (2019) 144:282–5. doi: 10.1055/a-0805-5284
6. Maggiore SM, Battilana M, Serano L, Petrini F. Ventilatory support after extubation in critically Ill patients. Lancet Respir Med. (2018) 6:948–62. doi: 10.1016/s2213-2600(18)30375-8
8. Quintard H, l’Her E, Pottecher J, Adnet F, Constantin JM, De Jong A, et al. Experts’ guidelines of intubation and extubation of the Icu patient of French society of anaesthesia and intensive care medicine (Sfar) and French-speaking intensive care society (Srlf) : in collaboration with the pediatric association of French-speaking anaesthetists and intensivists (Adarpef), French-speaking group of intensive care and paediatric emergencies (Gfrup) and intensive care physiotherapy society (Skr). Ann Intensive Care. (2019) 9:13. doi: 10.1186/s13613-019-0483-1
9. Maggiore SM, Idone FA, Vaschetto R, Festa R, Cataldo A, Antonicelli F, et al. Nasal high-flow versus venturi mask oxygen therapy after extubation. Effects on oxygenation, comfort, and clinical outcome. Am J Respir Crit Care Med. (2014) 190:282–8. doi: 10.1164/rccm.201402-0364OC
10. Ritchie JE, Williams AB, Gerard C, Hockey H. Evaluation of a humidified nasal high-flow oxygen system, using oxygraphy, capnography and measurement of upper airway pressures. Anaesth Intensive Care. (2011) 39:1103–10. doi: 10.1177/0310057x1103900620
11. Maggiore SM, Jaber S, Grieco DL, Mancebo J, Zakynthinos S, Demoule A, et al. High-flow versus venturimask oxygen therapy to prevent re-intubation in hypoxemic patients after extubation: a multicenter, randomized clinical trial. Am J Respir Crit Care Med. (2022) [Epub ahead of print]. doi: 10.1164/rccm.202201-0065OC
12. Mauri T, Turrini C, Eronia N, Grasselli G, Volta CA, Bellani G, et al. Physiologic effects of high-flow nasal cannula in acute hypoxemic respiratory failure. Am J Respir Crit Care Med. (2017) 195:1207–15. doi: 10.1164/rccm.201605-0916OC
13. Hernández G, Vaquero C, Colinas L, Cuena R, González P, Canabal A, et al. Effect of postextubation high-flow nasal cannula VS noninvasive ventilation on reintubation and postextubation respiratory failure in high-risk patients: a randomized clinical trial. JAMA. (2016) 316:1565–74. doi: 10.1001/jama.2016.14194
14. Frat JP, Thille AW, Mercat A, Girault C, Ragot S, Perbet S, et al. High-flow oxygen through nasal cannula in acute hypoxemic respiratory failure. N Engl J Med. (2015) 372:2185–96. doi: 10.1056/NEJMoa1503326
15. Cho JY, Kim HS, Kang H, Kim SH, Choe KH, Lee KM, et al. Comparison of postextubation outcomes associated with high-flow nasal cannula VS. Conventional oxygen therapy in patients at high risk of reintubation: a randomized clinical trial. J Korean Med Sci. (2020) 35:e194. doi: 10.3346/jkms.2020.35.e194
16. Ni YN, Luo J, Yu H, Liu D, Ni Z, Cheng J, et al. Can high-flow nasal cannula reduce the rate of endotracheal intubation in adult patients with acute respiratory failure compared with conventional oxygen therapy and noninvasive positive pressure ventilation?: a systematic review and meta-analysis. Chest. (2017) 151:764–75. doi: 10.1016/j.chest.2017.01.004
17. Xu Z, Li Y, Zhou J, Li X, Huang Y, Liu X, et al. High-flow nasal cannula in adults with acute respiratory failure and after extubation: a systematic review and meta-analysis. Respir Res. (2018) 19:202.
18. Thille AW, Muller G, Gacouin A, Coudroy R, Decavèle M, Sonneville R, et al. Effect of postextubation high-flow nasal oxygen with noninvasive ventilation VS high-flow nasal oxygen alone on reintubation among patients at high risk of extubation failure: a randomized clinical trial. JAMA. (2019) 322:1465–75. doi: 10.1001/jama.2019.14901
19. Thille AW, Coudroy R, Nay MA, Gacouin A, Decavèle M, Sonneville R, et al. Beneficial effects of noninvasive ventilation after extubation in obese or overweight patients: a post hoc analysis of a randomized clinical trial. Am J Respir Crit Care Med. (2022) 205:440–9. doi: 10.1164/rccm.202106-1452OC
20. Hutton B, Salanti G, Caldwell DM, Chaimani A, Schmid CH, Cameron C, et al. The prisma extension statement for reporting of systematic reviews incorporating network meta-analyses of health care interventions: checklist and explanations. Ann Intern Med. (2015) 162:777–84. doi: 10.7326/m14-2385
21. Rothaar RC, Epstein SK. Extubation failure: magnitude of the problem, impact on outcomes, and prevention. Curr Opin Crit Care. (2003) 9:59–66. doi: 10.1097/00075198-200302000-00011
22. Higgins JP, Altman DG, Gøtzsche PC, Jüni P, Moher D, Oxman AD, et al. The Cochrane collaboration’s tool for assessing risk of bias in randomised trials. BMJ. (2011) 343:d5928. doi: 10.1136/bmj.d5928
23. Higgins JP, Jackson D, Barrett JK, Lu G, Ades AE, White IR. Consistency and inconsistency in network meta-analysis: concepts and models for multi-arm studies. Res Synth Methods. (2012) 3:98–110. doi: 10.1002/jrsm.1044
24. Chaimani A, Higgins JP, Mavridis D, Spyridonos P, Salanti G. Graphical tools for network meta-analysis in stata. PLoS One. (2013) 8:e76654. doi: 10.1371/journal.pone.0076654
25. Salanti G, Ades AE, Ioannidis JP. Graphical methods and numerical summaries for presenting results from multiple-treatment meta-analysis: an overview and tutorial. J Clin Epidemiol. (2011) 64:163–71. doi: 10.1016/j.jclinepi.2010.03.016
26. Puhan MA, Schünemann HJ, Murad MH, Li T, Brignardello-Petersen R, Singh JA, et al. A grade working group approach for rating the quality of treatment effect estimates from network meta-analysis. BMJ. (2014) 349:g5630. doi: 10.1136/bmj.g5630
27. Salanti G, Del Giovane C, Chaimani A, Caldwell DM, Higgins JP. Evaluating the quality of evidence from a network meta-analysis. PLoS One. (2014) 9:e99682. doi: 10.1371/journal.pone.0099682
28. Ferrer M, Valencia M, Nicolas JM, Bernadich O, Badia JR, Torres A. Early noninvasive ventilation averts extubation failure in patients at risk: a randomized trial. Am J Respir Crit Care Med. (2006) 173:164–70. doi: 10.1164/rccm.200505-718OC
29. Fernandez R, Subira C, Frutos-Vivar F, Rialp G, Laborda C, Masclans JR, et al. High-flow nasal cannula to prevent postextubation respiratory failure in high-risk non-hypercapnic patients: a randomized multicenter trial. Ann Intensive Care. (2017) 7:47. doi: 10.1186/s13613-017-0270-9
30. Nava S, Gregoretti C, Fanfulla F, Squadrone E, Grassi M, Carlucci A, et al. Noninvasive ventilation to prevent respiratory failure after extubation in high-risk patients. Crit Care Med. (2005) 33:2465–70. doi: 10.1097/01.ccm.0000186416.44752.72
31. Khilnani GC, Galle AD, Hadda V, Sharma SK. Non-invasive ventilation after extubation in patients with chronic obstructive airways disease: a randomised controlled trial. Anaesth Intensive Care. (2011) 39:217–23. doi: 10.1177/0310057x1103900210
32. Ornico SR, Lobo SM, Sanches HS, Deberaldini M, Tófoli LT, Vidal AM, et al. Noninvasive ventilation immediately after extubation improves weaning outcome after acute respiratory failure: a randomized controlled trial. Crit Care. (2013) 17:R39. doi: 10.1186/cc12549
33. Vargas F, Clavel M, Sanchez-Verlan P, Garnier S, Boyer A, Bui HN, et al. Intermittent noninvasive ventilation after extubation in patients with chronic respiratory disorders: a multicenter randomized controlled trial (Vhyper). Intensive Care Med. (2017) 43:1626–36. doi: 10.1007/s00134-017-4785-1
34. Song HZ, Gu JX, Xiu HQ, Cui W, Zhang GS. The value of high-flow nasal cannula oxygen therapy after extubation in patients with acute respiratory failure. Clinics (Sao Paulo). (2017) 72:562–7.
35. Jing G, Li J, Hao D, Wang T, Sun Y, Tian H, et al. Comparison of high flow nasal cannula with noninvasive ventilation in chronic obstructive pulmonary disease patients with hypercapnia in preventing postextubation respiratory failure: a pilot randomized controlled trial. Res Nurs Health. (2019) 42:217–25. doi: 10.1002/nur.21942
36. Xu SX, Wu CS, Liu SY, Lu X. High-flow nasal cannula oxygen therapy and noninvasive ventilation for preventing extubation failure during weaning from mechanical ventilation assessed by lung ultrasound score: a single-center randomized study. World J Emerg Med. (2021) 12:274–80. doi: 10.5847/wjem.j.1920-8642.2021.04.004
37. Mohamed KAE, Abdalla MH. Role of non invasive ventilation in limiting re-intubation after planned extubation. Egypt J Chest Dis Tuberc. (2013) 62:669–74. doi: 10.1016/j.ejcdt.2013.08.010
38. Hu TY, Lee CH, Cheng KH, Tan MC, Hua HF, Kuo LK. Effect of high-flow nasal oxygen vs. conventional oxygen therapy on extubation outcomes and physiologic changes for patients with high risk of extubation failure in the medical ICU: a tertiary center, randomized, controlled trial. Int J Gerontol. (2020) 14:36–41. doi: 10.6890/IJGE.202002_14(1).0008
39. Adıyeke E, Ozgultekin A, Turan G, Iskender A, Canpolat G, Pektaş A, et al. Non-invasive mechanical ventilation after the successful weaning: a comparison with the venturi mask. Braz J Anesthesiol. (2016) 66:572–6. doi: 10.1016/j.bjane.2014.11.008
40. Frat JP, Brugiere B, Ragot S, Chatellier D, Veinstein A, Goudet V, et al. Sequential application of oxygen therapy via high-flow nasal cannula and noninvasive ventilation in acute respiratory failure: an observational pilot study. Respir Care. (2015) 60:170–8. doi: 10.4187/respcare.03075
41. Oczkowski S, Ergan B, Bos L, Chatwin M, Ferrer M, Gregoretti C, et al. ERS clinical practice guidelines: high-flow nasal cannula in acute respiratory failure. Eur Respir J. (2022) 59:2101574. doi: 10.1183/13993003.01574-2021
42. Zhou X, Yao S, Dong P, Chen B, Xu Z, Wang H. Preventive use of respiratory support after scheduled extubation in critically ill medical patients-a network meta-analysis of randomized controlled trials. Crit Care. (2020) 24:370. doi: 10.1186/s13054-020-03090-3
43. Maitra S, Bhattacharjee S, Som A. Noninvasive ventilation and oxygen therapy after extubation in patients with acute respiratory failure: a meta-analysis of randomized controlled trials. Indian J Crit Care Med. (2019) 23:414–22.
44. Rouse B, Chaimani A, Li T. Network meta-analysis: an introduction for clinicians. Intern Emerg Med. (2017) 12:103–11.
45. Nishimura M. High-flow nasal cannula oxygen therapy in adults: physiological benefits, indication, clinical benefits, and adverse effects. Respir Care. (2016) 61:529–41. doi: 10.4187/respcare.04577
46. Natalini D, Grieco DL, Santantonio MT, Mincione L, Toni F, Anzellotti GM, et al. Physiological effects of high-flow oxygen in tracheostomized patients. Ann Intensive Care. (2019) 9:114.
47. Masip J, Roque M, Sánchez B, Fernández R, Subirana M, Expósito JA. Noninvasive ventilation in acute cardiogenic pulmonary edema: systematic review and meta-analysis. JAMA. (2005) 294:3124–30. doi: 10.1001/jama.294.24.3124
Keywords: high-flow nasal catheter, extubation failure, reintubation, respiratory failure, non-invasive ventilation, high-risk patients, network meta-analysis
Citation: Zheng X, Wang R, Giri M, Duan J, Ma M and Guo S (2022) Efficacy of preventive use of oxygen therapy after planned extubation in high-risk patients with extubation failure: A network meta-analysis of randomized controlled trials. Front. Med. 9:1026234. doi: 10.3389/fmed.2022.1026234
Received: 23 August 2022; Accepted: 29 September 2022;
Published: 13 October 2022.
Edited by:
Savino Spadaro, University of Ferrara, ItalyReviewed by:
Federico Longhini, Magna Græcia University, ItalyArtem N. Kuzovlev, Research Institute of General Resuscitation V.A. Negovsky, Russia
Copyright © 2022 Zheng, Wang, Giri, Duan, Ma and Guo. This is an open-access article distributed under the terms of the Creative Commons Attribution License (CC BY). The use, distribution or reproduction in other forums is permitted, provided the original author(s) and the copyright owner(s) are credited and that the original publication in this journal is cited, in accordance with accepted academic practice. No use, distribution or reproduction is permitted which does not comply with these terms.
*Correspondence: Shuliang Guo, guosl999@sina.com