- 1Department of Dermatology, Yueyang Hospital of Integrated Traditional Chinese and Western Medicine, Shanghai University of Traditional Chinese Medicine, Shanghai, China
- 2Institute of Dermatology, Shanghai Academy of Traditional Chinese Medicine, Shanghai, China
Psoriasis is a chronic inflammatory skin disease with an underlying autoimmune pathogenesis that has brought great distress to patients. Current treatment options include topical therapy, systemic therapy, and phototherapy. By disrupting the stratum corneum, nanocarriers have unique advantages in allowing drug carriers to be tailored to achieve targeted drug delivery, improve efficacy, and minimize adverse effects. Furthermore, despite their limited success in market translatability, nanocarriers have been extensively studied for psoriasis, owing to their excellent preclinical results. As topical formulations are the first line of treatment, utilize the safest route, and facilitate a targeted approach, this study, we specifically describes the management of psoriasis using topical agents in conjunction with novel drug delivery systems. The characteristics, advantages, weaknesses, and mechanisms of individual nanocarriers, when applied as topical anti-psoriatic agents, were reviewed to distinguish each nanocarrier.
1 Introduction
Psoriasis is a recurrent inflammatory autoimmune skin disorder that ranges in severity from highly inflammatory red erythema patches supported by silvery scales to the entire skin surface, exacerbating itching, irritation, and body pain (1). The etiology of psoriasis involves a combination of hereditary and environmental factors that lead to histological changes in the skin (2, 3). At present, the treatment and management of psoriasis mainly involve topical agents, phototherapy, and systemic therapy. Topical agents are generally recommended as first-line treatment for mild psoriasis. However, for more severe conditions, phototherapy or systemic therapy is more suitable than topical agents (4). Although these methods can lessen the symptoms and signs of psoriasis, they all have limitations and are unable to completely cure the disease (5–7).
Therefore, recent research has attempted to design and develop a novel drug delivery system to compensate for the deficiencies of traditional drug delivery systems. The nanotechnology-based delivery systems have shown great advantages in increasing drug concentration at the targeted site, drug encapsulation efficiency, and skin penetration, thereby providing better efficiency, reduced side effects, and improved patient compliance. This review examines the various challenges in treating psoriasis, contemporary research into new drug delivery systems, and future potential for therapy.
2 Core pathogenesis: Massive proliferation of keratinocytes
The main clinical manifestation of psoriasis is evident in the outermost layer of the skin, which comprises keratinocytes. Keratinocytes were initially thought to be the primary cause of infection, with the immune system resulting in an excessive proliferation of skin cells, leading to immune-mediated disorders (8). Psoriasis occurs when skin cells accumulate in the epidermis instead of shedding, causing visible lesions (9). Additionally, the recognition of antimicrobial peptides is closely related to dendritic cell (DC) activation. LL-37, the most studied psoriasis-associated antimicrobial peptide, binds to DNA and stimulates toll-like receptors in plasmacytoid DC (10). Subsequently, plasmacytoid DC activation produces type I IFN (IFN-α and IFN-β), which further promotes the phenotypic maturation of myeloid DC. Th1 cells then differentiate and together with Th1 cytokines secrete a mass of inflammatory cytokines, of which the most pivotal are IL-12 and IL-23 in psoriasis. Subsequently, IL12 stimulates Th1 to produce IFN and TNF-α and IL23 stimulates Th17 to produce IL17 and IL22 (11–13).
The maintenance stage of psoriatic inflammation is the proliferation of keratinocytes, mainly involved in four aspects. The most important is the activation of adaptive immune responses by T-cell subsets, represented here by the involvement of Th17 cytokines, such as IL-23, IL-22, and IL-17 (14), followed by the inflammatory milieu activating keratinocyte proliferation through IL-17, TNF-α, and IFN-γ (10). However, LL37 and DNA greatly increase the type I IFN production. Moreover, with the help of cytokine (IL-1, IL-6, and TNF-α), chemokine, and AMP secretion, keratinocytes can also participate actively in the inflammatory cascade.
Previous studies have shown that the TNF-α and IL-23/IL-17A axis is the main driver of psoriasis, and the interaction between IL-17A and keratinocytes is a key issue in developing psoriasis. This review aimed to highlight the important role of keratinocytes in the pathogenesis of psoriasis because patients with psoriasis are typically characterized by epidermal hyperproliferation, which aggravates the burden of traditional drug administration. Furthermore, we will present the unique advantages of the novel drug delivery system by breaking through the thick stratum corneum to explore the treatment of psoriasis patients (Figure 1).
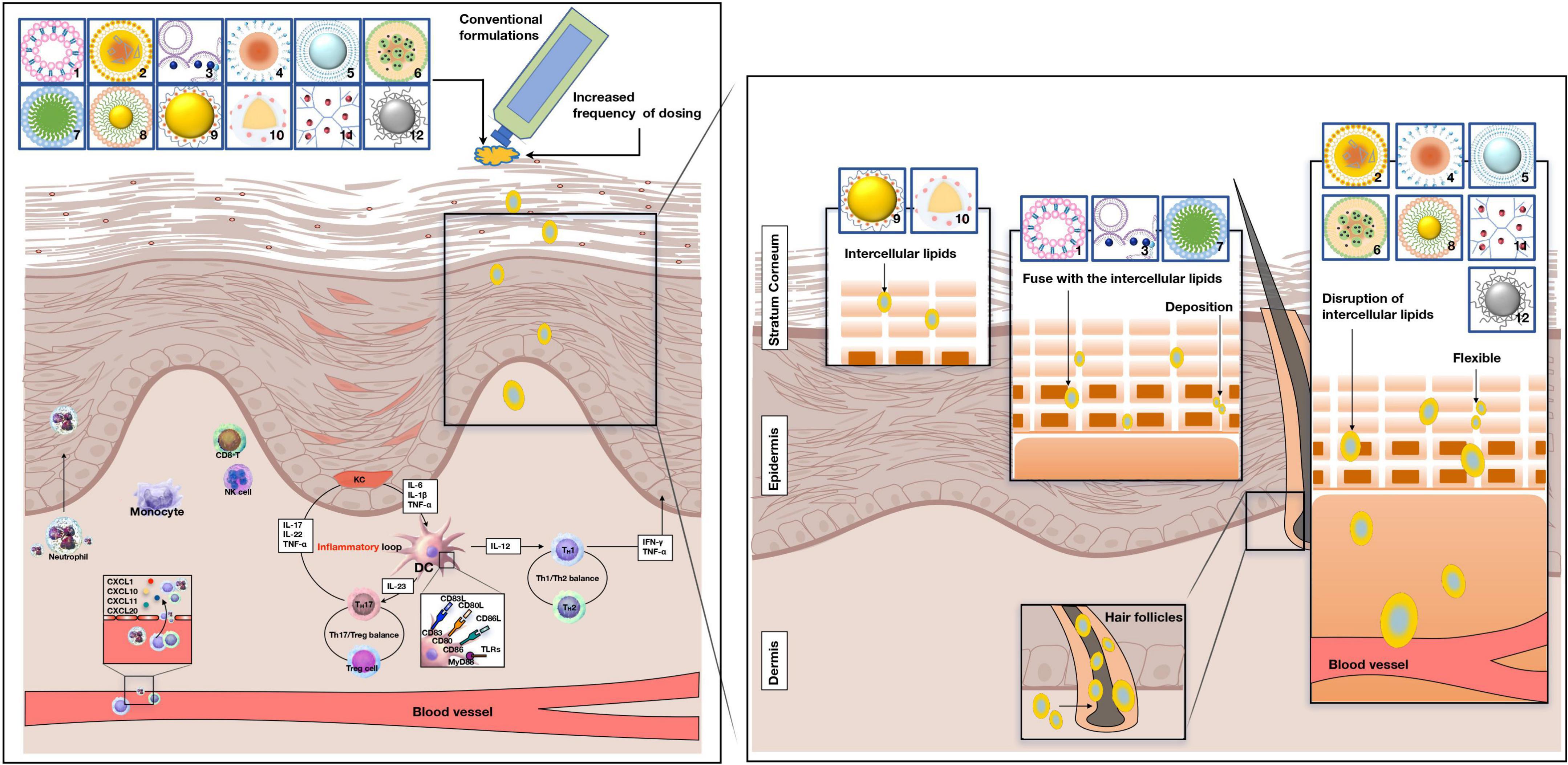
Figure 1. Potential penetration routes of novel drug systems and conventional formulations. Pathogenesis of psoriasis is related to the KC/DC/T loop. 1: liposomes, 2: nanostructured lipid carriers, 3: niosomes, 4: nanoemulsions, 5: ethosomes, 6: solid lipid nanoparticles, 7: micelles, 8: gold particle, 9: nanocapsules, 10: nanosphere, 11: dendrimers, 12: sliver particle. pDC, plasmacytoid dendritic cells; DC, dendritic cells; KC, keratinocyte. NK -cell, natural killer cell; IL-17A, interleukin-17A; IL-17 F, interleukin-17 F; IL-17, interleukin-17; IL-12, interleukin-12. TNF-α, tumor necrosis factor-α; IL-6, interleukin-6; IL-1β, interleukin-1β; IFN-γ, interferon-γ; Th1 cell, T helper 1 cells; Th2 cell, T helper 2 cell; Treg cell, T regulatory cells.
3 Treatment challenges: Difficulties in penetrating the horny psoriatic layer
The function of the skin in psoriasis and how the pathological condition of the skin minimizes or exceeds the barrier function of the cuticle and epidermis have been a concern for researchers for many years. An experimental study in Japan reported that psoriatic skin has lower water content, free fatty acids, and natural moisturizing factors than normal skin, while the sebum content remains unaffected (15). The main concern regarding an effective response to dermatological agents is their need to reach the targeted site and remain at effective concentrations for a period. Abnormal thickening of the stratum corneum (SC) results from the hyperproliferation of keratinocytes and as a direct result of hindered penetration of the skin; topical drug delivery to psoriatic skin presents a difficult challenge for conventional formulations.
4 Conventional management for psoriasis: The multi-organ pitfalls remains
Treatment choices for psoriasis management comprise three main approaches: topical treatment, systemic treatment, and phototherapy (Figure 2A). Topical therapy is generally considered the first-line treatment for mild-to-moderate localized psoriasis and can be used with phototherapy or systemic therapy for moderate-to-severe psoriasis. Topical agents used include coal tar, dithranol (DIT), and retinoids, as well as tacrolimus (TAC), corticosteroids, salicylic acid (SA), tazarotene, and vitamin D analogs. However, owing to the lack of continuous elimination of the lesion, this may relapse and increase the psychological burden of the patient (16, 17).
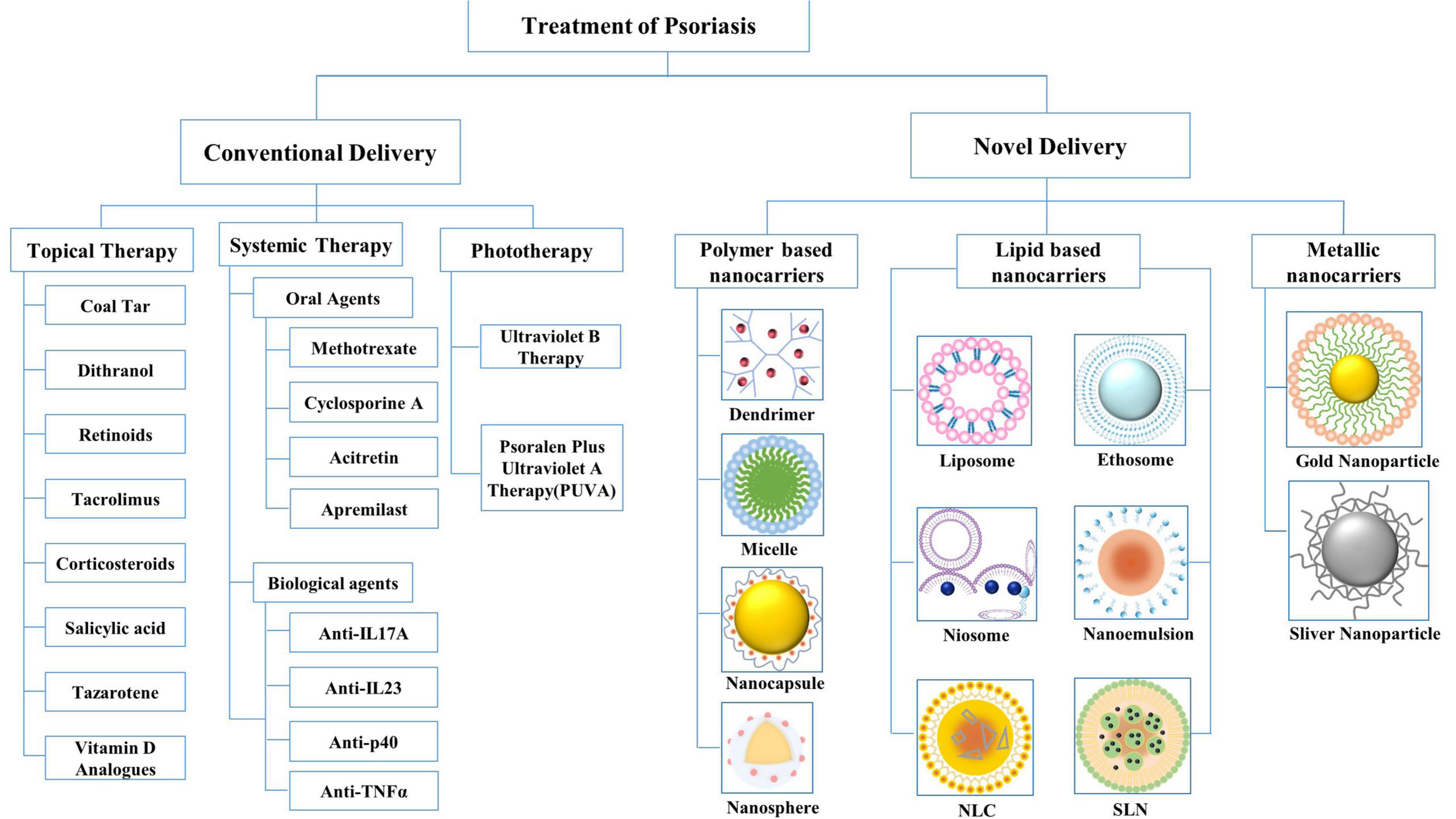
Figure 2. Conventional drug delivery systems and novel drug delivery systems for the treatment of psoriasis. NLC, nanostructured lipid carriers; SLN, solid lipid nanoparticles.
Oral retinoids, methotrexate (MTX), cyclosporine (CYC), and fumaric acid esters are utilized in the treatment of psoriasis. Organ toxicity may occur after systematic treatment with these drugs, such as the fact that MTX is toxic to the liver and cyclosporine is nephrotoxic (18, 19). Biological agents, specifically anti IL-17A, anti-IL-23, and anti p-40 agents, have been shown to be effective against psoriasis in recent years. However, as anti-IL17A agents are associated with mycosis and neutropenia, anti-TNF-α treatments are not recommended for patients with heart problems (20, 21).
Compared with biological agents, apremilast (a small-molecule inhibitor of phosphodiesterase 4) acts at an earlier point in the inflammatory cascade, resulting in broad regulation of multiple inflammatory mediators (22). As for the conventional phototherapies, narrow-band UVB and photochemotherapy, such as PUVA (psoralen plus UVA), are used to a lower extent. Therefore, current research aims to apply novel nanocarriers to safely and effectively delivery anti-psoriatic drugs. Thus, we decided to restrict this study to topical approaches.
5 Promising strategies: Advanced topical nanocarrier systems
Nanoparticles offer advantageous properties as alternatives to conventional formulations. Therefore, by adding a targeting moiety, controlling the size of the vector, and combining hydrophobic drugs in hydrophilic carriers, the carrier can be customized. An ideal drug carrier should cross the thick stratum corneum and selectively recognize target cells by surface ligands, and most importantly, the drug-ligand complex must be stable in the biological environment and the drug carrier must be biodegradable and non-toxic (23). Novel drug delivery systems can be classified into three broad categories based on their main components: polymer-based, lipid-based, and metallic nanocarriers (Figure 2B). In this review, we discuss the above-mentioned nanocarriers, which have been proven effective in clinical and experimental studies in the past few years. The advantages and disadvantages of nanocarriers for psoriasis treatment are summarized in Table 1.
5.1 Mechanism of action of nanostructures
Lipid-based nanocarriers improve skin surface adhesion, contact with SC, and boost the penetration efficiency of biologically active ingredients into the skin (24). Polymer-based nanocarriers provide the facility of enhanced concentration gradient at the skin surface and offer tunable physical or chemical properties that can achieve sustained or controlled release of the incorporated drug at the delivery site (25). Metallic nanocarriers are conjugated to diverse targeted peptides for functional nanoparticles to penetrate biological tissues successfully (26). The potential penetration routes of the novel drug delivery system are shown in Figure 1.
5.2 Lipid based nanocarriers
Lipid-based nanocarriers comprise physiological lipids whose structures are safe and non-toxic. These mainly include the following six types: liposomes, ethosomes, niosomes, nanoemulsions (NEs), solid lipid nanoparticles (SLNs), and nanostructured lipidic carriers (NLCs).
5.2.1 Liposomes
Liposomes are simple, self-assembling spherical vesicles consisting of one or more lipid bilayers arranged in concentric circles and enclosing equal volumes of aqueous compartments, similar to bilayer membranes of living cells (27). Liposomes are widely used as drug transporters for lipophilic and hydrophilic molecules because of their amphiphilic nature and bilayer structure (28). To evaluate the anti-psoriasis effects of cationic liposomes, dioleoyl-3-trimethylammonium propane (DOTAP) was used to prepare the CYC-loaded liposomes. The results demonstrated a strong affinity for anionic skin membranes, resulting in improved efficacy in an imiquimod-induced psoriatic plaque model (29). Another study showed that peptide-modified curcumin-loaded liposomes assisted in skin permeation and drug retention of liposomes. Consequently, peptide-modified curcumin-loaded liposomes significantly improved psoriatic lesions and decreased epidermal thickness (30). These findings proved that various modifications of liposomes could improve skin permeability. However, there is no definitive evidence of its effectiveness in patients with psoriasis, as it seems to accumulate a majority of drugs in the upper layers of the SC. One study addressed this deficiency by designing capsaicin and anti-TNF-α small interfering RNA-encapsulated cyclic cationic head lipid-polymer hybrid nanocarriers. Based on in vitro skin distribution studies, this complex effectively delivered FITC-siRNA up to 360 μm skin depth, suggesting that anti-TNF-α small interfering RNA and capsaicin can be delivered into the deeper dermal milieu with the cooperation of novel cationic lipid-polymer hybrid nanoparticles (31).
5.2.2 Ethosomes
Ethosomes are novel ultra-deformable nanovesicles with high-quality alcohol in water, comprising phosphides that are generally used at a concentration of 0.5–10% (32). Interactions with the polar head group of lipids enable fluidity of the skin tissue structure lipid bilayer, which triggers drug release into deeper layers of the epidermis. One study compared the safety and clinical efficacy of liposomes and ethosomes in psoriasis patients. After treatment, the mean psoriasis area and severity index change for liposomes and ethosomes were −68.66% and −81.84%, respectively, indicating that ethosomal preparations were more effective than liposomes (33). In another study, ethosomal gel loaded with MTX-incorporated SA was assessed for its anti-psoriatic potential in imiquimod-induced rice. Based on the results obtained from continuous monitoring for 24 h, MTX-SA-ethosome gel releases MTX slowly over an extended period, with >30% drug retention detected in the skin (34). These studies have confirmed the synergistic effect of incorporating alcohol into liposomes. However, the risk of allergies may concomitantly increase with an increase in the amount of ethanol. Along with safety concerns, the ethanolic core of ethosomes may evaporate at relatively high temperatures in the local inflammatory area of psoriatic skin. Both issues require further pharmacodynamic investigation and clarification of their clinical efficacy.
5.2.3 Niosomes
Niosomes self-assemble from amphiphilic non-ionic surfactants with cholesterol and are oriented into a bilayer structure with a neutral total charge (35). The presence of non-ionic surfactants modifies the horny layer of psoriasis into looser, more permeable tissue; therefore, the residence time and local concentration of the drug in the SC and epidermis can be improved (36). Niosomes containing MTX in chitosan gels had maximum penetrability, with a significantly reduced total score, from 6.2378 ± 1.4857 to 2.0023 ± 0.1371, compared with commercially available gels (37). This study was combined with other experimental results, which demonstrated that MTX-encapsulated noisomes exhibited noticeable changes in ameliorating skin lesions, decreasing spleen index and epidermal thickness, and downregulating the mRNA expression of proinflammatory cytokines (38). In addition, celastrol niosomes offer an enhanced ex vivo osmotic profile of drug deposition in viable skin layers, and a significantly higher degree of keratosis, reduction in drug activity, and epidermal thickness were achieved (39). In the literature review, we noted that the composition, size, and surface charge of niosomes play important roles in determining the permeability, stability, and retention of drugs in the skin layer.
5.2.4 Nanoemulsions
Nanoemulsions are formed by dispersing two immiscible liquids (frequently water and oil) with droplet sizes ranging from 20 to 500 nm (40). Three types of NEs have been well-studied for particular applications: oil-in-water (o/w) emulsions, water-in-oil (w/o) emulsions, and bicontinuous NEs. The effectiveness of transdermal drug absorption of w/o NEs is promoted via skin appendages by increasing the solubility of drugs and producing a larger concentration gradient between NEs and the skin (41). Similarly, to promote the permeation of CYC into the SC, one study introduced a nanoemulsion for transporting cyclosporine as an anti-inflammatory drug with the addition of nutmeg and virgin coconut oil mixture. The emulsified CYC confirmed not only an increase in drug loading but also increased skin hydration in wholesome volunteers due to the greater content of fatty acids in the combined oil solution (42). Moreover, some specifically designed NE systems have been recommended as moisturizers for daily skin care of patients with psoriasis. Therefore, scholars developed o/w NEs using rice bran oil, and the results confirmed that elevated NEs maintained normal skin pH and hydration (43). However, the low viscosity of NEs may also be overcome through a hydrogel thickening process, limiting its topical application.
5.2.5 Solid lipid nanoparticles
Unlike conventional liposomes, SLNs are solid lipids with an average diameter of 10–1000 nm that remain solid at room and body temperatures (44). There are two main factors that affect drug release characteristics: drug deposition patterns and the melting points of lipids. The beneficial effects of SLNs can be summarized as the “occlusion effect,” which occurs because of the formation of an occlusive hydrophobic film on top of the SC, resulting in better hydration and transcutaneous penetration (35). One study verified that betamethasone dipropionate (BD)- calcipotriol (CT)-loaded SLNs extensively increased the dermal absorption of BD/CT and delayed the abrupt growth of keratinocytes. Consistently, an in vivo mouse tail model demonstrated that the administration of BD-CT-SLNs reduced epidermal thickness and increased melanocyte count without side effects compared to Daivobet™, which can verify the anti-psoriatic activity of BD-CT-loaded SLNs (45). Similar observations were reported when SLNs were applied as a topical solution containing a combination of MTX and etanercept (46). These results show that SLNs have great potential to limit dose-dependent toxicity by reducing the systemic exposure of the encapsulated drug.
5.2.6 Nanostructured lipidic carriers
Nanostructured lipidic carriers were designed to fill in the gap between SLNs regarding drug solubility and leakage by replacing up to 30% of the solid lipid mass of SLNs with liquid lipids. Although a certain amount of liquid lipids is replenished, the output of NLCs by mixing remains in a solid form at room or body temperature (47). When incorporating liquid lipids (an imperfect and less-ordered crystalline structure of NLCs) was created, displaying a considerable “occlusion effect,” which is appropriate for topical administration. A comparative study evaluated the potential use of carbomer gel-bearing methotrexate-loaded NLC carriers for topical application of methotrexate in contrast to SLNs. The skin drug deposition study indicated the greatest deposition of drug-enriched NLC5 hydrogel (28.8%), in contrast to plain drug-enriched hydrogel (11.4%) and drug-enriched SLN hydrogel (18.6%) (48). Fluorescence microscopy showed that these lipid-based systems have a localization effect in deeper skin regions. Another randomized controlled trial further confirmed the anti-psoriasis effects of NLCs by formulating and characterizing acitretin (ACT) NLCs. A notably higher deposition of acitretin was discovered in human cadaver skin from ACTNLC gel (81.38 ± 1.23%) than from ACT plain gel (47.28 ± 1.02%) (49).
5.3 Polymer-based nanocarriers
Polymer-based nanocarriers are colloidal structures composed of macromolecules that contain natural or synthetic polymers as the chief excipients. Compared with lipid-based nanocarriers, polymers tend to accumulate in the dermis layer, suggesting that the former is suitable for topical treatment, whereas the latter is more appropriate for transdermal treatment (50). Four types of polymer-based nanocarriers, namely, micelles, dendrimers, nanospheres, and nanocapsules, are discussed in the next section.
5.3.1 Micelles
Micelles are spherical structures with diameters less than 100 nm, formed by the self-assembly of amphiphilic molecules in an aqueous system through a hydrophobic effect (51). In the micelle structure, the hydrophobic core carries lipophilic drugs and the hydrophilic shell carries hydrophilic drugs, which are suitable for drug administration. To enhance the cutaneous bioavailability of TAC, TAC-loaded polymeric micelles were formulated using biodegradable and biocompatible methoxy-poly (ethylene glycol)-dihexyl-substituted polylactide diblock copolymer. The results showed that compared with Protopic, TAC deposition in skin was significantly increased by this formula (0.1% W/W; TAC ointment, 1.50 ± 0.59 and 0.47 ± 0.20 μg/cm, respectively) (52). In a similar way, silibinin-loaded polymeric micelles were prepared in another study. The average particle size of the optimized samples was 18.3 ± 2.1 nm, the encapsulation efficiency was 75.8 ± 5.8%, and the release time of silybin was prolonged. Moreover, silibinin permeation through psoriatic skin after 48 h of treatment with polymeric micelles and the aqueous control was 80.35 and 92.6, respectively (53). These micelles have been designed for targeted drug delivery because of their easy structural deformation under the influence of pH, temperature, or reduction-oxidation reactions in vivo. Therefore, micelles are more suitable for local administration or in combination with other polymer materials when controlled drug release is required (54).
5.3.2 Dendrimers
Dendrimers are highly-branched and nano-sized micromolecules with a controlled, globular, reactive three-dimensional structure. Drug molecules form drug-dendritic molecular conjugates through non-covalent interactions or covalently linked functional groups (55). The three dendrimer types were peptide dendrimers, glycodendrimers, and lysine-core dendrimers. One study explored the potential of DIT-loaded polypropylene imine (PPI) dendrimers and their characterization was performed using spectroscopy and transmission electron microscopy. Confocal laser scanning-microscopy images and skin penetration studies demonstrated that PPI can be utilized to enhance the local bioavailability of molecules in a controlled manner. PPI enhances transdermal absorption in a controlled manner, while DIT accumulation in the skin via dendritic molecular vectors may help optimize drug targeting to the epidermis and dermis (56). Another study focused on the enhancement of transdermal delivery of 8-methoxyp-soralen (8-MOP) DOTAP by the dendritic molecules G3 and G4 of polyamidoamine (PAMAM). Another study focused on the enhancement effect of PAMAM dendrimers G3 and G4 on transdermal delivery of 8-MOP. Enhanced in vivo 8-MOP skin penetration into the deeper layers of the skin was obtained, and G4 PAMAM dendrimers provided a better penetration enhancement effect for 8-MOP compared to G3 PAMAM (57). Moreover, one study compared the PAMAM dendrimers’ suitability with DOTAP liposomes for topical delivery of siRNA against TNF-α. The results showed that phenotypic and histopathological features were improved, and IL-6, TNF-α, IL-17, and IL-22 levels decreased in the dendritic plexus and liposome treatment groups compared to the imiquimod group (58).
5.3.3 Nanocapsule
Nanocapsule is a type of nanoparticle that consists of one or more active components (core) and a protective matrix (shell), with the core consisting of a liquid suspension containing the medicine and the polymer constituting the shell (59). One study created tretinoin-loaded nanocapsules and assessed the influence of nanoencapsulation on the photostability of tretinoin from different perspectives. Inferring that nanocapsules are a suitable carrier for tretinoin to treat psoriasis, photodegradation experiments showed a 2-fold increase in drug stability in a methanolic solution, with an increased half-life between 85 and 100 min (60). In another experimental investigation, the stability of DIT-loaded lipid-core nanocapsules was compared to that of medication-free solutions by photodegradation against UVA light. It showed greater stability (half-life times of approximately 4 and 1 h for the DIT-loaded lipid-core nanocapsules), and an irritation test was conducted to evaluate the safety of the formulations; it was proved that the drug’s toxicity was reduced due to nanoencapsulation (IS = 0) compared to the effects observed with DIT dispersion (10.43 ± 0.67) (61). Vertical Franz diffusion cells were used to study and test the dexamethasone-loaded polymeric nanocapsules. The results of the in vitro release showed that there was minor drug release per cm2 after 2 and 24 h (62).
5.3.4 Nanosphere
Nanospheres are matrix systems in which medicine is either disseminated inside the polymer matrix or adsorbed on the surface of the sphere. The polymer matrix constituents and capacity to absorb fluid affect the speed of drug release (63). Nanospheres have received considerable attention recently, owing to their protective shell and ability to oxidize easily. Previous study created polymeric betamethasone disodium 21-phosphate (BP) nanoparticles and PEG-block-PLA/PLGA copolymers and homopolymers from poly (D, L-lactic acid) and poly (D, L-lactic/glycolic acid). Small nanoparticles released more BP than larger ones, whereas PLGA homopolymers released BP more quickly than PLA homopolymers (64). Tyrosine-derived nanospheres (TyroSpheres) encapsulated in anti-proliferative paclitaxel were further developed. The findings demonstrated that TyroSpheres increased [approximately 4,000 times better than that of phosphate-buffered saline (PBS)] and permitted sustained, dose-controlled release over a 72 h period under conditions simulating skin permeation. By enabling paclitaxel to be delivered into the epidermis at concentrations of >100 ng/cm2 of skin surface area and by increasing the cytotoxicity of loaded paclitaxel to human keratinocytes, tyrospheroids may effectively treat psoriasis (65).
5.4 Metallic nanocarriers
Metallic nanomaterials have been of significant interest to the scientific community for many decades. These particles have antibacterial, antifungal, and anti-skin cancer effects, and therefore, are promising for the management of dermatological diseases. Types of metallic nanoparticles have been synthesized using different elements, such as gold nanoparticles (AuNPs) and silver nanoparticles (AgNPs).
5.4.1 Gold nanoparticles
Solid colloidal particles, called AuNPs, with sizes ranging from 1 to 100 nm, are created from metal precursors. Nanoparticles and their contents can also pass through biological barriers that are difficult to access and penetrate, owing to their small size. Using different chemistries or because of their high affinity for thiolated molecules, they can function easily with all types of electron-donating compounds (26, 66). The ease of functionalization, increased surface area to volume ratio, small particle size, and the anti-inflammatory action they perform provide a synergistic effect when loaded with anti-inflammatory drugs (67). Combining topical AuNPs and AgNPs with cornus mas inhibits proliferation in human plaque psoriasis by downregulating the activity of nuclear factor-3B, as well as decreasing cluster of differentiation 68-positive macrophages and IL-12 and TNF-α production (68). In another study, woodfordia fruticosa (flower extract)-enriched AuNPs were used to reduce hyperplasia, parakeratosis, and serum concentrations of TNF-α, IL-22, and IL-23 and showed that 1% AuNPs (ointment) mixed with Swiss albino mice showed the lowest energy level and had a significant therapeutic value (69).
5.4.2 Silver nanoparticles
Silver nanoparticles are one of the most promising metal nanoparticles and have been widely used in nanomedicine, especially for the diagnosis and treatment of cancer. In addition, the potential of AgNPs for the delivery of antimicrobial, antibacterial, antifungal, and anti-material agents has been explored. Moreover, AgNPs have been effectively used as delivery carriers for anti-psoriasis drugs (70). A team prepared biocompatible AgNPs containing fruit extracts of European blackberry and examined their anti-inflammatory effects. The synthesized nanoparticles have good anti-inflammatory effects and have been studied both in vivo and in vitro. In vitro, anti-inflammatory effects were shown by a reduction in cytokine production and maintenance of low levels after UVB irradiation. In vivo, AgNPs pre-administration reduced cytokine levels in the foot tissue and had a long-term protective effect. AuNPs are considered promising anti-psoriasis treatments (71). Another study evaluated the anti-inflammatory capability of nanoparticles created in rats using carrageenan-induced hind foot edema models and albumin denaturation and suggested that silver nanoparticles may reduce or prevent the release of acute inflammatory mediators. This study unequivocally demonstrated that Selaginella myosurus mediated by AgNPs might be a source of anti-inflammatory medications (72).
In addition to the above-mentioned nanocarriers, diverse novel carriers have been developed for the effective delivery of various anti-psoriatic drugs. Table 2 lists the topical applications of nanocarriers in drug delivery for psoriasis therapy, which collectively constitutes a large category of human conditions. Novel delivery systems have gained a unique position for safe and effective drug delivery to other dermatological diseases, including atopic dermatitis, melanoma, and acne. Considering the unique features of each nanocarriers, we suggest that a proper combination should be considered based on the physicochemical properties of the loaded drugs and the clinical characteristics of patients with psoriasis.
6 Conclusion and future perspective
Although psoriasis cannot be permanently treated, controlling its clinical manifestations can significantly improve the quality of life of patients and eliminate their psychological burden. Drug delivery for skin disorders has always been a clinical challenge given that it is essential to achieve maximum possible epidermal penetration and retention with minimum drug absorption into the bloodstream to avoid side effects. Hyper-keratinized skin thickens the cuticle, making it difficult for different active ingredients to circumvent, which directly affects drug delivery and retention. Accordingly, novel drug delivery systems, such as liposomes, niosomes, SLNs, and NLCs hold promise in preclinical and experimental studies, owing to their ability to overcome key formulation challenges.
Many studies have explored this, but further research should be performed. Contemporary research focuses more on polymer and lipid nanoparticles, while metal nanoparticles may attract more interest in future work because of their small size and outstanding performance. Additionally, hybrid nanosystems can combine the advantages of different types of nanoparticles to obtain the most appropriate drug delivery system for patients. This approach has not been thoroughly studied in the local treatment of psoriasis but may greatly improve the management of these psoriatic plaques.
In conclusion, we noted that published data on nano-dermatology appear to be successful at different stages of the healthcare process, offering a personalized approach to immune-mediated inflammatory dermatosis. In the future, high-prognosis psoriasis models (in vivo and in vitro models) are needed to improve the adoption rates, which may be a key approach in the fight against psoriasis.
Author contributions
MZ and SH contributed to manuscript writing, editing, and data collection. XS, YZ, YL, LL, JW, CW, and NL contributed to data analysis. XL contributed to conceptualization and supervision. All authors have read and approved the final manuscript.
Funding
This work was sponsored by the National Natural Science Foundation of China (Nos. 82074427 and 81874470), the Xinglin Scholar, Shanghai University of Traditional Chinese Medicine (No. RY411.14.12), Science and Technology Commission of Shanghai Municipality (Nos. 22Y11922200 and 21Y21920102), and Shanghai Health Care Commission (No. 20224Z0019).
Conflict of interest
The authors declare that the research was conducted in the absence of any commercial or financial relationships that could be construed as a potential conflict of interest.
Publisher’s note
All claims expressed in this article are solely those of the authors and do not necessarily represent those of their affiliated organizations, or those of the publisher, the editors and the reviewers. Any product that may be evaluated in this article, or claim that may be made by its manufacturer, is not guaranteed or endorsed by the publisher.
Abbreviations
DC, dendritic cell; SC, stratum corneum; MTX, methotrexate; CYC, cyclosporine; NEs, nano-emulsions; SLNs, solid lipid nanoparticles; NLCs, nanostructured lipidic carrierss; BD, betamethasone dipropionate; CT, calcipotriol; SA, salicylic acid; ACT, acitretin; DIT, Dithranol; PPI, polypropylene imine; TAC, tacrolimus; PAMAM, polyamidoamine; 8-MOP, 8-methoxyp-soralen; DOTAP, dioleoyl-3-trimethylammonium propane; BP, betamethasone disodium 21-phosphate; AuNPs, gold nanoparticle; AgNPs, silver nanoparticle.
References
1. Sala M, Elaissari A, Fessi H. Advances in psoriasis physiopathology and treatments: up to date of mechanistic insights and perspectives of novel therapies based on innovative skin drug delivery systems (ISDDS). J Control Release. (2016) 239:182–202. doi: 10.1016/j.jconrel.2016.07.003
2. Mabuchi T, Chang TW, Quinter S, Hwang ST. Chemokine receptors in the pathogenesis and therapy of psoriasis. J Dermatol Sci. (2012) 65:4–11. doi: 10.1016/j.jdermsci.2011.11.007
3. Pradhan M, Singh D, Singh MR. Novel colloidal carriers for psoriasis: current issues, mechanistic insight and novel delivery approaches. J Control Release. (2013) 170:380–95. doi: 10.1016/j.jconrel.2013.05.020
4. Gungor S, Rezigue M. Nanocarriers mediated topical drug delivery for psoriasis treatment. Curr Drug Metab. (2017) 18:454–68. doi: 10.2174/1389200218666170222145240
5. Cai XC, Ru Y, Liu L, Sun XY, Zhou YQ, Luo Y, et al. Efficacy and safety of biological agents for the treatment of pediatric patients with psoriasis: a bayesian analysis of six high-quality randomized controlled trials. Front Immunol. (2022) 13:896550. doi: 10.3389/fimmu.2022.896550
6. Liu L, Wang J, Li HJ, Zhang S, Jin MZ, Chen ST, et al. Sphingosine-1-phosphate and its signal modulators alleviate psoriasis-like dermatitis: preclinical and clinical evidence and possible mechanisms. Front Immunol. (2021) 12:759276. doi: 10.3389/fimmu.2021.759276
7. Wang J, Zhang S, Xing M, Hong S, Liu L, Ding XJ, et al. Current evidence on the role of lipid lowering drugs in the treatment of psoriasis. Front Med. (2022) 9:900916.
8. Traub M, Marshall K. Psoriasis–pathophysiology, conventional, and alternative approaches to treatment. Altern Med Rev. (2007) 12:319–30.
9. Armstrong AW, Read C. Pathophysiology, clinical presentation, and treatment of psoriasis: a review. JAMA. (2020) 323:1945–60. doi: 10.1001/jama.2020.4006
10. Morizane S, Yamasaki K, Mühleisen B, Kotol PF, Murakami M, Aoyama Y, et al. Cathelicidin antimicrobial peptide LL-37 in psoriasis enables keratinocyte reactivity against TLR9 ligands. J Invest Dermatol. (2012) 132:135–43. doi: 10.1038/jid.2011.259
11. Nestle FO, Conrad C, Tun-Kyi A, Homey B, Gombert M, Boyman O, et al. Plasmacytoid predendritic cells initiate psoriasis through interferon-alpha production. J Exp Med. (2005) 202:135–43. doi: 10.1084/jem.20050500
12. Gregorio J, Meller S, Conrad C, Di Nardo A, Homey B, Lauerma A, et al. Plasmacytoid dendritic cells sense skin injury and promote wound healing through type I interferons. J Exp Med. (2010) 207:2921–30. doi: 10.1084/jem.20101102
13. Santini SM, Lapenta C, Donati S, Spadaro F, Belardelli F, Ferrantini M. Interferon-α-conditioned human monocytes combine a Th1-orienting attitude with the induction of autologous Th17 responses: role of IL-23 and IL-12. PLoS One. (2011) 6:e17364. doi: 10.1371/journal.pone.0017364
14. Nestle FO, Turka LA, Nickoloff BJ. Characterization of dermal dendritic cells in psoriasis. Autostimulation of T lymphocytes and induction of Th1 type cytokines. J Clin Invest. (1994) 94:202–9. doi: 10.1172/JCI117308
15. Takahashi H, Tsuji H, Minami-Hori M, Miyauchi Y, Iizuka H. Defective barrier function accompanied by structural changes of psoriatic stratum corneum. J Dermatol. (2014) 41:144–8. doi: 10.1111/1346-8138.12393
16. Torsekar R, Gautam MM. Topical therapies in psoriasis. Indian Dermatol Online J. (2017) 8:235–45. doi: 10.4103/2229-5178.209622
17. Bos JD, Spuls PI. Topical treatments in psoriasis: today and tomorrow. Clin Dermatol. (2008) 26:432–7. doi: 10.1016/j.clindermatol.2007.10.025
18. Lindqvist T, Salah LA, Gillstedt M, Wennberg AM, Osmancevic A. Methotrexate management in psoriasis: are we following the guidelines? Acta Derm Venereol. (2018) 98:449–51. doi: 10.2340/00015555-2857
19. Ho VC, Griffiths CE, Berth-Jones J, Papp KA, Vanaclocha F, Dauden E, et al. Intermittent short courses of cyclosporine microemulsion for the long-term management of psoriasis: a 2-year cohort study. J Am Acad Dermatol. (2001) 44:643–51. doi: 10.1067/mjd.2001.112400
20. Bai F, Li GG, Liu Q, Niu X, Li R, Ma H. Short-Term Efficacy and Safety of IL-17, IL-12/23, and IL-23 Inhibitors Brodalumab, Secukinumab, Ixekizumab, Ustekinumab, Guselkumab, Tildrakizumab, and Risankizumab for the Treatment of Moderate to Severe Plaque Psoriasis: a systematic review and network meta-analysis of randomized controlled trials. J Immunol Res. (2019) 2019:2546161. doi: 10.1155/2019/2546161
21. Ten Bergen LL, Petrovic A, Krogh Aarebrot A, Appel S, The TNF. /IL-23/IL-17 axis-Head-to-head trials comparing different biologics in psoriasis treatment. Scand J Immunol. (2020) 92:e12946. doi: 10.1111/sji.12946
22. Haber SL, Hamilton S, Bank M, Leong SY, Pierce E. Apremilast: a novel drug for treatment of psoriasis and psoriatic arthritis. Ann Pharmacother. (2016) 50:282–90. doi: 10.1177/1060028015627467
23. Barratt G. Colloidal drug carriers: achievements and perspectives. Cell Mol Life Sci. (2003) 60:21–37. doi: 10.1007/s000180300002
24. Zhang Z, Tsai PC, Ramezanli T, Michniak-Kohn BB. Polymeric nanoparticles-based topical delivery systems for the treatment of dermatological diseases. Wiley Interdiscip Rev Nanomed Nanobiotechnol. (2013) 5:205–18. doi: 10.1002/wnan.1211
25. Kim ST, Jang DJ, Kim JH, Park JY, Lim JS, Lee SY, et al. Topical administration of cyclosporin A in a solid lipid nanoparticle formulation. Pharmazie. (2009) 64:510–4.
26. Daraee H, Eatemadi A, Abbasi E, Fekri Aval S, Kouhi M, Akbarzadeh A, et al. Application of gold nanoparticles in biomedical and drug delivery. Artif Cells Nanomed Biotechnol. (2016) 44:410–22. doi: 10.3109/21691401.2014.955107
27. Akbarzadeh A, Rezaei-Sadabady R, Davaran S, Joo SW, Zarghami N, Hanifehpour Y, et al. Liposome: classification, preparation, and applications. Nanoscale Res Lett. (2013) 8:102. doi: 10.1186/1556-276X-8-102
28. Allen TM, Cullis PR. Liposomal drug delivery systems: from concept to clinical applications. Adv Drug Deliv Rev. (2013) 65:36–48. doi: 10.1016/j.addr.2012.09.037
29. Walunj M, Doppalapudi S, Bulbake U, Khan W. Preparation, characterization, and in vivo evaluation of cyclosporine cationic liposomes for the treatment of psoriasis. J Liposome Res. (2020) 30:68–79. doi: 10.1080/08982104.2019.1593449
30. Yu F, Zhang Y, Yang C, Li F, Qiu B, Ding W. Enhanced transdermal efficiency of curcumin-loaded peptide-modified liposomes for highly effective antipsoriatic therapy. J Mater Chem B. (2021) 9:4846–56. doi: 10.1039/D1TB00557J
31. Desai PR, Marepally S, Patel AR, Voshavar C, Chaudhuri A, Singh M. Topical delivery of anti-TNFα siRNA and capsaicin via novel lipid-polymer hybrid nanoparticles efficiently inhibits skin inflammation in vivo. J Control Release. (2013) 170:51–63. doi: 10.1016/j.jconrel.2013.04.021
32. Touitou E, Dayan N, Bergelson L, Godin B, Eliaz M. Ethosomes – novel vesicular carriers for enhanced delivery: characterization and skin penetration properties. J Control Release. (2000) 65:403–18. doi: 10.1016/S0168-3659(99)00222-9
33. Fathalla D, Youssef EMK, Soliman GM. Liposomal and ethosomal gels for the topical delivery of anthralin: preparation, comparative evaluation and clinical assessment in psoriatic patients. Pharmaceutics. (2020) 12:446. doi: 10.3390/pharmaceutics12050446
34. Chandra A, Aggarwal G, Manchanda S, Narula A. Development of topical gel of methotrexate incorporated ethosomes and salicylic acid for the treatment of psoriasis. Pharm Nanotechnol. (2019) 7:362–74. doi: 10.2174/2211738507666190906123643
35. Fang JY, Fang CL, Liu CH, Su YH. Lipid nanoparticles as vehicles for topical psoralen delivery: solid lipid nanoparticles (SLN) versus nanostructured lipid carriers (NLC). Eur J Pharm Biopharm. (2008) 70:633–40. doi: 10.1016/j.ejpb.2008.05.008
36. Sinico C, Fadda AM. Vesicular carriers for dermal drug delivery. Expert Opin Drug Deliv. (2009) 6:813–25. doi: 10.1517/17425240903071029
37. Lakshmi PK, Devi GS, Bhaskaran S, Sacchidanand S. Niosomal methotrexate gel in the treatment of localized psoriasis: phase I and phase II studies. Indian J Dermatol Venereol Leprol. (2007) 73:157–61. doi: 10.4103/0378-6323.32709
38. Yang X, Tang Y, Wang M, Wang Y, Wang W, Pang M, et al. Co-delivery of methotrexate and nicotinamide by cerosomes for topical psoriasis treatment with enhanced efficacy. Int J Pharm. (2021) 605:120826. doi: 10.1016/j.ijpharm.2021.120826
39. Meng S, Sun L, Wang L, Lin Z, Liu Z, Xi L, et al. Loading of water-insoluble celastrol into niosome hydrogels for improved topical permeation and anti-psoriasis activity. Colloids Surf B Biointerfaces. (2019) 182:110352. doi: 10.1016/j.colsurfb.2019.110352
40. Singh Y, Meher JG, Raval K, Khan FA, Chaurasia M, Jain NK, et al. Nanoemulsion: concepts, development and applications in drug delivery. J Control Release. (2017) 252:28–49. doi: 10.1016/j.jconrel.2017.03.008
41. Wu H, Ramachandran C, Weiner ND, Roessler BJ. Topical transport of hydrophilic compounds using water-in-oil nanoemulsions. Int J Pharm. (2001) 220:63–75. doi: 10.1016/S0378-5173(01)00671-8
42. Musa SH, Basri M, Fard Masoumi HR, Shamsudin N, Salim N. Enhancement of physicochemical properties of nanocolloidal carrier loaded with cyclosporine for topical treatment of psoriasis: in vitro diffusion and in vivo hydrating action. Int J Nanomed. (2017) 12:2427–41. doi: 10.2147/IJN.S125302
43. Bernardi DS, Pereira TA, Maciel NR, Bortoloto J, Viera GS, Oliveira GC, et al. Formation and stability of oil-in-water nanoemulsions containing rice bran oil: in vitro and in vivo assessments. J Nanobiotechnol. (2011) 9:44. doi: 10.1186/1477-3155-9-44
44. Woo JO, Misran M, Lee PF, Tan LP. Development of a controlled release of salicylic acid loaded stearic acid-oleic acid nanoparticles in cream for topical delivery. ScientificWorldJournal. (2014) 2014:205703. doi: 10.1155/2014/205703
45. Sonawane R, Harde H, Katariya M, Agrawal S, Jain S. Solid lipid nanoparticles-loaded topical gel containing combination drugs: an approach to offset psoriasis. Expert Opin Drug Deliv. (2014) 11:1833–47. doi: 10.1517/17425247.2014.938634
46. Ferreira M, Barreiros L, Segundo MA, Torres T, Selores M, Costa Lima SA, et al. Topical co-delivery of methotrexate and etanercept using lipid nanoparticles: a targeted approach for psoriasis management. Colloids Surf B Biointerfaces. (2017) 159:23–9. doi: 10.1016/j.colsurfb.2017.07.080
47. Haider M, Abdin SM, Kamal L, Orive G. Nanostructured lipid carriers for delivery of chemotherapeutics: a review. Pharmaceutics. (2020) 12:288. doi: 10.3390/pharmaceutics12030288
48. Tripathi P, Kumar A, Jain PK, Patel JR. Carbomer gel bearing methotrexate loaded lipid nanocontainers shows improved topical delivery intended for effective management of psoriasis. Int J Biol Macromol. (2018) 120:1322–34. doi: 10.1016/j.ijbiomac.2018.08.136
49. Agrawal Y, Petkar KC, Sawant KK. Development, evaluation and clinical studies of Acitretin loaded nanostructured lipid carriers for topical treatment of psoriasis. Int J Pharm. (2010) 401:93–102. doi: 10.1016/j.ijpharm.2010.09.007
50. Abdel-Mottaleb MM, Neumann D, Lamprecht A. Lipid nanocapsules for dermal application: a comparative study of lipid-based versus polymer-based nanocarriers. Eur J Pharm Biopharm. (2011) 79:36–42. doi: 10.1016/j.ejpb.2011.04.009
51. Larsson J, Sanchez-Fernandez A, Leung AE, Schweins R, Wu B, Nylander T, et al. Molecular structure of maltoside surfactants controls micelle formation and rheological behavior. J Colloid Interface Sci. (2021) 581:895–904. doi: 10.1016/j.jcis.2020.08.116
52. Lapteva M, Mondon K, Möller M, Gurny R, Kalia YN. Polymeric micelle nanocarriers for the cutaneous delivery of tacrolimus: a targeted approach for the treatment of psoriasis. Mol Pharm. (2014) 11:2989–3001. doi: 10.1021/mp400639e
53. Chavoshy F, Zadeh BSM, Tamaddon AM, Anbardar MH. Delivery and anti-psoriatic effect of silibinin-loaded polymeric micelles: an experimental study in the psoriatic skin model. Curr Drug Deliv. (2020) 17:787–98. doi: 10.2174/1567201817666200722141807
54. Li N, Qin Y, Dai D, Wang P, Shi M, Gao J, et al. Transdermal delivery of therapeutic compounds with nanotechnological approaches in psoriasis. Front Bioeng Biotechnol. (2021) 9:804415. doi: 10.3389/fbioe.2021.804415
55. Liu M, Fréchet JM. Designing dendrimers for drug delivery. Pharm Sci Technol Today. (1999) 2:393–401. doi: 10.1016/S1461-5347(99)00203-5
56. Agrawal U, Mehra NK, Gupta U, Jain NK. Hyperbranched dendritic nano-carriers for topical delivery of dithranol. J Drug Target. (2013) 21:497–506. doi: 10.3109/1061186X.2013.771778
57. Borowska K, Laskowska B, Magoń A, Mysliwiec B, Pyda M, Wołowiec S. PAMAM dendrimers as solubilizers and hosts for 8-methoxypsoralene enabling transdermal diffusion of the guest. Int J Pharm. (2010) 398:185–9. doi: 10.1016/j.ijpharm.2010.07.019
58. Kojima C. Preclinical studies of dendrimer prodrugs. Expert Opin Drug Metab Toxicol. (2015) 11:1303–15. doi: 10.1517/17425255.2015.1052404
59. Mora-Huertas CE, Fessi H, Elaissari A. Polymer-based nanocapsules for drug delivery. Int J Pharm. (2010) 385:113–42. doi: 10.1016/j.ijpharm.2009.10.018
60. Ourique AF, Pohlmann AR, Guterres SS, Beck RC. Tretinoin-loaded nanocapsules: Preparation, physicochemical characterization, and photostability study. Int J Pharm. (2008) 352:1–4. doi: 10.1016/j.ijpharm.2007.12.035
61. Savian AL, Rodrigues D, Weber J, Ribeiro RF, Motta MH, Schaffazick SR, et al. Dithranol-loaded lipid-core nanocapsules improve the photostability and reduce the in vitro irritation potential of this drug. Mater Sci Eng C Mater Biol Appl. (2015) 46:69–76. doi: 10.1016/j.msec.2014.10.011
62. Marchiori ML, Lubini G, Dalla Nora G, Friedrich RB, Fontana MC, Ourique AF, et al. Hydrogel containing dexamethasone-loaded nanocapsules for cutaneous administration: preparation, characterization, and in vitro drug release study. Drug Dev Ind Pharm. (2010) 36:962–71. doi: 10.3109/03639041003598960
63. Carino GP, Jacob JS, Mathiowitz E. Nanosphere based oral insulin delivery. J Control Release. (2000) 65:261–9. doi: 10.1016/S0168-3659(99)00247-3
64. Ishihara T, Kubota T, Choi T, Takahashi M, Ayano E, Kanazawa H, et al. Polymeric nanoparticles encapsulating betamethasone phosphate with different release profiles and stealthiness. Int J Pharm. (2009) 375:148–54. doi: 10.1016/j.ijpharm.2009.04.001
65. Sheihet L, Dubin RA, Devore D, Kohn J. Hydrophobic drug delivery by self-assembling triblock copolymer-derived nanospheres. Biomacromolecules. (2005) 6:2726–31. doi: 10.1021/bm050212u
66. Sibuyi NRS, Moabelo KL, Fadaka AO, Meyer S, Onani MO, Madiehe AM, et al. Multifunctional gold nanoparticles for improved diagnostic and therapeutic applications: a review. Nanoscale Res Lett. (2021) 16:174. doi: 10.1186/s11671-021-03632-w
67. Bessar H, Venditti I, Benassi L, Vaschieri C, Azzoni P, Pellacani G, et al. Functionalized gold nanoparticles for topical delivery of methotrexate for the possible treatment of psoriasis. Colloids Surf B Biointerfaces. (2016) 141:141–7. doi: 10.1016/j.colsurfb.2016.01.021
68. Crisan D, Scharffetter-Kochanek K, Crisan M, Schatz S, Hainzl A, Olenic L, et al. Topical silver and gold nanoparticles complexed with Cornus mas suppress inflammation in human psoriasis plaques by inhibiting NF-κB activity. Exp Dermatol. (2018) 27:1166–9. doi: 10.1111/exd.13707
69. Raghuwanshi N, Yadav TC, Srivastava AK, Raj U, Varadwaj P, Pruthi V, et al. Structure-based drug designing and identification of Woodfordia fruticosa inhibitors targeted against heat shock protein (HSP70-1) as suppressor for Imiquimod-induced psoriasis like skin inflammation in mice model. Mater Sci Eng C Mater Biol Appl. (2019) 95:57–71. doi: 10.1016/j.msec.2018.10.061
70. Pradhan M, Alexander A, Singh MR, Singh D, Saraf S, Saraf S. Understanding the prospective of nano-formulations towards the treatment of psoriasis. Biomed Pharmacother. (2018) 107:447–63. doi: 10.1016/j.biopha.2018.07.156
71. David L, Moldovan B, Vulcu A, Olenic L, Perde-Schrepler M, Fischer-Fodor E, et al. Green synthesis, characterization and anti-inflammatory activity of silver nanoparticles using European black elderberry fruits extract. Colloids Surf B Biointerfaces. (2014) 122:767–77. doi: 10.1016/j.colsurfb.2014.08.018
Keywords: novel drug delivery systems, psoriasis, topical therapy, transdermal, nanocarriers
Citation: Zhang M, Hong S, Sun X, Zhou Y, Luo Y, Liu L, Wang J, Wang C, Lin N and Li X (2022) Exploration of and insights into advanced topical nanocarrier systems for the treatment of psoriasis. Front. Med. 9:1017126. doi: 10.3389/fmed.2022.1017126
Received: 11 August 2022; Accepted: 28 November 2022;
Published: 15 December 2022.
Edited by:
Stefania Guida, Vita-Salute San Raffaele University, ItalyReviewed by:
Fazil Ahmad, Imam Abdulrahman Bin Faisal University, Saudi ArabiaAnnalisa Saltari, University Hospital Zurich, Switzerland
Copyright © 2022 Zhang, Hong, Sun, Zhou, Luo, Liu, Wang, Wang, Lin and Li. This is an open-access article distributed under the terms of the Creative Commons Attribution License (CC BY). The use, distribution or reproduction in other forums is permitted, provided the original author(s) and the copyright owner(s) are credited and that the original publication in this journal is cited, in accordance with accepted academic practice. No use, distribution or reproduction is permitted which does not comply with these terms.
*Correspondence: Xin Li, 13661956326@163.com
†These authors have contributed equally to this work and share first authorship