- 1Tumor Etiology and Screening Department of Cancer Institute and General Surgery, The First Affiliated Hospital of China Medical University, Key Laboratory of Cancer Etiology and Prevention, China Medical University, Liaoning Provincial Education Department, Shenyang, China
- 2Tangdu Hospital of the Fourth Military Medical University, Xi’an, China
- 3Department of General Surgery, The First Hospital of China Medical University, Shenyang, China
- 4Department of Hepatobiliary Surgery, Institute of General Surgery, The First Hospital of China Medical University, Shenyang, China
Helicobacter pylori (H. pylori) is one of the globally recognized causative factors of gastric cancer (GC). Currently, no definite therapy and drugs for H. pylori-related GC have been widely acknowledged although H. pylori infection could be eradicated in early stage. Inflammation and immune response are spontaneous essential stages during H. pylori infection. H pylori may mediate immune escape by affecting inflammation and immune response, leading to gastric carcinogenesis. As an important component of transcriptome, non-coding RNAs (ncRNAs) have been proven to play crucial roles in the genesis and development of H. pylori-induced GC. This review briefly described the effects of ncRNAs on H. pylori-related GC from the perspective of inflammation and immune response, as well as their association with inflammatory reaction and immune microenvironment. We aim to explore the potential of ncRNAs as markers for the early diagnosis, prognosis, and treatment of H. pylori-related GC. The ncRNAs involved in H. pylori-related GC may all hold promise as novel therapeutic targets for immunotherapy.
Introduction
Gastric cancer (GC) is one of the leading malignancies in terms of morbidity and mortality worldwide, which has attracted extensive attention among global health problems (1). Its high incidence and mortality are mainly attributed to some unclear pathogenesis. The chronic infection of Helicobacter pylori (H. pylori) has been well-accepted as one of the multiple pathogenic factors of GC. It has been listed as a primary carcinogen by the International Agency for Cancer (2, 3). Chronic H. pylori infection can result in diseases such as gastritis, gastric ulcer and GC over time, and gastric mucosa-associated lymphoid tissue (MALT) lymphoma may also develop in some patients (4–7). However, the specific mechanism of H. pylori infection on gastric carcinogenesis remains unidentified in spite of the known causality between them. Exploration for the mechanism would be conducive to GC prevention and treatment.
As a key element of pathogenic factors for H. pylori-related GC, the virulence factors of H. pylori associated with immune response mainly consist of cytotoxin-associated gene A (CagA), vacuolating cytotoxin A (VacA), and H. pylori neutrophil-activating protein (HP-NAP), etc. (8). Among them, CagA was reported to exist in more than 60% of H. pylori strains considered to be a strong activator of NF-κB and a major mediator of carcinogenesis (9). CagA can not only bind to NF-κB via beta-catenin but also independently affect NF-κB and produce inflammatory factors such as IL-8 to mediate immune response (9, 10). VacA inhibits capital histocompatibility complex class II (MHC II)-dependent pathways and releases pro-inflammatory factors including IL-1β, IL-6, IL-10, and tumor necrosis factor-alpha (TNFα) by forming vacuoles within macrophages, thus inducing immune escape and protecting H. pylori (11). Unlike VacA, HP-NAP up-regulates MHC II, promotes Th1 immune response, induces the expression of IL-12 and IL-23 in neutrophils and monocytes, and triggers ROS release to destroy epithelial cells (12, 13). The recognition of lipopolysaccharide (LPS) from H. pylori by toll-like receptors (TLRs, mainly TLR4 and TLR2) in human body could activate the NF-κB pathway and promote the chemotaxis of immune cells such as neutrophils and dendritic cells to release large amounts of inflammatory factors attempting to phagocytose H. pylori. However, the specific virulence factors of H. pylori could protect themselves from phagocytosis initiated by innate immune response. Consequently, the large quantity of inflammatory factors have to accumulate at H. pylori colonization sites and cause a long-term inflammation in epithelium, leading to chronic gastritis and even GC (14).
The unique virulence factors enable H. pylori to escape from the eradication by immune system and colonize in gastric mucosa making carcinogenic effects. After H. pylori infection, innate immunity is first activated to release pro-inflammatory factors and immune cells intending to phagocytose and eliminate H. pylori, then local immune microenvironment is altered. Once the changes fail to resist the pathogenic effects of virulence factors, H. pylori could colonize in gastric mucosa, break through the barrier and survive in the deep site of stomach chronically, resulting in gastritis, gastric ulcer and even GC over time. Therefore, inflammation and immune response are essential parts during the initiation of H. pylori-related GC.
Mounting studies about H. pylori-related GC have focused on non-coding RNAs (ncRNAs). NcRNAs consist of microRNAs (miRNAs), long ncRNAs (lncRNAs), circular RNAs (circRNAs), small nucleolar RNAs (snoRNAs), and piRNAs (15). In the early stage after discovery, ncRNAs were regarded as useless “garbage” in body due to the lack of capability to encode proteins. With the depth of research, however, the important regulatory function of ncRNAs in many basic cellular processes has been gradually revealed including development, differentiation, proliferation, transcription, post-transcriptional modification, immune regulation, cell apoptosis, and metabolism (16). Despite the inability of ncRNAs to directly encode proteins, more than 60% of their downstream target genes have the competence (17). It has been suggested that rare ncRNAs can independently function in diseases. They usually interact with each other to construct powerful networks and affect many proteins determining cell fates as well as specific cellular biological process by regulating functional stability. The complex interactions make the dysregulation of ncRNAs quite common in cancer (18–20). The immune inflammatory response occurring in H. pylori-related GC can alter the expression patterns of ncRNAs, thereby affecting the expression of downstream proteins or target genes and GC biological behaviors.
In the present review, we integrated the articles studying ncRNAs with immune inflammation in H. pylori-related GC, summarize the research frontiers of ncRNAs in this field and explore the potential of ncRNAs as diagnostic and prognostic markers for H. pylori-related GC, aiming to provide theoretical basis for further investigation of ncRNAs on the immune direction in H. pylori-related GC. The immunization of ncRNAs in H. pylori-related GC might give a new sight for GC diagnosis and treatment.
MiRNAs and Helicobacter pylori-related gastric cancer
MiRNAs are short ncRNAs with approximately 22 nucleotides in length. They can inhibit the expression or function of downstream target genes by inducing degradation or translational inhibition through binding to the 3′ untranslated region (3′-UTR) of target genes (especially mRNAs) with their 5′ ends (21). MiRNAs are the most extensively studied ncRNAs so far. The association of miRNAs with H. pylori-related GC has been gradually emphasized on immune inflammation. TLRs could be activated by LPS from H. pylori, and aberrant TLRs may promote the expression of some miRNAs. MiRNAs can also affect the NF-κB pathway by regulating TLRs expression and the TLR signaling pathway in turn, inducing the release of pro-inflammatory factors, transcription factors and cascades (22, 23). The structure of LPS could also be modulated by small RNAs to influence the immune recognition in host thereby enhancing H. pylori resistance (24). Therefore, miRNAs are shown to have the potential to serve as detective markers for early diseases (25–28).
MiR-155
MiR-155 has emerged as a key factor in innate immunity and inflammatory reaction. H. pylori could induce miR-155 in gastric epithelial cells and gastric mucosa, which has been widely reported to damage H. pylori by triggering immune response (23, 29–33). The induction of miR-155 in T cells mediated by H. pylori might be based on a cAMP-Foxp3-dependent manner (34). MiR-155 promotes the release of inflammatory factors including TNF-α, IL-6, and IL-23 in exosomes from macrophages infected with H. pylori and simultaneously increases the expression of CD40, CD63, CD81, and MCH-I, suggesting that miR-155 may regulate H. pylori-induced inflammation in host cells via exosomes (35). MiR-155 can also promote H. pylori elimination by inducing autophagy to enhance the sterilization ability of host to intracellular H. pylori (36). The association of miR-155 with immunity in H. pylori infection appears to be proved in mice. The generation of miR-155–/– mice might be owing to an inherent defect in T cells that impairs specific Th1 and Th17 cells making them disabled to proliferate, produce IFN-γ and IL-17, thus control H. pylori infection effectively (37). In addition, miR-155 is up-regulated in macrophages during H. pylori infection dependent on TLR and type IV secretion system (T4SS), inhibiting cell apoptosis caused by DNA damage (38).
However, some researchers believed that miR-155 negatively regulated inflammatory reaction and reduced immune response of body to protect H. pylori. Xiao et al. (39) found that the induction of miR-155 by H. pylori was dependent on the activation of NF-κB and AP-1 pathways. After miR-155 overexpression, a down-regulation was demonstrated in IkappaB kinase epsilon (IKK-ε), Fas-associated death domain (FADD) as well as Sma- and Mad-related protein 2 (SMAD2), which negatively regulated the release of IL-8 and growth-related oncogene-α (GRO-α). Except for miR-155, myeloid differentiation protein 88 (MyD88) was also observed to be involved in the negative regulation of H. pylori-induced inflammatory reaction as a target gene of miR-155 (40).
The diverse roles of miR-155 in H. pylori-induced immune inflammatory response mentioned above indicated it as a potential marker for H. pylori-related GC.
MiR-223
MiR-223 was also suggested to be involved in pathways associated with innate immunity and inflammatory reaction in patients with H. pylori infection (41–43). CagA of H. pylori may induce miR-223-3P expression through the NF-κB pathway. And miR-223-3p can directly target AT-rich interactive-domain 1A (ARID1A), a tumor suppressor protein with ATP enzymatic activity, to promote GC proliferation and migration. Therefore, H. pylori might participate in CagA-mediated biological effects in GC cells via the NF-κB/miR-223-3p/ARID1A axis (44). MiR-223-3p and IL-10 secreted by macrophages exert inhibitory effects on pro-IL-1β and inflammasome NLRP3 both secreted by monocytes during H. pylori infection (32, 45). Furthermore, the mucosal expression level of miR-223 was significantly decreased following with the disappearance of neutrophils from gastric mucosa in patients after H. pylori eradication (46). A recent study, however, showed that miR-233 expression decreased in H. pylori-associated autoimmune atrophic gastritis and multifocal atrophic gastritis. Hence, the anti-inflammatory function of miR-233 might vary with different microenvironments (47). These findings made miR-223 also a potential marker for the diagnosis or treatment of H. pylori-related GC.
MiR-375
MiR-375 appears to be closely associated with immune inflammatory response in H. pylori-related GC. It was shown to be down-regulated after H. pylori infection accompanied by the activation of JAK2-STAT3 (48–51). Janus kinase 2 (JAK2) was identified as a target gene of miR-375, and miR-375 could negatively regulate the expression of programmed cell death-ligand 1 (PD-L1) in GC through the JAK2/STAT3 signaling pathway (50). H. pylori may affect the JAK2-STAT3 pathway releasing cytokines including IL-6, IL-10, and VEGF by down-regulating miR-375, inhibit the maturity of dendritic cells, reduce CD4+ and CD8+ T cells, then inhibit immune response mediating the immune escape of H. pylori to promote gastric carcinogenesis (52). Moreover, the regulation of JAK2-STAT3 signaling by miR-375 could release IL-1β, IL-6, IL-8, and TNF-α by activating downstream target genes BCL-2 and TWIST1, which promotes tumor transformation and gets involved in H. pylori-induced cell proliferation and migration (53). Rossi et al. found that the levels of IL-6, IL-12A, and IL-2 were significantly elevated in H. pylori-positive patients followed by the down-regulation of miR-103, miR-181c, miR-370, and miR-375 (54). All above-mentioned results suggested that miR-375 participated in H. pylori-related GC by regulating immune response.
MiR-146
MiR-146 might be involved in the development of H. pylori-related gastric diseases via the NF-KB pathway. H. pylori infection could trigger inflammatory reaction of body and induce the production of IL-17A, GRO-α, IL-8, and miR-146α in GC cells successively. For its mechanism, IL-17A might mediate miR-146α to regulate inflammatory reaction in an NF-κB-dependent manner during H. pylori infection (55). Other than H. pylori-related GC, miRNAs especially miR-146a and miR155 also received much attention in H. pylori-related pediatric gastritis, which were likely to be associated with its prognosis (56, 57).
Other miRNAs
Most miRNAs involved in the regulation of H. pylori-related GC had association with the NF-κB pathway. The decrease of miR-204 level induced by H. pylori infection could up-regulate the downstream target gene BIRC2, enhance the activity of BIRC2/TNF-α/NF-κB signaling pathway, thus promote angiogenesis and metastasis of GC cells, leading to poor prognosis of H. pylori-related GC patients (58). H. pylori infection was reported to induce miR-18a-3P and miR-4286 expression in GC through TLR4/NF-κB molecules related to immune recognition, inhibit the expression of downstream target gene BARAP1, thereby participate in innate immune response of body and inflammatory pathways associated with NF-κB (59). H. pylori activates NF-κB and reduces miR-218 expression to increase the level of downstream target gene called epidermal growth factor receptor-amplified and overexpressed protein (ECOP) promoting cell proliferation. Accordingly, Gao et al. believed that miR-218 could be considered as a therapeutic target for H. pylori-related GC. The inhibition of NF-κB by increasing miR-218 expression level artificially might become a kind of therapy to prevent the progression from precancerous lesion to cancer (60). In gastric epithelial cells infected with H. pylori, Tip-α inhibits miR-3178 targeting TRAF3 to increase TNF-α and IL-6 activating NF-κB to promote GC cell proliferation (61). Let-7b targeting TLR4 was shown to decrease in gastric epithelial cells infected with H. pylori. The knockdown of TLR4 concurrent with let-7b overexpression could reduce the expression of downstream genes associated with immune inflammatory response including NF-κB, MyD88, NF-κB1/p50, and RelA/p65 (62). Lin et al. found that serum miR-130b level was elevated in human and mice after H. pylori infection activating the NF-κB pathway positively correlated with myeloid-suppressor Schlafen4 (Slfn4). Further research revealed that gastric SLFN4+ cells infected with H. pylori might induce miR-130b and exhibit a T cell suppressive phenotype resulting in H. pylori-induced gastric metaplasia, tumor formation and growth (63). Based on these findings, H. pylori infection may activate the NF-κB pathway by regulating miRNAs to affect biological function facilitating the development of inflammation and cancer.
Additionally, miRNAs may also influence H. pylori-related GC by regulating PD-1/PD-L1 involved in immune response. PD-L1 might be a downstream target of miR-140. MiR-140 could increase IFN-γ and TNF-α levels, inhibit the PD-L1 and mTOR signaling pathways, and raise CD8+ T cells, thus inhibiting the proliferation of H. pylori-positive GC to exert anti-cancer roles (64). Xie et al. (65) reported that H. pylori could regulate B7-H1 binding to PD-1 by inhibiting miR-152 and miR-200b in GC cells, thereby suppress T cell proliferation and immune response. H. pylori might also alter the levels of miR-326 and miR-663 in CD4+ T cells (66).
Methylation has been suggested to be another mechanism by which miRNAs affect H. pylori through immune inflammatory response. The extract of Celastrus orbiculatus (COE) regulates H. pylori-induced inflammatory response by inhibiting miR-21 expression and the methylation level of target gene programmed cell death 4 (PDCD4) (67). Furthermore, the tumor suppressor factor miR-124 silences spermine oxidase (SMOX) in H. pylori-related GC via DNA methylation (68). JARID1B is a histone demethylase and its up-regulation is associated with immune cell infiltration in H. pylori-related GC. Therefore, the miR-29c/JARID1B/cyclinD1 axis could be a novel therapeutic pathway for GC (69).
Some other miRNAs were also indicated to be associated with immunity or inflammation in H. pylori-related GC. H. pylori up-regulates the immune receptor CD300E by down-regulating miR-4270 to enhance the pro-inflammatory potential and impair the sterilization ability of macrophages (70). Chronic inflammation caused by H. pylori infection was shown to increase CD44V9 by down-regulating miR-328 in gastric mucosa (71). Except for miR-223-3p mentioned above, miR-22 can also directly target NLRP3, reduce inflammasomes levels and maintain the homeostasis of gastric microenvironment. H. pylori disrupts microenvironment homeostasis by inhibiting the regulation of miR-22 on downstream factor NLRP3 (72). In conclusion, miRNAs could regulate the expression of inflammatory factors and suppress immune response after H. pylori infection, contributing to the immune escape of H. pylori and ultimately GC (Table 1 and Figure 1).
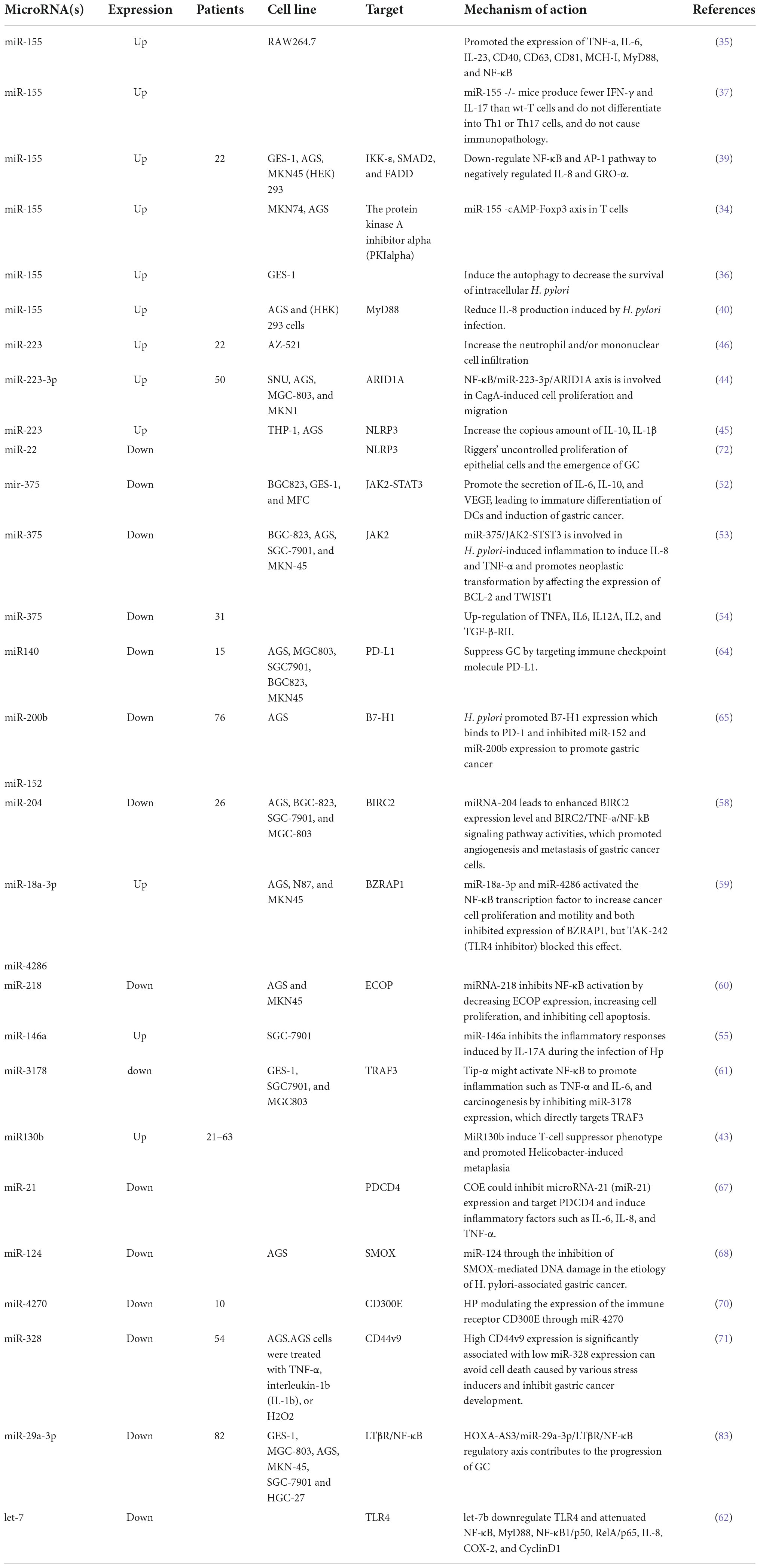
Table 1. The regulation of miRNAs on inflammation and immune response in H. pylori-related gastric cancer.
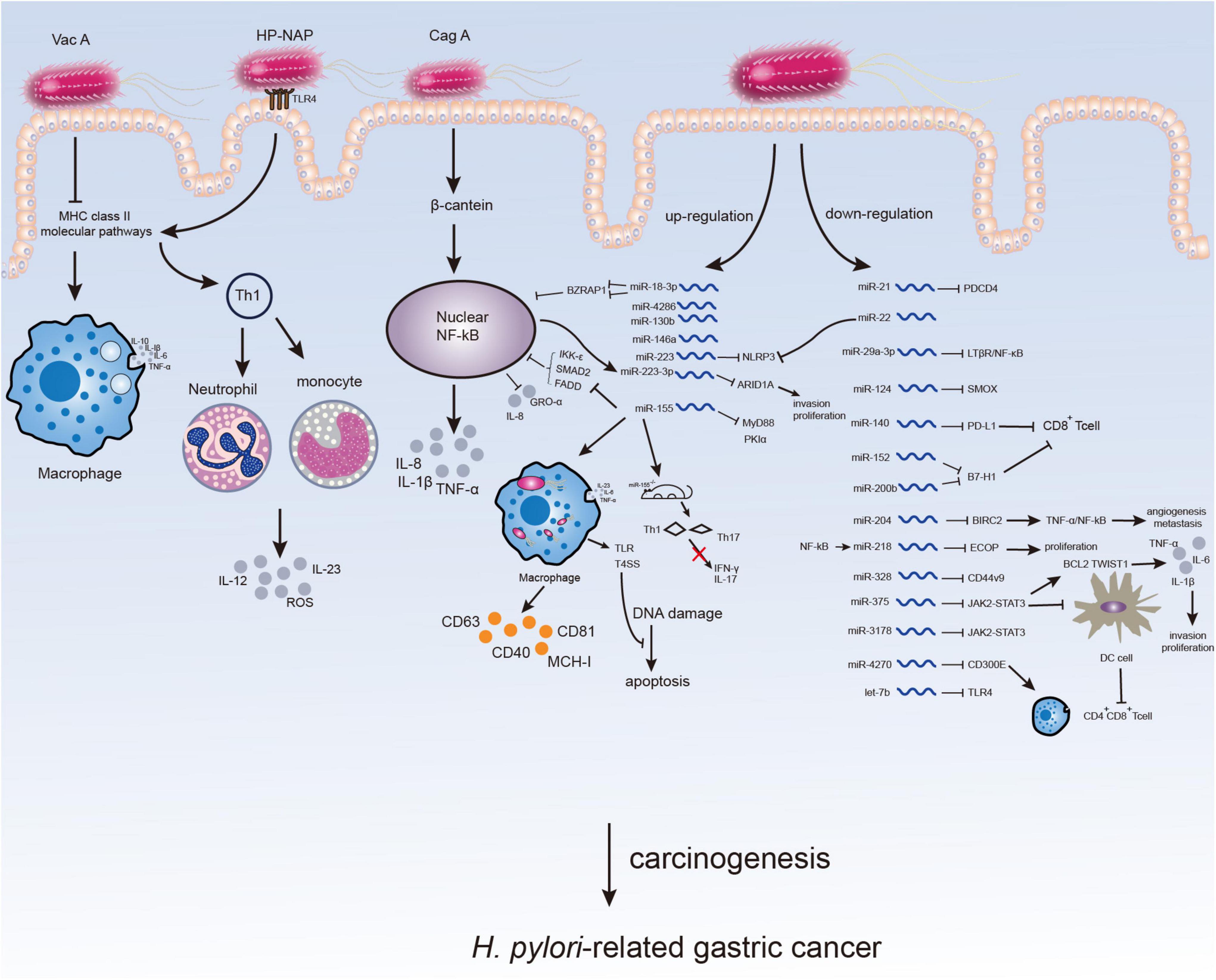
Figure 1. Microenvironment changes in H. pylori-infected gastric mucosa. The virulence factors of H. pylori can affect immune response or inflammation of host inducing H. pylori-related gastric carcinogenesis. The participation of H. pylori may not only directly through virulence factors but also by altering the expression of ncRNAs especially miRNAs, which might serve as potential therapeutic targets for H. pylori-related GC.
LncRNAs and Helicobacter pylori-related gastric cancer
LncRNAs are long ncRNAs with more than 200 nucleotides in length. They play vital roles in cellular process despite not encoding proteins (73, 74). LncRNAs have been widely proven to be associated with GC genesis and development by affecting biological function (75).
It has been preliminarily explored for the regulatory relationship with mechanisms of lncRNAs in H. pylori-related GC. However, few studies have focused on immunity and inflammation, which is ought to be future research direction in this field. LncRNAs were demonstrated to be implicated in viral infection recently (76). In H. pylori-infected GC, a decreased expression of TNF-α, IL-1β, and IL-8 was detected after knockdown of plasmacytoma variant translocation 1 (lncPVT1) and the migration of GC cells was inhibited, suggesting that lncPVT1 might activate immune function to affect GC caused by H. pylori (77). Similar to this result, another study reported that the overexpression of lncRNA H19 induced inflammatory reaction via the NF-κB pathway, released pro-inflammatory cytokines including TNF-α, IL-1β, IL-6, and IL-8, then promoted GC cell proliferation, migration and invasion infected with H. pylori (78). Besides, circulating lncRNA H19 expression was significantly increased in H. pylori-positive peptic ulcer patients and further increased in GC patients statistically positively correlated with the levels of TNF-α, IFN-γ, and gastrin. Hence, lncRNA H19 could be applied to distinguishing GC from peptic ulcer with positive H. pylori (79). LncRNA SGK1 was found to be elevated in T cells of H. pylori-related GC, induce Th2 and Th17 cells, reduce Th1 cell differentiation through the SGK1/JunB signaling pathway and be associated with poor prognosis of H. pylori-infected GC (80). LncRNAs may also affect the progression of H. pylori-related MALT. It was shown that lncRNA GHRLOS exhibited significant change in gastric MALT patients, which had differential expression both in H. pylori-positive gastritis and GC tissue (81). That might be a sound evidence for lncRNAs as important factors in the development of gastric malignancies. The above findings indicated that lncRNAs might participate in gastric malignant tumors induced by H. pylori with the potential to be predictive biomarkers.
Other than independent effects, lncRNAs can also interact with miRNAs forming ceRNA networks to jointly regulate H. pylori. MiR-375 has been identified as an inhibitor of H. pylori-related GC, and the expression of lncRNA SOX2OT was down-regulated after miR-375 overexpression, suggesting the relationship of co-regulation between lncRNAs and miRNAs on H. pylori-related GC (82). LncRNA HOXA-AS3 is elevated in GC relevant with H. pylori infection. It negatively regulates miR-29a-3p and inhibits the downstream target gene LTβR regulating the NF-κB pathway to affect GC cell migration, proliferation, metastasis and invasion (83). CDK2, a negative regulator of T cells, was determined to form a cross-network with lncRNAs and miRNAs integrating lncRNA-TF-mRNA and ceRNA networks related to H. pylori, regulating immune microenvironment with pathogenic roles of H. pylori (84). A ceRNA network constituted by the lncRNA-RP11-1094M14.8/miR-1269a/CXCL9 axis was revealed to be linked to a variety of immune cells via CXCL9, making it a potential target for GC with different degrees of immune cell infiltration (85).
LncRNAs can not only influence immune function directly by themselves but also affect the carcinogenesis of H. pylori by forming ceRNA networks with miRNAs to jointly regulate H. pylori-related tumor microenvironment.
CircRNAs and Helicobacter pylori-related gastric cancer
CircRNAs are recognized to form a covalently closed loop structure by unique reverse splicing with the lack of terminal 5′ cap and 3′ polyadenylated tail (86). Due to their structural property, circRNAs have a high level of stability and tissue-specificity in physiological environment of eukaryotes. Most circRNAs are aberrantly expressed in pathological conditions such as cancer (87), which are also potential markers for disease progression.
The regulatory relationship of circRNAs with GC has been extensively studied. In recent years, circRNAs have shown promise as biomarkers for cancer diagnosis and prognosis especially for early cancer detection (88, 89). CircSOBP is closely associated with GC metastasis and poor survival of 5-year follow-up (90). CircARID1A regulates GC proliferation by forming an RNA-protein ternary complex with IGF2BP3 and SLC7A5, thus the circARID1A-IGF2BP3-SLC7A5 axis could be a novel therapeutic target for GC (91). The circ0008287/miR-548c-3p/CLIC1 axis promotes cell apoptosis and immune escape by impairing the function of CD8 + T cells in GC (92). CircEIF4G3 can inhibit GC cell proliferation and metastasis by regulating the miR-4449/SIK1 axis (93). Moreover, circ0002360 up-regulates PDLIM4 expression by sponging miR-629-3p (94). All these reports suggested the great potential of circRNAs to be prognostic biomarkers and therapeutic targets for GC.
However, the association of circRNAs with H. pylori and H. pylori-related GC remains rarely explored. Only a few studies presented that circRNAs might regulate the biological function or prognosis of H. pylori-related GC independently or by networks with miRNAs (95). H. pylori infection was found to increase circFNDC3B expression. In early gastric cancer (EGC) patients treated with endoscopic submucosal dissection (ESD), the expression of miR-942 and miR-510 was suppressed while their target genes CD44 and CDH1 were increased in the group with high circFNDC3B expression when compared with the low expression group, which contributed to a higher recurrence rate in EGC patients consequently (96). CD44 was considered as a stem cell-like cancer cell marker affecting EGC initiation with H. pylori infection (97). Earlier studies also confirmed that circFNDC3B could mediate GC cell migration and invasion by promoting epithelial-mesenchymal transition (EMT) (98). Additionally, H. pylori can upregulate circMAN1A2 expression in GC cells, and circMAN1A2 may promote proliferation, migration and invasion of H. pylori-induced GC by sponging miR-1236-3p to increase MTA2 expression (99).
In spite of the previous research on circRNAs associated with H. pylori-related GC, further investigations are needed to support circRNAs in serving as diagnostic biomarkers and therapeutic targets for H. pylori-related GC.
Other ncRNAs and Helicobacter pylori-related gastric cancer
Except for the highly studied miRNAs, lncRNAs, and circRNAs, ncRNAs also contain snoRNAs and piRNAs. The research on them could also be conducive to deeply understanding the association of ncRNAs with H. pylori-related GC although the known mechanisms are limited. H. pylori was suggested to be delivered into host cells by two snoRNAs enriched in outer membrane vesicles of bacteria including sR-2509025 and sR-989262, reduce LPS stimulation and inhibit IL-8 secretion, thereby mediating immune escape (100). The association of other ncRNAs with H. pylori-related GC worth further exploration (Figure 2).
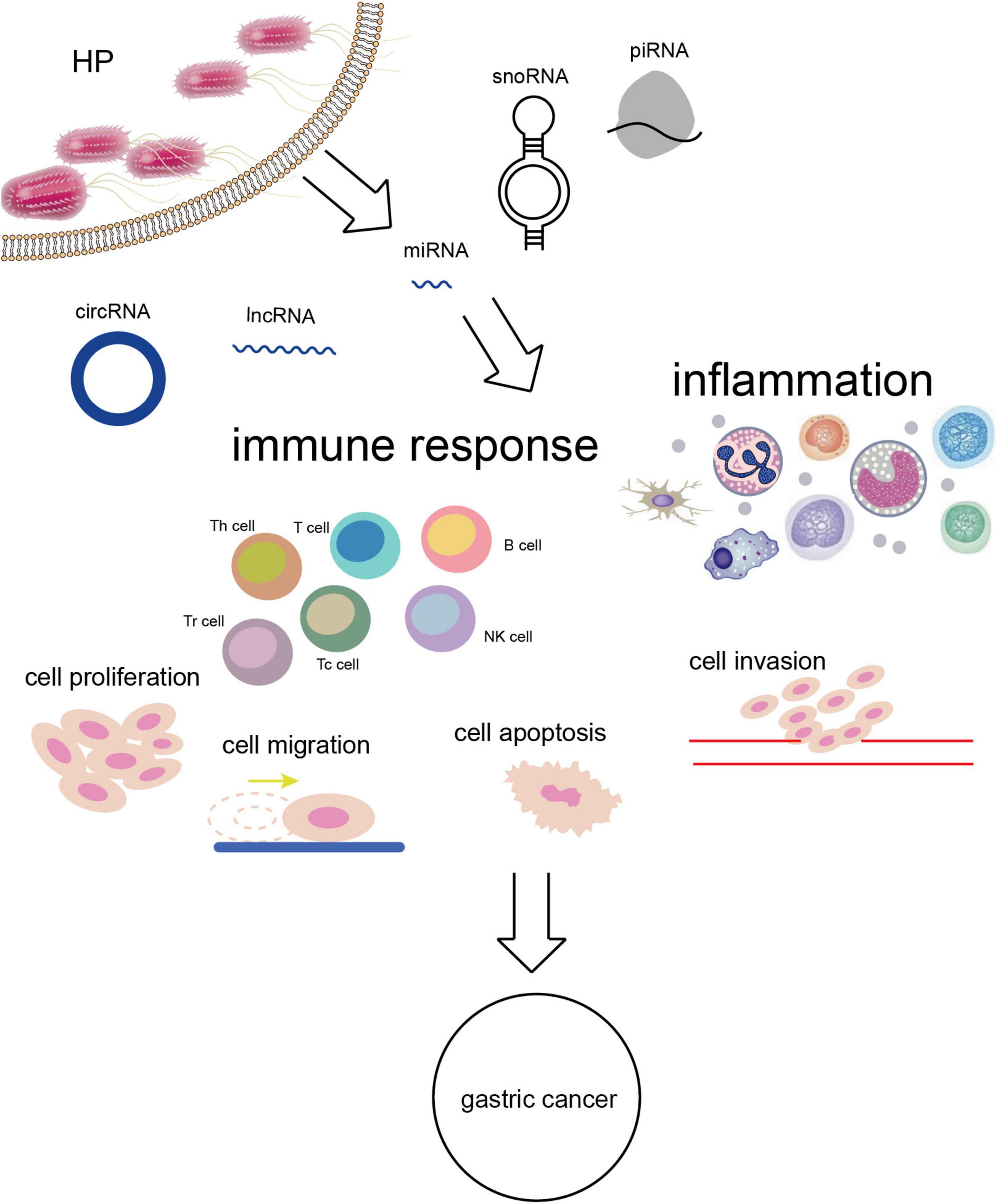
Figure 2. H. pylori infection alters the expression of ncRNAs leading to a series of biological effects in vivo and GC eventually.
Summary
NcRNAs play critical roles in transcriptome with regulatory function in all aspects of physiological process, pathological process and disease progression. As one of the important pathogenic factors of GC, the association of H. pylori with ncRNAs has been extensively studied. NcRNAs have been clarified as a key link from persistent infection of H. pylori to GC and to make profound impacts despite the specific mechanisms to be confirmed. The regulation of ncRNAs on the immune microenvironment of body after H. pylori infection could be a therapeutic target for H. pylori in the future. The present review elaborated the bridge role of ncRNAs between H. pylori and GC from the perspective of immune inflammatory response, indicating that ncRNAs held promise as biomarkers for the early diagnosis, prognosis, and treatment of H. pylori-related GC. However, relevant exact molecular mechanisms need to be verified and more clinical data should be involved as additional evidence to the prognosis study, aiming to improve the survival and life quality of H. pylori-related GC patients.
Author contributions
B-GW, Y-KL, and ZL conceived and designed this study and revised the manuscript. A-RL and L-YJ collected the data for the review. A-RL and Z-WY wrote the manuscript. All authors contributed to the article and approved the submitted version.
Funding
This research was supported by the Natural Science Foundation of Liaoning Province (2022-YGJC-59 and 2022-MS-196).
Conflict of interest
The authors declare that the research was conducted in the absence of any commercial or financial relationships that could be construed as a potential conflict of interest.
Publisher’s note
All claims expressed in this article are solely those of the authors and do not necessarily represent those of their affiliated organizations, or those of the publisher, the editors and the reviewers. Any product that may be evaluated in this article, or claim that may be made by its manufacturer, is not guaranteed or endorsed by the publisher.
References
1. Sung H, Ferlay J, Siegel RL, Laversanne M, Soerjomataram I, Jemal A, et al. Global cancer statistics 2020: GLOBOCAN estimates of incidence and mortality worldwide for 36 Cancers in 185 countries. CA Cancer J Clin. (2021) 71:209–49.
2. Wang F, Meng W, Wang B, Qiao L. Helicobacter Pylori-induced gastric inflammation and gastric cancer. Cancer Lett. (2014) 345:196–202.
4. Bravo D, Hoare A, Soto C, Valenzuela MA, Quest AF. Helicobacter pylori in human health and disease: mechanisms for local gastric and systemic effects. World J Gastroenterol. (2018) 24:3071–89. doi: 10.3748/wjg.v24.i28.3071
5. Diaz P, Valenzuela Valderrama M, Bravo J, Quest AFG. Helicobacter pylori and gastric cancer: adaptive cellular mechanisms involved in disease progression. Front Microbiol. (2018) 9:5. doi: 10.3389/fmicb.2018.00005
6. Bagheri N, Azadegan-Dehkordi F, Rafieian-Kopaei M, Rahimian G, Asadi-Samani M, Shirzad H. Clinical relevance of Helicobacter pylori virulence factors in Iranian patients with gastrointestinal diseases. Microb Pathog. (2016) 100:154–62.
7. Blosse A, Levy M, Robe C, Staedel C, Copie-Bergman C, Lehours P. Deregulation of miRNA in Helicobacter pylori-induced gastric MALT lymphoma: from mice to human. J Clin Med. (2019) 8:845. doi: 10.3390/jcm8060845
8. Espinoza JL, Matsumoto A, Tanaka H, Matsumura I. Gastric microbiota: an emerging player in Helicobacter pylori-induced gastric malignancies. Cancer Lett. (2018) 414:147–52. doi: 10.1016/j.canlet.2017.11.009
9. Suzuki M, Mimuro H, Kiga K, Fukumatsu M, Ishijima N, Morikawa H, et al. Helicobacter pylori CagA phosphorylation-independent function in epithelial proliferation and inflammation. Cell Host Microbe. (2009) 5:23–34. doi: 10.1016/j.chom.2008.11.010
10. Deng J, Miller SA, Wang HY, Xia W, Wen Y, Zhou BP, et al. beta-catenin interacts with and inhibits NF-kappa B in human colon and breast cancer. Cancer Cell. (2002) 2:323–34.
11. Supajatura V, Ushio H, Wada A, Yahiro K, Okumura K, Ogawa H, et al. Cutting edge: VacA, a vacuolating cytotoxin of Helicobacter pylori, directly activates mast cells for migration and production of proinflammatory cytokines. J Immunol. (2002) 168:2603–7. doi: 10.4049/jimmunol.168.6.2603
12. Amedei A, Cappon A, Codolo G, Cabrelle A, Polenghi A, Benagiano M, et al. The neutrophil-activating protein of Helicobacter pylori promotes Th1 immune responses. J Clin Invest. (2006) 116:1092–101. doi: 10.1172/JCI27177
13. Toh JWT, Wilson RB. Pathways of gastric carcinogenesis, Helicobacter pylori virulence and interactions with antioxidant systems, vitamin C and phytochemicals. Int J Mol Sci. (2020) 21:6451. doi: 10.3390/ijms21176451
14. Fehlings M, Drobbe L, Moos V, Renner Viveros P, Hagen J, Beigier-Bompadre M, et al. Comparative analysis of the interaction of Helicobacter pylori with human dendritic cells, macrophages, and monocytes. Infect Immun. (2012) 80:2724–34. doi: 10.1128/IAI.00381-12
15. Adams BD, Parsons C, Walker L, Zhang WC, Slack FJ. Targeting noncoding RNAs in disease. J Clin Invest. (2017) 127:761–71.
16. Deveson IW, Hardwick SA, Mercer TR, Mattick JS. The dimensions, dynamics, and relevance of the mammalian noncoding transcriptome. Trends Genet. (2017) 33:464–78. doi: 10.1016/j.tig.2017.04.004
17. Friedman RC, Farh KK, Burge CB, Bartel DP. Most mammalian mRNAs are conserved targets of microRNAs. Genome Res. (2009) 19:92–105.
18. Rupaimoole R, Slack FJ. MicroRNA therapeutics: towards a new era for the management of cancer and other diseases. Nat Rev Drug Discov. (2017) 16:203–22. doi: 10.1038/nrd.2016.246
19. Qi X, Lin Y, Chen J, Shen B. Decoding competing endogenous RNA networks for cancer biomarker discovery. Brief Bioinform. (2020) 21:441–57. doi: 10.1093/bib/bbz006
21. Esquela-Kerscher A, Slack FJ. Oncomirs - microRNAs with a role in cancer. Nat Rev Cancer. (2006) 6:259–69.
22. Banerjee S, Thompson WE, Chowdhury I. Emerging roles of microRNAs in the regulation of toll-like receptor (TLR)-signaling. Front Biosci (Landmark Ed). (2021) 26:771–96. doi: 10.2741/4917
23. Huang WT, Kuo SH, Kuo YC, Lin CW. miR-155-regulated mTOR and toll-like receptor 5 in gastric diffuse large B-cell lymphoma. Cancer Med. (2022) 11:555–70. doi: 10.1002/cam4.4466
24. Pernitzsch SR, Alzheimer M, Bremer BU, Robbe-Saule M, De Reuse H, Sharma CM. Small RNA mediated gradual control of lipopolysaccharide biosynthesis affects antibiotic resistance in Helicobacter pylori. Nat Commun. (2021) 12:4433. doi: 10.1038/s41467-021-24689-2
25. Zhu XL, Ren LF, Wang HP, Bai ZT, Zhang L, Meng WB, et al. Plasma microRNAs as potential new biomarkers for early detection of early gastric cancer. World J Gastroenterol. (2019) 25:1580–91.
26. Ghafouri-Fard S, Vafaee R, Shoorei H, Taheri M. MicroRNAs in gastric cancer: biomarkers and therapeutic targets. Gene. (2020) 757:144937.
27. Vinchure OS, Kulshreshtha R. miR-490: a potential biomarker and therapeutic target in cancer and other diseases. J Cell Physiol. (2021) 236:3178–93. doi: 10.1002/jcp.30119
28. ZiaSarabi P, Sorayayi S, Hesari A, Ghasemi F. Circulating microRNA-133, microRNA-17 and microRNA-25 in serum and its potential diagnostic value in gastric cancer. J Cell Biochem. (2019) 120:12376–81. doi: 10.1002/jcb.28503
29. Link A, Schirrmeister W, Langner C, Varbanova M, Bornschein J, Wex T, et al. Differential expression of microRNAs in preneoplastic gastric mucosa. Sci Rep. (2015) 5:8270.
30. Ou Y, Ren H, Zhao R, Song L, Liu Z, Xu W, et al. Helicobacter pylori CagA promotes the malignant transformation of gastric mucosal epithelial cells through the dysregulation of the miR-155/KLF4 signaling pathway. Mol Carcinog. (2019) 58:1427–37. doi: 10.1002/mc.23025
31. Wan J, Xia L, Xu W, Lu N. Expression and function of miR-155 in diseases of the gastrointestinal tract. Int J Mol Sci. (2016) 17:709.
32. Pachathundikandi SK, Blaser N, Backert S. Mechanisms of inflammasome signaling, microRNA induction and resolution of inflammation by Helicobacter pylori. Curr Top Microbiol Immunol. (2019) 421:267–302. doi: 10.1007/978-3-030-15138-6_11
33. Mahbobi R, Fallah F, Behmanesh A, Yadegar A, Hakemi-Vala M, Ehsanzadeh SJ, et al. Helicobacter pylori infection mediates inflammation and tumorigenesis-associated genes through miR-155-5p: an integrative omics and bioinformatics-based investigation. Curr Microbiol. (2022) 79:192. doi: 10.1007/s00284-022-02880-y
34. Fassi Fehri L, Koch M, Belogolova E, Khalil H, Bolz C, Kalali B, et al. Helicobacter pylori induces miR-155 in T cells in a cAMP-Foxp3-dependent manner. PLoS One. (2010) 5:e9500. doi: 10.1371/journal.pone.0009500
35. Wang J, Deng Z, Wang Z, Wu J, Gu T, Jiang Y, et al. MicroRNA-155 in exosomes secreted from Helicobacter pylori infection macrophages immunomodulates inflammatory response. Am J Transl Res. (2016) 8:3700–9.
36. Wu K, Zhu C, Yao Y, Wang X, Song J, Zhai J. MicroRNA-155-enhanced autophagy in human gastric epithelial cell in response to Helicobacter pylori. Saudi J Gastroenterol. (2016) 22:30–6. doi: 10.4103/1319-3767.173756
37. Oertli M, Engler DB, Kohler E, Koch M, Meyer TF, Muller A. MicroRNA-155 is essential for the T cell-mediated control of Helicobacter pylori infection and for the induction of chronic gastritis and colitis. J Immunol. (2011) 187:3578–86. doi: 10.4049/jimmunol.1101772
38. Koch M, Mollenkopf HJ, Klemm U, Meyer TF. Induction of microRNA-155 is TLR- and type IV secretion system-dependent in macrophages and inhibits DNA-damage induced apoptosis. Proc Natl Acad Sci USA. (2012) 109:E1153–62. doi: 10.1073/pnas.1116125109
39. Xiao B, Liu Z, Li BS, Tang B, Li W, Guo G, et al. Induction of microRNA-155 during Helicobacter pylori infection and its negative regulatory role in the inflammatory response. J Infect Dis. (2009) 200:916–25. doi: 10.1086/605443
40. Tang B, Xiao B, Liu Z, Li N, Zhu ED, Li BS, et al. Identification of MyD88 as a novel target of miR-155, involved in negative regulation of Helicobacter pylori-induced inflammation. FEBS Lett. (2010) 584:1481–6. doi: 10.1016/j.febslet.2010.02.063
41. Liu TY, Chen SU, Kuo SH, Cheng AL, Lin CW. E2A-positive gastric MALT lymphoma has weaker plasmacytoid infiltrates and stronger expression of the memory B-cell-associated miR-223: possible correlation with stage and treatment response. Mod Pathol. (2010) 23:1507–17. doi: 10.1038/modpathol.2010.139
42. Saha K, Sarkar D, Khan U, Karmakar BC, Paul S, Mukhopadhyay AK, et al. Capsaicin inhibits inflammation and gastric damage during H pylori infection by targeting NF-kB-miRNA Axis. Pathogens. (2022) 11:641. doi: 10.3390/pathogens11060641
43. Vasapolli R, Venerito M, Schirrmeister W, Thon C, Weigt J, Wex T, et al. Inflammatory microRNAs in gastric mucosa are modulated by Helicobacter pylori infection and proton-pump inhibitors but not by aspirin or NSAIDs. PLoS One. (2021) 16:e0249282. doi: 10.1371/journal.pone.0249282
44. Yang F, Xu Y, Liu C, Ma C, Zou S, Xu X, et al. NF-kappaB/miR-223-3p/ARID1A axis is involved in Helicobacter pylori CagA-induced gastric carcinogenesis and progression. Cell Death Dis. (2018) 9:12. doi: 10.1038/s41419-017-0020-9
45. Pachathundikandi SK, Backert S. Helicobacter pylori controls NLRP3 expression by regulating hsa-miR-223-3p and IL-10 in cultured and primary human immune cells. Innate Immun. (2018) 24:11–23. doi: 10.1177/1753425917738043
46. Matsushima K, Isomoto H, Inoue N, Nakayama T, Hayashi T, Nakayama M, et al. MicroRNA signatures in Helicobacter pylori-infected gastric mucosa. Int J Cancer. (2011) 128:361–70.
47. Zingone F, Pilotto V, Cardin R, Maddalo G, Orlando C, Fassan M, et al. Autoimmune atrophic gastritis: the role of miRNA in relation to Helicobacter pylori infection. Front Immunol. (2022) 13:930989. doi: 10.3389/fimmu.2022.930989
48. Gong R, Han R, Zhuang X, Tang W, Xu G, Zhang L, et al. MiR-375 mitigates retinal angiogenesis by depressing the JAK2/STAT3 pathway. Aging (Albany NY). (2022) 14:6594–604. doi: 10.18632/aging.204232
49. Li L, Xiao C, He K, Xiang G. Circ_0072088 promotes progression of hepatocellular carcinoma by activating JAK2/STAT3 signaling pathway via miR-375. IUBMB Life. (2021) 73:1153–65. doi: 10.1002/iub.2520
50. Yan XL, Luo QY, Zhou SN, Pan WT, Zhang L, Yang DJ, et al. MicroRNA-375 reverses the expression of PD-L1 by inactivating the JAK2/STAT3 signaling pathways in gastric cancer. Clin Res Hepatol Gastroenterol. (2021) 45:101574. doi: 10.1016/j.clinre.2020.10.015
51. Zhao Q, Liu Y, Wang T, Yang Y, Ni H, Liu H, et al. MiR-375 inhibits the stemness of breast cancer cells by blocking the JAK2/STAT3 signaling. Eur J Pharmacol. (2020) 884:173359. doi: 10.1016/j.ejphar.2020.173359
52. Zhang Z, Chen S, Fan M, Ruan G, Xi T, Zheng L, et al. Helicobacter pylori induces gastric cancer via down-regulating miR-375 to inhibit dendritic cell maturation. Helicobacter. (2021) 26:e12813. doi: 10.1111/hel.12813
53. Miao L, Liu K, Xie M, Xing Y, Xi T. miR-375 inhibits Helicobacter pylori-induced gastric carcinogenesis by blocking JAK2-STAT3 signaling. Cancer Immunol Immunother. (2014) 63:699–711. doi: 10.1007/s00262-014-1550-y
54. Rossi AF, Cadamuro AC, Biselli-Perico JM, Leite KR, Severino FE, Reis PP, et al. Interaction between inflammatory mediators and miRNAs in Helicobacter pylori infection. Cell Microbiol. (2016) 18:1444–58.
55. Li N, Wang J, Yu W, Dong K, You F, Si B, et al. MicroRNA146a inhibits the inflammatory responses induced by interleukin17A during the infection of Helicobacter pylori. Mol Med Rep. (2019) 19:1388–95.
56. Oana SM, Claudia B, Lelia RA, Simona M, Claudia C, Daniela DE. Differential expression of tissular miRNA-155 in pediatric gastritis. J Clin Med. (2022) 11:3351. doi: 10.3390/jcm11123351
57. Cortes-Marquez AC, Mendoza-Elizalde S, Arenas-Huertero F, Trillo-Tinoco J, Valencia-Mayoral P, Consuelo-Sanchez A, et al. Differential expression of miRNA-146a and miRNA-155 in gastritis induced by Helicobacter pylori infection in paediatric patients, adults, and an animal model. BMC Infect Dis. (2018) 18:463. doi: 10.1186/s12879-018-3368-2
58. Chen P, Guo H, Wu X, Li J, Duan X, Ba Q, et al. Epigenetic silencing of microRNA-204 by Helicobacter pylori augments the NF-kappaB signaling pathway in gastric cancer development and progression. Carcinogenesis. (2020) 41:430–41. doi: 10.1093/carcin/bgz143
59. Tsai CC, Chen TY, Tsai KJ, Lin MW, Hsu CY, Wu DC, et al. NF-kappaB/miR-18a-3p and miR-4286/BZRAP1 axis may mediate carcinogenesis in Helicobacter pylori-associated gastric cancer. Biomed Pharmacother. (2020) 132:110869. doi: 10.1016/j.biopha.2020.110869
60. Gao C, Zhang Z, Liu W, Xiao S, Gu W, Lu H. Reduced microRNA-218 expression is associated with high nuclear factor kappa B activation in gastric cancer. Cancer. (2010) 116:41–9. doi: 10.1002/cncr.24743
61. Zou, M, Wang F, Jiang A, Xia A, Kong S, Gong C, et al. MicroRNA-3178 ameliorates inflammation and gastric carcinogenesis promoted by Helicobacter pylori new toxin, Tip-alpha, by targeting TRAF3. Helicobacter. (2017) 22:e12348. doi: 10.1111/hel.12348
62. Teng GG, Wang WH, Dai Y, Wang SJ, Chu YX, Li J. Let-7b is involved in the inflammation and immune responses associated with Helicobacter pylori infection by targeting Toll-like receptor 4. PLoS One. (2013) 8:e56709. doi: 10.1371/journal.pone.0056709
63. Ding L, Li Q, Chakrabarti J, Munoz A, Faure-Kumar E, Ocadiz-Ruiz R, et al. MiR130b from Schlafen4(+) MDSCs stimulates epithelial proliferation and correlates with preneoplastic changes prior to gastric cancer. Gut. (2020) 69:1750–61. doi: 10.1136/gutjnl-2019-318817
64. Zhao M, Liu Q, Liu W, Zhou H, Zang X, Lu J. MicroRNA140 suppresses Helicobacter pyloripositive gastric cancer growth by enhancing the antitumor immune response. Mol Med Rep. (2019) 20:2484–92.
65. Xie G, Li W, Li R, Wu K, Zhao E, Zhang Y, et al. Helicobacter pylori promote B7-H1 expression by suppressing miR-152 and miR-200b in gastric cancer cells. PLoS One. (2017) 12:e0168822. doi: 10.1371/journal.pone.0168822
66. Kalani M, Hodjati H, Ghoddusi Johari H, Doroudchi M. Memory T cells of patients with abdominal aortic aneurysm differentially expressed micro RNAs 21, 92a, 146a, 155, 326 and 663 in response to Helicobacter pylori and Lactobacillus acidophilus. Mol Immunol. (2021) 130:77–84. doi: 10.1016/j.molimm.2020.11.007
67. Zhu Y, Liu L, Hu L, Dong W, Zhang M, Liu Y, et al. Effect of Celastrus orbiculatus in inhibiting Helicobacter pylori induced inflammatory response by regulating epithelial mesenchymal transition and targeting miR-21/PDCD4 signaling pathway in gastric epithelial cells. BMC Complement Altern Med. (2019) 19:91. doi: 10.1186/s12906-019-2504-x
68. Murray-Stewart T, Sierra JC, Piazuelo MB, Mera RM, Chaturvedi R, Bravo LE, et al. Epigenetic silencing of miR-124 prevents spermine oxidase regulation: implications for Helicobacter pylori-induced gastric cancer. Oncogene. (2016) 35:5480–8. doi: 10.1038/onc.2016.91
69. Zheng L, Wu Y, Shen L, Liang X, Yang Z, Li S, et al. Mechanisms of JARID1B Up-regulation and its role in Helicobacter pylori-induced gastric carcinogenesis. Front Oncol. (2021) 11:757497. doi: 10.3389/fonc.2021.757497
70. Pagliari M, Munari F, Toffoletto M, Lonardi S, Chemello F, Codolo G, et al. Helicobacter pylori affects the antigen presentation activity of macrophages modulating the expression of the immune receptor CD300E through miR-4270. Front Immunol. (2017) 8:1288. doi: 10.3389/fimmu.2017.01288
71. Ishimoto T, Izumi D, Watanabe M, Yoshida N, Hidaka K, Miyake K, et al. Chronic inflammation with Helicobacter pylori infection is implicated in CD44 overexpression through miR-328 suppression in the gastric mucosa. J Gastroenterol. (2015) 50:751–7. doi: 10.1007/s00535-014-1019-y
72. Li S, Liang X, Ma L, Shen L, Li T, Zheng L, et al. MiR-22 sustains NLRP3 expression and attenuates H. pylori-induced gastric carcinogenesis. Oncogene. (2018) 37:884–96. doi: 10.1038/onc.2017.381
73. Sirey TM, Roberts K, Haerty W, Bedoya-Reina O, Rogatti-Granados S, Tan JY, et al. The long non-coding RNA Cerox1 is a post transcriptional regulator of mitochondrial complex I catalytic activity. Elife. (2019) 8:e45051.
74. Delas MJ, Sabin LR, Dolzhenko E, Knott SR, Munera Maravilla E, Jackson BT, et al. lncRNA requirements for mouse acute myeloid leukemia and normal differentiation. Elife. (2017) 6:e25607. doi: 10.7554/eLife.25607
75. Xin Z, Zhang L, Liu M, Wang Y, Zhang Y, Zhao W, et al. Helicobacter pylori infection-related long non-coding RNA signatures predict the prognostic status for gastric cancer patients. Front Oncol. (2021) 11:709796. doi: 10.3389/fonc.2021.709796
76. Wang Y, Huang L, Wang Y, Luo W, Li F, Xiao J, et al. Single-cell RNA-sequencing analysis identifies host long noncoding RNA MAMDC2-AS1 as a co-factor for HSV-1 nuclear transport. Int J Biol Sci. (2020) 16:1586–603. doi: 10.7150/ijbs.42556
77. Jing XH, Li LX, Han TT, Shi J. [Effects of long non-coding RNA plasmacytoma variant translocation 1 gene on inflammatory response and cell migration in Helicobacter pylori infected gastric epithelial cell line]. Zhongguo Yi Xue Ke Xue Yuan Xue Bao. (2020) 42:228–35.
78. Zhang Y, Yan J, Li C, Wang X, Dong Y, Shen X, et al. LncRNA H19 induced by Helicobacter pylori infection promotes gastric cancer cell growth via enhancing NF-kappaB-induced inflammation. J Inflamm (Lond). (2019) 16:23. doi: 10.1186/s12950-019-0226-y
79. Mohamed WA, Schaalan MF, Ramadan B. The expression profiling of circulating miR-204, miR-182, and lncRNA H19 as novel potential biomarkers for the progression of peptic ulcer to gastric cancer. J Cell Biochem. (2019) 120:13464–77. doi: 10.1002/jcb.28620
80. Yao Y, Jiang Q, Jiang L, Wu J, Zhang Q, Wang J, et al. Lnc-SGK1 induced by Helicobacter pylori infection and highsalt diet promote Th2 and Th17 differentiation in human gastric cancer by SGK1/Jun B signaling. Oncotarget. (2016) 7:20549–60. doi: 10.18632/oncotarget.7823
81. Zhang J, Wei J, Wang Z, Feng Y, Wei Z, Hou X, et al. Transcriptome hallmarks in Helicobacter pylori infection influence gastric cancer and MALT lymphoma. Epigenomics. (2020) 12:661–71. doi: 10.2217/epi-2019-0152
82. Shafiee M, Aleyasin SA, Mowla SJ, Vasei M, Yazdanparast SA. The effect of MicroRNA-375 overexpression, an inhibitor of Helicobacter pylori-induced carcinogenesis, on lncRNA SOX2OT. Jundishapur J Microbiol. (2016) 9:e23464. doi: 10.5812/jjm.23464
83. Qu F, Zhu B, Hu YL, Mao QS, Feng Y. LncRNA HOXA-AS3 promotes gastric cancer progression by regulating miR-29a-3p/LTbetaR and activating NF-kappaB signaling. Cancer Cell Int. (2021) 21:118. doi: 10.1186/s12935-021-01827-w
84. Liu S, Yin H, Zheng S, Chu A, Li Y, Xing C, et al. Differentially expressed mRNAs and their long noncoding RNA regulatory network with Helicobacter pylori-associated diseases including atrophic gastritis and gastric cancer. Biomed Res Int. (2020) 2020:3012193. doi: 10.1155/2020/3012193
85. Zhang K, Zhang L, Mi Y, Tang Y, Ren F, Liu B, et al. A ceRNA network and a potential regulatory axis in gastric cancer with different degrees of immune cell infiltration. Cancer Sci. (2020) 111:4041–50. doi: 10.1111/cas.14634
88. Kristensen LS, Jakobsen T, Hager H, Kjems J. The emerging roles of circRNAs in cancer and oncology. Nat Rev Clin Oncol. (2022) 19:188–206.
89. Ghafouri-Fard S, Honarmand Tamizkar K, Jamali E, Taheri M, Ayatollahi SA. Contribution of circRNAs in gastric cancer. Pathol Res Pract. (2021) 227:153640.
90. Yan, X, Wang J, Bai Y. Potentials of circSOBP in the diagnosis and prognosis of gastric cancer. Scand J Gastroenterol. (2022):1–5. doi: 10.1080/00365521.2022.2088246
91. Ma Q, Yang F, Huang B, Pan X, Li W, Yu T, et al. CircARID1A binds to IGF2BP3 in gastric cancer and promotes cancer proliferation by forming a circARID1A-IGF2BP3-SLC7A5 RNA-protein ternary complex. J Exp Clin Cancer Res. (2022) 41:251. doi: 10.1186/s13046-022-02466-3
92. Li B, Liang L, Chen Y, Liu J, Wang Z, Mao Y, et al. Circ_0008287 promotes immune escape of gastric cancer cells through impairing microRNA-548c-3p-dependent inhibition of CLIC1. Int Immunopharmacol. (2022) 111:108918. doi: 10.1016/j.intimp.2022.108918
93. Zang X, Jiang J, Gu J, Chen Y, Wang M, Zhang Y, et al. Circular RNA EIF4G3 suppresses gastric cancer progression through inhibition of beta-catenin by promoting delta-catenin ubiquitin degradation and upregulating SIK1. Mol Cancer. (2022) 21:141. doi: 10.1186/s12943-022-01606-9
94. Yu Z, Lan J, Li W, Jin L, Qi F, Yu C, et al. Circular RNA hsa_circ_0002360 promotes proliferation and invasion and inhibits oxidative stress in gastric cancer by sponging miR-629-3p and regulating the PDLIM4 expression. Oxid Med Cell Longev. (2022) 2022:2775433. doi: 10.1155/2022/2775433
95. Wu Z, Liu P, Zhang G. Identification of circRNA-miRNA-immune-related mRNA regulatory network in gastric cancer. Front Oncol. (2022) 12:816884. doi: 10.3389/fonc.2022.816884
96. Zhang J, Bai J, Zhu H, Li W, An Q, Wang D. The upregulation of circFNDC3B aggravates the recurrence after endoscopic submucosal dissection (ESD) in early gastric cancer (EGC) patients. Sci Rep. (2022) 12:6178. doi: 10.1038/s41598-022-07154-y
97. Takaishi S, Okumura T, Tu S, Wang SS, Shibata W, Vigneshwaran R, et al. Identification of gastric cancer stem cells using the cell surface marker CD44. Stem Cells. (2009) 27:1006–20.
98. Hong Y, Qin H, Li Y, Zhang Y, Zhuang X, Liu L, et al. FNDC3B circular RNA promotes the migration and invasion of gastric cancer cells via the regulation of E-cadherin and CD44 expression. J Cell Physiol. (2019) 234:19895–910. doi: 10.1002/jcp.28588
99. Guo R, Cui X, Li X, Zang W, Chang M, Sun Z, et al. CircMAN1A2 is upregulated by Helicobacter pylori and promotes development of gastric cancer. Cell Death Dis. (2022) 13:409. doi: 10.1038/s41419-022-04811-y
Keywords: Helicobacter pylori, non-coding RNA, H. pylori-related GC, inflammation, immune response, microRNA
Citation: Liu A-r, Yan Z-w, Jiang L-y, Lv Z, Li Y-k and Wang B-g (2022) The role of non-coding RNA in the diagnosis and treatment of Helicobacter pylori-related gastric cancer, with a focus on inflammation and immune response. Front. Med. 9:1009021. doi: 10.3389/fmed.2022.1009021
Received: 01 August 2022; Accepted: 20 September 2022;
Published: 13 October 2022.
Edited by:
Jianping Liu, Karolinska Institutet (KI), SwedenReviewed by:
Lexing Yu, National Center for Liver Cancer, ChinaXuan Su, Sun Yat-sen University Cancer Center (SYSUCC), China
Copyright © 2022 Liu, Yan, Jiang, Lv, Li and Wang. This is an open-access article distributed under the terms of the Creative Commons Attribution License (CC BY). The use, distribution or reproduction in other forums is permitted, provided the original author(s) and the copyright owner(s) are credited and that the original publication in this journal is cited, in accordance with accepted academic practice. No use, distribution or reproduction is permitted which does not comply with these terms.
*Correspondence: Ben-gang Wang, Ymd3YW5nQGNtdS5lZHUuY24=; Yan-ke Li, bGl5YW5rZTE0MzdAMTYzLmNvbQ==; Zhi Lv, emx2QGNtdS5lZHUuY24=
†These authors have contributed equally to this work