- Hunan Key Laboratory of Kidney Disease and Blood Purification, Department of Nephrology, The Second Xiangya Hospital, Central South University, Changsha, China
Background: Immunoglobulin A nephropathy (IgAN) is the most common primary glomerular disease and poses a global major public health burden. The preparation of Tripterygium wilfordii Hook F (TwHF) is widely applied for treating patients with Immunoglobulin A nephropathy in China, while the molecular mechanisms remain unclear. This study aimed to verify the therapeutic mechanism of TwHF on IgAN by undertaking a holistic network pharmacology strategy in combination with in vitro and in vivo experiments.
Methods: TwHF active ingredients and their targets were obtained via the Traditional Chinese Medicine Systems Pharmacology Database. The collection of IgAN-related target genes was collected from GeneCards and OMIM. TwHF-IgAN common targets were integrated and visualized by Cytoscape. Gene ontology (GO) and Kyoto Encyclopedia of Genes and Genomes (KEGG) analyses were performed to determine the predominant molecular mechanisms and pathways of TwHF on the treatment of IgAN. The protein-protein interaction network was constructed by the STRING online search tool, and hub genes were identified using R software. The expression of hub gene and related signaling were evaluated in TwHF-treated mice through immunohistochemistry and western blot and further validated in human mesangial cells (HMCs). In addition, Cell counting kit 8 (CCK8) and flow cytometry were used to detect the effects of TwHF on cell proliferation and cell cycle of mesangial cells.
Results: A total of 51 active ingredients were screened from TwHF and 61 overlapping targets related to IgAN were considered potential therapeutic targets, GO functions and KEGG analyses demonstrated that these genes were primarily associated with DNA-binding transcription factor binding, lipid and atherosclerosis pathway. Genes with higher degrees including AKT1, CXCL8, MMP9, PTGS2, CASP3, JUN are hub genes of TwHF against IgAN. Verification of hub gene JUN both in vitro and in vivo showed that TwHF significantly attenuated JUN phosphorylation in the kidneys of IgAN mice and aIgA1-activated HMCs, meanwhile suppressing HMCs proliferation and arresting G1-S cell cycle progression.
Conclusion: Our research strengthened the mechanisms of TwHF in treating IgAN, inhibition of JUN activation may play a pivotal role in TwHF in alleviating IgAN renal injury.
Introduction
Immunoglobulin A nephropathy (IgAN) is the most common primary glomerular disease worldwide, especially in the Asia-Pacific region, the proportion is up to 50% of primary glomerular disease in China (1–3). It is characterized by Immunoglobulin A (IgA) deposition in the glomerular mesangial region accompanied by mesangial cells proliferation and matrix expansion. Clinical manifestations are microscopic or gross hematuria, with or without various degrees of proteinuria, elevated blood pressure, and edema. About 20–40% of patients progress to end-stage renal disease (ESRD) within 20 years after diagnosis, bringing a heavy burden to the individual and the whole society (4). “Multi-hits” theory is considered to be the possible pathogenesis: the overproduction of abnormal glycosylated IgA1; autoantibodies against abnormal IgA1; IgA1 immune complexes deposited in the mesangial area; stimulation of complement, cytokines, and immune-inflammatory response leading to kidney damage (5). While there is still a lack of clear understanding of the pathogenesis of IgA nephropathy (IgAN) and short of effective therapeutics for disease progression.
Tripterygium wilfordii Hook F (TwHF) is the dried root of the genus Tripterygium wilfordii in the euonymus family, which has been used in traditional Chinese medicine for more than 2,000 years (6). As early as 1977, Professor Leishi Li used TwHF to treat various types of glomerulonephritis including IgA nephropathy, and confirmed for the first time that TwHF was beneficial for reducing haematuria and eliminating edema and gradually applied to kidney injury (7). Modern pharmaceutical technology has extracted a variety of biological components from TwHF including alkaloids, diterpenes, triterpenes, sesquiterpenes, and polysaccharides. Among them, diterpene alkaloids such as triptolide and triterpenes are the main active components with immunosuppressive, anti-inflammatory, and anti-tumor effects (8), and their preparation Tripterygium glycoside tablets (TGT) has accumulated a great deal of clinical experience in the treatment of IgAN and has shown efficiency in reducing urine protein and serum creatinine levels (9–11). However, there is still no satisfactory explanation for its pharmacological mechanism related to IgAN.
Network pharmacology is used to identify the interactive network of “compound-targets-disease,” which owns the ability to elucidate complexities for multi-components, multi-targets, and multi pathways from a systemic perspective (12, 13). Thus, we aimed to analyze the pharmacological mechanisms of TwHF involved in IgAN employing a network pharmacology approach combined with experimental research, laying a stable foundation for exploring pharmacological mechanisms of TwHF in treating IgAN.
Materials and Methods
Recognition of TwHF Active Ingredients and Correlated Targets
Traditional Chinese Medicine Systems Pharmacology Database (TCMSP, http://tcmspw.com/tcmsp.php, Lab of Systems Pharmacology, China), a unique systematic pharmacology platform that contains the relationships among drugs, targets, and diseases, was applied to identify the chemical constituents of TwHF. The ingredients with oral bioavailability (OB) ≥ 30% and the drug-likeness (DL) ≥0.18 (a suggested criterion of TCMSP database) were selected as active components for further analysis (14). Meanwhile, the target associated with active ingredients of TwHF were gathered based on the TCMSP database and further verified for official target names in the UniProt database (https://www.uniprot.org, UniProt Consortium, UK/USA/Switzerland) (15).
IgAN Targets Identification and TwHF-IgAN Targets Network Construction
GeneCards (https://www.genecards.org, LifeMap Sciences, USA) and Online Mendelian Inheritance in Man (OMIM, https://www.omim.org, Johns Hopkins University, USA) were used to search for IgAN-related targets, both of which are authoritative human gene compendium web server about genome, transcriptome, disease, and function (16, 17). The overlapping targets of TwHF ingredients and IgAN were obtained using the VennDiagram R package (bioconductor, USA), and the TwHF-IgAN targets network was visualized via Cytoscape software (version 3.7.2, https://www.cytoscape.org/, The Cytoscape Consoritum, USA).
GO and KEGG Pathway Enrichment Analyses
Gene ontology (GO) enrichment and Kyoto Encyclopedia of Genes and Genomes (KEGG) pathway analyses were used to explore the potential biological processes and utilities, cellular components, and molecular functions of overlapped targets. Significant relevant signals were identified via clusterProfiler package (bioconductor, USA) in R software (× 64 4.1.0) with p < 0.05 and q < 0.05. A protein-protein interaction (PPI) network of overlapped target genes was built based on the STRING database (https://cn.string-db.org/, STRING CONSORTIUM, UK/Switzerland/Denmark) (18). Densely connected clusters in the PPI network were visualized by R.
Experimental Animals and Cells
Female BALB/c mice were obtained from Hunan SJA Laboratory Animal Co. Ltd. (Changsha, China) and housed under pathogen-free conditions, the IgAN mouse model was induced by oral mucosal immune as previously described (19). At 11 weeks of age, mice were randomly assigned to receive TGT gavage (7 mg/kg dissolved in saline) or PBS for 6 weeks. All experiments were performed following the animal experimental guidelines issued by the Animal Care and Use Committee at the Xiangya Medical School of Central South University.
The human mesangial cells (HMCs) (Cellbio, China) were cultivated in Dulbecco's Modified Eagle's Medium (DMEM)/F-12 medium (Gibco, USA) supplemented with 10% fetal bovine serum (FBS) under 5% CO2 at 37°C. Monomeric human IgA1 (Abcam, ab91020) was heated and aggregated at 65°C for 150 min on a dry plate heater to obtain aIgA1 as previously described (20). Triptolide was purchased from ApexBio Technology (Houston, USA). Cells were incubated with aIgA1 alone or with a combination of triptolide (0–75 nM) concentrations for 24 h.
Immunohistochemistry Staining
Formaldehyde-fixed and paraffin-embedded tissue sections (4 mm thick) were used for immunohistochemistry as previously described (21). After dehydration, slides in citrate solution were subjected to a microwave antigen retrieval process. Primary antibodies were employed against the JUN (9165s, Cell Signaling Technology, USA), p-JUN (3270s, Cell Signaling Technology, USA), with periodic acid-Schiff (PAS) used instead of primary antibodies as negative controls for staining. Finally, horseradish peroxidase (HRP)-conjugated polymer (Abcam, USA) was used for the visualized detection under light microscopy (Nikon Tokyo, Japan). OD values were analyzed by ImageJ software (Media Cybernetics, USA). All the analyses were repeated no less than three times, and representative images are displayed. The use of renal specimens of IgAN patients was approved by the Ethics Committee of the Second Xiangya Hospital of Central South University according to the Declaration of Helsinki.
Western Blot Analysis
Cells and renal tissues were lysed in RIPA Lysis buffer (Beyotime Biotechnology, China) supplemented with PMSF, and protein concentration was determined by BCA assay (Thermo Fisher Scientific, USA). Proteins were subjected to 10% SDS-PAGE and transferred to PVDF membranes (Millipore, USA). The membranes were blocked with 5% bovine serum albumin (BSA) at room temperature for 1 h, and incubated overnight at 4°C with the primary antibodies: JUN (9165s, Cell Signaling Technology, USA), p-JUN (3270s, Cell Signaling Technology, USA), β-actin (GB11001, Servicebio, China). Secondary antibodies were used and visualized by enhanced chemiluminescence (Millipore, USA).
Cell Proliferation and Cell Cycle Analysis
Cell proliferation was accessed by Cell Counting Kit-8 (CCK8) assay (Dojindo, Japan) following the manufacturer's recommended procedure. In brief, 10 μl of CCK8 was added to treated cells in 96-wells and incubated at 37°C. The absorbance was detected at 450 nm using a Spectrophotometer (Molecular Devices, USA).
Cell cycle was measured by Cell Cycle and Apoptosis Kit (Wellbiology, China), and the treated cells were fixed overnight with 70% cold ethanol at 4°C, then stained with a mixture of propidium iodide and RNase A at 37°C for 30 min. The distribution of the cell cycle phase was measured by FlowJo software version 7.6.1 (Tree Star, USA).
Statistical Analysis
Statistical analysis was performed in R version. 4.1.0 (The R Foundation) and Graphed Prism 6 (GraphPad Software Inc., USA). Benjamini–Hochberg multiple testing correction was used to calculate the adjusted p-value or q-value. The data from in vitro and ex vivo experiments analyzed using Student's t-test and were presented as the mean ± SD. For all statistical analyses, p-value < 0.05 was considered significant.
Results
Common Targets of TwHF Active Ingredients and IgAN
The flow chart of the study was shown in Figure 1. The active chemical components of TwHF were selected via the Traditional Chinese Medicine Systems Pharmacology Database (TCMSP, http://tcmspw.com/tcmsp.php). With oral bioavailability (OB) ≥ 30% and the drug-likeness (DL) ≥0.18, 51 candidate compounds (Supplementary Table 1) and 122 corresponding targets were collected for further analysis. To visualize the interaction between TwHF targets and IgAN, 1311 potential IgAN targets were obtained using the GeneCards (https://www.genecards.org) and Online Mendelian Inheritance in Man (OMIM, https://www.omim.org) databases. The common targets of TwHF ingredients and IgAN were screened (Figure 2A). By mapping 61 target genes to TwHF and IgAN, a network that comprises 91 nodes and 282 edges was established (Figure 2B).
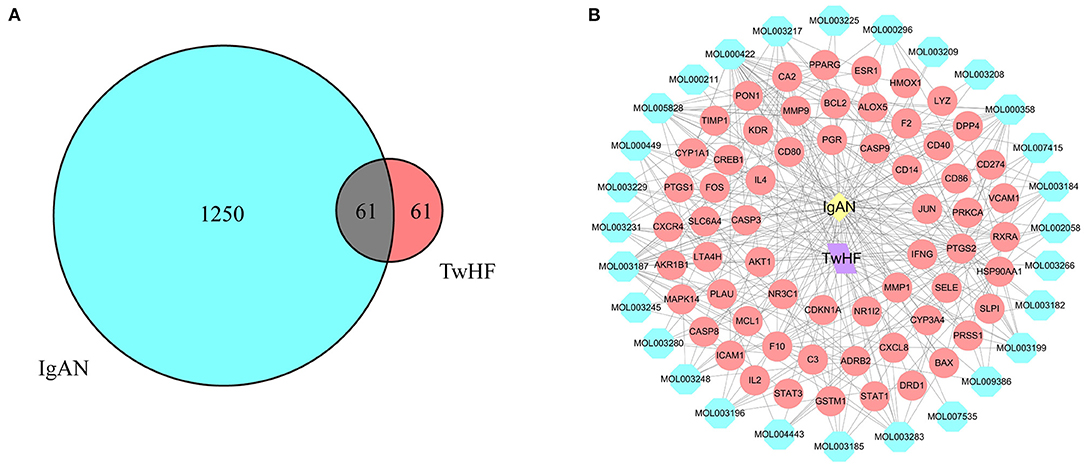
Figure 2. Network of potential targets between IgAN and TwHF ingredients. (A) Overlapping target genes between IgAN and TwHF. (B) Network of putative targets. The yellow and purple nodes stand for IgAN and TwHF, respectively. The blue nodes represent the active components of TwHF, and the red nodes represent the corresponding targets. The lines stand for interactions.
Functional Enrichment Analysis
To identify comprehensive information on biological processes, cellular components, and molecular functions gene function, GO and KEGG pathway analyses of common targets were performed via clusterProfiler package in R software. Values of p < 0.05 and q < 0.05 were set as the threshold. The top 20 GO terms with the highest degree of enrichment were shown in Figure 3A. GO terms mainly concentrated on DNA-binding transcription factor binding, RNA polymerase II-specific DNA-binding transcription factor binding, and endopeptidase activity. Figure 3B presented 20 enriched KEGG pathways of the common targets, lipid and atherosclerosis pathway, Kaposi sarcoma-associated herpesvirus infection, and hepatitis B were identified as the top three significantly relevant signal pathways.
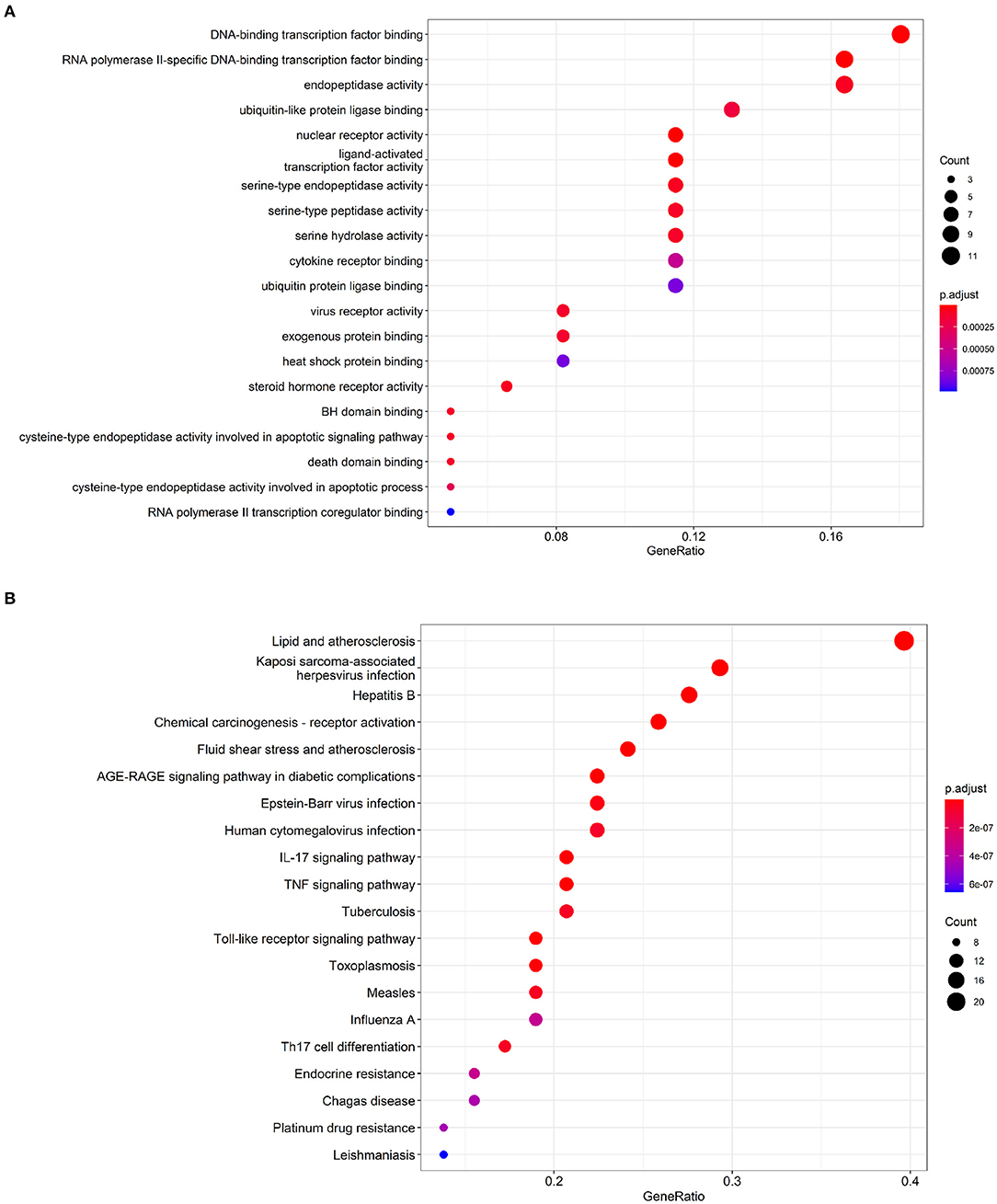
Figure 3. Functional enrichment analysis of the IgAN-TwHF ingredients common targets. (A) Top 20 GO terms and (B) Top 20 enriched KEGG pathways of targets. The color depth of the nodes refers to the adj p-value. The size of the nodes refers to the number of genes.
PPI Network Construction and key Genes Recognition
The STRING database (https://string-db.org) was used to explore potential interactions between targets, and visualization of protein-protein interaction (PPI) network was shown in Figure 4A. 592 interactions were calculated by R software, the top 30 candidate hub genes which may play a central role in this network were identified in Figure 4B. Targets with higher degrees including AKT1, CXCL8, MMP9, PTGS2, CASP3, and JUN were identified as key genes.
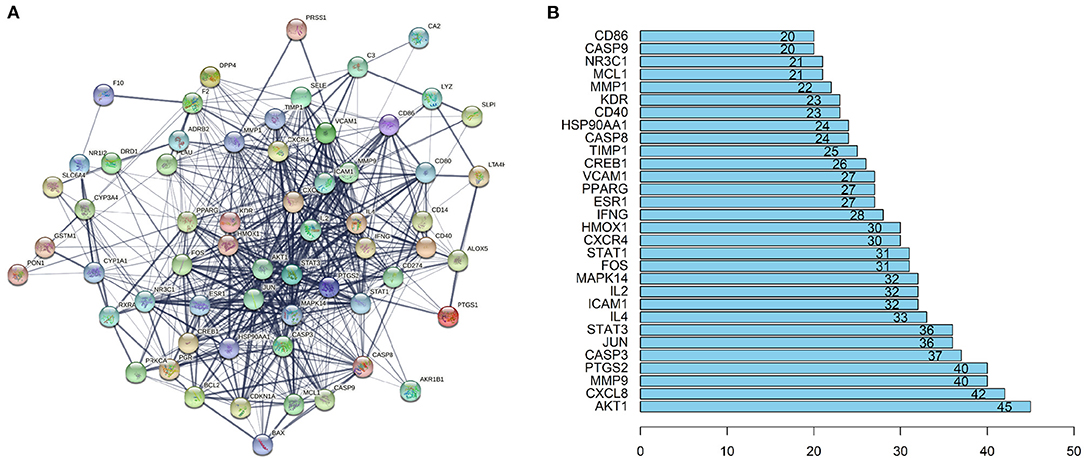
Figure 4. PPI network and the key genes of the IgAN-TwHF ingredients common targets. (A) The PPI network of targets was constructed using string. (B) The Top 30 key genes were obtained from the PPI network. The numbers on the bar represent the number of related nodes for each gene.
Targets Verification
Tripterygium wilfordii polyglycosides (TGT) is widely used in clinical practice on IgAN in China, with triptolide as the effective non-steroidal immunosuppressive ingredient (9). Considering we have previously found an important role of JUN signaling in IgAN mesangial cells, we determined to verify JUN (also known as c-JUN) as the target of TGT or triptolide treatment both in vivo and in vitro.
The immunohistochemical results of p-JUN were significantly increased in IgAN mice compared with controls, while treatment with TGT significantly decreased p-JUN expression (Figure 5A). Western blot analysis further confirmed that TGT suppressed phosphorylation of JUN in IgAN (Figure 5B).
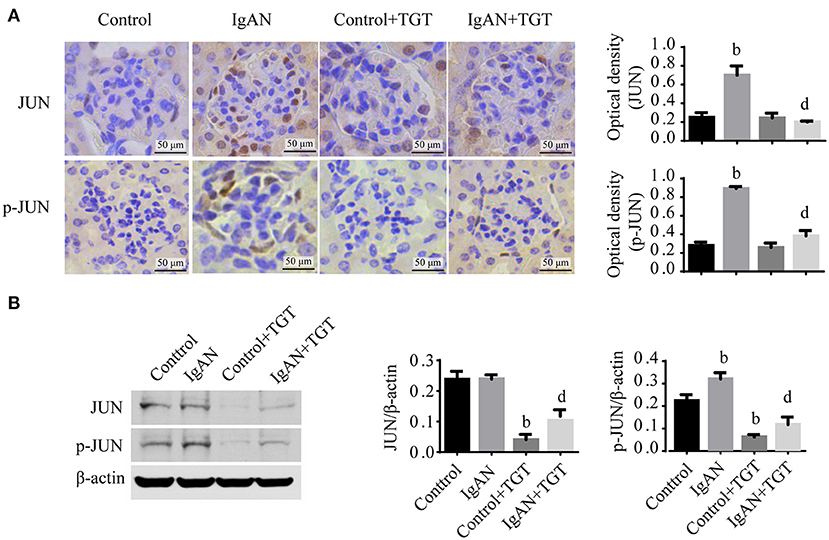
Figure 5. TGT downregulated JUN signaling in the kidney of IgAN mice. (A) Representative images and quantitative analysis of glomerular immunohistostaining with JUN and p-JUN in TGT treated mice or control mice. The average optical density was analyzed by ImageJ software. (B) Western blot analysis for JUN, p-JUN expressions in IgAN. Data are presented as mean ± SD of three independent experiments. bp < 0.01 vs. control group, dp < 0.01 vs. IgAN group.
Since mesangial cell proliferation is a prominent pathological feature of IgAN, in vitro, we investigated the effect of TwHF on HMCs. Moreover, 25 μg/ml aIgA1-stimulated HMCs were used to mimic the IgAN HMCs model, as previously described (21). The CCK8 assay showed that triptolide diminished cell proliferation in a concentration-dependent manner within 25–75 nM after 24 h treatment (Figure 6A), and there was no significant cell morphology alteration. In addition, flow cytometry was performed to detect cell cycle. Results showed that aIgA1 increased the proportion of cells in S and G2/M phase after 24 h incubation, with the application of 50 nM triptolide, more cells were arrested in G0/G1 phase (Figure 6B).
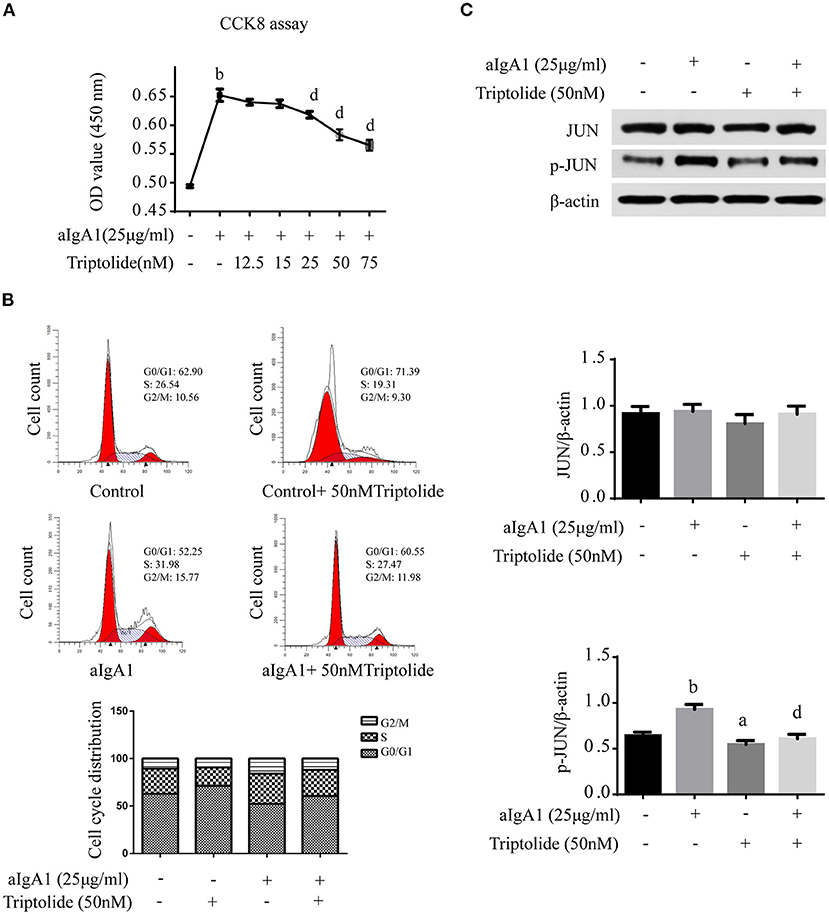
Figure 6. Triptolide inhibited HMCs proliferation and reduced phosphorylation of JUN signaling. (A) The proliferation of HMCs with different concentrations of triptolide intervention was assessed by CCK8 assay. (B) The cell cycle of HMCs treated with 25 μg/ml aIgA1 and 50 nM triptolide was detected by flow cytometry. (C) Immunoblot analyses and quantitative determination of the protein levels of JUN, p-JUN in HMCs. Data are the mean ± SD of three independent experiments. ap <0.05, bp < 0.01 vs. no treatment group, dp < 0.01 vs. only aIgA1-treated group.
Finally, we examined the effect of triptolide on the JUN signaling in IgAN. Western blot analysis showed that 50 nM triptolide efficiently suppressed the phosphorylation of JUN (Figure 6C).
Discussion
The first description of IgA nephropathy was proposed by Jean Berger in 1968, thus it is also named Berger's s disease (22). At present, there is still no specific treatment for IgAN. Supportive care is the basis including angiotensin-converting enzyme inhibitors (ACEI) or angiotensin receptor blockers (ARB), adequate blood pressure control, low-salt diet, and aerobic exercise. TwHF contains several active ingredients which affect multiple targets and pathways in the progression of IgAN and has been used as an adjuvant on IgAN treatment (11). A wide spectrum of biological activities about TwHF including anti-inflammatory, immunosuppressive, antioxidation, and antifibrosis has been demonstrated (9, 23–26). Triptolide, a diterpenoid trioxide, is the most abundant and pharmacologically active of the metabolites found in TwHF extracts and is primarily responsible for the anti-inflammatory and immunosuppressive effects of TwHF preparation (27, 28). Evidence has emerged to elucidate the biological effects of triptolide or its pharmaceutical preparation TGT in IgAN. TGT was reported to inhibit the secretion of serum inflammatory factor TNF-α, IL-6, and TGF-β1 in IgAN (29). Our team previously showed that triptolide decrease pro-inflammatory cytokines IL-1β and IL-18 in serum via the NLRP3/TLR4 pathway, and reduced the deposition of immune complexes-induced mesangial cell proliferation in IgAN rats (30). The early study was also observed that triptolide could effectively up-regulate the expression of nephrin protein, protect podocytes and reduce urinary protein in the rat model of IgAN (31). All the above evidence proves that TwHF has great benefits on IgAN, however, the pharmacological mechanisms of TwHF associated with IgAN only focus on a single chemical molecule. Here, we performed a comprehensive and systematic evaluation of the molecular mechanisms of TwHF on IgAN and highlighted new perspectives of TwHF for delaying IgAN progression and strengthening the therapeutic theory.
In this study, 51 active ingredients were selected from TwHF and 61 common targets were matched between the components of TwHF and IgAN based on network pharmacology. Functional enrichment analysis suggested that TwHF mainly interfere with the binding of transcription factors to regulatory regions of genes and induce or inhibit its gene expression. According to the PPI network, candidate key genes of TwHF against IgAN were identified as AKT1, CXCL8, MMP9, PTGS2, CASP3, and JUN. AKT and CXCL8 were reported to be involved in the inflammatory response and were activated during renal injury (32–35), polymorphism of CXCL8 was associated with increased susceptibility to IgAN (36). Studies also suggested that triptolide could attenuate renal tubular epithelial-mesenchymal transition via AKT signaling in diabetic kidney disease (37). In addition, TwHF extracts contained novel inhibitors of MMP including MMP9 thus might have therapeutic potential in arthritis and other conditions associated with increased MMPs like IgAN (38, 39). CASP3 is known as a representative effector of apoptosis, cleaved caspase-3 active fragment could mediate apoptosis in endothelial cells (40). PTGS2 also known as cyclooxygenase 2 (COX2), is the key enzyme in prostaglandin biosynthesis, and is responsible for the prostanoid biosynthesis involved in inflammation and mitogenesis. COX-derived prostanoids appear to be involved in the pathogenesis of diabetic nephropathy (41), and a recent study regarded prostaglandins as potential targets for the treatment of polycystic kidney disease (42). JUN is a positive regulator of cell proliferation which promotes cell proliferation by accelerating G1-S cell cycle progression (43–45). It could bind with FOS to form AP-1 (Activator protein-1) early transcription response factor and mediate many biological functions (46, 47). Our previous study confirmed the pivotal role of c-Jun N-terminal protein kinase (JNK)/JUN in IgAN mesangial cell proliferation and renal lesions, activation of c-Jun promoted cyclinD1 and proliferating cell nuclear antigen (PCNA) expression, accelerating the proliferation of IgAN mesangial cells (19). Considering that JUN ranked among the best in the results of functional enrichment analysis and PPI network, we thus verified whether TwHF could attenuate IgAN renal injury by regulating JUN. As shown in our study, TGT treatment could reduce the proliferation of mesangial cells and inhibit p-JUN signaling. In vitro, JUN phosphorylation was enhanced after aIgA1 treatment, while was abolished when triptolide was added. Cell cycle detection showed that the HMCs were arrested in the G1 phase after triptolide intervention.
JUN is generally considered to be essential for many biological functions especially in the progression of the G1 phase of the cell cycle (48, 49). Cells lacking JUN increased p53 (cell cycle arrest inducer) and p21 (cell cycle protease inhibitor 1, p53 target gene) expressions while overexpressing JUN reversed the levels of p53 and p21 and showed accelerated cell cycle transition as well as cell proliferation (50, 51). In addition, JUN could regulate the transcription of Rb kinase cyclinD1 to promote cell cycle transition (52). JUN signaling was also reported to be associated with renal interstitial fibrosis (53, 54). Here, we verified JUN as potential therapeutic targets for TwHF treatment based on network pharmacology tools, further research is required to detect the variety of changes occurring to cellular processes and downstream of JUN signaling with/without administration of TwHF following knockdown of JUN expression.
Data Availability Statement
The original contributions presented in the study are included in the article/Supplementary Material, further inquiries can be directed to the corresponding author/s.
Ethics Statement
The studies involving human participants were reviewed and approved by Ethics Committee of the Second Xiangya Hospital of Central South University. The patients/participants provided their written informed consent to participate in this study. The animal study was reviewed and approved by Animal Care Ethics Committee of Xiangya Medical School, Central South University.
Author Contributions
MX contributed to the study design, experiments, and draft of the manuscript. DL, HL, and JZ contributed to data collection and statistical analysis/interpretation. CT, GC, and YL contributed to review and editing. HL gave the final approval for the article to be published. All authors have read and approved the final manuscript.
Funding
This work was supported by the National Natural Science Foundation of China (82070737 and 81770714), Scientific Research Key Project of Hunan Traditional Chinese Medicine Administration (2021040), and PRO∙Run Fund of the Nephrology Group of CEBM (KYS2021-03-02-7).
Conflict of Interest
The authors declare that the research was conducted in the absence of any commercial or financial relationships that could be construed as a potential conflict of interest.
Publisher's Note
All claims expressed in this article are solely those of the authors and do not necessarily represent those of their affiliated organizations, or those of the publisher, the editors and the reviewers. Any product that may be evaluated in this article, or claim that may be made by its manufacturer, is not guaranteed or endorsed by the publisher.
Acknowledgments
We sincerely appreciate the time and effort of all who contributed to this study.
Supplementary Material
The Supplementary Material for this article can be found online at: https://www.frontiersin.org/articles/10.3389/fmed.2021.794962/full#supplementary-material
References
1. O'Shaughnessy MM, Hogan SL, Thompson BD, Coppo R, Fogo AB, Jennette JC. Glomerular disease frequencies by race, sex and region: results from the international kidney biopsy survey. Nephrol Dial Transplant. (2018) 33:661–9. doi: 10.1093/ndt/gfx189
2. Lai KN, Tang SC, Schena FP, Novak J, Tomino Y, Fogo AB, et al. IgA nephropathy. Nat Rev Dis Primers. (2016) 2:16001. doi: 10.1038/nrdp.2016.1
3. Chen T, Li X, Li Y, Xia E, Qin Y, Liang S, et al. Prediction and risk stratification of kidney outcomes in iga nephropathy. Am J Kidney Dis. (2019) 74:300–309. doi: 10.1053/j.ajkd.2019.02.016
4. Barbour S, Coppo R, Zhang H, Liu Z, Suzuki Y, Matsuzaki K, et al. evaluating a new international risk-prediction tool in IgA nephropathy. JAMA Intern Med. (2019) 179:942–52. doi: 10.1001/jamainternmed.2019.0600
5. Rodrigues JC, Haas M, Reich HN. IgA Nephropathy. Clin J Am Soc Nephrol. (2017) 12:677–86. doi: 10.2215/CJN.07420716
6. Tong L, Zhao Q, Datan E, Lin G, Minn I, Pomper M, et al. Triptolide: reflections on two decades of research and prospects for the future. Nat Prod Rep. (2021) 38:843–60. doi: 10.1039/D0NP00054J
7. Leishi L, Xun Z, Guangyong C, Daxi J, Zhida B. Clinical study of Tripterygium wilfordii in the treatment of glomerulonephritis. Chin J Intern Med. (1981) 20:216–20.
8. Xu XL, Yang LJ, Jiang JG. Renal toxic ingredients and their toxicology from traditional Chinese medicine. Expert Opin Drug Metab Toxicol. (2016) 12:149–59. doi: 10.1517/17425255.2016.1132306
9. Zhu B, Wang Y, Jardine M, Jun M, Lv J, Cass A, et al. Tripterygium preparations for the treatment of CKD: a systematic review and meta-analysis. Am J Kidney Dis. (2013) 62:515–30. doi: 10.1053/j.ajkd.2013.02.374
10. Wang Z, Yu C, Zhou LN, Chen X. Effects of tripterygium wilfordii induction therapy to iga nephropathy patients with heavy proteinuria. Biol Pharm Bull. (2017) 40:1833–38. doi: 10.1248/bpb.b17-00134
11. Chen Y, Gao Q, Zhao X, Chen X, Zhang F, Chen J, et al. Meta-analysis of tripterygium wilfordii hook f in the immunosuppressive treatment of IgA nephropathy. Intern Med. (2010) 49:2049–55. doi: 10.2169/internalmedicine.49.3704
12. Li Y, Xiao X, Wang H, Zhou Q, Jin Z, Zhang Y, et al. Integrating network pharmacology and experimental models to investigate the mechanisms of dihydroartemisinin in preventing NSCLC progression via mTOR/HIF-1α signaling. Eur J Pharmacol. (2021) 21:174411. doi: 10.1016/j.ejphar.2021.174411
14. Ru J, Li P, Wang J, Zhou W, Li B, Huang C, et al. TCMSP: a database of systems pharmacology for drug discovery from herbal medicines. J Cheminform. (2014) 6:13. doi: 10.1186/1758-2946-6-13
15. UniProt Consortium T. UniProt: the universal protein knowledgebase. Nucleic Acids Res. (2018) 46:2699. doi: 10.1093/nar/gky092
16. Fishilevich S, Zimmerman S, Kohn A, Iny Stein T, Olender T, Kolker E, et al. Genic insights from integrated human proteomics in GeneCards. Database (Oxford). (2016) 2016. doi: 10.1093/database/baw030
17. Amberger J, Hamosh A. Searching online mendelian inheritance in man (omim): a knowledgebase of human genes and genetic phenotypes. Curr Protoc Bioinformatics. (2017) 58:12. doi: 10.1002/cpbi.27
18. Szklarczyk D, Gable A, Lyon D, Junge A, Wyder S, Huerta-Cepas J, et al. STRING v11: protein-protein association networks with increased coverage, supporting functional discovery in genome-wide experimental datasets. Nucleic Acids Res. (2019) 47:D607–13. doi: 10.1093/nar/gky1131
19. Li Y, Xia M, Peng L, Liu H, Chen G, Wang C, et al. Downregulation of miR-214-3p attenuates mesangial hypercellularity by targeting PTEN-mediated JNK/c-Jun signaling in IgA nephropathy. Int J Biol Sci. (2021) 17:3343–55. doi: 10.7150/ijbs.61274
20. Hyun YY, Kim IO, Kim MH, Nam DH, Lee MH, Kim JE, et al. Adipose-derived stem cells improve renal function in a mouse model of IgA nephropathy. Cell Transplant. (2012) 21:2425–39. doi: 10.3727/096368912X639008
21. Xia M, Liu D, Tang X, Liu Y, Liu H, Liu Y, et al. Dihydroartemisinin inhibits the proliferation of IgAN mesangial cells through the mTOR signaling pathway. Int Immunopharmacol. (2020) 80:106125. doi: 10.1016/j.intimp.2019.106125
23. Zhao X, Tang X, Yan Q, Song H, Li Z, Wang D, et al. Triptolide ameliorates lupus via the induction of miR-125a-5p mediating treg upregulation. Int Immunopharmacol. (2019) 71:14–21. doi: 10.1016/j.intimp.2019.02.047
24. Li H, Guo F, Luo Y, Zhu J, Wang J. Efficacy of tripterygium glycosides tablet in treating ankylosing spondylitis: a systematic review and meta-analysis of randomized controlled trials. Clin Rheumatol. (2015) 34:1831–8. doi: 10.1007/s10067-015-3043-6
25. Zhang Y, Mao X, Li W, Chen W, Wang X, Ma Z, et al. Tripterygium wilfordii: An inspiring resource for rheumatoid arthritis treatment. Med Res Rev. (2021) 41:1337–1374. doi: 10.1002/med.21762
26. Zhu W, Li Y, Gong J, Zuo L, Zhang W, Cao L, et al. Tripterygium wilfordii Hook. f. versus azathioprine for prevention of postoperative recurrence in patients with Crohn's disease: a randomized clinical trial. Dig Liver Dis. (2015) 47:14–9. doi: 10.1016/j.dld.2014.09.008
27. Lin N, Sato T, Ito A. Triptolide, a novel diterpenoid triepoxide from Tripterygium wilfordii Hook. f., suppresses the production and gene expression of pro-matrix metalloproteinases 1 and 3 and augments those of tissue inhibitors of metalloproteinases 1 and 2 in human synovial fibroblasts. Arthritis Rheum. (2001) 44:2193–200. doi: 10.1002/1529-0131(200109)44:9<2193::AID-ART373>3.0.CO;2-5
28. Song X, Zhang Y, Dai E. Therapeutic targets of thunder god vine (Tripterygium wilfordii hook) in rheumatoid arthritis (Review). Mol Med Rep. (2020) 21:2303–10. doi: 10.3892/mmr.2020.11052
29. Pengxiao S, Genshang Z, Shuang W, Jiansheng W, Yingying Z, Jingqian M, et al. The impact of tripterygium wilfordii polyglycosidium on inflammatory factor and renal function of rats with IgA nephropathy. Chin J Exp Surg. (2017) 34:631–4. doi: 10.3760/cma.j.issn.1001-9030.2017.04.030
30. He L, Peng X, Liu G, Tang C, Liu H, Liu F, et al. Anti-inflammatory effects of triptolide on IgA nephropathy in rats. Immunopharmacol Immunotoxicol. (2015) 37:421–7. doi: 10.3109/08923973.2015.1080265
31. Hong Y, Zhou W, Li K, Sacks S. Triptolide is a potent suppressant of C3, CD40 and B7h expression in activated human proximal tubular epithelial cells. Kidney Int. (2002) 62:1291–300. doi: 10.1111/j.1523-1755.2002.kid586.x
32. Kim I, Park Y, Song S, Seong E, Lee D, Bae S, et al. Akt1 is involved in tubular apoptosis and inflammatory response during renal ischemia-reperfusion injury. Mol Biol Rep. (2020) 47:9511–20. doi: 10.1007/s11033-020-06021-1
33. Tian J, Wang Y, Guo H, Li R. The Akt/mTOR/p70S6K pathway is activated in IgA nephropathy and rapamycin may represent a viable treatment option. Exp Mol Pathol. (2015) 99:435–40. doi: 10.1016/j.yexmp.2015.08.004
34. Bai Y, Li H, Dong J. Up-regulation of miR-20a weakens inflammation and apoptosis in high-glucose-induced renal tubular cell mediating diabetic kidney disease by repressing CXCL8 expression. Arch Physiol Biochem. (2020) 20:1–8. doi: 10.1080/13813455.2020.1785506
35. Cui S, Zhu Y, Du J, Khan M, Wang B, Wei J, et al. CXCL8 antagonist improves diabetic nephropathy in male mice with diabetes and attenuates high glucose-induced mesangial injury. Endocrinology. (2017) 158:1671–84. doi: 10.1210/en.2016-1781
36. Suh J, Hahn W, Cho B. Polymorphisms of CXCL8 and its receptor CXCR2 contribute to the development and progression of childhood IgA nephropathy. J Interferon Cytokine Res. (2011) 31:309–15. doi: 10.1089/jir.2010.0031
37. Xue M, Cheng Y, Han F, Chang Y, Yang Y, Li X, et al. triptolide attenuates renal tubular epithelial-mesenchymal transition via the MiR-188-5p-mediated PI3K/AKT pathway in diabetic kidney disease. Int J Biol Sci. (2018) 14:1545–57. doi: 10.7150/ijbs.24032
38. Sylvester J, Liacini A, Li W, Dehnade F, Zafarullah M. Tripterygium wilfordii Hook F extract suppresses proinflammatory cytokine-induced expression of matrix metalloproteinase genes in articular chondrocytes by inhibiting activating protein-1 and nuclear factor-kappaB activities. Mol Pharmacol. (2001) 59:1196–205. doi: 10.1124/mol.59.5.1196
39. Mi C, Shi H, Ma J, Han L, Lee J, Jin X. Celastrol induces the apoptosis of breast cancer cells and inhibits their invasion via downregulation of MMP-9. Oncol Rep. (2014) 32:2527–32. doi: 10.3892/or.2014.3535
40. Zhai Y, Liu Y, Qi Y, Long X, Gao J, Yao X, et al. The soluble VEGF receptor sFlt-1 contributes to endothelial dysfunction in IgA nephropathy. PloS ONE. (2020) 15:e0234492. doi: 10.1371/journal.pone.0234492
41. Hao C, Breyer M. Physiological regulation of prostaglandins in the kidney. Annu Rev Physiol. (2008) 70:357–77. doi: 10.1146/annurev.physiol.70.113006.100614
42. Aukema H. Prostaglandins as potential targets for the treatment of polycystic kidney disease. Prostaglandins Leukot Essent Fatty Acids. (2021) 164:102220. doi: 10.1016/j.plefa.2020.102220
43. Kullmann M, Pegka F, Ploner C, Hengst L. Stimulation of c-Jun/AP-1-Activity by the Cell Cycle Inhibitor p57. Front Cell Dev Biol. (2021) 9:664609. doi: 10.3389/fcell.2021.664609
44. Kwak A, Lee M, Lee M, Yoon G, Cho S, Chae J, et al. The 3-deoxysappanchalcone induces ROS-mediated apoptosis and cell cycle arrest via JNK/p38 MAPKs signaling pathway in human esophageal cancer cells. Phytomedicine. (2021) 86:153564. doi: 10.1016/j.phymed.2021.153564
45. Babu R, Naveen Kumar M, Patil R, Devaraju K, Ramesh G, Sharma S. Effect of estrogen and tamoxifen on the expression pattern of AP-1 factors in MCF-7 cells: role of c-Jun, c-Fos, and Fra-1 in cell cycle regulation. Mol Cell Biochem. (2013) 380:143–51. doi: 10.1007/s11010-013-1667-x
46. Wu Q, Wu W, Fu B, Shi L, Wang X, Kuca K. JNK signaling in cancer cell survival. Med Res Rev. (2019) 39:2082–104. doi: 10.1002/med.21574
47. Brennan A, Leech J, Kad N, Mason J. Selective antagonism of cJun for cancer therapy. J Exp Clin Cancer Res. (2020) 39:184. doi: 10.1186/s13046-020-01686-9
48. Yamaguchi M. Role of regucalcin in cell nuclear regulation: involvement as a transcription factor. Cell Tissue Res. (2013) 354:331–41. doi: 10.1007/s00441-013-1665-z
49. Muthukrishnan S, Yang X, Friesel R, Oxburgh L. Concurrent BMP7 and FGF9 signalling governs AP-1 function to promote self-renewal of nephron progenitor cells. Nat Commun. (2015) 6:10027. doi: 10.1038/ncomms10027
50. Schreiber M, Kolbus A, Piu F, Szabowski A, Möhle-Steinlein U, Tian J, et al. Control of cell cycle progression by c-Jun is p53 dependent. Genes Dev. (1999) 13:607–19. doi: 10.1101/gad.13.5.607
51. Ogawa E, Okuyama R, Egawa T, Nagoshi H, Obinata M, Tagami H, et al. p63/p51-induced onset of keratinocyte differentiation via the c-Jun N-terminal kinase pathway is counteracted by keratinocyte growth factor. J Biol Chem. (2008) 283:34241–9. doi: 10.1074/jbc.M804101200
52. Wisdom R, Johnson R, Moore C. c-Jun regulates cell cycle progression and apoptosis by distinct mechanisms. EMBO J. (1999) 18:188–97. doi: 10.1093/emboj/18.1.188
53. Liu Y, Shen P, Zhou Y, Tang L, Chai H. c-Jun N-terminal kinase/transforming growth factor-β/Smad3 pathway: Is it associated with endoplasmic reticulum stress-mediated renal interstitial fibrosis? Mol Med Rep. (2019) 20:755–62. doi: 10.3892/mmr.2019.10276
Keywords: Tripterygium wilfordii Hook F, IgA nephropathy, network pharmacology, JUN, mesangial cell
Citation: Xia M, Liu D, Liu H, Zhao J, Tang C, Chen G, Liu Y and Liu H (2021) Based on Network Pharmacology Tools to Investigate the Mechanism of Tripterygium wilfordii Against IgA Nephropathy. Front. Med. 8:794962. doi: 10.3389/fmed.2021.794962
Received: 14 October 2021; Accepted: 16 November 2021;
Published: 15 December 2021.
Edited by:
Rujun Gong, University of Toledo Medical Center, United StatesReviewed by:
Guisen Li, Sichuan Academy of Medical Sciences and Sichuan Provincial People's Hospital, ChinaDongwei Liu, First Affiliated Hospital of Zhengzhou University, China
Copyright © 2021 Xia, Liu, Liu, Zhao, Tang, Chen, Liu and Liu. This is an open-access article distributed under the terms of the Creative Commons Attribution License (CC BY). The use, distribution or reproduction in other forums is permitted, provided the original author(s) and the copyright owner(s) are credited and that the original publication in this journal is cited, in accordance with accepted academic practice. No use, distribution or reproduction is permitted which does not comply with these terms.
*Correspondence: Hong Liu, bGl1aG9uZzYxOEBjc3UuZWR1LmNu