- 1Health Management Center, The First Affiliated Hospital of Zhengzhou University, Zhengzhou, China
- 2College of Public Health, Zhengzhou University, Zhengzhou, China
- 3Department of Nephropathy, The First Affiliated Hospital of Zhengzhou University, Zhengzhou, China
- 4Gene Hospital of Henan Province, The First Affiliated Hospital of Zhengzhou University, Zhengzhou, China
Objective: To investigate the correlation between the structure and function alterations of gut microbiota and biochemical indicators in males with hyperuricemia (HUA) and high levels of liver enzymes, in order to provide new evidences and therapeutic targets for the clinical diagnosis and treatment of HUA.
Methods: A total of 69 patients with HUA (HUA group) and 118 healthy controls were enrolled in this study. Their age, height, waist circumference, weight, and pressure were measured. The clinical parameters such as fasting plasma glucose (FBG), aspartate aminotransferase (AST), alanine aminotransferase (ALT), serum uric acid (SUA), serum creatinine (Scr), total cholesterol (TC), triglyceride (TG), low-density lipoprotein (LDL), high-density lipoprotein (HDL), white blood cell (WBC), platelet (PLT), and absolute value of neutrophils (NEUT) were examined. We used whole-genome shotgun sequencing technology and HUMAnN2 MetaCyc pathway database to detect the composition and pathways of the gut microbiota. The main statistical methods were student's t test, chi-square tests, and Wilcoxon rank sum test. The correlations among bacterial diversity, microbial pathways, and biochemical indicators were evaluated by the R function “cor.test” with spearman method.
Results: The gut bacterial diversity in HUA group reduced significantly and the community of the microbiota was of significant difference between the two groups. The pathways that can produce 5-aminoimidazole ribonucleotide (PWY-6122, PWY-6277, and PWY-6121), aromatic amino acids, and chorismate (COMPLETE-ARO-PWY, ARO-PWY, and PWY-6163) were enriched in the HUA group; while the pathways that can produce short-chain fatty acids (SCFAs, such as CENTFERM-PWY and PWY-6590) and the gut microbiotas that can produce SCFAs (Roseburia hominis, Odoribacter splanchnicus, Ruminococcus callidus, Lachnospiraceae bacterium 3_1_46FAA, Bacteroides uniformis, Butyricimonas synergistica) and equol (Adlercreutzia equolifaciens) were enriched in healthy controls.
Conclusion: The structure and function of the gut microbiota in males with HUA and high levels of liver enzymes have altered apparently. In-depth study of related mechanisms may provide new ideas for the treatment of HUA.
Introduction
Hyperuricemia (HUA) is a group of heterogeneous chronic metabolic pathological conditions caused by purine metabolism disorder and/or uric acid excretion disorder. The excessive production and/or decreased excretion of uric acid level caused by any cause can lead to the occurrence of HUA. HUA is not only the direct cause of gout, but closely related to the onset and progression of many diseases such as diabetes, metabolic syndrome, chronic kidney disease, cerebrovascular-related diseases, and hyperlipidemia. Studies have confirmed that uric acid level is an independent risk factor leading to the progression of cardiovascular-related diseases such as hypertension and coronary heart disease, and it has an important impact on its prognosis (1). The latest research data show that the prevalence of HUA in the Chinese population has risen from less than 1.5% in the early 1980s to as high as 13% (2), and it is still rising and the incidence is showing a younger trend. Therefore, it is of great significance to study in depth the pathogenesis and further explore effective treatment measures of HUA.
Healthy people excrete uric acid in two ways mainly, of which 70% is excreted through the kidney, and the remaining 30% is excreted through the intestine (3). Intestinal microbes are a group of microorganisms designated to be planted in the intestinal tract, which have a symbiotic relationship with the human host and have a variety of important physiological functions such as participation in digestion, metabolism, promotion of nutrient absorption, synthesis of trace elements, and regulation of immune function. They are of great significance for the maintenance of our health (4). Researches in recent years have shown that changes in intestinal microbiota are not only related to diabetes, obesity, hypertension, and other diseases, but closely related to the occurrence of HUA. Studies have shown that changes in the gut microbiota and HUA promote each other and jointly aggravate the disease progression. For example, the intestinal microbiota can participate not only in the catabolism of purine and uric acid through the regulation of Escherichia coli and Lactobacillus (5–7), but also in the other two ways to affect the excretion of uric acid such as the production of short-chain fatty acids (SCFAs) (8–10) and changes in the number and distribution of uric acid transporters (11, 12) in the body. Besides, purine metabolism increases the production of uric acid, which can induce intestinal oxidative stress and then further promotes the infiltration of inflammatory cells and causes chronic inflammation in the intestinal tract. However, there are not many studies on the gut microbiota as an entry point to investigate HUA, and most of them are animal experiments. In view of the fact that most patients with HUA are asymptomatic and the intestinal microecology can change correspondingly in other disease states, it is more important and suitable to select the asymptomatic people who take physical examination as the research subjects to explore the correlation between HUA and gut microbiota. This study intends to explore the relationship among the serum uric acid (SUA) level, liver enzymes, and the detection of intestinal microbes in the physical examination population, and to provide more evidence support for finding the relationship among the serum uric acid, liver enzymes, and intestinal microecology.
Materials and Methods
Study Design
Totally, 89 persons and 118 participants were randomly enrolled in HUA group and healthy controls from the adult population who underwent the physical examination in the Department of health management center, the First Affiliated Hospital of Zhengzhou University, namely HUA group and healthy control group (healthy controls). According to the multidisciplinary expert consensus on the diagnosis and treatment of HUA-related diseases in China, the diagnostic criteria of HUA was defined as the fasting plasma uric acid value >420 μmol/L(7 mg/dL) on different days, excluding concomitant diabetes, tumors and hematological diseases. Exclusion criteria: persons with clear gastrointestinal diseases or severe diseases of cardiovascular, respiratory, kidney, and other systems; pregnant and lactating woman; minors under 18 years old; antibiotics and microbiota regulators, yogurt, gastrointestinal motility drugs, and other preparations that may affect the intestinal microbiota had been used in the past 2 months. According to the test, 89 people had HUA, including 2 females, 11 persons used antibiotics and yogurt preparations recently, and 7 people were of high blood sugar or used hypoglycemic drugs or antihypertensive drugs. After excluding the above population, 69 people were enrolled in HUA group (Figure 1A). The research protocol was approved by the Ethics Committee of the First Affiliated Hospital of Zhengzhou University (2018-KY-56 and 2018-KY-90), and all of the study subjects signed an informed consent form.
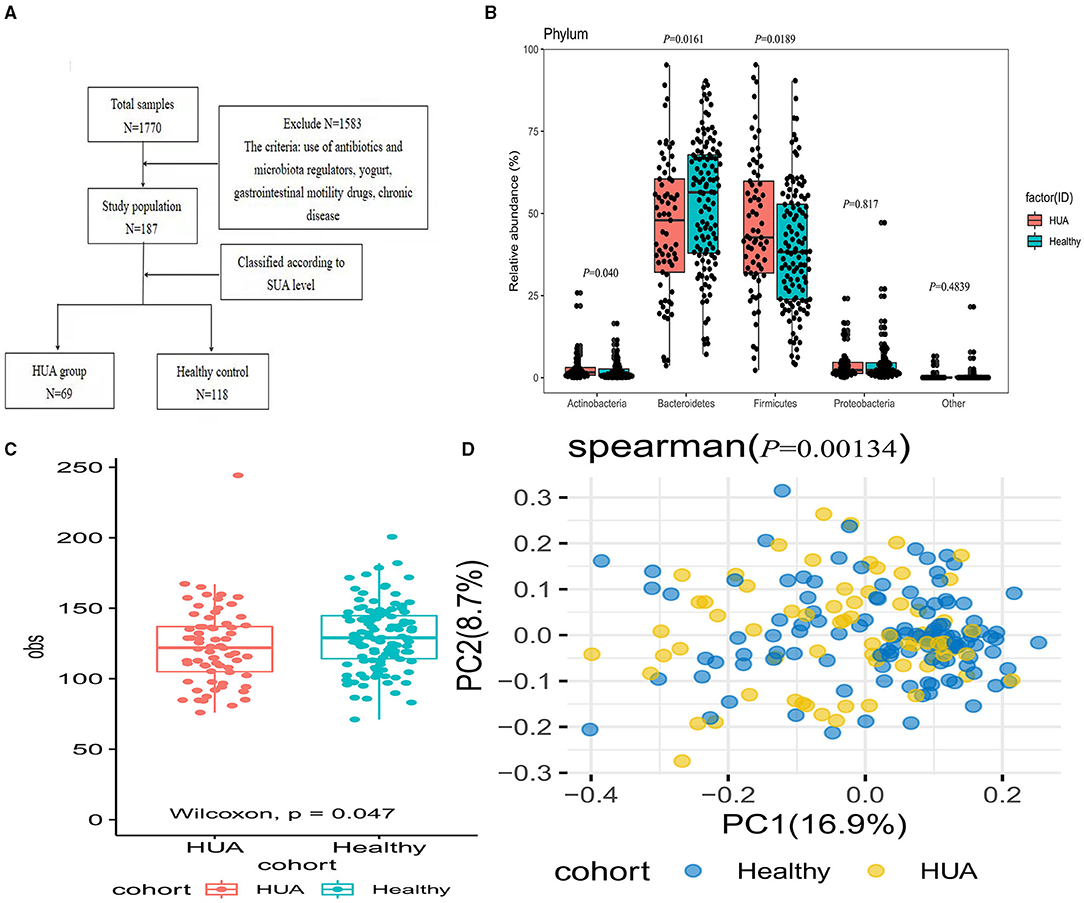
Figure 1. Comparison of the microbial community between HUA group and healthy controls. (A) Enrollment flow; (B) The abundance of gut microbiota between HUA and healthy controls at the phylum level; (C) Alpha diversity was analyzed at the species level by obs index between HUA group (N = 69) and healthy controls (N = 118); (D) Beta diversity by Pearson distance between the two groups.
General Information and Related Biochemical Indicators
The height, weight, waist circumference (Computerized body scale, SK-X80), and blood pressure (OMRON Medical automatic electronic blood pressure monitor, HBP-9021) were measured by designated staff after all subjects are enrolled in the group. Each indicator was measured for three times and the average value was taken, and then the body mass index (BMI) is calculated according to the following formula: BMI = weight Kg/ (height m)2. Every study subject was required to fast and no water for 8–12 h and got 5 mL of cubital venous blood on an empty stomach in the morning. All the test tubes were placed in the specimen box and sent to the clinical central laboratory within 20 min by the logistics trolley transported by the hospital's special specimens after the blood was drawn. UniCel DxI 800 Immunoassay System from Beckman Coulter was used to test the blood samples. The following laboratory tests were collected such as fasting plasma glucose (FPG), aspartate aminotransferase (AST), alanine aminotransferase (ALT), glutamyl transpeptidase (GGT), total bilirubin (TBIL), indirect bilirubin (IBIL), SUA, serum creatinine (Scr), total cholesterol (TC), triglyceride (TG), low-density lipoprotein (LDL), high-density lipoprotein (HDL), white blood cell (WBC), platelet (PLT), and absolute value of neutrophils (NEUT).
Collection of Stool Samples
Approximately 1 g of fresh stool specimen was collected from each study subject, placed the microbial sample preservation tube at −20°C, and transferred to −80°C for cryopreservation within 30 min. All blood and stool samples were collected before 10 o'clock on the day.
Statistical Analysis
R program (version 4.0.5) was used for the statistical analyses. Before analyzing the differences of the gut microbiota, we removed species with low expression levels and occurrence rates (positivity rates <10%). The laboratory test, demography, bacterial species, and pathways were analyzed by standardized statistical test methods. The main statistical methods were student's t test, chi-square tests, and Wilcoxon rank sum test. The continuous variables were expressed as mean ± SD ( s) and classified variables were represented by counts. Normal test and homogeneity test were applied to analyze the difference between groups, and P ≥ 0.05 was selected as the normal and homogeneity variances. Then, we used parametric test (t test) or non-parametric test (rank sum test) and P < 0.05 was regarded as statistically significant. The correlations among bacterial diversity, microbial pathways, and biochemical indicators were evaluated by the R function “cor.test” with Spearman method. The “ADE4” package in R program was applied to perform the principal coordinate analysis (PCoA) and the “vegan” package was used to calculate observed species number (obs) and Spearman index for each sample.
DNA Extraction, Shotgun Metagenomic Sequencing, and Reads Quantity Control
We extracted DNA from 1770 stool samples according to the operating instructions of the MagPure Stool DNA KF kit. All samples were tested using DNA nanospheres (DNB) based on DNA libraries construction and probe-anchored synthesis technology (cPAS) (MGI2000, MGI, Shenzhen, China) based on 100 bp paired-end reads of shotgun metagenomic sequencing. The overall accuracy (OA ≥ 0.8) control strategy were used to make sure the quality control (QC) of raw sequencing reads to filter out low-quality reads (13, 14). SOAPaligner/soap2 was applied to filter out human reads and hg19 was selected as the standard point of the high-quality reads (identity ≥ 0.9) (RRID:SCR_005503).
Microbiota Composition and Function Profiling
The taxonomic annotation and quantification on the base of MetaPhlAn2 with default settings were performed (15), and the generating gut microbial profiling of all levels such as bacteria, archaea, viruses, and eukaryotes were included. The HMP Unified Metabolic Analysis Network 2 (HUMAnN2) and National Center for Biotechnology Information (NCBI) (nlm.nih.gov) database (2014 Edition) were applied to annotate the nonredundant gene set and the functional genes into Kyoto Encyclopedia of Genes and Genomes (KEGG) metabolic pathway, and then the corresponding metabolic pathways were generated (13, 14).
Results
Clinical Characteristics of Subjects
A total of 69 patients with HUA (HUA group) and 118 healthy participants (healthy controls) were included in this cross-sectional cohort study. The level of SUA in the HUA group was significantly higher than that of the control group, accompanied by WC, BMI, AST, ALT, GGT, Scr, and TG were all higher than the control group, while HDL was lower than the control group. The differences were statistically significant (all P < 0.05) (Table 1).
Community Diversity in HUA Group and Healthy Controls
As shown in Figure 1B, four main abundant phylas dominated in the gut microbiota were discovered at the phylum level and there were significant differences in the phylum of Bacteroidetes, Firmicutes, and Actinobacteria between the two groups (Supplementary Table 1). Alpha and beta diversity was used for the evaluation of the community diversity at the species level. In Figure 1C, alpha diversity by obs was significantly different between HUA group and healthy controls (Wilcoxon rank sum test; P < 0.05). We then investigated the community variance among participants based on beta diversity by Pearson distance. Comparisons of the Pearson distance between the two groups showed significant differences in the first and second principal components (Wilcoxon rank sum test; P < 0.05) (Figure 1D).
Analysis of the Composition of Gut Microbiota Between HUA and Healthy Controls and the Correlation Among the Gut Microbiomes With a Panel of Clinical Characteristics
Analysis of the Composition of Gut Microbiota Between HUA and Healthy Controls
Totally 75 different species were identified between HUA group and healthy controls at the species level (Wilcoxon rank sum test; P < 0.05) (Supplementary Table 2), and 51 species were of significant difference after removing the low occurrence rate and low abundance species (P < 0.05; Figure 2A). In Figure 2A, we can see 37 species were enriched in the healthy controls and 14 species were enriched in the HUA group. From the view of species-level analysis, three high-abundance species (i.e., Ruminococcus gnavus and torques and Lachnospiraceae bacterium 1_4_56FAA) belonging to the Firmicutes were enriched in HUA group; and 12 species (i.e., Alistipes finegoldii, senegalensis and shahii; Bacteroides caccae, faecis, intestinalis, ovatus, plebeius, uniformis and xylanisolvens, Odoribacter splanchnicus and Parabacteroides distasonis) belonging to the Bacteroidetes; five species (i.e., Roseburia hominis, Ruminococcus callidus and lactaris, Lachnospiraceae bacterium 7_1_58FAA, and Coprococcus_sp_ART55_1) belonging to the Firmicutes; three species (i.e., Adlercreutzia equolifaciens; Butyricimonas synergistica, and Akkermansia muciniphila) belonging to other were enriched in healthy controls (Figure 2B).
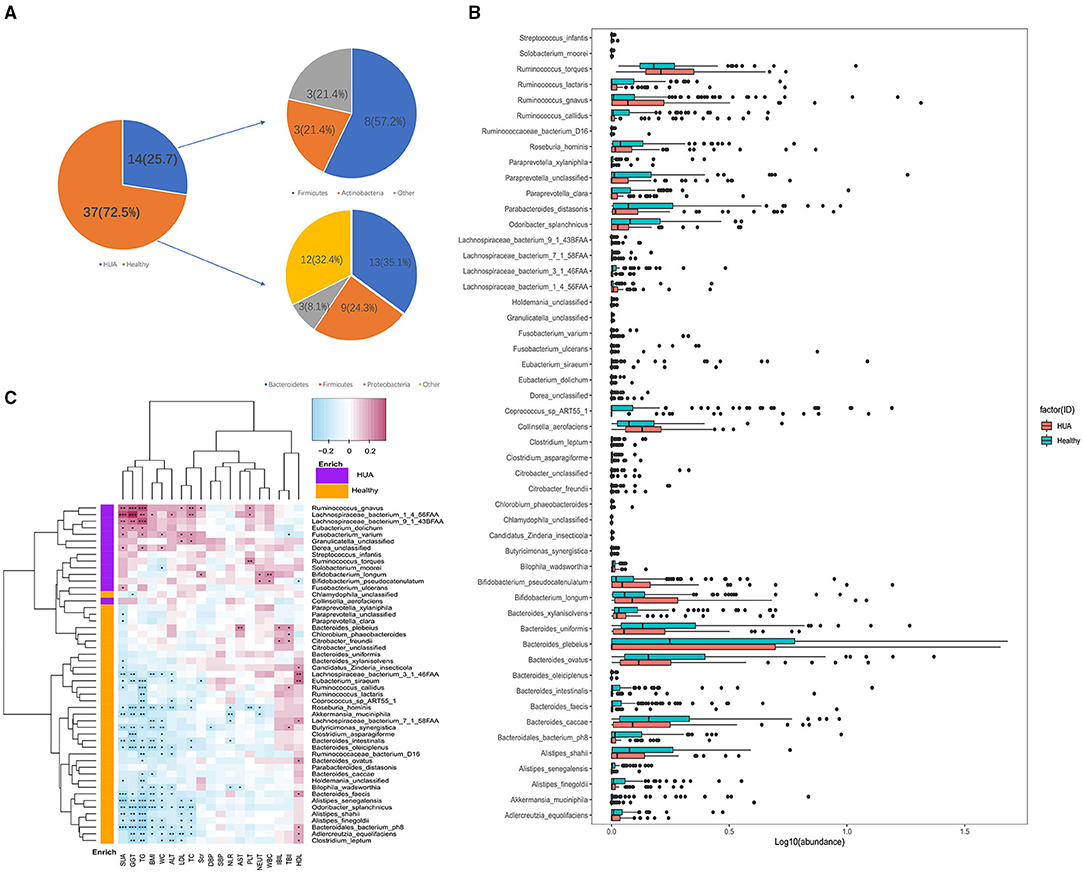
Figure 2. Microbiome differences between HUA group and healthy controls and the correlations with clinical characteristics. (A,B) Abundance of bacterial species that were of significant difference between HUA group and healthy controls. (C) Correlation matrix for bacterial species and clinical characteristics. Cells in red represented positive correlations while blue indicated negative. Cells with *, **, or *** asterisk represented P < 0.05, 0.01, or 0.001 respectively.
Correlation Among the Gut Microbiomes With a Panel of Clinical Characteristics
Spearman's correlation analysis was applied to explore the association between the species abundances and clinical characteristics in this article. Totally 187 samples from HUA subjects and healthy controls were analyzed and 187 associations with original P values <0.05 were found out. In Figure 2C, we could see that except HDL, TBIL, and IBIL, most of the gut microbiomes enriched in HUA group had significant positive correlations with the clinical characteristics, while those microbiomes enriched in the healthy controls were highly negatively correlated with these clinical indexes (Supplementary Table 3). Among the clinical parameters, SUA and TG had the largest number of correlations with bacterial species (n = 26 and n = 28 respectively, P < 0.05), followed by GGT (n = 21, P < 0.05), WC (n = 19, P < 0.05), BMI (n = 15, P < 0.05), TC (n = 14, P < 0.05), ALT (n = 13, P < 0.05), LDL, and HDL (both n = 10, P < 0.05). Besides, Roseburia hominis had the most frequent correlations with clinical parameters among the 51 bacterial species (n = 10, P < 0.05), followed by Odoribacter splanchnicus and Adlerreutzia equolifaciens (both n = 8, P < 0.05), Butyricimonas synergistica, Bacteroides intestinalis, Bacteroides faecis, Alistipes senegalensis, Alistipes fonegoldii, Lachnospiraceae bacterium 1_4_56FAA, and Ruminococcus gnavus (all n = 7, P < 0.05), Lachnospiraceae bacterium 3_1_46FAA (n = 6, P < 0.05), among the above bacterial species, two were enriched in HUA group (Ruminococcus gnavus and Lachnospiraceae bacterium 1_4_56FAA).
Functional Changes Brought About by the Microbiome in HUA Group and Healthy Controls
The Functional Differences From the Comparison of Different Participants
For each sample, MetaCyc pathway database by HUMAnN2 was further used to build the functional profiles with 494 pathways. Totally 41 pathways were identified in the comparison of MetaCyc pathway abundance between HUA group and healthy controls (Wilcoxon rank sum test; P < 0.05), and 28 pathways were of significant difference after removing the ones that were in low abundance and occurrence (Supplementary Table 4, Figure 3). Among the 28 pathways, 12 were enriched in healthy controls and 16 were enriched in HUA group. Within the 12 pathways in healthy controls, two were involved in the way of fermentation to SCFAs (CENTFERM-PWY and PWY-6590);one was related to the biosynthesis of GDP mannose (PWY-7323); five were related to the biosynthesis of gluconeogenesis (GLUCONEO-PWY and PWY66-399), pyrimidine deoxyribonucleotides (pwy-7198 and pwy-7210), and carbohydrate such as colanic acid (COLANSYN-PWY); two were responsible for energy generation (pwy66-398 tricarboxylic acid cycle, TCA) and energy metabolism (PWY-7383); one was related to superpathway of guanosine nucleotides degradation (PWY-6595); one was for the interconversion of arginine, ornithine, and proline (ARGORNPROST-PWY). Within the 16 HUA-enriched pathways, nine were related to the biosynthesis of 5-aminoimidazole ribonucleotide (PWY-6122, PWY-6277, and PWY-6121); pyrimidine deoxyribonucleotides (PWY-6545), aromatic amino acids, and chorismate (COMPLETE-ARO-PWY, ARO-PWY and PWY-6163) and tetrapyrrole (PWY-5188); one was responsible for L-glutamine biosynthesisIII, which is the way of nitrogen remobilization (PWY-6549); one was for purine ribonucleosides degradation (PWY0-1296); one was related to carbohydrate degradation, utilization, and assimilation (PWY-6737); one was involved in L-glutamate degradation (P162-PWY); one was for energy generation (PWY-5913); one was for the biosynthesis superpathway of L-lysine, L-threonine, and L-methionine II (PWY-724); and one was related to C4 photosynthetic carbon assimilation cycle (PWY-7117).
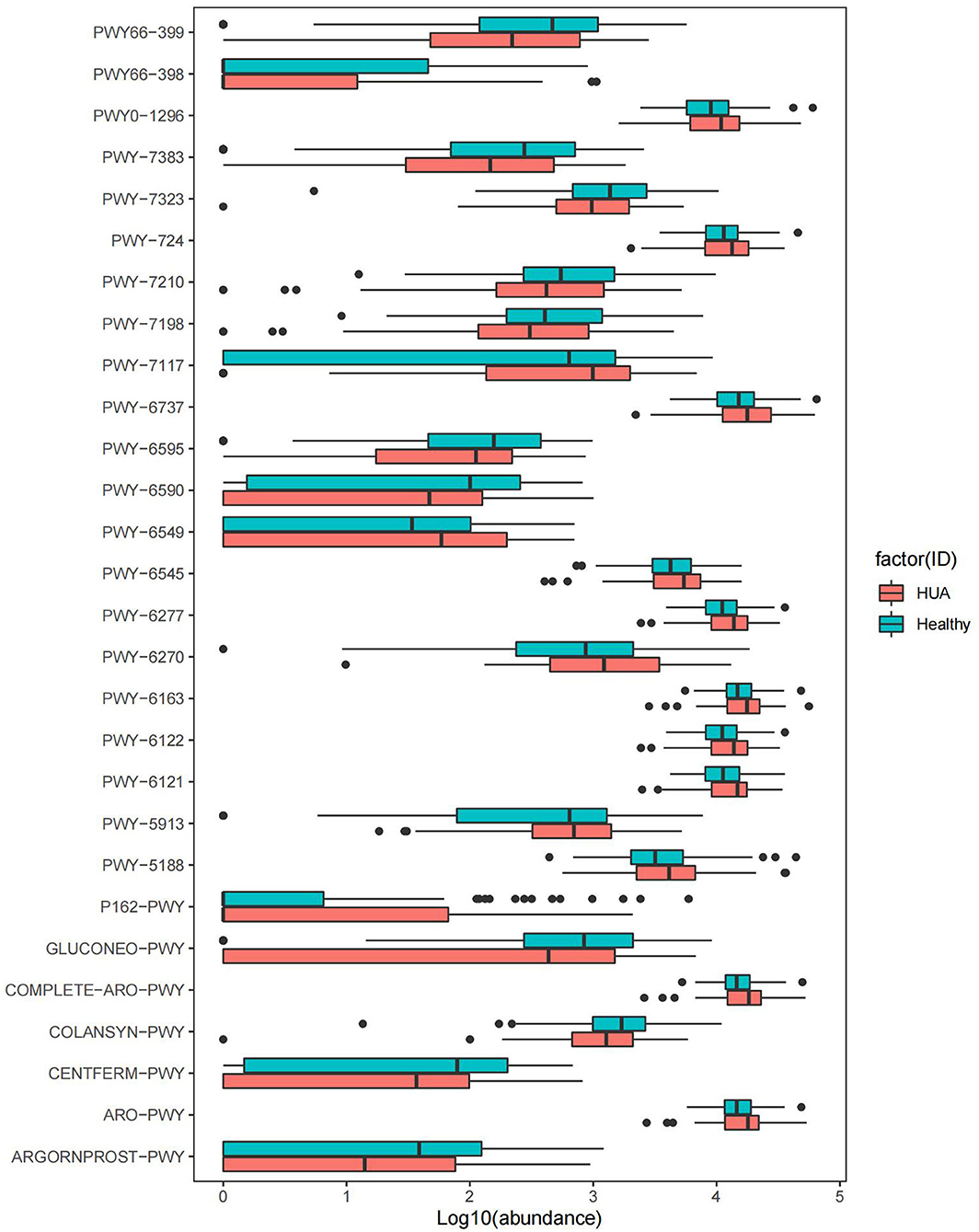
Figure 3. MetaCyc pathways with significant difference abundance between HUA group and healthy controls (Wilcoxon rank sum test, P < 0.05).
Correlation Between Microbial Pathways and a Panel of Clinical Characteristics
Spearman's correlation analyses were applied for the analysis of MetaCyc pathways with significant difference and a panel of clinical characteristics, and then heatmaps were constructed (P < 0.05) (Figure 4, Supplementary Table 5). In Figure 4, we could see that except HDL, most of the pathways enriched in HUA group had highly positive correlations with the clinical characteristics, while those pathways enriched in the healthy controls mostly had significant negative correlations with these clinical indexes. Especially, SUA, TC, TG, LDL, BMI, and GGT had highly negative correlations with the pathway of producing SCFAs, such as PWY-6590 and CENTFERM-PWY (P < 0.05). Most of the above clinical index also had significantly negative correlation with PWY-6595, which is responsible for the degradation from purine to allantoin (P < 0.05). Besides, TG, ALT, and WC had highly negative correlations with the pathway of PWY66-399 and PWY-7383, while HDL is just the opposite (P < 0.05).
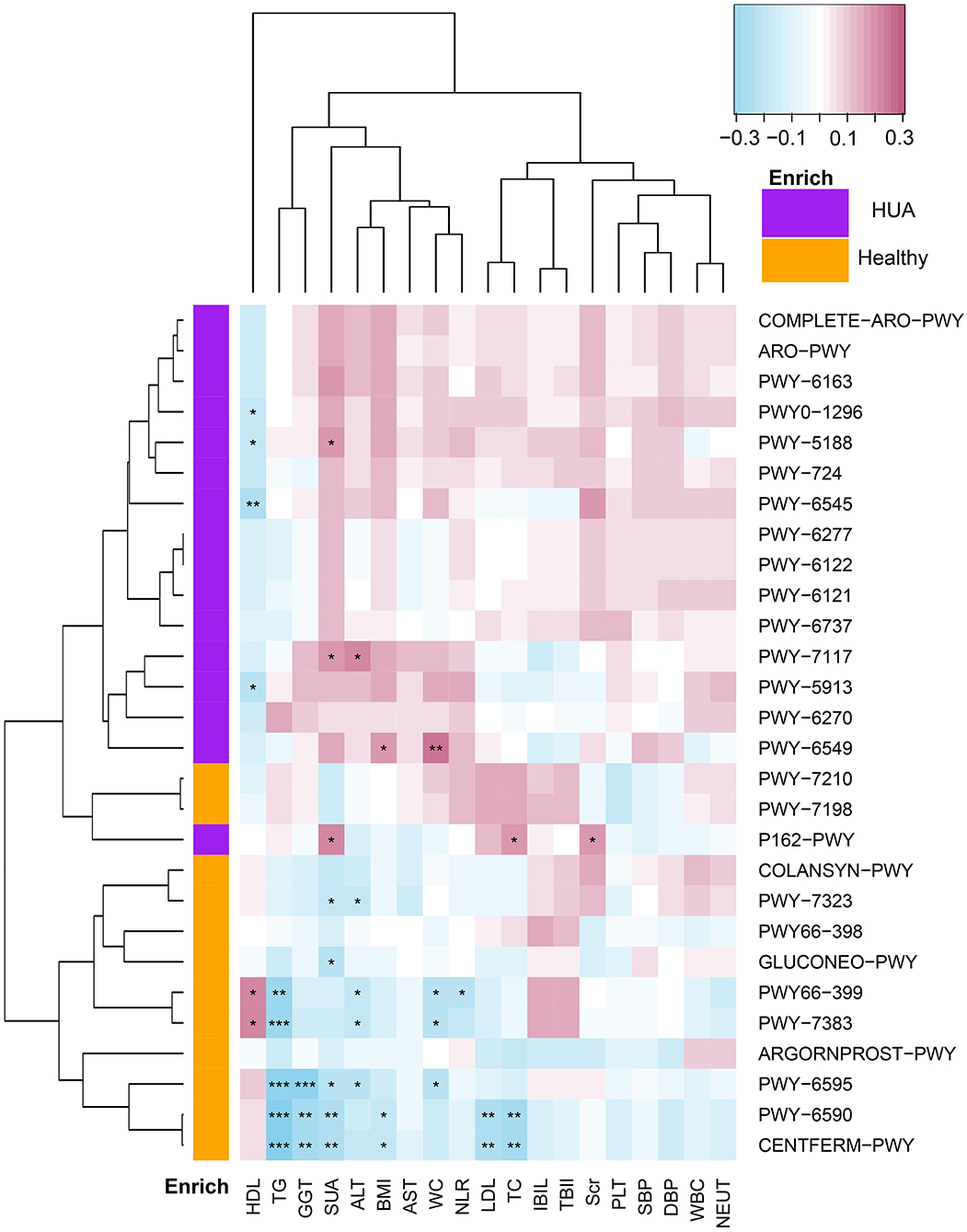
Figure 4. Spearman's correlation matrix for HUA group and healthy controls correlated pathways and clinical characteristics. Different cell color represented for relative correlation type (purple: negative, red: positive). Cells with *, **, or *** asterisk represented P < 0.05, 0.01, or 0.001 respectively.
Correlation Between Microbial Pathways and a Panel of Bacterial Species
Spearman's correlation analyses were applied to analyze the correlations between bacterial species and microbial pathways (P < 0.05) and heatmaps were constructed with Spearman's correlations (Figure 5, Supplementary Table 6). Apparently, most of the bacterial species enriched in healthy controls had highly positive correlations with the pathways enriched in healthy group, and negative correlations with the pathways enriched in HUA group, while those pathways enriched in the HUA group had the opposite correlation trend. In particular, pwy-6590 and TFERM-pwy pathways had the stronger correlation with most of the bacterial species that can produce butyric acid enriched in healthy controls such as Odoribacter splanchnicus, Roseburia hominis, Bacteroides uniformis, Lachnospiraceae bacterium 3_1_46FAA, Ruminococcus callidus, and Butyricimonas synergistica. Besides, Lachnospiraceae bacterium 1_4_56FAA, Ruminococcus gnavuswas and torques, Streptococcus infantis, and Solobacterium moorei had significantly positive correlation with the pathway of producing 5-aminoimidazole ribonucleotide (pwy-6121, pwy-6122 and pwy-6277) and aromatic amino acids (pwy-6316, ARO-PWY and COMPLETE-ARO-PWY), and most of them are conditional pathogens.
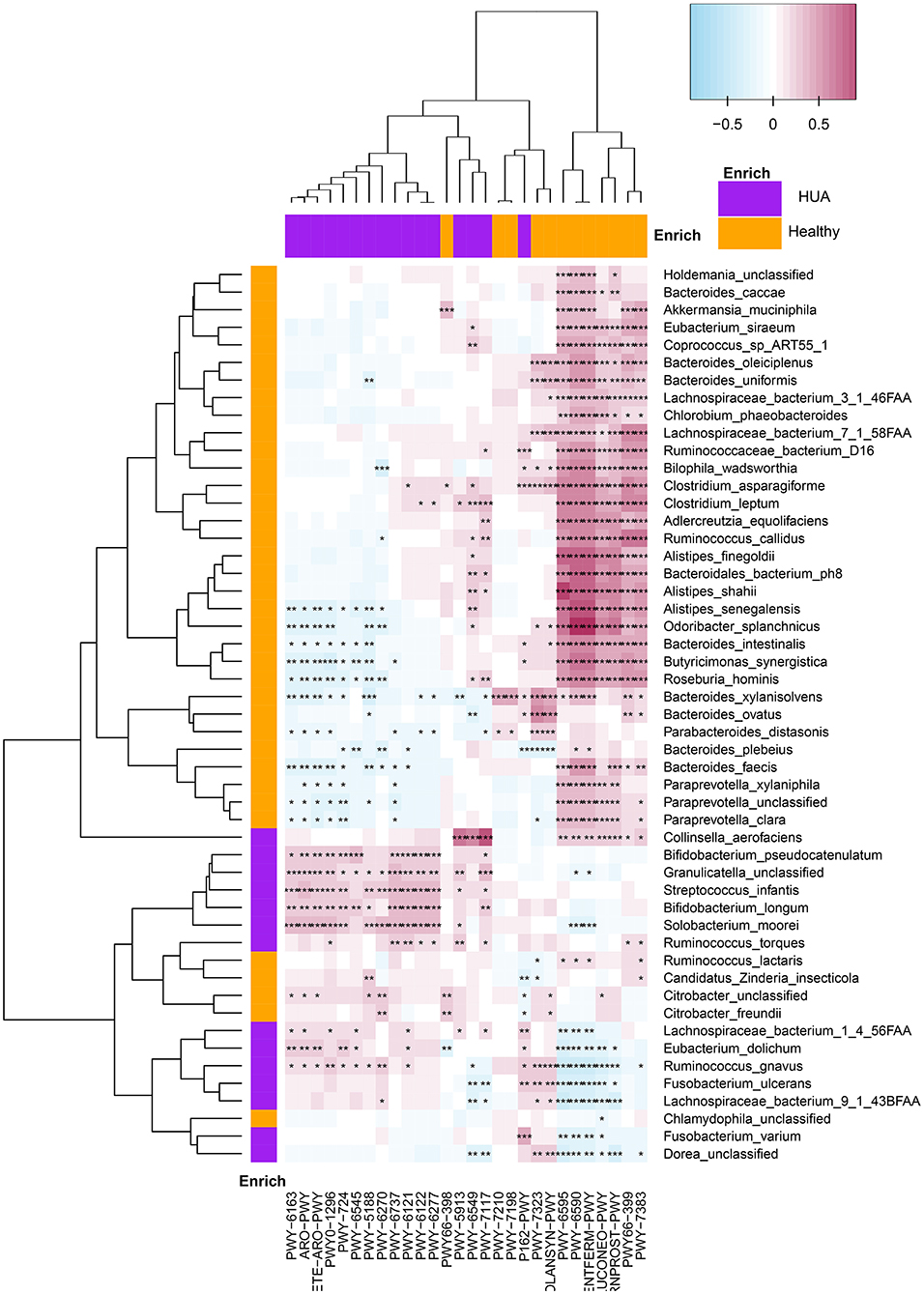
Figure 5. Spearman's correlation matrix for microbial pathways and bacterial species in HUA group and healthy controls. Different cell color represented for relative correlation type (purple: negative, red: positive). Cells with *, **, or *** asterisk represented P < 0.05, 0.01, or 0.001 respectively.
Discussions
It has been recognized that disturbances of the normal gut microbiome participate in the pathogenesis and progression of diverse chronic diseases, such as obesity (16), diabetes (17), hypertension (18), and liver cirrhosis (19), and the effect of gut microbiota in HUA has been explored gradually in recent years. For example, the current known interactions between the gut microbiota and HUA include that certain microbiota can regulate the synthesis and catabolism of purine and uric acid (such as Escherichia coli and Lactobacillus), produce some SCFAs or change the number and distribution of uric acid transporter. All of those studies have shown a strong correlation between the gut microbiota and HUA. However, most of them are animal experiments and the microbiota sequencing method is 16S rRNA, specific alterations of the gut microbiota composition and function between HUA (especially in males with HUA and high levels of liver enzymes) in asymptomatic people for physical examination have been rarely reported.
In view of the fact that most patients with HUA are asymptomatic and the intestinal microecology can change correspondingly in other disease states, our study is the first to select asymptomatic people for physical examination as the research subjects to explore the gut microbiome disturbances in males with HUA and high levels of liver enzymes and choose whole-genome shotgun sequencing technology, which are the two highlights and advantages of this research. Our results elucidated that there may do exist some targeted biomarkers of the gut microbiota and have the potential to be used as non-invasive, safe, and easy diagnostic tools for HUA. The new targeted biomarkers could be applied as a supplement to the traditional HUA diagnostic method to some extent.
In this study, we found that the level of SUA accompanied by WC, BMI, AST, ALT, GGT, Scr, and TG in the HUA group were significantly higher than that of the control group, while HDL was lower than the control group. Besides, the levels of FPG, blood pressure, TC, LDL, and inflammation indicators (WBC, PLT, NEUT, and NLR) were slightly, but not significantly, higher in the HUA group than in the healthy controls, TBIL and IBIL were of the opposite trend. Previous studies have confirmed that HUA is closely related to the occurrence of gout, metabolic syndrome, type 2 diabetes, hypertension, cardiovascular disease, chronic kidney disease, and so on, and it is an independent risk factor for the disease (20), which is consistent with our results. Numerous studies have shown that HUA is closely related to metabolic syndrome (obesity, hypertension, hyperlipidemia, insulin resistance), and the two factors are mutually causal and form a vicious circle (21–24). This comorbidity of chronic diseases suggests that there is a certain correlation between chronic diseases. Our results also suggest that HUA is associated with obesity and metabolism-related indicators, such as high levels of liver enzymes (5, 25), worse homeostasis in lipid and bilirubin metabolism, and poorer kidney function. Although the mean hepatic function was within the “current consensus normal reference range,” it had been already at a high level. Study has verified that when ALT exceeds 26 U/L, the occurrence of liver fibrosis increased, and it is suitable to adjust the reference range of liver function (26). In short, HUA is a metabolic disease related to habits, customs, age, gender, and inherited factors, and HUA is also correlated with obesity, hypertension, diabetes, renal failure, dyslipidemia, and liver diseases (27–29). Of course, the specific mechanism needs further study.
As for the gut microbiotas, we found that the gut bacterial diversity in HUA group was reduced significantly compared with that of healthy controls and the community of the microbiota was also of significant difference between the two groups. Just as Requena et al. (30) had mentioned the diversity of microbiota was closely associated with our health, the result of our study indicated that the gut microbiota may have altered significantly from a normal healthy status to the development of HUA. In the phyla level, compared with healthy controls, the abundance of Bacteroidetes in HUA group decreased [consistent with the previous research result (31)], while Firmicutes and Actinobacteria increased significantly. At the species level, totally 51 species were of significant difference after removing the low occurrence rate and low abundance species. Among them, 37 species were enriched in the healthy controls and 14 species were enriched in the HUA group. This suggests that maybe it is these significantly different populations in abundance that cause the significant changes in the composition of gut microbiota HUA.
Among the 51 species, compared with the species of healthy controls, the relative abundances of Bacteroidetes (Bacteroides uniformis, caccae, ovatus and plebeius, Alistipes finegoldii, senegalensis, and shahii); Firmicutes (Roseburia hominis, Ruminococcus callidus and lactaris, and Lachnospiraceae bacterium 3_1_46FAA); other (Adlercreutzia equolifaciens, Odoribacter splanchnicus, Butyricimonas synergistica, and Akkermansia muciniphila) decreased in the HUA group; while Firmicutes (Ruminococcus gnabus and torques, Lachnospiraceae bacterium 9_1_43FAA); Actinobacteria (Collinsella aerofaciens) increased. Interestingly, most of the gut microbiomes enriched in HUA group not only had significant positive correlations with the clinical characteristics, but also most of them are responsible for the production of SCFAs, while those microbiomes enriched in the healthy controls had highly negatively correlated with these clinical indexes.
In our study, some species that are known as SCFAs (e.g. butanoate) producer [Roseburia hominis (32, 33), Odoribacter splanchnicus (34), Ruminococcus callidus (35), Lachnospiraceae bacterium 3_1_46FAA (36), Bacteroides uniformis (37) and Butyricimonas synergistica (38)], together with the metabolic pathway of producing butanoate (CENTFERM-PWY and PWY-6590) were enriched in the healthy controls. Importantly, both of the two pathways had significantly positive correlations with the above bacterials. Especially, the bacterial of Odoribacter splanchnicus had the strongest correlation with them (r = 0.903 and 0.902 relatively) and had frequent correlations with clinical parameters, Roseburia hominis was the bacteria that most negatively correlated with clinical parameters and can produce large amounts of SCFAs, which play key protective roles against inflammation (39–41). As is known to all, Odoribacter splanchnicus is expressed in human intestine and its complete genome had been sequenced (42), and now it is recognized as a common butyric-acid producing bacterium, which has been reclassified from Bacteroides splanchnicus (34). In a recent study, Odoribacter splanchnicus together with Akkermansia muciniphila, has been associated with a healthy fasting serum lipid profile (43). The administration of prebiotics and the use of polyphenols have been stated as effective dietary strategies to modulate gut microbiota composition, which could induce the abundance of friendly bacteria, namely Akkermansia muciniphila, associated to health benefits in metabolic syndrome (44–46). Our study evidenced that HUA state may reduce or inhibit the growth of those “beneficial” bacterial members.
Besides, another relevant bacterium was Adlercreutzia equolifaciens, which was enriched in the healthy controls. A. equolifaciens is involved in metabolizing polyphenols and produced bioactive molecules involved in ameliorating metabolic disorders in obesity and diabetes (47). The study had verified that it can convert ingested isoflavones, which is abundant in legumes and soya beans, into equol (48). Equol has a high affinity for the estrogen receptor (49) and may be a selective estrogen receptor modulator. The incidence of HUA is high in men and postmenopausal women, and studies have concluded that it is the estrogen in premenopausal women that reduces its incidence through the follow ways, such as estrogen can directly affect the kidney excretion of uric acid by regulating transporters (49–51); inhibit the xanthine oxidase system (52); reduce the production of uric acid by maintaining the stability of lipid metabolism (53–55). Thus, we can propose Adlercreutzia equolifaciens may make contributions to alleviate the damage of HUA to our body in some estrogen-protection way. This finding is of great significance and maybe can provide a direction for the further exploration of new therapeutic targets for HUA based on gut microbiota.
On the contrary, Lachnospiraceae bacterium 1_4_56FAA, Ruminococcus gnavuswas and torques were enriched in the HUA group. Not only did they had positive correlations with most clinical indexes, but also the pathways that are significantly enriched in HUA group, such as superpathway biosynthesis of 5-aminoimidazole ribonucleotide (PWY-6122, PWY-6277 and PWY-6121), aromatic amino acids and chorismate synthesis (COMPLETE-ARO-PWY, ARO-PWY and PWY-6163), and L-lysine, L-threonine and L-methionine biosynthesis (PWY-724). Consistent with our results, all of the current studies have shown that the above three bacterials are closely related to immune inflammation in different diseases (56–60), though the inflammation indicators (WBC, PLT, NEUT, and NLR) were slightly, but not significantly, higher in the HUA group than in the healthy controls. Especially, R. gnavus can produce specific antigens and stimulates immune cells to produce corresponding antibodies, thus increasing inflammation (61, 62). As to the functional alterations, 5-aminoimidazole ribonucleotide is the key intermediate for purine nucleotide biosynthesis, the increased biosynthesis of 5-aminoimidazole ribonucleotide may increase the production of purines, thereby increase the level of uric acid. Besides, the aromatic amino acids and chorismate can act as substrates in other secondary metabolite pathways, such as indole derivatives and phenolic compounds, which are just the metabolites of our gut microbiotas. So far, no studies have been able to confirm the correlations between these structural and functional alterations of gut microbiota in HUA, but their significant alterations suggest that they may have important physiological significance in the occurrence and development of HUA, which is a research direction worthy for our further study.
There are some limitations in our study. First, this is a cross-sectional study, and it is not able to verify the causality between the discovered species and HUA, hence, further germ-free mice studies are still needed. Second, the study subjects come from the same region, thus, it is better to conduct a multicenter study from different regions owing to the influence of different regions and eating habits on the gut microbiota. Third, the gender in the HUA group is male adult, therefore, it is necessary to be cautious temporarily to apply the results obtained in this article to the female, minors or other population.
In conclusion, our study demonstrated the structural and functional alterations of gut microbiota in males with HUA and high levels of liver enzymes. In our study, the main clues of the correlation mechanisms between HUA and gut microbiome point to the pathways that can produce 5-aminoimidazole ribonucleotide, aromatic amino acids, and chorismate, which were enriched in the HUA group; the pathways that can produce SCFAs and the gut microbiotas that can produce SCFAs and equol were enriched in healthy controls. Generally, the potential mechanisms underlying the gut microbiome-Equol/SCFAs-uric acid accumulation link remains much for further exploration. The novel correlations between some microbiota species/pathways and uric acid accumulation could provide brand new directions for specific microbiota-targeted therapies.
Data Availability Statement
The datasets presented in this study can be found in online repositories. The names of the repository/repositories and accession number(s) can be found at: https://www.ebi.ac.uk/ena,PRJEB48022.
Ethics Statement
The studies involving human participants were reviewed and approved by Ethics Committee from the First Affiliated Hospital of Zhengzhou University. The patients/participants provided their written informed consent to participate in this study.
Author Contributions
SD and SS: Conceptualization. JC, YW, and AL: Methodology and formal analysis. QQ, YZ, WL, TL, and XG: Resources. SS: Writing and original draft preparation. SD, LT, and AL: Writing and review and editing. JC and SY: Visualization. All authors have read and agreed to submit the manuscript.
Funding
This research was equally supported and funded by the Henan Province Medical Science and Technology Research Plan (LHGJ20200311 and LHGJ20200279), Chinese National Science and Technology Major Project (2018ZX10305410), Henan Province Youth Talent Promotion Project (Grant No.2021HYTP052), the Henan Province Postdoctoral Research Grant (001801005).
Conflict of Interest
The authors declare that the research was conducted in the absence of any commercial or financial relationships that could be construed as a potential conflict of interest.
Publisher's Note
All claims expressed in this article are solely those of the authors and do not necessarily represent those of their affiliated organizations, or those of the publisher, the editors and the reviewers. Any product that may be evaluated in this article, or claim that may be made by its manufacturer, is not guaranteed or endorsed by the publisher.
Acknowledgments
We sincerely thank the group members from Henan Genomics Hospital trial. We also thank the clinicians and the participants who were enrolled in this study. We also thank Traci Raley, MS, ELS, from Liwen Bianji (Edanz) (www.liwenbianji.cn/) for editing the language of a draft of this manuscript.
Supplementary Material
The Supplementary Material for this article can be found online at: https://www.frontiersin.org/articles/10.3389/fmed.2021.779994/full#supplementary-material
Supplementary Table. The detailed experimental results between HUA group and healthy controls.
Abbreviations
WC, waist circumference; BMI, body mass index; FPG, fasting plasma glucose; DBP, diastolic blood pressure; SBP, systolic blood pressure; AST, aspartate aminotransferase; ALT, alanine aminotransferase; GGT, glutamyl transpeptidase; TBIL, total bilirubin; IBIL, indirect bilirubin; SUA, serum uric acid; Scr, serum creatinine; TC, total cholesterol; TG, triglyceride; LDL, low-density lipoprotein; HDL, high-density lipoprotein; WBC, white blood cell; PLT, platelet; NEUT, absolute value of neutrophil; NLR, neutrophil to lymphocyte ratio; SCFAs, short-chain fatty acids.
References
1. Kopel E. Serum uric acid and cardiovascular risk: an early wake-up call. J Adolesc Health. (2015) 56:363–4. doi: 10.1016/j.jadohealth.2015.01.014
2. Wu J, Qiu L, Cheng XQ, Xu T, Wu W, Zeng XJ. Hyperuricemia and clustering of cardiovascular risk factors in the Chinese adult population. Sci Rep. (2017) 7:5456. doi: 10.1038/s41598-017-05751-w
3. Sorensen LB, Levinson DJ. Origin and extrarenal elimination of uric acid in man. Nephron. (1975) 14:7–20. doi: 10.1159/000180432
4. Lloyd-Price J, Abu-Ali G, Huttenhower C. The healthy human microbiome. Genome Med. (2016) 8:51. doi: 10.1186/s13073-016-0307-y
5. Yu Y, Liu Q, Li H, Wen C, He Z. Alterations of the gut microbiome associated with the treatment of hyperuricaemia in male rats. Front Microbiol. (2018) 9:2233. doi: 10.3389/fmicb.2018.02233
6. Cao T, Li X, Mao T, Liu H, Zhao QX, Ding X, et al. Probiotic therapy alleviates hyperuricemia in C57BL/6 mouse model. Biomed Res. (2017) 28:2244–9.
7. Yamada N, Iwamoto C, Kano H, Yamaoka N, Fukuuchi T, Kaneko K. Evaluation of purine utilization by Lactobacillus gasseri strains with potential to decrease the absorption of food-derived purines in the human intestine. Nucleos Nucleot Nucl. (2016) 35:670–6. doi: 10.1080/15257770.2015.1125000
8. Vieira AT, Macia L, Galvao I, Martins FS, Canesso MC, Amaral FA, et al. Role for gut microbiota and the Metabolite-Sensing receptor GPR43 in a murine model of gout. Arthritis Rheumatol. (2015) 67:1646–56. doi: 10.1002/art.39107
9. Vieira AT, Galvao I, Macia LM, Sernaglia EM, Vinolo MA, Garcia CC. Dietary fiber and the short-chain fatty acid acetate promote resolution of neutrophilic inflammation in a model of gout in mice. J Leukoc Biol. (2017) 101:275–84. doi: 10.1189/jlb.3A1015-453RRR
10. Cleophas MC, Crisan TO, Lemmers H, Toenhake-Dijkstra H, Fossati G, Jansen TL. Suppression of monosodium urate crystal-induced cytokine production by butyrate is mediated by the inhibition of class I histone deacetylases. Ann Rheum Dis. (2016) 75:593–600. doi: 10.1136/annrheumdis-2014-206258
11. Ichida K, Matsuo H, Takada T, Nakayama A, Murakami K, Shimizu T. Decreased extra-renal urate excretion is a common cause of hyperuricemia. Nat Commun. (2012) 3:764. doi: 10.1038/ncomms1756
12. Matsuo H, Tsunoda T, Ooyama K, Sakiyama M, Sogo T, Takada T. Hyperuricemia in acute gastroenteritis is caused by decreased urate excretion via ABCG2. Sci Rep. (2016) 6:31003. doi: 10.1038/srep31003
13. Fang C, Zhong H, Lin Y, Chen B, Han M, Ren H. Assessment of the cPAS-based BGISEQ-500 platform for metagenomic sequencing. Gigascience. (2018) 7:1–8. doi: 10.1093/gigascience/gix133
14. Li A, Li T, Gao X, Yan H, Chen J, Huang M, et al. Gut microbiome alterations in patients with thyroid nodules. Front Cell Infect Microbiol. (2021) 11:643968. doi: 10.3389/fcimb.2021.643968
15. Truong DT, Franzosa EA, Tickle TL, Scholz M, Weingart G, Pasolli E. MetaPhlAn2 for enhanced metagenomic taxonomic profiling. Nat Methods. (2015) 12:902–3. doi: 10.1038/nmeth.3589
16. Ley RE, Turnbaugh PJ, Klein S, Gordon JI. Microbial ecology: Human gut microbes associated with obesity. Nature. (2006) 444:1022–3. doi: 10.1038/4441022a
17. Qin J, Li Y, Cai Z, Li S, Zhu J, Zhang F, et al. A metagenome-wide association study of gut microbiota in type 2 diabetes. Nature. (2012) 490:55–60. doi: 10.1038/nature11450
18. Manco M, Putignani L, Bottazzo GF. Gut microbiota, lipopolysaccharides, and innate immunity in the pathogenesis of obesity and cardiovascular risk. Endocr Rev. (2010) 31:817–44. doi: 10.1210/er.2009-0030
19. Qin N, Yang F, Li A, Prifti E, Chen Y, Shao L, et al. Alterations of the human gut microbiome in liver cirrhosis. Nature. (2014) 513:59–64. doi: 10.1038/nature13568
20. Zhu Y, Pandya BJ, Choi HK. Comorbidities of gout and hyperuricemia in the US general population: NHANES 2007-2008. Am J Med. (2012) 125:679–87. doi: 10.1016/j.amjmed.2011.09.033
21. Yang T, Chu CH, Bai CH, You SL, Chou YC, Chou WY. Uric acid level as a risk marker for metabolic syndrome: a Chinese cohort study. Atherosclerosis. (2012) 220:525–31. doi: 10.1016/j.atherosclerosis.2011.11.014
22. Lee JW, Cho YK, Ryan M, Kim H, Lee SW, Chang E. Serum uric Acid as a predictor for the development of nonalcoholic Fatty liver disease in apparently healthy subjects: a 5-year retrospective cohort study. Gut Liver. (2010) 4:378–83. doi: 10.5009/gnl.2010.4.3.378
23. Xu C, Yu C, Xu L, Miao M, Li Y. serum uric acid increases the risk for nonalcoholic Fatty liver disease: A prospective observational study. PLoS ONE. (2010) 5:e11578. doi: 10.1371/journal.pone.0011578
24. Sertoglu E, Ercin CN, Celebi G, Gurel H, Kayadibi H, Genc H, et al. The relationship of serum uric acid with non-alcoholic fatty liver disease. Clin Biochem. (2014) 47:383–8. doi: 10.1016/j.clinbiochem.2014.01.029
25. Chen S, Guo X, Yu S, Sun G, Yang H, Li Z, et al. Association between serum uric acid and elevated alanine aminotransferase in the general population. Int J Environ Res Public Health. (2016) 13:841. doi: 10.3390/ijerph13090841
26. Lomonaco R, Godinez LE, Bril F, Shrestha S, Mansour L, Budd J. Advanced liver fibrosis is common in patients with type 2 diabetes followed in the outpatient setting: the need for systematic screening. Diabetes Care. (2021) 44:399–406. doi: 10.2337/dc20-1997
27. Zhang N, Chang Y, Guo X, Chen Y, Ye N, Sun YAB. and Body Roundness Index: Two new body indices for detecting association between obesity and hyperuricemia in rural area of China. Eur J Intern Med. (2016) 29:32–6. doi: 10.1016/j.ejim.2016.01.019
28. Cho J, Kim C, Kang DR, Park JB. Hyperuricemia and uncontrolled hypertension in treated hypertensive patients: K-MetS study. Medicine. (2016) 95:e4177. doi: 10.1097/MD.0000000000004177
29. Sattui SE, Gaffo AL. Treatment of hyperuricemia in gout: current therapeutic options, latest developments and clinical implications. Ther Adv Musculoskelet Dis. (2016) 8:145–59. doi: 10.1177/1759720X16646703
30. Requena T, Martinez-Cuesta MC, Pelaez C. Diet and microbiota linked in health and disease. Food Funct. (2018) 9:688–704. doi: 10.1039/C7FO01820G
31. Do MH, Lee E, Oh MJ, Kim Y, Park HY. High-Glucose or -Fructose diet cause changes of the gut microbiota and metabolic disorders in mice without body weight change. Nutrients. (2018) 10:761 doi: 10.3390/nu10060761
32. Li X, Jberg H, Canibe O, Jensen N, Phylogenetic BB. diversity of cultivable butyrate-producing bacteria from pig gut content and feces. J Anim Sci. (2016) 94:377–81. doi: 10.2527/jas.2015-9868
33. Machiels K, Joossens M, Sabino J, De Preter V, Arijs I, Eeckhaut V, et al. A decrease of the butyrate-producing species Roseburia hominis and Faecalibacterium prausnitzii defines dysbiosis in patients with ulcerative colitis. Gut. (2014) 63:1275–83. doi: 10.1136/gutjnl-2013-304833
34. Asano K, Yoshimura S, Nakane A. Alteration of intestinal microbiota in mice orally administered with salmon cartilage proteoglycan, a prophylactic agent. PLoS ONE. (2013) 8:e75008. doi: 10.1371/journal.pone.0075008
35. Ye X, Zhou L, Zhang Y, Xue S, Gan QF, Fang S. Effect of host breeds on gut microbiome and serum metabolome in meat rabbits. BMC Vet Res. (2021) 17:24. doi: 10.1186/s12917-020-02732-6
36. Wu IW, Lee CC, Hsu HJ, Sun CY, Chen YC, Yang KJ. Compositional and functional adaptations of intestinal microbiota and related metabolites in CKD patients receiving dietary protein restriction. Nutrients. (2020) 12:nu12092799. doi: 10.3390/nu12092799
37. Zhu W, Yan J, Zhi C, Zhou Q, Yuan X. 1,25(OH)2D3 deficiency-induced gut microbial dysbiosis degrades the colonic mucus barrier in Cyp27b1 knockout mouse model. Gut Pathog. (2019) 11:8. doi: 10.1186/s13099-019-0291-z
38. Sakamoto M, Takagaki A, Matsumoto K, Kato Y, Goto K, Benno Y. Butyricimonas synergistica gen. Nov, Sp Nov And Butyricimonas virosa sp Nov, Butyric acid-producing bacteria in the family ‘Porphyromonadaceae’ isolated from rat faeces. Int J Syst Evol Microbiol. (2009) 59:1748–53. doi: 10.1099/ijs.0.007674-0
39. Chu JR, Kang SY, Kim SE, Lee SJ, Lee YC, Sung MK. Prebiotic UG1601 mitigates constipation-related events in association with gut microbiota: a randomized placebo-controlled intervention study. World J Gastroenterol. (2019) 25:6129–44. doi: 10.3748/wjg.v25.i40.6129
40. Ticinesi A, Mancabelli L, Tagliaferri S, Nouvenne A, Milani C, Del Rio D, et al. The Gut-Muscle axis in older subjects with low muscle mass and performance: a proof of concept study exploring fecal microbiota composition and function with shotgun metagenomics sequencing. Int J Mol Sci. (2020) 21:8946. doi: 10.3390/ijms21238946
41. Zheng J, Hoffman KL, Chen JS, Shivappa N, Sood A, Browman GJ. Dietary inflammatory potential in relation to the gut microbiome: Results from a cross-sectional study. Br J Nutr. (2020) 124:931–42. doi: 10.1017/S0007114520001853
42. Goker M, Gronow S, Zeytun A, Nolan M, Lucas S, Lapidus A. Complete genome sequence of Odoribacter splanchnicus type strain (1651/6). Stand Genomic Sci. (2011) 4:200–9. doi: 10.4056/sigs.1714269
43. Brahe LK, Le Chatelier E, Prifti E, Pons N, Kennedy S, Hansen T. Specific gut microbiota features and metabolic markers in postmenopausal women with obesity. Nutr Diabetes. (2015) 5:e159. doi: 10.1038/nutd.2015.9
44. Marchesi JR, Adams DH, Fava F, Hermes GD, Hirschfield GM, Hold G. The gut microbiota and host health: a new clinical frontier. Gut. (2016) 65:330–9. doi: 10.1136/gutjnl-2015-309990
45. Anhe FF, Roy D, Pilon G, Dudonne S, Matamoros S, Varin TV, et al. Polyphenol-rich cranberry extract protects from diet-induced obesity, insulin resistance and intestinal inflammation in association with increased Akkermansia spp. Population in the gut microbiota of mice. Gut. (2015) 64:872–83. doi: 10.1136/gutjnl-2014-307142
46. Everard A, Lazarevic V, Derrien M, Girard M, Muccioli GG, Neyrinck AM. Responses of gut microbiota and glucose and lipid metabolism to prebiotics in genetic obese and diet-induced leptin-resistant mice. Diabetes. (2011) 60:2775–86. doi: 10.2337/db11-0227
47. Maruo T, Sakamoto M, Ito C, Toda T, Benno Y. Adlercreutzia equolifaciens gen. Nov, Sp Nov, An equol-producing bacterium isolated from human faeces, and emended description of the genus Eggerthella. Int J Syst Evol Microbiol. (2008) 58:1221–7. doi: 10.1099/ijs.0.65404-0
48. Setchell KD, Zhao X, Jha P, Heubi JE, Brown NM. The pharmacokinetic behavior of the soy isoflavone metabolite S-(-)equol and its diastereoisomer R-(+)equol in healthy adults determined by using stable-isotope-labeled tracers. Am J Clin Nutr. (2009) 90:1029–37. doi: 10.3945/ajcn.2009.27981
49. Takiue Y, Hosoyamada M, Kimura M, Saito H. The effect of female hormones upon urate transport systems in the mouse kidney. Nucleosides Nucleotides Nucleic Acids. (2011) 30:113–9. doi: 10.1080/15257770.2010.551645
50. Zeng M, Chen B, Qing Y, Xie W, Dang W, Zhao M. Estrogen receptor beta signaling induces autophagy and downregulates Glut9 expression. Nucleosides Nucleotides Nucleic Acids. (2014) 33:455–65. doi: 10.1080/15257770.2014.885045
51. Ee PL, Kamalakaran S, Tonetti D, He X, Ross DD, Beck WT. Identification of a novel estrogen response element in the breast cancer resistance protein (ABCG2) gene. Cancer Res. (2004) 64:1247–51. doi: 10.1158/0008-5472.CAN-03-3583
52. Budhiraja R, Kayyali US, Karamsetty M, Fogel M, Hill NS, Chalkley R. Estrogen modulates xanthine dehydrogenase/xanthine oxidase activity by a receptor-independent mechanism. Antioxid Redox Signal. (2003) 5:705–11. doi: 10.1089/152308603770380007
53. Zhang Y, Klein K, Sugathan A, Nassery N, Dombkowski A, Zanger UM. Transcriptional profiling of human liver identifies sex-biased genes associated with polygenic dyslipidemia and coronary artery disease. PLoS ONE. (2011) 6:e23506. doi: 10.1371/journal.pone.0023506
54. Palmisano BT, Zhu L, Stafford JM. Role of estrogens in the regulation of liver lipid metabolism. Adv Exp Med Biol. (2017) 1043:227–56. doi: 10.1007/978-3-319-70178-3_12
55. Bryzgalova G, Gao H, Ahren B, Zierath JR, Galuska D, Steiler TL. Evidence that oestrogen receptor-alpha plays an important role in the regulation of glucose homeostasis in mice: Insulin sensitivity in the liver. Diabetologia. (2006) 49:588–97. doi: 10.1007/s00125-005-0105-3
56. Zeng H, Ishaq SL, Zhao FQ, Wright AG. Colonic inflammation accompanies an increase of beta-catenin signaling and Lachnospiraceae/Streptococcaceae bacteria in the hind gut of high-fat diet-fed mice. J Nutr Biochem. (2016) 35:30–6. doi: 10.1016/j.jnutbio.2016.05.015
57. Hansen SG, Skov MN, Justesen US. Two cases of Ruminococcus gnavus bacteremia associated with diverticulitis. J Clin Microbiol. (2013) 51:1334–6. doi: 10.1128/JCM.03382-12
58. Kim YJ, Kang HY, Han Y, Lee MS, Lee HJA. Bloodstream infection by Ruminococcus gnavus in a patient with a gall bladder perforation. Anaerobe. (2017) 47:129–31. doi: 10.1016/j.anaerobe.2017.05.007
59. Roux AL, El SF, Duffiet P, Bauer T, Heym B, Gaillard JL. Ruminococcus gnavus total hip arthroplasty infection in a 62-year-old man with ulcerative colitis. J Clin Microbiol. (2015) 53:1428–30. doi: 10.1128/JCM.03040-14
60. Mutlu EA, Keshavarzian A, Losurdo J, Swanson G, Siewe B, Forsyth C. A compositional look at the human gastrointestinal microbiome and immune activation parameters in HIV infected subjects. PLoS Pathog. (2014) 10:e1003829. doi: 10.1371/journal.ppat.1003829
61. Bunker JJ, Drees C, Watson AR, Plunkett CH, Nagler CR, Schneewind O, et al. B cell superantigens in the human intestinal microbiota. Sci Transl Med. (2019) 11:507. doi: 10.1126/scitranslmed.aau9356
Keywords: uric acid, liver enzymes, short-chain fatty acids, purine, aromatic amino acids, gut microbiota, equol
Citation: Sheng S, Chen J, Zhang Y, Qin Q, Li W, Yan S, Wang Y, Li T, Gao X, Tang L, Li A and Ding S (2021) Structural and Functional Alterations of Gut Microbiota in Males With Hyperuricemia and High Levels of Liver Enzymes. Front. Med. 8:779994. doi: 10.3389/fmed.2021.779994
Received: 20 September 2021; Accepted: 15 October 2021;
Published: 19 November 2021.
Edited by:
Ding Shi, Zhejiang University, ChinaReviewed by:
Ana Isabel Alvarez- Mercado, University of Granada, SpainYf Gu, Zhejiang University, China
Copyright © 2021 Sheng, Chen, Zhang, Qin, Li, Yan, Wang, Li, Gao, Tang, Li and Ding. This is an open-access article distributed under the terms of the Creative Commons Attribution License (CC BY). The use, distribution or reproduction in other forums is permitted, provided the original author(s) and the copyright owner(s) are credited and that the original publication in this journal is cited, in accordance with accepted academic practice. No use, distribution or reproduction is permitted which does not comply with these terms.
*Correspondence: Suying Ding, ZmNjZGluZ3N5JiN4MDAwNDA7enp1LmVkdS5jbg==; Lin Tang, dGFuZ2xpbiYjeDAwMDQwO3p6dS5lZHUuY24=; Ang Li, bGlhJiN4MDAwNDA7emp1LmVkdS5jbg==