- 1Department of Respiratory and Critical Care Medicine, National Clinical Research Center of Respiratory Disease, Key Laboratory of Pulmonary Diseases of Health Ministry, Tongji Hospital, Tongji Medical College, Huazhong University of Science and Technology, Wuhan, China
- 2Department of Science, Western University, London, ON, Canada
- 3Department of Pulmonary and Critical Care Medicine, The First Affiliated Hospital of Sun Yat-sen University, Guangzhou, China
- 4Institute of Respiratory Diseases of Sun Yat-sen University, Guangzhou, China
Background: Aging is a strong risk factor and an independent prognostic factor in idiopathic pulmonary fibrosis (IPF). In this study, we aimed to conduct a comprehensive analysis based on gene expression profiles for the role of aging in pulmonary fibrosis.
Method: Four datasets (GSE21411, GSE24206, GSE47460, and GSE101286) for patients with clinical IPF and one dataset for bleomycin (BLM)-induced pulmonary fibrosis (BIPF) mouse model (GSE123293) were obtained from Gene Expression Omnibus (GEO). According to different age ranges, both patients with IPF and BIPF mice were divided into young and aged groups. The differently expressed genes (DEGs) were systemically analyzed using Gene Ontology (GO) functional, Kyoto Encyclopedia of Genes and Genomes (KEGG), and hub genes analysis. Finally, we verified the role of age and core genes associated with age in vivo.
Results: Via the expression profile comparisons of aged and young patients with IPF, we identified 108 aging-associated DEGs, with 21 upregulated and 87 downregulated. The DEGs were associated with “response to glucocorticoid,” “response to corticosteroid,” and “rhythmic process” in GO biological process (BP). For KEGG analysis, the top three significantly enriched KEGG pathways of the DEGs included “IL-17 signaling pathway,” “Mineral absorption,” and “HIF-1-signaling pathway.” Through the comparisons of aged and young BIPF mice, a total number of 778 aging-associated DEGs were identified, with 453 genes increased and 325 genes decreased. For GO and KEGG analysis, the DEGs were enriched in extracellular matrix (ECM) and collagen metabolism. The common DEGs of patients with IPF and BIPF mice were enriched in the BP category, including “induction of bacterial agglutination,” “hyaluronan biosynthetic process,” and “positive regulation of heterotypic cell-cell adhesion.” We confirmed that aged BIPF mice developed more serious pulmonary fibrosis. Finally, the four aging-associated core genes (Slc2a3, Fga, Hp, and Thbs1) were verified in vivo.
Conclusion: This study provides new insights into the impact of aging on pulmonary fibrosis. We also identified four aging-associated core genes (Slc2a3, Fga, Hp, and Thbs1) related to the development of pulmonary fibrosis.
Introduction
The process of aging is featured by gradual functional impairments of tissue and organisms of the body, leading to reduced resilience to environmental damages and increased risks of occurrence of diseases and death (1). Idiopathic pulmonary fibrosis (IPF) is an age-associated chronic lethal disease caused by an unknown reason (2). It is commonly diagnosed in patients over 50 years old, and the incidence of this disease significantly increases in the population older than 50 years old. (3). For this reason, better understandings of the pathophysiology of IPF and how the process of aging is related to the incidence of IPF are necessary for the development of new therapies for clinical treatments of this disease.
Despite the repeated proposals of the association between aging and IPF, the mechanism underlying this association is still unclear (4, 5). Lopez-Otin et al. enumerate nine tentative aging hallmarks: genomic instability, telomere attrition, epigenetic alterations, loss of proteostasis, deregulated nutrient sensing, mitochondrial dysfunction, cellular senescence, stem cell exhaustion, and altered intercellular communication (6). Meanwhile, heterozygous mutations in four telomere-related genes are also found to associate with the development of pulmonary fibrosis (7). Moreover, biomarkers of aging, including p21 and p16, are also found significantly upregulated in both epithelial cells and fibroblasts in lung tissue of patients with IPF as compared with that of healthy people (8). In animal studies of IPF using the bleomycin (BLM)-induced pulmonary fibrosis (BIPF), aged BIPF mice failed to resolve fibrosis as compared with young mice (9). However, despite the wide-recognized association between aging and IPF, its potential mechanism is unclear (10).
In this study, to investigate the mechanisms underlying the association between pulmonary fibrosis and aging, using gene expression profiles of both patients with IPF and BIPF mice, we comprehensively analyzed the effects of aging in the development of pulmonary fibrosis and verified our findings in in vivo studies.
Method
Microarray Data Acquisition and Process
To identify the genes associated with aging in patients with IPF, four microarray datasets (GSE21411, GSE24206, GSE47460, and GSE101286) were downloaded from Gene Expression Omnibus (GEO, http://www.ncbi.nlm.nih.gov/geo) (11–14). IPF was diagnosed according to the ATS/ERS criteria published (15, 16). Young patients with IPF were defined as age ≤ 55 years old, and aged patients with IPF were defined as age ≥70 years old. Gender- and age-matched healthy controls were gotten from GSE47460. In order to explore the genes associated with age in BIPF mice, we downloaded one microarray dataset (GSE123293) from GEO (17). In this dataset, mice were divided into young (2–4 months old) and aged (18–20 months old) groups. Gender- and age-matched mouse controls were from GSE123293. Detailed information of GSE datasets was summarized in Table 1. Preprocessed expression matrix and probe annotation files of the four GSE datasets were obtained from the GEO repository. Probe annotations and the corresponding expression profiles from different datasets were mapped into a common gene expression list according to the method described by Shi et al. (18). Probes obtained from different datasets were listed using official gene symbols. Multiple expression results of the certain gene were replaced by the median value of the expression results (19, 20). Log2 fold-change (FC) of all expression results was normalized using the Cross-platform Normalization (xpn) method by platforms.
Differently expressed genes (DEGs) analysis, Gene Ontology (GO) functional analysis, and Kyoto Encyclopedia of Genes and Genomes (KEGG) analysis were performed.
To identify DEGs between young and aged groups, the limma R package (http://www.bioconductor.org/packages) was used to perform the negative binomial distribution method according to the absolute value of FC (>1.5) and false discovery rate (FDR) <0.05. According to the hypergeometric distribution algorithm, pathway enrichment analyses of the GO biological process (BP), molecular function (MF), and cell component (CC) were carried out by the “cluster-profler” R package. The same method was applied for the KEGG analysis. The cutoff value was p < 0.05. The Cytoscape (version 3.8.0) was used to select hub or core genes.
Animal
Specific pathogen-free (SPF) C57 BL/6N male mice (young mice, 2–4 months old; aged mice, 18–20 months old) were purchased from the HFK Bioscience company (Beijing, China). All animals were kept under the SPF environment at Tongji Medical College. All procedures for animal experiments were approved by the Animal Care and Use Committee of Tongji Hospital.
The animal model of pulmonary fibrosis was established by single intratracheal administration of BLM (2 mg/kg, MCE, USA) or normal saline (NS) as control. Mice were grouped as follows: NS (young mice intratracheal with NS), aged NS (aged mice intratracheal with NS), BLM (young mice intratracheal with BLM), and aged BLM (aged mice intratracheal with BLM). Mice were sacrificed 21 days after the establishment of the BIPF mice model. Bronchoalveolar lavage fluid (BALF) was carried out. Samples of lung tissue were collected. The left lung was paraffin embedded for hematoxylin-eosin (HE) staining and masson trichrome (MT) staining (21). Lung hydroxyproline contents were measured by hydroxyproline colorimetric assay (Biovision, Milpitas, USA).
Quantitative Real-Time Polymerase Chain Reaction (RT-PCR)
Briefly, the total RNA of the lung tissue was extracted by RNAiso plus kit (TaKaRa) and reversely transcripted to cDNA. RT-PCR was carried out using SYBR Premix Ex Taq (Tarkara, Shiga, Japan). The relative mRNA expression was calculated using the 2−ΔΔCT method normalized to the level of β-actin. The primers of four core genes are listed in Supplementary Table 1.
Statistical Analysis
Data analysis was carried out by Rstudio (version 3.6.2) and GraphPad Prism 7. The microarray data were analyzed by different R packages. Results were expressed as means ± SEM. A two-way analysis of variance (ANOVA) followed by Tukey's multiple comparison post-test was used. p < 0.05 was considered as statistically significant.
Results
DEGs Associated With Age in IPF
To determine the core gene profiles related to age in patients with IPF, four microarray datasets were obtained from GEO. Information of the included studies is listed in Table 1; Supplementary Table 2. According to the age criterion, this study included 19 young patients with IPF and 33 aged patients with IPF. Gene expression profiles were compared between the two groups, generating 108 DEGs, with 21 upregulated and 87 downregulated (Figure 1A). For further understanding of the function related to these 108 DEGs, GO enrichment analysis, including BP, MF, and CC, was performed. In BP analysis, the DEGs were found to associate with “response to glucocorticoid,” “response to corticosteroid,” and “rhythmic process” (Figure 1B). In the CC category, the DEGs were related to the “integral component of postsynaptic membrane,” “intrinsic component of postsynaptic membrane,” and “integral component of synaptic membrane” (Figure 1C). Moreover, DEGs were enriched in the MF category related to “receptor ligand activity” and “growth factor activity” (Figure 1D). For KEGG pathway enrichment analysis, the top three significant KEGG pathways of the DEGs included “IL-17 signaling pathway,” “Mineral absorption,” and “HIF-1-signaling pathway” (Figure 1E). More information on IPF DEGs analysis was shown in Supplementary File 1. The same biological analysis was performed in human young healthy control (HC) and aged HC (Supplementary File 3; Supplementary Figure 1). Different from young and aged IPF, DEGs of young and aged HC were enriched in inflammation and immune.
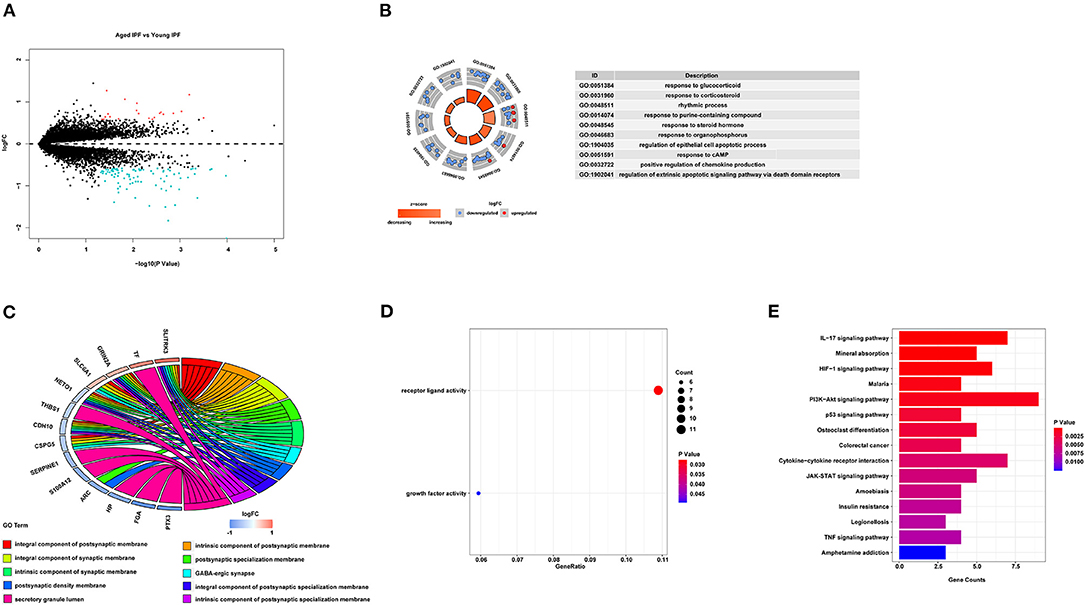
Figure 1. DEGs associated with age in IPF. (A) Volcano-plot of the 108 age-associated DEGs in patients with IPF. Pink circle: upregulated genes with fold change over 1.5; blue circles: downregulated genes with a fold change over 1.5. (B) Top 10 GO biological processes analysis of the 108 age-associated DEGs. Outer gray circle: a scatter plot for each term of the logFC of the assigned genes; red circles: upregulation genes; blue circles: downregulation genes. (C) Top 10 GO cellular components analysis of the 108 age-associated DEGs. (D) All GO molecular functions analysis of the 108 age-associated DEGs. (E) Top 15 significantly enriched KEGG pathways. DEGs, differently expressed genes; IPF, idiopathic pulmonary fibrosis; GO, gene ontology; FC, fold change; KEGG, Kyoto Encyclopedia of Genes and Genomes.
DEGs Associated With Age in BIPF Mice
For further identification of the expression features associated with aging in BIPF mice, we obtained one dataset from GEO and compared the DEGs between aged and young BIPF mice. A total number of 778 DEGs were identified, with 453 genes increased and 325 genes decreased (Figure 2A). Slightly different from the results obtained from patients with IPF, the top five BP terms were enriched in “extracellular matrix organization,” “extracellular structure organization,” “cell chemotaxis,” “collagen metabolic process,” and “neutrophil chemotaxis” (Figure 2B). In the CC category, the DEGs were associated with “extracellular matrix,” “collagen-containing extracellular matrix,” and “extracellular matrix component” (Figure 2C). “Extracellular matrix component,” “glycosaminoglycan binding,” and “collagen binding” were the most important MF terms (Figure 2D). Furthermore, KEGG pathway analysis showed enrichments in “ECM-receptor interaction,” “Cytokine-cytokine receptor interaction,” and “Amoebiasis” (Figure 2E). Detailed information of BIPF DEGs analysis was displayed in Supplementary File 2. The same bioinformatical analysis was conducted in young mouse control (MC) and aged MC (Supplementary File 4; Supplementary Figure 2). Similar to HC age-associated DEGs, inflammation and immune were enriched in DEGs of young and aged MC.
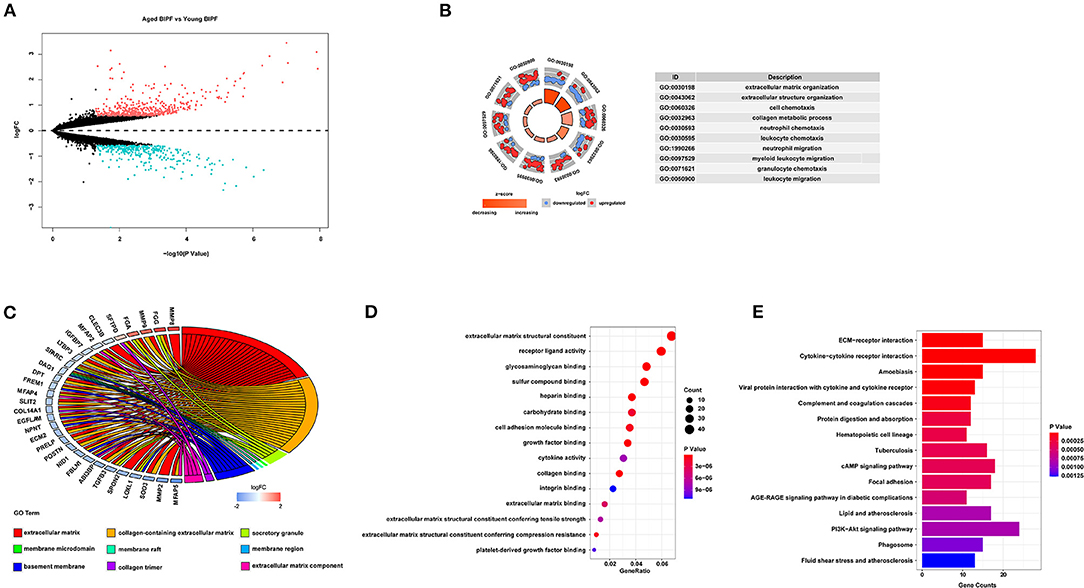
Figure 2. Age-associated DEGs in BIPF mice. (A) Volcano plot of the 778 age-associated DEGs. Pink circle: upregulated genes with fold change over 1.5; blue circles: downregulated genes with a fold change over 1.5. (B) Top 10 GO biological processes of the 778 age-associated DEGs. Outer gray circle: a scatter plot for each term of the logFC of the assigned genes; red circles: upregulation genes; blue circles: downregulation genes. (C) Top nine GO cellular components of the 778 age-associated DEGs. (D) Top 15 GO molecular functions of the 778 age-associated DEGs. (E) Top 15 significantly enriched KEGG pathways. DEGs, differently expressed genes; BIPF, bleomycin-induced pulmonary fibrosis; GO, gene ontology; FC, fold change; KEGG, Kyoto Encyclopedia of Genes and Genomes.
Common DEGs Associated With Age in Pulmonary Fibrosis
To comprehensively explore the effects of aging in pulmonary fibrosis, we combined the results of patients with IPF and BIPF mice. As shown in the Venn diagram, eight common DEGs were found in both aged patients with IPF and aged BIPF mice (Figure 3A). The eight common DEGs were enriched in the BP category related to “induction of bacterial agglutination,” “hyaluronan biosynthetic process,” and “positive regulation of heterotypic cell-cell adhesion” (Figure 3B). In CC analysis, the eight common genes were associated with “extracellular space,” “extracellular exosome,” and “extracellular region” (Figure 3C). Subsequently, to confirm the aging-related core genes, we overlapped the eight common genes with the top 100 core DEGs in BIPF mice. In the results, the four aging-related core genes were identified: solute carrier family 2 member 3 (Slc2a3), fibrinogen alpha chain (Fga), haptoglobin (Hp), and thrombospondin 1 (Thbs1) (Figure 3D). The heatmap showed the four core genes expression in both patients with IPF and BIPF mice (Figures 3E,F). Detailed information on the four core genes is displayed in Table 2. There was no common gene among aged and young IPF DEGs, BIPF DEGs, HC DEGs, and MC DECs (Supplementary Figure 3; Supplementary File 5).
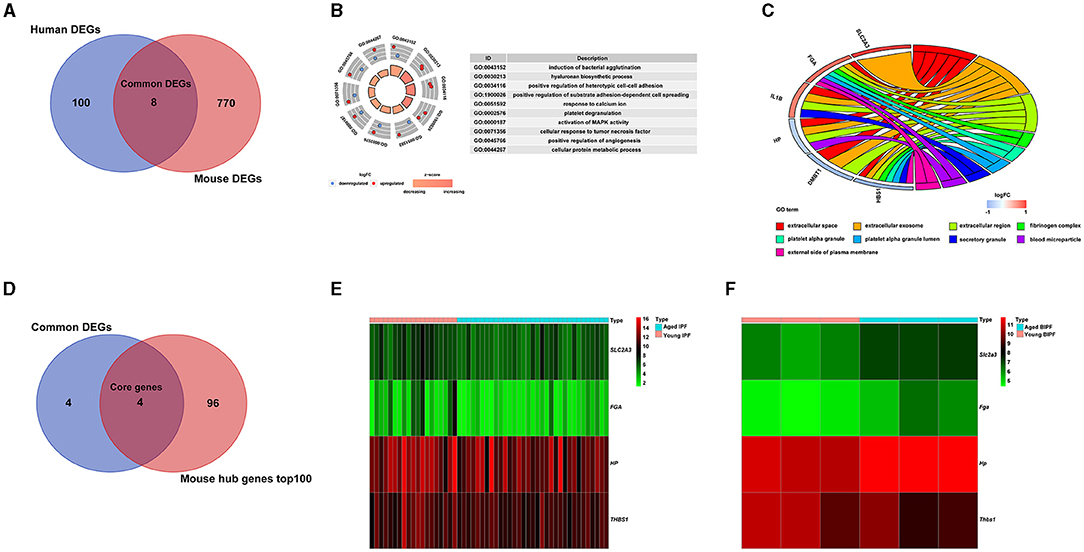
Figure 3. Common age-associated DEGs in pulmonary fibrosis. (A) A Venn diagram of DEGs of patients with IPF and BIPF mice. (B) Significant enrichments of GO biological processes analysis of the eight common DEGs. (C) Significant enrichments of GO cellular components analysis of the eight common DEGs. (D) A Venn diagram of core genes extracted by common DEGs analysis and mouse hub genes datasets. (E) Heatmap of the four core genes in young and aged IPF. (F) Heatmap of the four core genes in young and aged BIPF mice. DEGs, differently expressed genes; IPF, idiopathic pulmonary fibrosis; BIPF, bleomycin-induced pulmonary fibrosis; GO, gene ontology.
In vivo Validations of the Impact of Aging and Four Core Genes
Aging is an important risk factor in patients with IPF. In this study, we compared the fibrosis degree between young and aged mice. In histopathological analysis, we found that, with the increase of age, fibrosis appeared in lung tissue spontaneously (Figures 4A,B). Under the stimulation of BLM, more serious pulmonary fibrosis was developed in aged mice. This finding was consistent with the previous study (5). For further confirmation of the expression changes in the four core genes (Slc2a3, Fga, Hp, and Thbs1) in NS, aged NS, BLM, and aged BLM groups, qRT-PCR verification was conducted. The results demonstrated that Slc2a3 and Fga were significantly increased with age in both NS and BLM-stimulated mice (Figures 5A,B). Meanwhile, the Hp and Thbs1 were found to significantly decreased with the increase of age (Figures 5C,D).
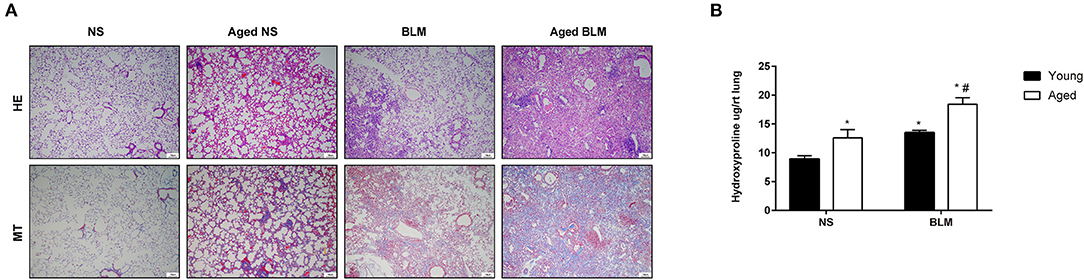
Figure 4. Aging aggravates pulmonary fibrosis in vivo. Young C57BL/6N male mice (2–4 months) and aged C57BL/6 male mice (16–18 months) were intratracheally injected with 50 μl of NS or 2-mg/kg BLM. (A) Representative HE and MT staining images of lung sections of each group. Magnification X200. Scale bar, 10 um. (B) Hydroxyproline measurements. The four groups were NS (young mice intratracheal with NS), aged NS (aged mice intratracheal with NS), BLM (young mice intratracheal with BLM), and aged BLM (aged mice intratracheal with BLM). Values were expressed as mean ± SEM (n = 4). *p < 0.05, compared with the young NS group, #p < 0.05, compared with the young BLM group. Two-way ANOVA was used. NS, normal saline; BLM, bleomycin; HE, hematoxylin-eosin staining; MT, masson trichrome staining.
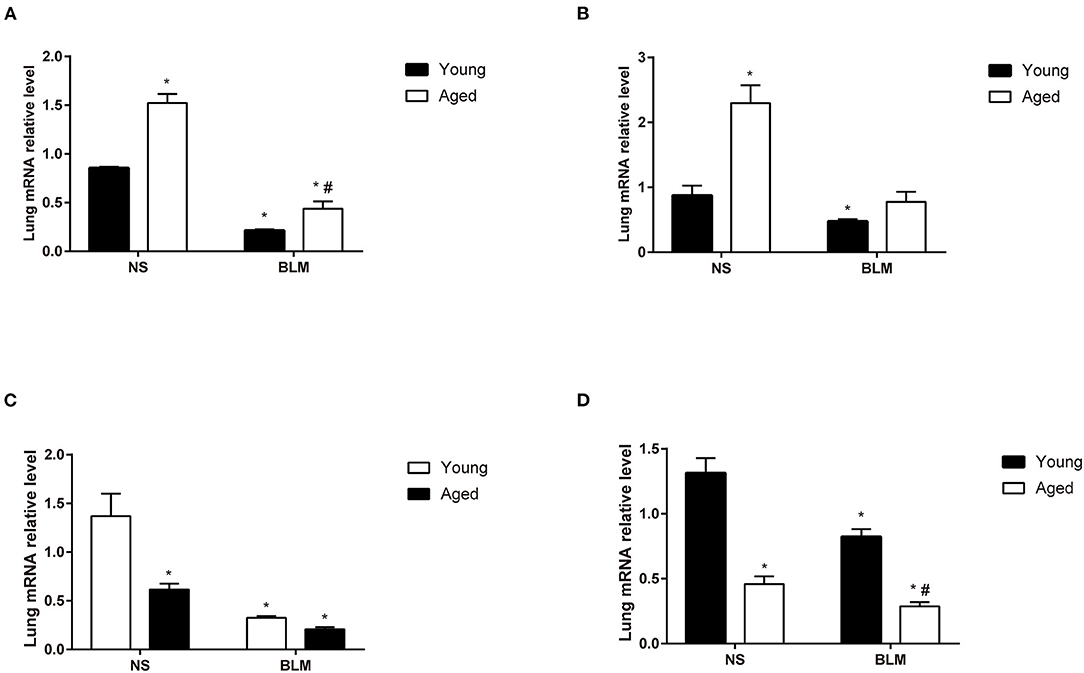
Figure 5. The expression of the four core genes in the lungs of BIPF mice. (A) Slc2a3, (B) Fga, (C) Hp, and (D) Thbs1 in the NS, aged NS, BLM, and aged BLM groups. The four groups were NS (young mice intratracheal with NS), aged NS (aged mice intratracheal with NS), BLM (young mice intratracheal with BLM), and aged BLM (aged mice intratracheal with BLM). Values were expressed as mean ± SEM (n = 4), *p < 0.05, compared with the young NS group, #p < 0.05, compared with the young BLM group. Two-way ANOVA was used. BIPF, bleomycin-induced pulmonary fibrosis; NS, normal saline; BLM, bleomycin.
Discussion
Aging is a strong risk factor and an independent prognostic biomarker for progressive IPF (22). However, a comprehensive analysis based on gene expression profiles for the investigation of the role played by aging in IPF was missing. In this study, using four human IPF datasets, we identified 108 altered aging-related DEGs in IPF. Similarly, through the analysis of a dataset of the BIPF mouse model, we identified 778 aging-related DEGs. Followed by that, both GO and KEGG analyses of the DEGs were conducted separately. The resulting DEGs were subsequently combined and generated eight common DEGs and four core genes. Finally, we confirmed that aging aggravated pulmonary fibrosis and validated the four aging-associated core genes in experimental pulmonary fibrosis.
In the present study, the 108 aging-associated DEGs of human IPF were found to be involved functionally in “response to glucocorticoid” and “response to corticosteroid” BP terms. Given the clinical practice at the time some of these samples were collected, it is likely some of these patients may have been receiving steroids, which could further influence this result. Although glucocorticoid showed no benefit to patients with IPF in several retrospective studies (23, 24), Zhang et al. implied that IPF with different gene types may benefit differently from glucocorticoid therapy (25). Previous research reported that dexamethasone treatments targeting the macrophages in long significantly alleviated pulmonary fibrosis through the regulation of the immune microenvironment (26). Generally, more comprehensive experimental designs might apply for exploring the role of glucocorticoids in IPF. Postsynaptic components are involved in most top five enriched CC terms, indicating an important role of the nerve system in aged patients with IPF. A longitudinal IPF cohort demonstrated that patients with IPF treated with α1 adrenoreceptor blockade displayed improvements in survival rates (27). Moreover, vagotomy attenuated symptoms of pulmonary fibrosis in BIPF mice by enhancing fibrogenic factors and fibrogenic cells (28). Growth factor activity was one significantly enriched MF term. Genes related to growth factor activity were downregulated in aged-related disease (29, 30). Nutrient metabolism imbalance was enriched in KEGG analysis. Loss of pyruvate kinase M2 resulted in cone degeneration in an age-dependent manner (31). Moreover, mitochondrial energy production reduction and cellular redox hemostasis loss played important roles in hearing loss associated with aging (32).
Different from DEGs of IPF, the significant GO enrichment of DEGs in the mouse model of BIPF was extracellular matrix (ECM) and collagen metabolism. Persistent ECM accumulation was considered to participate in the development of several diseases, including IPF, causing difficulties in reversing the disease progression of such disease (33). The ECM of aged lungs was featured by the upregulated transcriptional level of fibronectin extracellular domain A (Fn-EDA) and matrix metalloproteinases (MMPs), including MMP-2 and MMP-9 (5). Collagen contents were found higher in adult mice; however, its transcriptional level was found higher in young mice. This phenomenon was caused by dysregulations in collagen degradation (9). Despite the wide recognition of the association between ECM dysregulations and the process of aging, its underlying mechanisms were still unclear.
Age-associated DEGs of IPF and BIPF exhibited differences, while age-associated DEGs of HC and MC overlapped more. Inflammation and immunomodulation were enriched in age-associated DEGs of HC and MC. Age-related chronic inflammation is a major contributor to diseases with advancing age (34). Extensive evidence indicates that persistent low-grade inflammation is present in the aged population and that age-associated inflammation occurs in the lungs (35, 36). BALF collected from aged mice and aged humans contains increased levels of IL-6, TNF, and complement components, reflective of a disordered innate immunity (37). Although collagen and matrix synthesis are important in aged pulmonary fibrosis, the disordered inflammation and immune response cannot be ignored in aged pulmonary fibrosis (Figure 2B).
The GO terms of the common eight genes were enriched in the hyaluronan (HA) biosynthetic process. Hp, one of the four core genes, produced important effects in the process of lung injuries and repairments (38). Extracellular matrix Hp on type 2 alveolar epithelial cells (AEC2s) was critical for AEC2 renewal, repairments of lung injuries, and limitations of the development of fibrosis (39). Thbs1 is associated with aging and promoted matrix homeostasis through the interaction with collagen, lysyl oxidase precursors, and sites of collagen cross-linking (40, 41). The relationship between Thbs1 and aged pulmonary fibrosis was worth studying. Slc2a3, mediating the uptake of various monosaccharides, was reported to be associated with pulmonary fibrosis. Aging altered the uptakes of glucose and the transcription profile of Slc2a3/Slc2a4 before and after spinal cord injury, which is potentially related to the inflammation level (42). Fga was cleaved by the protease thrombin to produce monomers. Subsequently, the monomers polymerized with fibrinogen beta (Fgb) and fibrinogen gamma (Fgg), forming the insoluble fibrin matrix. The level of Fga was higher in patients with IPF compared with that in healthy controls (43). Moreover, previous research found that the lack of a C-terminal fragment of Fga preceded the progress of fibrosis in patients with liver diseases (44). Most importantly, differences in the expression profiles of the four core genes were found in both patients with IPF and BIPF mice. In order to clarify that the expression changes of the four core genes were due to aging in the context of disease or aging alone, we analyzed the age-associated DEGs in HC and MC. Comprehensively, analysis of our research results, the bioinformatics analysis results, and the expression change of four core genes were due to aging in the context of pulmonary fibrosis.
Therefore, considering the crucial roles of these four core genes on the basis of this study and previous studies above, further studies may be focused on exploring the mechanisms under pulmonary fibrosis in the context of aging. There were still several limitations in the study. Firstly, we could not assess the role of other aging marks in pulmonary fibrosis, such as telomere attrition, epigenetic alterations, and genomic instability. They need more professional research methods, whereas we only explored the role of age in pulmonary fibrosis from the perspective of gene transcription. Secondly, the expression of the four core genes needs to be verified in humans. Thirdly, while our study was able to identify a number of novel pathways in aged IPF, it was limited by the available clinical data (such as the history of glucocorticoid use) and biases inherent in enrichment analyses. Fourthly, some gene information is lost in the process of data sets integration because it cannot be repeated in different datasets. Furthermore, more and larger-scale studies should be conducted.
It was widely recognized that aging was a dependent risk factor in pulmonary fibrosis. As shown by our study, aging-aggravated BLM induced pulmonary fibrosis in BIPF mice. The pathogenesis of young and aged pulmonary fibrosis was not exactly the same from the perspective of gene expression profiles. Four aging-associated core genes (Slc2a3, Fga, Hp, and Thbs1) were related to the development of pulmonary fibrosis.
Data Availability Statement
The datasets presented in this study can be found in online repositories. The names of the repository/repositories and accession number(s) can be found in the article/Supplementary Material.
Ethics Statement
The animal study was reviewed and approved by Animal Care and Use Committee of Tongji Hospital.
Author Contributions
YL and JC: conceptualization, investigation, formal analysis, and writing original draft. SW: conceptualization, formal analysis, and writing original draft. ZT and MW: formal analysis. YF: formal analysis and writing original draft. JZ and JX: conceptualization and supervision. KT: conceptualization, investigation, and formal analysis. All authors contributed to the article and approved the submitted version.
Funding
This study was supported by the National Natural Science Foundation of China (Nos. 81973986, 82170049, 82070032, and 81800041), Health Research Fund of Wuhan (No. WX21Q07), Health and family planning research project of Hubei (No. WJ2019M116), and the National Major Science and Technology Project for the Control and Prevention of Major Infectious Diseases of China (2017ZX10103004).
Conflict of Interest
The authors declare that the research was conducted in the absence of any commercial or financial relationships that could be construed as a potential conflict of interest.
Publisher's Note
All claims expressed in this article are solely those of the authors and do not necessarily represent those of their affiliated organizations, or those of the publisher, the editors and the reviewers. Any product that may be evaluated in this article, or claim that may be made by its manufacturer, is not guaranteed or endorsed by the publisher.
Acknowledgments
We thank all the patients with IPF and healthy controls involved in our study.
Supplementary Material
The Supplementary Material for this article can be found online at: https://www.frontiersin.org/articles/10.3389/fmed.2021.744239/full#supplementary-material
Abbreviations
IPF, idiopathic pulmonary fibrosis; BLM, bleomycin; BIPF, bleomycin (BLM)-induced pulmonary fibrosis; GEO, gene expression omnibus; FC, fold change; DEGs, differently expressed genes; GO, gene ontology; KEGG, Kyoto Encyclopedia of Genes and Genomes; BP, biological process; CC, cell component; MF, molecular function; SPF, specific pathogen-free; NS, normal saline; BALF, bronchoalveolar lavage fluid; HE, hematoxylin-eosin; MT, masson trichrome; RT-PCR, real-time polymerase chain reaction; ANOVA, one-way analysis of variance; HC, healthy control; MC, mouse control; Slc2a3, solute carrier family 2 member 3; Fga, fibrinogen alpha chain; Hp, haptoglobin; Thbs1, thrombospondin 1; ECM, extracellular matrix; Fn-EDA, fibronectin extracellular domain A; MMPs, matrix metalloproteinases; AEC2, type 2 alveolar epithelial cells; Fgb, fibrinogen beta; Fgg, fibrinogen gamma.
References
1. Kirkwood TB. Understanding the odd science of aging. Cell. (2005) 120:437–47. doi: 10.1016/j.cell.2005.01.027
2. Selman M, Pardo A. Revealing the pathogenic and aging-related mechanisms of the enigmatic idiopathic pulmonary fibrosis. an integral model. Am J Respir Critic Care Med. (2014) 189:1161–72. doi: 10.1164/rccm.201312-2221PP
3. Raghu G, Rochwerg B, Zhang Y, Garcia CAC, Azuma A, Behr J, et al. An official ATS/ERS/JRS/ALAT clinical practice guideline: treatment of idiopathic pulmonary fibrosis an update of the 2011 clinical practice guideline. Am J Respir Crit Care Med. (2015) 192:E3–19. doi: 10.1164/rccm.201506-1063ST
4. Mays PK, McAnulty RJ, Laurent GJ. Age-related changes in lung collagen metabolism. A role for degradation in regulating lung collagen production. Am Rev Respirat Dis. (1989) 140:410–6. doi: 10.1164/ajrccm/140.2.410
5. Sueblinvong V, Neujahr DC, Mills ST, Roser-Page S, Ritzenthaler JD, Guidot D, et al. Predisposition for disrepair in the aged lung. Am J Med Sci. (2012) 344:41–51. doi: 10.1097/MAJ.0b013e318234c132
6. López-Otín C, Blasco MA, Partridge L, Serrano M, Kroemer G. The hallmarks of aging. Cell. (2013) 153:1194–217. doi: 10.1016/j.cell.2013.05.039
7. Newton CA, Batra K, Torrealba J, Kozlitina J, Glazer CS, Aravena C, et al. Telomere-related lung fibrosis is diagnostically heterogeneous but uniformly progressive. Eur Respir J. (2016) 48:1710–20. doi: 10.1183/13993003.00308-2016
8. Schafer MJ, White TA, Iijima K, Haak AJ, Ligresti G, Atkinson EJ, et al. Cellular senescence mediates fibrotic pulmonary disease. Nat Commun. (2017) 8:14532. doi: 10.1038/ncomms14532
9. Podolsky MJ, Yang CD, Valenzuela CL, Datta R, Huang SK, Nishimura SL, et al. Age-dependent regulation of cell-mediated collagen turnover. JCI insight. (2020) 5:137519. doi: 10.1172/jci.insight.137519
10. Gulati S, Thannickal VJ. The Aging Lung and Idiopathic Pulmonary Fibrosis. Am J Med Sci. (2019) 357:384–9. doi: 10.1016/j.amjms.2019.02.008
11. Cho JH, Gelinas R, Wang K, Etheridge A, Piper MG, Batte K, et al. Systems biology of interstitial lung diseases: integration of mRNA and microRNA expression changes. BMC Med Genomics. (2011) 4:8. doi: 10.1186/1755-8794-4-8
12. Meltzer EB, Barry WT, D'Amico TA, Davis RD, Lin SS, Onaitis MW, et al. Bayesian probit regression model for the diagnosis of pulmonary fibrosis: proof-of-principle. BMC Med Genomics. (2011) 4:70. doi: 10.1186/1755-8794-4-70
13. Tan J, Tedrow JR, Dutta JA, Juan-Guardela B, Nouraie M, Chu Y, et al. Expression of RXFP1 is decreased in idiopathic pulmonary fibrosis. Implications for relaxin-based therapies. Am J Respirat Critic Care Med. (2016) 194:1392–402. doi: 10.1164/rccm.201509-1865OC
14. Horimasu Y, Ishikawa N, Taniwaki M, Yamaguchi K, Hamai K, Iwamoto H, et al. Gene expression profiling of idiopathic interstitial pneumonias (IIPs): identification of potential diagnostic markers and therapeutic targets. BMC Med Genet. (2017) 18:88. doi: 10.1186/s12881-017-0449-9
15. American Thoracic Society. Idiopathic pulmonary fibrosis: diagnosis and treatment. International consensus statement. American Thoracic Society (ATS), and the European Respiratory Society (ERS). Am J Respirat Critic Care Med. (2000) 161:646–64. doi: 10.1164/ajrccm.161.2.ats3-00
16. American Thoracic Society/European Respiratory Society International Multidisciplinary Consensus Classification of the Idiopathic Interstitial Pneumonias. This joint statement of the American Thoracic Society (ATS), and the European Respiratory Society (ERS) was adopted by the ATS board of directors, June 2001 and by the ERS Executive Committee, June 2001. Am J Respir Critic Care Med. (2002);165:277–304. doi: 10.1164/ajrccm.165.2.ats01
17. Calyeca J, Balderas-Martínez YI, Olmos R, Jasso R, Maldonado V, Rivera Q, et al. Accelerated aging induced by deficiency of Zmpste24 protects old mice to develop bleomycin-induced pulmonary fibrosis. Aging. (2018) 10:3881–96. doi: 10.18632/aging.101679
18. Shi L, Reid LH, Jones WD, Shippy R, Warrington JA, Baker SC, et al. The MicroArray Quality Control (MAQC) project shows inter- and intraplatform reproducibility of gene expression measurements. Nat Biotechnol. (2006) 24:1151–61. doi: 10.1038/nbt1239
19. Warnat P, Eils R, Brors B. Cross-platform analysis of cancer microarray data improves gene expression based classification of phenotypes. BMC Bioinform. (2005) 6:265. doi: 10.1186/1471-2105-6-265
20. Shabalin AA, Tjelmeland H, Fan C, Perou CM, Nobel AB. Merging two gene-expression studies via cross-platform normalization. Bioinformatics. (2008) 24:1154–60. doi: 10.1093/bioinformatics/btn083
21. Gao P, Tang K, Lu Y, Huang Z, Wang S, Wang M, et al. Pentraxin 3 promotes airway inflammation in experimental asthma. Respir Res. (2020) 21:237. doi: 10.1186/s12931-020-01499-6
22. Qu J, Yang SZ, Zhu Y, Guo T, Thannickal VJ, Zhou Y. Targeting mechanosensitive MDM4 promotes lung fibrosis resolution in aged mice. J Exp Med. (2021) 218:20202033. doi: 10.1084/jem.20202033
23. Peikert T, Daniels CE, Beebe TJ, Meyer KC Ryu JH. Assessment of current practice in the diagnosis and therapy of idiopathic pulmonary fibrosis. Respir Med. (2008) 102:1342–8. doi: 10.1016/j.rmed.2008.03.018
24. Atkins CP, Loke YK, Wilson AM. Outcomes in idiopathic pulmonary fibrosis: a meta-analysis from placebo controlled trials. Respir Med. (2014) 108:376–87. doi: 10.1016/j.rmed.2013.11.007
25. Zhang HT, Fang SC, Wang CY, Wang W, Wu J, Wang C, et al. MMP-9 1562C>T gene polymorphism and efficacy of glucocorticoid therapy in idiopathic pulmonary fibrosis patients. Genet Test Mol Biomarkers. (2015) 19:591–7. doi: 10.1089/gtmb.2015.0057
26. Sang X, Wang Y, Xue Z, Qi D, Fan G, Tian F, et al. Macrophage-targeted lung delivery of dexamethasone improves pulmonary fibrosis therapy via regulating the immune microenvironment. Front Immunol. (2021) 12:613907. doi: 10.3389/fimmu.2021.613907
27. Gao R, Peng X, Perry C, Sun H, Ntokou A, Ryu C, et al. Macrophage-derived netrin-1 drives adrenergic nerve-associated lung fibrosis. J Clin Investig. (2021) 131:e136542. doi: 10.1172/JCI136542
28. Song N, Liu J, Shaheen S, Du L, Proctor M, Roman J, et al. Vagotomy attenuates bleomycin-induced pulmonary fibrosis in mice. Sci Rep. (2015) 5:13419. doi: 10.1038/srep13419
29. Duran-Ortiz S, List EO, Basu R, Kopchick JJ. Extending lifespan by modulating the growth hormone/insulin-like growth factor-1 axis: coming of age. Pituitary. (2021) 24:438–56. doi: 10.1007/s11102-020-01117-0
30. Potilinski MC, Tate PS, Lorenc VE, Gallo JE. New insights into oxidative stress and immune mechanisms involved in age-related macular degeneration tackled by novel therapies. Neuropharmacology. (2021) 188:108513. doi: 10.1016/j.neuropharm.2021.108513
31. Rajala A, Wang Y, Soni K, Rajala RVS. Pyruvate kinase M2 isoform deletion in cone photoreceptors results in age-related cone degeneration. Cell Death Dis. (2018) 9:737. doi: 10.1038/s41419-018-0712-9
32. Tian C, Kim YJ, Hali S, Choo OS, Lee JS, Jung SK, et al. Suppressed expression of LDHB promotes age-related hearing loss via aerobic glycolysis. Cell Death Dis. (2020) 11:375. doi: 10.1038/s41419-020-2577-y
33. Spagnolo P, Maher TM, Richeldi L. Idiopathic pulmonary fibrosis: Recent advances on pharmacological therapy. Pharmacol Ther. (2015) 152:18–27. doi: 10.1016/j.pharmthera.2015.04.005
34. Varghese M, Song J, Singer K. Age and Sex: Impact on adipose tissue metabolism and inflammation. Mech Ageing Dev. (2021) 199:111563. doi: 10.1016/j.mad.2021.111563
35. Hinojosa E, Boyd AR, Orihuela CJ. Age-associated inflammation and toll-like receptor dysfunction prime the lungs for pneumococcal pneumonia. J Infect Dis. (2009) 200:546–54. doi: 10.1086/600870
36. Schneider JL, Rowe JH, Garcia-de-Alba C, Kim CF, Sharpe AH, Haigis MC. The aging lung: physiology, disease, and immunity. Cell. (2021) 184:476–88. doi: 10.1016/j.cell.2021.03.005
37. Moliva JI, Rajaram MV, Sidiki S, Sasindran SJ, Guirado E, Pan XJ, et al. Molecular composition of the alveolar lining fluid in the aging lung. Age (Dordrecht, Netherlands). (2014) 36:9633. doi: 10.1007/s11357-014-9633-4
38. Jiang D, Liang J, Fan J, Yu S, Chen S, Luo Y, et al. Regulation of lung injury and repair by Toll-like receptors and hyaluronan. Nat Med. (2005) 11:1173–9. doi: 10.1038/nm1315
39. Liang J, Zhang Y, Xie T, Liu N, Chen H, Geng Y, et al. Hyaluronan and TLR4 promote surfactant-protein-C-positive alveolar progenitor cell renewal and prevent severe pulmonary fibrosis in mice. Nat Med. (2016) 22:1285–93. doi: 10.1038/nm.4192
40. Rosini S, Pugh N, Bonna AM, Hulmes DJS, Farndale RW, Adams JC. Thrombospondin-1 promotes matrix homeostasis by interacting with collagen and lysyl oxidase precursors and collagen cross-linking sites. Sci Signal. (2018) 11:2566. doi: 10.1126/scisignal.aar2566
41. Ahuja N, Li Q, Mohan AL, Baylin SB, Issa JP. Aging and DNA methylation in colorectal mucosa and cancer. Cancer Res. (1998) 58:5489–94.
42. von Leden RE, Moritz KE, Bermudez S, Jaiswal S, Wilson CM, Dardzinski BJ, et al. Aging alters glucose uptake in the naïve and injured rodent spinal cord. Neurosci Lett. (2019) 690:23–8. doi: 10.1016/j.neulet.2018.10.004
43. Zhang Y, Xin Q, Wu Z, Wang C, Wang Y, Wu Q, et al. Application of isobaric tags for relative and absolute quantification (iTRAQ) coupled with two-dimensional liquid chromatography/tandem mass spectrometry in quantitative proteomic analysis for discovery of serum biomarkers for idiopathic pulmonary fibrosis. Med Sci Monit. (2018) 24:4146–53. doi: 10.12659/MSM.908702
Keywords: aging, pulmonary fibrosis, genetic signature, idiopathic pulmonary fibrosis, glucocorticoids
Citation: Lu Y, Chen J, Wang S, Tian Z, Fan Y, Wang M, Zhao J, Tang K and Xie J (2021) Identification of Genetic Signature Associated With Aging in Pulmonary Fibrosis. Front. Med. 8:744239. doi: 10.3389/fmed.2021.744239
Received: 22 July 2021; Accepted: 20 September 2021;
Published: 20 October 2021.
Edited by:
Marta Bueno, University of Pittsburgh, United StatesReviewed by:
Eleanor Valenzi, University of Pittsburgh, United StatesYuli Huang, Southern Medical University, China
Mingyi Wang, National Institutes of Health (NIH), United States
Copyright © 2021 Lu, Chen, Wang, Tian, Fan, Wang, Zhao, Tang and Xie. This is an open-access article distributed under the terms of the Creative Commons Attribution License (CC BY). The use, distribution or reproduction in other forums is permitted, provided the original author(s) and the copyright owner(s) are credited and that the original publication in this journal is cited, in accordance with accepted academic practice. No use, distribution or reproduction is permitted which does not comply with these terms.
*Correspondence: Jianping Zhao, Zhaojp88@126.com; Kun Tang, thomasvsummers@163.com; Jungang Xie, xiejjgg@hotmail.com
†These authors share first authorship