- 1Department of Gastroenterology, The Second Affiliated Hospital and Yuying Children's Hospital of Wenzhou Medical University, Wenzhou, China
- 2Department of Pathophysiology, School of Basic Medicine Science, Wenzhou Medical University, Wenzhou, China
Background and Aims: Acute liver injury (ALI) is a clinical syndrome characterized by rapid loss of liver function, which may progress to life-threatening liver failure. We conducted this meta-analysis to examine the evidence on the effects of probiotics or prebiotics on ALI.
Methods and Results: Several databases, including PubMed, EMBASE, and Cochrane Library, were scrutinized from the inception through February 2021 by combining key search terms, yielding 26 eligible studies, which concluded that modulation of gut microbiota significantly decreased aspartate transaminase [standardized mean difference (SMD): −1.51, 95% confidence interval (CI): −2.03 to −1.00], alanine aminotransferase (SMD: −1.42, 95% CI: −1.85 to −0.98), and bilirubin (SMD: −0.91, 95% CI: −1.33 to −0.49). In addition, administration of probiotics or prebiotics also promoted proliferation of Bifidobacterium (SMD: 1.21, 95% CI: −0.18 to 2.60) and inhibited Enterococcus (SMD: −1.00, 95% CI: −1.39 to −0.61), contributing to lower levels of endotoxin (SMD: −2.14, 95% CI: −2.91 to −1.37). Tight junction protein ZO-1 (SMD: 1.95, 95% CI: 0.14 to 3.76) was upregulated after intervention, thereby reducing bacterial translocation to the liver [odds ratio (OR) = 0.23, 95% CI: 0.13–0.44] and mesenteric lymph node (OR = 0.14, 95% CI: 0.08 to 0.26), with decreased tumor necrosis factor-α (SMD: −2.84, 95% CI: −3.76 to −1.93) and interleukin-6 (SMD: −2.62, 95% CI: −4.14 to −1.10). Oxidative stress was also relieved by reducing malondialdehyde (SMD: −1.83, 95% CI: −2.55 to −1.10) while elevating superoxide dismutase (SMD: 1.78, 95% CI: 1.00–2.55) and glutathione (SMD: 1.83, 95% CI: 0.76–2.91).
Conclusion: Our findings suggest that probiotics and prebiotics could be a promising therapeutic strategy in ALI and possess a potential for clinical applications.
Systematic Review Registration: https://www.crd.york.ac.uk/PROSPERO/display_record.php?RecordID=255888, CRD42021255888.
Introduction
Acute liver injury (ALI) is defined as a rapid degeneration of liver biochemistry within 6 months in patients with no prior liver disease (1). With the further loss of liver function and impaired metabolism of toxic substances, patients with ALI may develop jaundice, coagulation dysfunction, and hepatic encephalopathy, marking the progression to acute liver failure characterized by multisystem complications and high mortality of up to 80% (2). Liver transplantation is considered to be the only definitive treatment in the stage of acute liver failure (3), but it is limited by a shortage of graft availability, which creates the urgent need to seek complementary and promising therapies to prevent the progression of ALI.
For decades, emerging evidence has indicated the tight connections between the gut microbiota and liver injury, continuously enriching the theory of the gut–liver axis. In the context of disease, disturbances of the intestinal barrier increase the portal influx of bacteria or their metabolites to the liver, causing dysfunction in the metabolism of bile acids, and promote systemic inflammation and liver damage, which in turn intensifies gut dysbiosis (4). In addition, pathogen-associated molecular patterns, such as lipopolysaccharides (LPS), from certain intestinal bacteria stimulate nuclear factor kappa B (NF-κB) via toll-like receptors (TLRs) and nod-like receptors, resulting in the production of inflammatory mediators and chemokines, with the activation of stellate cells involved in fibrosis progression (5).
Microbial agents are typically referred to as probiotics and prebiotics, which are defined as living microorganisms that contribute health-promoting benefits to the host and nondigestible food ingredients that are selectively utilized by host microorganisms to stimulate health benefits, respectively. Studies have shown that both strategies of regulating intestinal microbiomes could exert a curative effect through the gut–liver axis. Since the regulation of intestinal microecology has long been known to possess definite therapeutic efficacy in the treatment of chronic liver diseases, including arresting the progression of hepatic fibrosis and preventing the occurrence of hepatic encephalopathy (4), the relationship between intestinal flora and ALI has received increasing attention. It has been previously observed that correction of the gut microbiota composition significantly attenuated t-BHP-induced liver injury by intensifying gut barrier with increased expression of tight junction proteins, including claudin-1, occludin, and zonula occludens (ZO-1), thus suppressing bacterial translocation (BT) and the expression of LPS-stimulated proinflammatory mediators. In addition, beneficial bacteria were verified to regulate tumor necrosis factor-α (TNF-α) expression by inhibiting the TLR4-associated NF-κB signaling pathway and correcting the Th17/Treg imbalance via the mediation of innate immune cells.
However, the effectiveness of probiotics and prebiotics on ALI has not been systematically evaluated. Therefore, the purpose of this study was to comprehensively analyze and quantify credible evidence from published studies, seeking to offer valuable information for future research on the treatment of ALI.
Methods
Protocol Registration
This meta-analysis was registered on PROSPERO (ID: CRD42021255888; https://www.crd.york.ac.uk/PROSPERO/display_record.php?RecordID=255888).
Search Strategy
We performed this meta-analysis in accordance with the recommended Preferred Reporting Items for Systematic Reviews and Meta-analyses guidelines (6). A comprehensive search of English literature published up to January 2021 was conducted in PubMed, EMBASE, the Cochrane Central Register of Controlled Trials, and clinicaltrials.gov databases using combinations of the Mesh terms and synonyms, including “probiotics,” “yogurt,” “yogurt,” “Lactobacillus,” “Bifidobacterium,” “Enterococcus,” “Streptococcus,” “Saccharomyces,” “Lactococcus,” “Bacillus,” “prebiotics,” “inulin,” “oligosaccharide,” “galactose oligosaccharide,” and “fructose oligosaccharide” for microbial agents, and “liver injury,” “toxic hepatitis,” “drug-induced liver injury,” and “chemical-induced liver injury” for ALI. Additional studies were also checked by a hand search of all the references of the retrieved articles. After eliminating duplicates, we screened the titles, abstracts, and full texts sequentially for eligible records according to the selection criteria. Any disagreement was solved through discussion.
Study Selection
The participants, intervention, comparison, outcome, and study design (PICOS) principle was followed during the literature screening. Studies that met the following inclusion criteria were considered for selection: Participants (P): patients or animal models of ALI regardless of the cause. It should be noted that no clinical studies were available at the end of the selection process, and all the literature involved was on animal research; therefore, the objectives were finally limited to animal models; Intervention (I): the intervention group received microbial agents, such as prebiotics or probiotics regardless of dosage, route, and duration; Comparison (C): control group without receiving microbial agents; Outcome (O): the studies should at least measure one of the following indicators: alanine transaminase (ALT), aspartate aminotransferase (AST), bilirubin, TNF-α, interleukin-6 (IL-6), IL-10, cholesterol, triglyceride, BT, endotoxin, malondialdehyde (MDA), superoxide dismutase (SOD), glutathione (GSH), or ZO-1; Study design (S): randomized controlled studies. Studies with the occurrence of acute liver failure during the trial or unavailable data were excluded.
Quality Evaluation and Data Extraction
Since all clinical studies were removed during the screening process, with only preclinical studies included according to the selection criteria, the methodological quality was evaluated based on the rules detailed in systematic review centre for laboratory animal experimentation's (SYRCLE) risk of bias tool (7). The following information was extracted from the literature involved: first author, publication year, animal species, sample size, modeling methods, intervention and dosage, route and duration, and comparison and outcome indicators. Disagreements were addressed through consensus. Getdata 2.20 was used in the data extraction.
Statistical Analyses
Pooled mean differences and standard deviations with 95% confidence intervals (CIs) were applied to determine differences in outcomes of continuous variables, with results of the dichotomous variables reported as odds ratio (OR) and 95% CI. Heterogeneity was assessed by using the Cochran Q-test and I2 statistics (I2 < 25%, low heterogeneity; 25–50%, moderate heterogeneity; I2 > 50%, high heterogeneity). A random-effects model will be performed due to the exploratory nature of the animal studies. A value of p < 0.05 was considered statistically significant. Subgroup analysis was also performed for indicators with more than 10 included studies according to the type of microbial agents, bacterial strain, animal model, and modeling method to explore the source of heterogeneity. We also used the meta-regression to detect where the potential factor for heterogeneity originated. Sensitivity analysis was conducted to identify the studies that significantly affected the findings by excluding studies from the analysis one by one to gauge the robustness of our results. Publication bias was estimated quantitatively using Egger's test. A contour-enhanced funnel plot with the “trim and fill” method was obtained as an aid to differentiate asymmetry caused by publication bias or other factors such as heterogeneity (34). If the missing studies were in the nonsignificant area, the asymmetry was due to publication bias. Otherwise, the observed asymmetry could be attributed to factors other than publication bias. Statistical analyses were performed using R (version 4.0.0) and the R Studio software (R Studio, Boston, MA, USA).
Results
Identification of Relevant Study
As shown in the PRISMA flow diagram, a total of 3,802 studies were initially retrieved by searching the databases, which reduced to 3,649 after the deletion of duplicates. Preliminary screening of the titles and abstracts reduced the number to 67 articles after the exclusion of 3,582 studies. A further 41 articles were rejected based on a detailed full-text evaluation, with 26 studies ultimately being eligible for the data extraction and analysis. All the literature involved was on animal studies, as there were no clinical studies that met the selection criteria (Figure 1) (8–33).
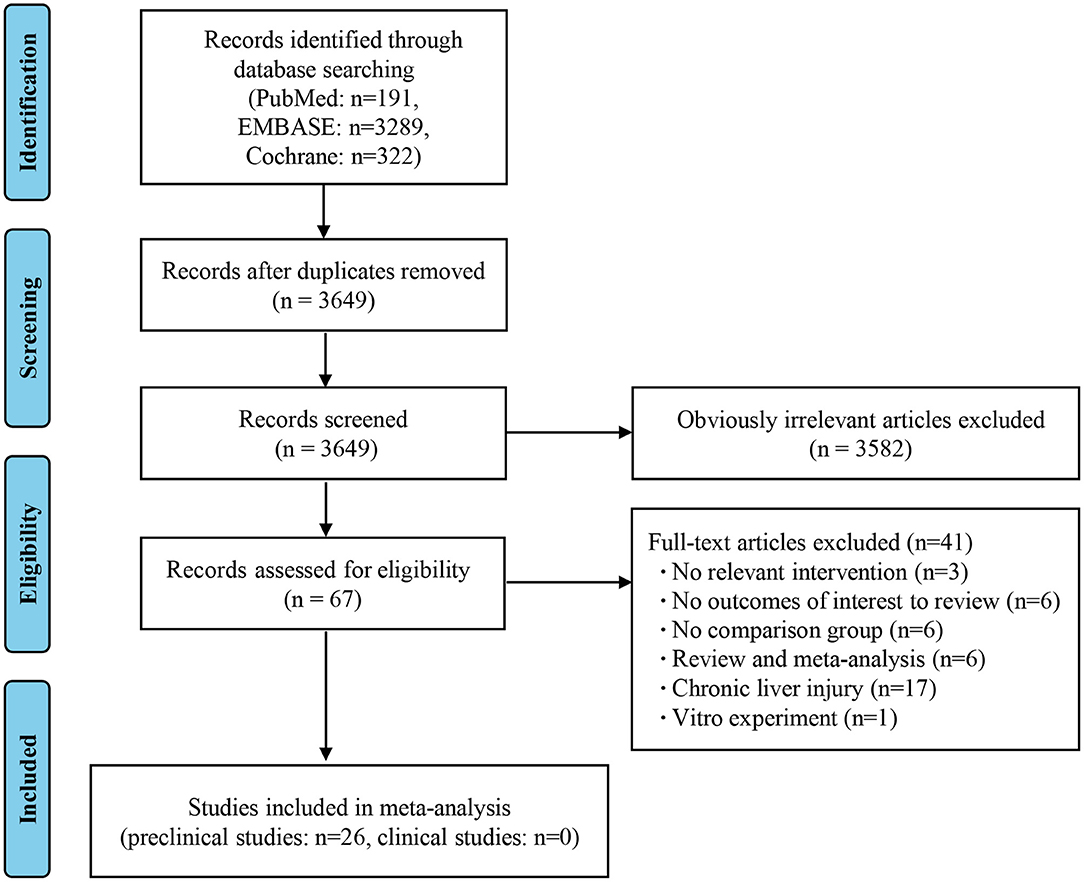
Figure 1. Flow diagram based on the Preferred Reporting Items for Systematic Reviews and Meta-Analyses (PRISMA).
Study Characteristics and Quality Assessment
Table 1 shows the characteristics of the 26 selected studies. The rodent animal model was applied as the research objects in all the literature involved, most of which used Sprague–Dawley rats. In addition, the modeling methods of ALI were different, among which the intraperitoneal injection of D-galactosamine had the highest frequency of utilization, followed by CCI4 and LPS. Probiotics, such as Lactobacillus and Bifidobacterium, were more commonly used in the intervention group compared to prebiotics (including lactulose and inulin), which were employed by only six studies. Oral gavage was the most commonly used route of drug delivery, while transrectal administration through a rectal tube was used by only two studies. Saline was used as the control in most of the studies, while a standard diet was used by Nardone, G.
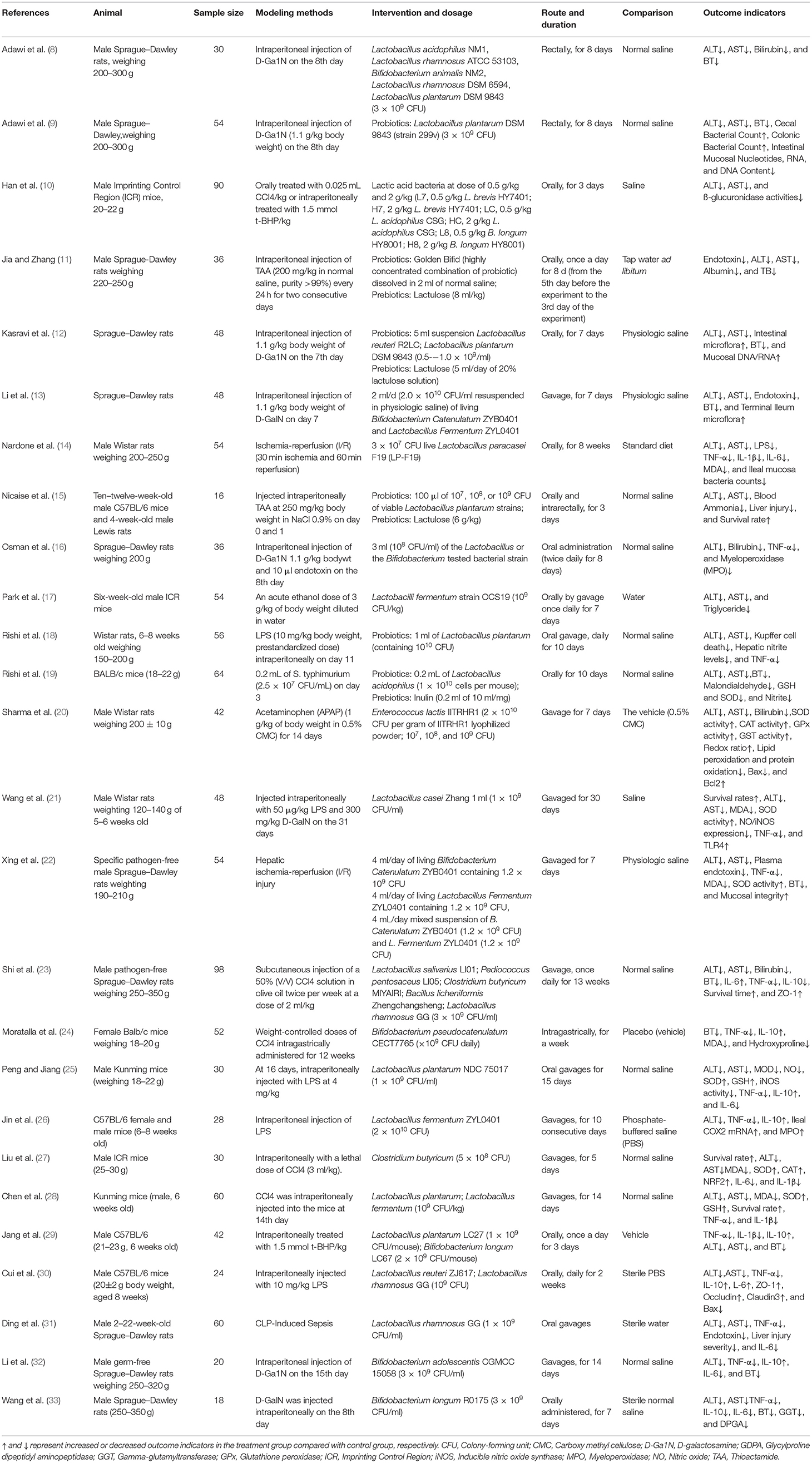
Table 1. Characteristics of included studies investigating the effects of probiotics or prebiotics on ALI.
Two reviewers independently evaluated the methodological quality by employing SYRCLE's risk of bias tool. Each item was judged as “low risk,” “unclear,” or “high risk.” Blinding was the major contributor to bias; the blinding and allocation bias were unclear in most studies, as specific details of relevant information were often not provided. All studies were scored as having a low risk of bias in reporting bias. Overall, the assessment result was similar across the studies used in this meta-analysis with high quality and low risk of bias (Supplementary Table 1). Discrepancies during the process of quality assessment were solved through discussion.
Effect of Microbial Agents on Liver Biochemical Indicators
A total of 22 and 27 preclinical studies evaluated the effect of microbial agents on the liver function indicators, AST and ALT, respectively. Our pooled analysis showed a significant difference between the microbial intervention group and those treated with placebo in AST (standardized mean difference (SMD): −1.51, 95% CI: −2.03 to −1.00, I2 = 82%, and p < 0.01) and ALT (SMD: −1.42, 95% CI: −1.85 to −0.98, I2 = 79%, and p < 0.01; Figures 2A,B), indicating the positive effects of microbial regulation on normalizing AST and ALT in an ALI model. Owing to the significant heterogeneity, subgroup analyses were carried out to find out potential sources. Heterogeneity was slightly altered after dividing into probiotics (AST—SMD: −1.53, 95% CI: −2.09 to −0.97, I2 = 83%, and p < 0.01; ALT—SMD: −1.54, 95% CI: −2.02 to −1.07, I2 = 80%, and p < 0.01) and prebiotics (AST—SMD: −1.50, 95% CI: −3.15 to 0.15, I2 = 84%, and p < 0.01; ALT—SMD: −0.72, 95% CI: −1.71 to 0.26, I2 = 71%, and p = 0.02), but reduced in the subgroup animal model of AST (Table 2), indicating that it might be the source of heterogeneity, which was attested using meta-regression (Table 3). Subgroup analysis and meta-regression of ALT failed to find the source of heterogeneity (Supplementary Tables 2A, 3A). Publication bias existed in Egger's test (both p < 0.01). Although there is an obvious asymmetry in the contour-enhanced funnel plot (Figures 3A,B), it was proven that the asymmetry was caused by factors other than publication bias after using the trim-and-fill method. Sensitivity analysis confirmed the robustness of the study (Supplementary Figures 1A,B). Results from the pooled random-effects model of eight selected studies with the relevant data showed a decrease in bilirubin level after being treated with microbial agents (SMD: −0.91, 95% CI: −1.33 to −0.49) with moderate heterogeneity (I2 = 51%, p = 0.03; Figure 2C), which further demonstrated the protective effect of microbial agents on the liver.
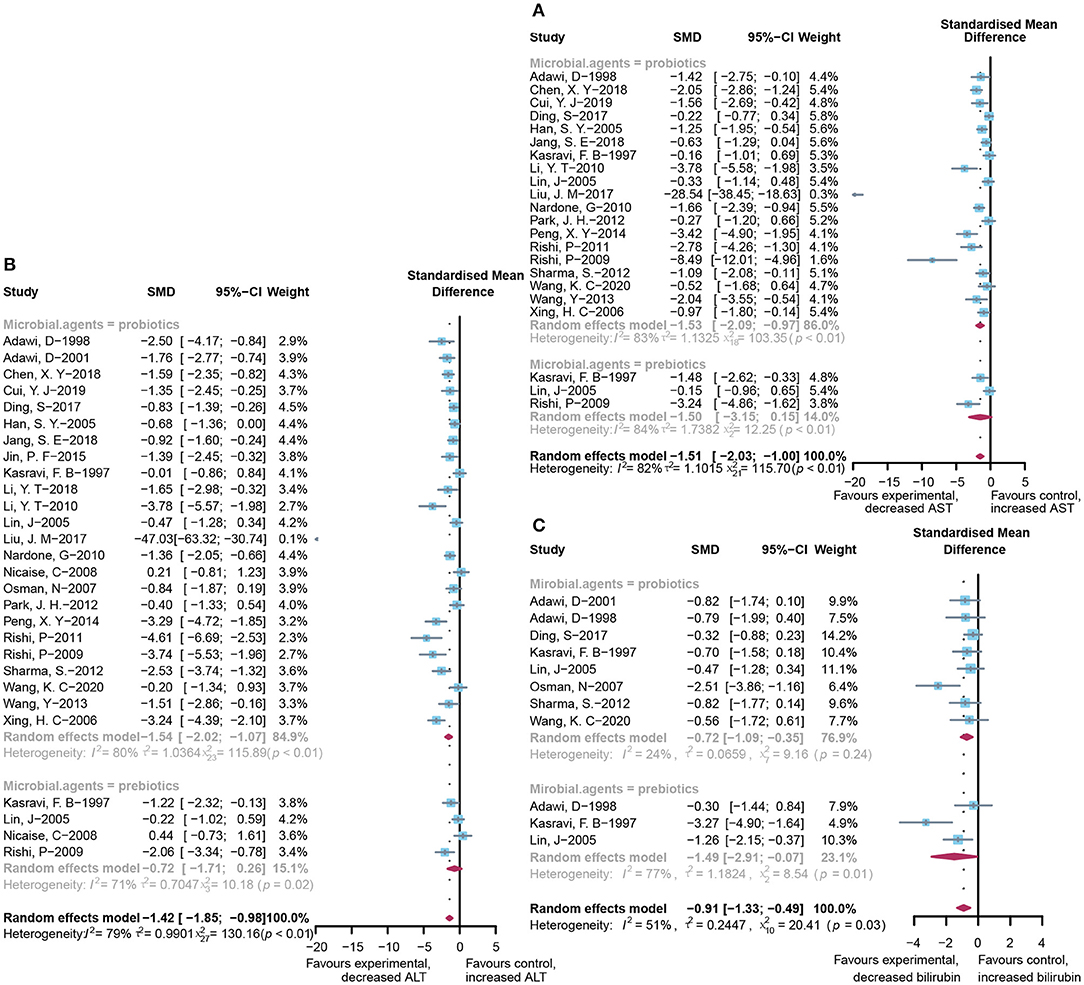
Figure 2. Effectiveness of microbial agents on liver biochemical index. (A) The effect of microbial agents on AST, (B) ALT, and (C) bilirubin.
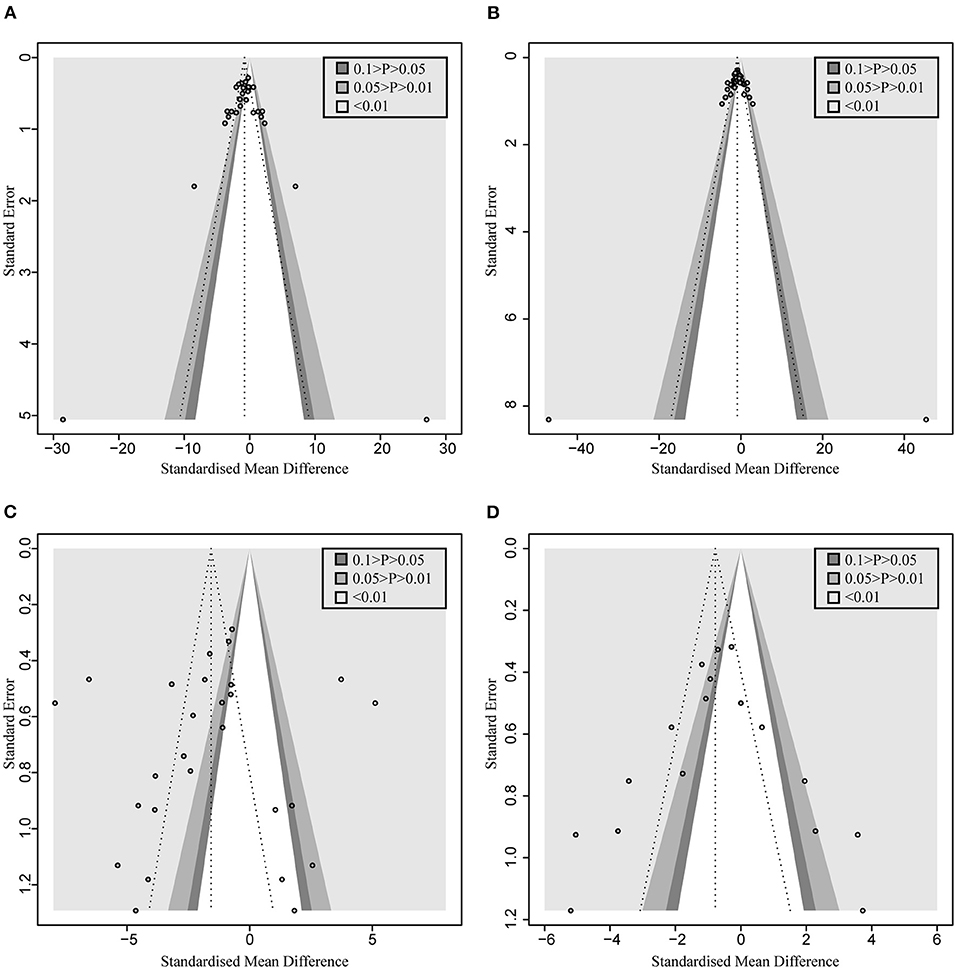
Figure 3. Contour-enhanced funnel plot with trim-and-fill method. (A) AST. (B) ALT. (C) TNF-α (D) MDA. If the missing studies were in the nonsignificant area, the asymmetry was due to publication bias. Otherwise, the observed asymmetry could be attributed to factors other than publication bias.
Effect of Microbial Agents on Bacteria-Originated Endotoxin
Integrated investigation of the terminal ileum flora, BT, and endotoxin was conducted to give a detailed evaluation of the changes in gut microbiota and toxin metabolism in ALI. The incidence of BT to the liver (OR = 0.23, 95% CI: 0.13–0.44) and mesenteric lymph node (OR = 0.14, 95% CI: 0.08–0.26; Figure 4A) was significantly reduced in the microbial intervention group without heterogeneity (I2 = 0%, p = 0.85 and I2 = 0%, p = 0.97, respectively). Notably, although the horizontal lines of most of the selected studies intersect with the invalid line, which may be caused by the small sample size, the combined results showed significance. After the use of prebiotics or probiotics, the abundance of detrimental Enterococcus in the ileocecal region was less than that of the control group (SMD: −1.00, 95% CI: −1.39 to −0.61) with good homogeneity (I2 = 0%, p = 0.76), while most studies believed that the colonization of Bifidobacterium, a beneficial bacterium, increased in this area (SMD: 1.21, 95% CI: −0.18 to 2.60, I2 = 87%, and p < 0.01; Figure 4B). Accordingly, endotoxin exhibited reduced levels in the microbial treatment group (SMD: −2.14, 95% CI: −2.91 to −1.37, I2 = 86%, and p < 0.01; Figure 4C), with increased expression of intestinal tight junction protein ZO-1 (SMD: 1.95, 95% CI: 0.14–3.76, I2 = 86%, and p < 0.01) (Figure 4D), indicating an augmented gut barrier and stable intestinal permeability.
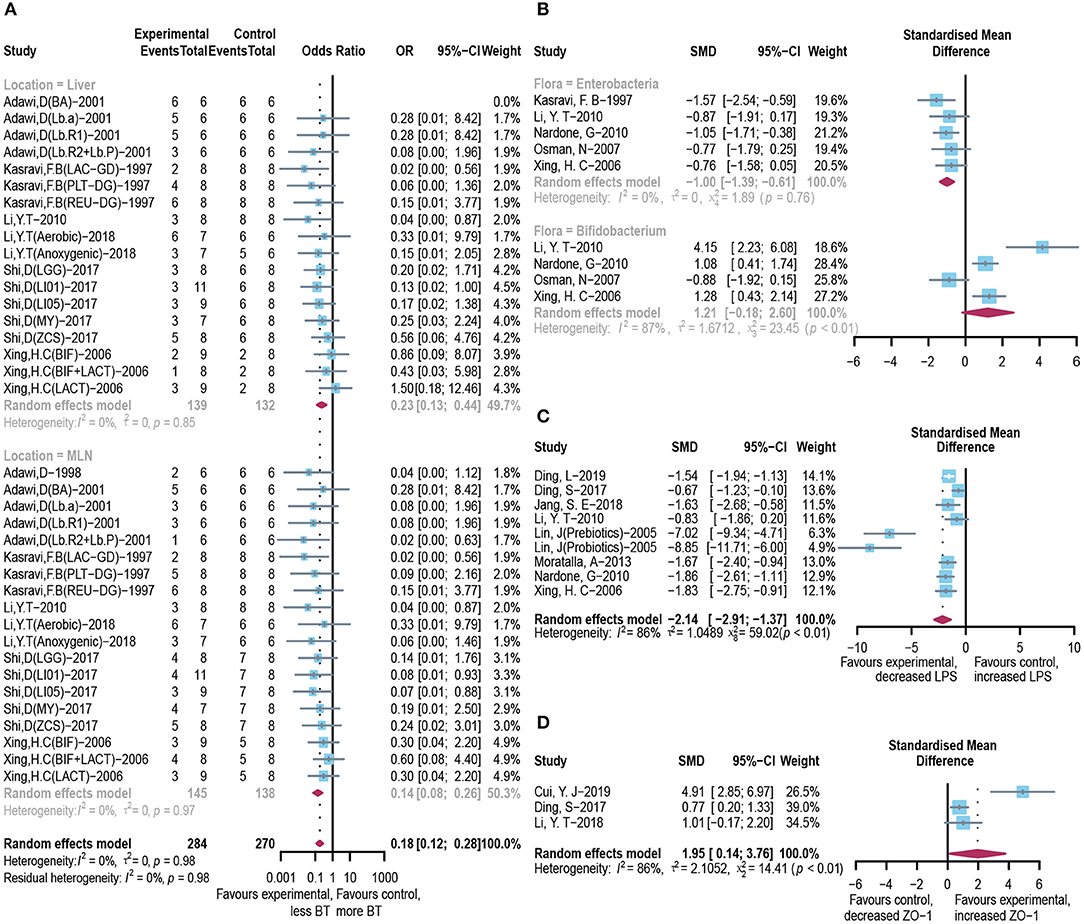
Figure 4. Effectiveness of microbial agents on BT. (A) The effect of microbial agents on BT to liver or mesenteric lymph node. (B) The effect of microbial agents on the abundance of Enterococcus and Bifidobacterium in the ileocecal region. (C) The effect of microbial agents on the level of LPS. (D) The effect of microbial agents on the expression of tight junction protein ZO-1.
Effect of Microbial Agents on Inflammation Mediators
Pooled analysis of inflammation mediators, such as TNF-α, IL-6, and IL-10, has also been applied to assess the inflammatory infiltration of the liver after the occurrence of acute injury. As shown in Figure 5, the administration of probiotics or prebiotics, compared to placebo, decreased TNF-α (SMD: −2.84, 95% CI: −3.76 to −1.93) (Figure 5A) and IL-6 (SMD: −2.62, 95% CI: −4.14 to −1.10) (Figure 5B) with significant heterogeneity (I2 = 93%, p < 0.01 and I2 = 95%, p < 0.01, respectively). However, except for significant heterogeneity (I2 = 89%, p < 0.01), an interesting trend was noted for the pooled result of IL-10, which showed higher levels in the microbial intervention group than in the placebo-treated group (SMD: 0.56, 95% CI: −0.66 to 1.79; Figure 5C), with the statistical combination intersected in the invalid line. To explore the sources of heterogeneity and the impact of different measurement levels on the results, inflammatory cytokines were separated into three subgroups, designated as “liver,” “serum,” and “gene expression.” Remarkably, compared to the control group, IL-10 levels increased in the liver (SMD: 1.98, 95% CI: 0.74–3.21, I2 = 71%, and p = 0.03) and decreased in the serum (SMD: −1.21, 95% CI: −2.80 to 0.39, I2 = 71%, and p = 0.06), showing the opposite trend. Subgroup analysis and meta-regression of TNF-α suggested that the animal model and modeling methods were the sources of heterogeneity (Supplementary Tables 2B, 3B). Egger's test suggested the existence of publication bias (p < 0.01). The contour-enhanced funnel plot with the trim-and-fill method (Figure 3C) indicates that publication bias was not the main cause of asymmetry. Sensitivity analysis confirmed the robustness of the study (Supplementary Figure 1C).
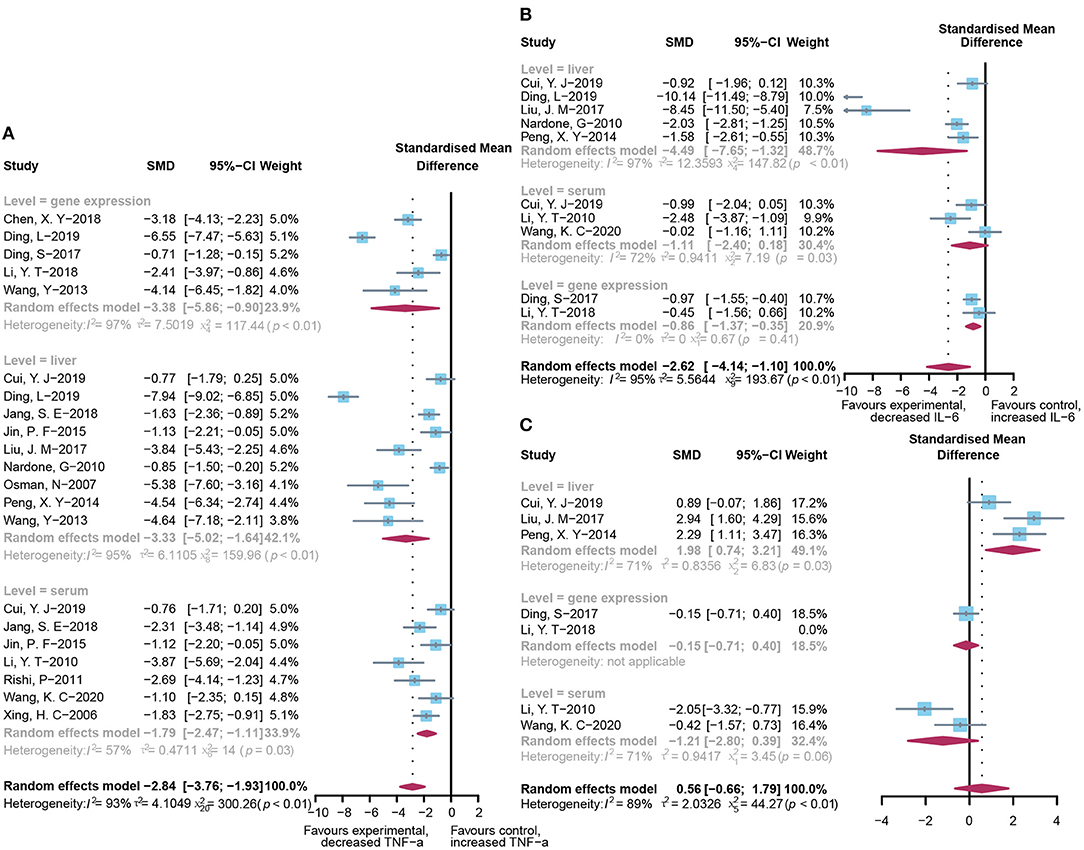
Figure 5. Effectiveness of microbial agents on inflammatory cytokines. (A) The effect of microbial agents on TNF-α. (B) The effect of microbial agents on IL-6. (C) The effect of microbial agents on IL-10.
Effect of Microbial Agents on Oxidative Stress
To assess the free radical-mediated lipid peroxidation injury and antioxidant status of tissues, we combined the levels of MDA, SOD, and GSH respectively. The treatment of microbial agents contributed to the decrease in MDA level (SMD: −1.83, 95% CI: −2.55 to −1.10, I2 = 83%, and p < 0.01), along with the enhancement of antioxidant capacity, which was reflected in the increased level of SOD (SMD: 1.78, 95% CI: 1.00–2.55, I2 = 74%, and p < 0.01) and GSH (SMD: 1.83, 95% CI: 0.76–2.91, I2 = 72%, and p = 0.01), with obvious heterogeneity (Figures 6A–C). Subgroup analysis suggested that heterogeneity was alleviated to a certain extent after grouping according to animal model and modeling methods, suggesting that these two might be the source of heterogeneity (Supplementary Table 2C), which was further verified using meta-regression to show that the p-values of both were <0.05 (p = 0.02 and 0.03, respectively; Supplementary Table 3C). The contour-enhanced funnel plot with the trim-and-fill method (Figure 3D) indicates that publication bias was not the main cause of asymmetry. Sensitivity analysis proved the robustness of the results (Supplementary Figure 1D).
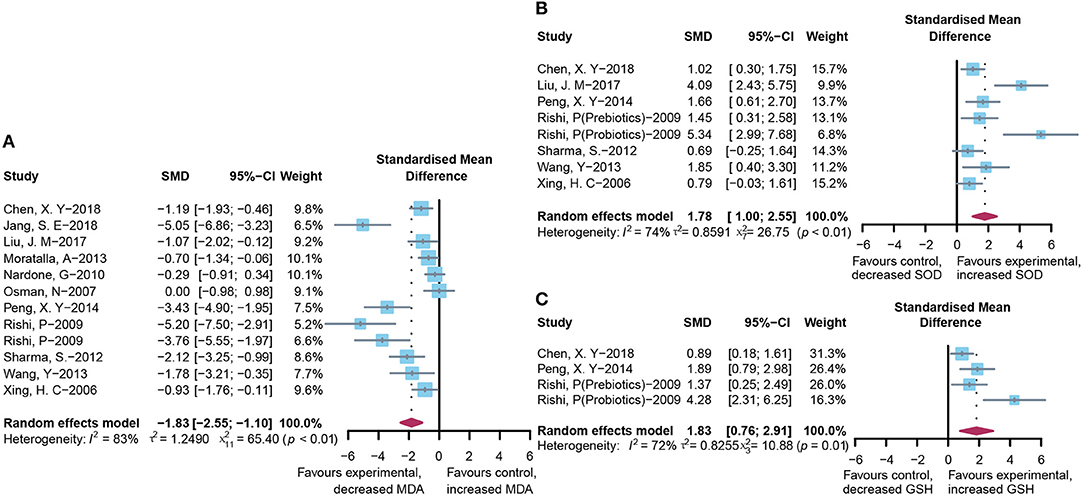
Figure 6. Effectiveness of microbial agents on oxidative stress. (A) The effect of microbial agents on MDA to evaluate free radical mediated lipid peroxidation injury. (B,C) The effect of microbial agents on SOD and GSH to evaluate antioxidant status of tissues.
Discussion
The crosstalk between the gut microbiota and the liver is increasingly emphasized, strengthened by the growing evidence that the alterations in composition or diversity of intestinal flora are involved in the progression and prognosis of many chronic liver diseases, including nonalcoholic fatty liver disease, liver cirrhosis, and hepatocellular carcinoma, and improvement in the gut microbiota has been shown to play a therapeutic role. The present study was designed to determine the effects of regulation of the microbiota using probiotics or prebiotics on ALI through the quantitative analysis of 26 reliable studies. To our knowledge, this is the first meta-analysis of microbial therapy for ALI.
The intimate bidirectional relationship between the gut and the liver constitutes the gut–liver axis, which also involves the biliary tract, the portal vein, the systemic circulation, and a series of systemic mediators. The gut-derived products permeate the intestinal barrier and translocate to the liver through the portal vein, thereby influencing liver function (35). Accordingly, the increased production of inflammatory cytokines during liver injury contributes to elevated systemic levels, reaching the intestine through the blood circulation and impairing intestinal mucosal immunity (36); this would, in turn, disturb the intestinal flora balance and disrupt the integrity of the gut barrier. Once this dynamic balance is disturbed under the invasion of various pathogenic factors, the dysfunction of the gut and liver is triggered. Fortunately, microbial agents, such as probiotics and prebiotics, can rectify the composition and metabolic activity of intestinal microflora, thereby restoring the homeostasis of the gut–liver axis.
The most predominant genera used in probiotic products are Lactobacillus and Bifidobacterium spp because of their antibacterial efficiency. These two beneficial bacteria compete with pathogenic bacteria for the same binding sites on intestinal epithelial cells and effectively abrogate the proliferation of pathogens by releasing antibacterial substances (37). In addition, the introduction of Lactobacillus and Bifidobacterium also contributes to the proliferation of other probiotics, resulting in the improvement in the microbial structure and abundance in specific intestinal sites. Our results showed that after the microecological intervention, with the increase in beneficial strains, such as Bifidobacterium, in the ileocecal area, the abundance of the harmful bacteria Enterococcus decreased, and correspondently, the rate of BT reduced. Besides the above classical health-promoting bacterial strains, other potential beneficial species, including Clostridium butyricum, Bacillus, and Pediococcus, which use oligosaccharides, were also promoted by prebiotics. The fermentation products of prebiotics can increase the production of mucin and regulate the action of hepatic lipogenic enzymes. As shown in our results, we observed a decline in aminotransferase and enhancement in the gut barrier compared to the control group. The prebiotics can thereby exert synergistic effects together with the stimulated beneficial bacteria in the treatment of liver injury (38).
The gut barrier plays an integral role in maintaining the homeostasis of the gut–liver axis. The progression of ALI is closely linked to deteriorated intestinal permeability, which can be restored by the administration of microbial agents. In our study, the intake of prebiotics or probiotics significantly increased the expression of the tight junction protein ZO-1 in the experimental group, indicating enhanced integrity of the gut barrier. Beneficial bacteria have been proven to produce metabolites, including secreted proteins, indole, short-chain fatty acids, and bacteriocins that enhance the gut barrier by directly promoting mucus secretion by goblet cells, increasing the release of antimicrobial peptides, or facilitating the expression of tight junction proteins. For instance, the soluble proteins p75 and p40 produced by Lactobacillus rhamnosus GG (LGG) transactivate the epidermal growth factor receptor (39), followed by the upregulation of a proliferation-inducing ligand in the epithelium (40), which, in turn, stimulates the secretion of immunoglobulin A and relieves cytokine-induced apoptosis in the intestinal epithelial cells (40). In addition, stimulated by p75 and p40, the intestine epithelial cells can produce heat stress proteins Hsp72 and Hsp25, which increase tight junction proteins and activate the Akt pathway in a phosphatidylinositol 3-kinase-dependent manner to enhance the survival of gut epithelial cells, thus forging an impregnable intestinal barrier to prevent BT and the invasion by toxins (41). Accordingly, we found less BT to the liver and mesenteric lymph node with a deceased level of endotoxin compared to the control group.
A serious liver injury could induce excessive partial or systemic inflammation, which may culminate in liver failure and even multiple organ dysfunction. BT and invasion of noxious entities through the portal system due to compromised gut barrier leads to the activation of Kupffer cells, followed by the combination of TLRs and LPS, resulting in the activation of mitogen-activated protein kinase (MAPK) and NF-κB that initiates the production of inflammatory cytokine TNF-α, IL-6, and IL-1β (42). In the inflammatory cascade, unsaturated fatty acids are driven by reactive oxygen species (ROS) to produce lipid peroxidase that triggers fatty acid side-chain reactions and generates MDA, which causes harm to protein and DNA, thereby disrupting basic physiological functions (43). Administration of microbial agents does not only ameliorate oxidative stress by suppressing ROS formation (44) but also significantly reduces the level of cytokines through inhibition of TLR (TLR4 and TLR5)-mediated endotoxin activation and decreases the phosphorylation of MAPK p38, thus attenuating the inflammatory response (44). Consistently, our findings suggested that probiotics or prebiotics inhibited the release of the cytokines TNF-α and IL-6, decreased the level of MDA, but increased the concentrations of the antioxidants SOD and GSH. According to subgroup analysis, the anti-inflammatory cytokine IL-10 showed an opposite trend in the liver and serum. It was reported that IL-10 plays a protective immunomodulatory role in inflammation-related liver diseases by downregulating the expression of major histocompatibility complex (MHC) II and maintaining the suppressive function of regulatory T cells; thus, the level of IL-10 in the liver is negatively correlated with the degree of liver injury (45). Since the modeling methods in most of the studies were applied locally instead of entering into the blood circulation directly, the concentration of cytokines in the liver would be high, making the increase in IL-10 in the serum relatively inconspicuous and inaccurate due to the limitation of observation time. The serum IL-10 level and the degree of liver injury appear to be positively correlated, which did not show significance in our results. Therefore, more evidence is needed to support and confirm the relationship between serum IL-10 and ALI.
The study presents several limitations. First, unavoidable heterogeneity emerged when we combined certain indicators due to the exploratory nature of animal studies, even if the random-effects model and subgroup analysis were performed. However, meta-regression indicated the source of heterogeneity, and sensitivity analysis confirmed that our results were robust. In addition, asymmetry appeared in the funnel plot, and Egger's test indicated the existence of publication bias. However, the contour-enhanced funnel plot with trim-and-fill methods ultimately demonstrated that heterogeneity was the main cause of the asymmetry, and the number of unpublished nonsignificant literature was estimated to be very small. In this context, our conclusions based on 26 articles are still of reference significance. Furthermore, we did not include symbiotics and postbiotics because the quality of the literature did not meet our expectations. Finally, we did not explore a dose–response relationship between microecological therapy and ALI efficacy because of the limited data provided by the literature.
Conclusion
Our study demonstrated that probiotics and prebiotics have a significant ameliorative effect on ALI through the gut–liver axis by upregulating tight junction protein ZO-1, correcting the abundance of the ileum flora, reducing BT and endotoxin invasion, and suppressing oxidative stress and proinflammatory mediators, thus, in turn, improving liver biochemical indicators. Our findings also provide a novel insight into the microbial therapies for ALI in clinical practice and imply a promising prospect of applying the gut–liver axis in the management of liver diseases. More clinical studies are required to facilitate the transformation of these preclinical research results into practical applications.
Data Availability Statement
The raw data supporting the conclusions of this article will be made available by the authors, without undue reservation.
Author Contributions
CX and FW conceived the study idea, guided the work, checked the data, and revised the manuscript. SX designed the retrieval strategy, analyzed the data, drew the pictures, and drafted the manuscript. MZ and QW screened the studies, assessed the methodology quality, extracted study information, and made the flow charts. ZXu and BP analyzed and checked the data. YX and ZD designed retrieval strategy and beautified the pictures. SW beautified the pictures and made the tables. ZXue guided the work and revised the manuscript. All co-authors had full access to and approved the final version of the manuscript.
Funding
This work was supported by grants from the Zhejiang Provincial Natural Science Foundation of China (LY20H180010) and Wenzhou Science and Technology Bureau (Y20180142).
Conflict of Interest
The authors declare that the research was conducted in the absence of any commercial or financial relationships that could be construed as a potential conflict of interest.
Publisher's Note
All claims expressed in this article are solely those of the authors and do not necessarily represent those of their affiliated organizations, or those of the publisher, the editors and the reviewers. Any product that may be evaluated in this article, or claim that may be made by its manufacturer, is not guaranteed or endorsed by the publisher.
Acknowledgments
We sincerely thank Ruijun Ni for her inspiring advice.
Supplementary Material
The Supplementary Material for this article can be found online at: https://www.frontiersin.org/articles/10.3389/fmed.2021.739337/full#supplementary-material
Abbreviations
ALI, Acute liver injury; ALT, Alanine transaminase; AST, Aspartate aminotransferase; BT, Bacterial translocation; CI, Confidence intervals; D-Ga1N, D-galactosamine; GSH, Glutathione; ICR, Imprinting Control Region; LPS, Lipopolysaccharides; MDA, Malondialdehyde; MD, Mean differences; NF-κB, Nuclear factor kappa B; OR, Odds ratio; ROS, Reactive oxygen species; SD, Standard deviations; SOD, Superoxide dismutase; TLRs, Toll-like receptors; ZO-1, Zonula occludens.
References
1. Crismale JF, Friedman SL. Acute liver injury and decompensated cirrhosis. Med Clin North Am. (2020) 104:647–62. doi: 10.1016/j.mcna.2020.02.010
2. Nibourg GA, Chamuleau RA, van der Hoeven TV, Maas MA, Ruiter AF, Lamers WH, et al. Liver progenitor cell line HepaRG differentiated in a bioartificial liver effectively supplies liver support to rats with acute liver failure. PLoS One. (2012) 7:e38778. doi: 10.1371/journal.pone.0038778
3. Zhou X, Cui L, Zhou X, Yang Q, Wang L, Guo G, et al. Induction of hepatocyte-like cells from human umbilical cord-derived mesenchymal stem cells by defined microRNAs. J Cell Mol Med. (2017) 21:881–93. doi: 10.1111/jcmm.13027
4. Milosevic I, Vujovic A, Barac A, Djelic M, Korac M, Radovanovic Spurnic A, et al. Gut-liver axis, gut microbiota, and its modulation in the management of liver diseases: a review of the literature. Int J Mol Sci. (2019) 20:395. doi: 10.3390/ijms20020395
5. Yiu JH, Dorweiler B, Woo CW. Interaction between gut microbiota and toll-like receptor: from immunity to metabolism. J Mol Med. (2017) 95:13–20. doi: 10.1007/s00109-016-1474-4
6. Page MJ, McKenzie JE, Bossuyt PM, Boutron I, Hoffmann TC, Mulrow CD, et al. The PRISMA 2020 statement: an updated guideline for reporting systematic reviews. BMJ. (2021) 372:n71. doi: 10.1136/bmj.n71
7. Hooijmans CR, Rovers MM, de Vries RB, Leenaars M, Ritskes-Hoitinga M, Langendam MW. SYRCLE's risk of bias tool for animal studies. BMC Med Res Methodol. (2014) 14:43. doi: 10.1186/1471-2288-14-43
8. Adawi D, Ahrné S, Molin G. Effects of different probiotic strains of Lactobacillus and Bifidobacterium on bacterial translocation and liver injury in an acute liver injury model. Int J Food Microbiol. (2001) 70:213–20. doi: 10.1016/S0168-1605(01)00550-5
9. Adawi D, Molin G, Jeppsson B. Inhibition of nitric oxide production and the effects of arginine and Lactobacillus administration in an acute liver injury model. Ann Surg. (1998) 228:748–55. doi: 10.1097/00000658-199812000-00005
10. Han SY, Huh CS, Ahn YT, Lim KS, Baek YJ, Kim DH. Hepatoprotective effect of lactic acid bacteria, inhibitors of beta-glucuronidase production against intestinal microflora. Arch Pharm Res. (2005) 28:325–9. doi: 10.1007/BF02977800
11. Jia L, Zhang MH. Comparison of probiotics and lactulose in the treatment of minimal hepatic encephalopathy in rats. World J Gastroenterol. (2005) 11:908–11. doi: 10.3748/wjg.v11.i6.908
12. Kasravi FB, Adawi D, Molin G, Bengmark S, Jeppsson B. Effect of oral supplementation of lactobacilli on bacterial translocation in acute liver injury induced by D-galactosamine. J Hepatol. (1997) 26:417–24. doi: 10.1016/S0168-8278(97)80060-8
13. Li YT, Wang L, Chen Y, Chen YB, Wang HY, Wu ZW, et al. Effects of gut microflora on hepatic damage after acute liver injury in rats. J Trauma. (2010) 68:76–83. doi: 10.1097/TA.0b013e31818ba467
14. Nardone G, Compare D, Liguori E, Di Mauro V, Rocco A, Barone M, et al. Protective effects of Lactobacillus paracasei F19 in a rat model of oxidative and metabolic hepatic injury. Am J Physiol Gastrointest Liver Physiol. (2010) 299:G669–G76. doi: 10.1152/ajpgi.00188.2010
15. Nicaise C, Prozzi D, Viaene E, Moreno C, Gustot T, Quertinmont E, et al. Control of acute, chronic, and constitutive hyperammonemia by wild-type and genetically engineered Lactobacillus plantarum in rodents. Hepatology. (2008) 48:1184–92. doi: 10.1002/hep.22445
16. Osman N, Adawi D, Ahrne S, Jeppsson B, Molin G. Endotoxin- and D-galactosamine-induced liver injury improved by the administration of Lactobacillus, Bifidobacterium and blueberry. Dig Liver Dis. (2007) 39:849–56. doi: 10.1016/j.dld.2007.06.001
17. Park JH, Kim Y, Kim SH. Green tea extract (Camellia sinensis) fermented by Lactobacillus fermentum attenuates alcohol-induced liver damage. Biosci Biotechnol Biochem. (2012) 76:2294–300. doi: 10.1271/bbb.120598
18. Rishi P, Bharrhan S, Singh G, Kaur IP. Effect of Lactobacillus plantarum and L-arginine against endotoxin-induced liver injury in a rat model. Life Sci. (2011) 89:847–53. doi: 10.1016/j.lfs.2011.09.007
19. Rishi P, Mavi SK, Bharrhan S, Shukla G, Tewari R. Protective efficacy of probiotic alone or in conjunction with a prebiotic in Salmonella-induced liver damage. FEMS Microbiol Ecol. (2009) 69:222–30. doi: 10.1111/j.1574-6941.2009.00703.x
20. Sharma S, Chaturvedi J, Chaudhari BP, Singh RL, Kakkar P. Probiotic Enterococcus lactis IITRHR1 protects against acetaminophen-induced hepatotoxicity. Nutrition. (2012) 28:173–81. doi: 10.1016/j.nut.2011.02.012
21. Wang Y, Li Y, Xie J, Zhang Y, Wang J, Sun X, et al. Protective effects of probiotic Lactobacillus casei Zhang against endotoxin- and d-galactosamine-induced liver injury in rats via anti-oxidative and anti-inflammatory capacities. Int Immunopharmacol. (2013) 15:30–7. doi: 10.1016/j.intimp.2012.10.026
22. Xing HC, Li LJ, Xu KJ, Shen T, Chen YB, Sheng JF, et al. Protective role of supplement with foreign Bifidobacterium and Lactobacillus in experimental hepatic ischemia-reperfusion injury. J Gastroenterol Hepatol. (2006) 21:647–56. doi: 10.1111/j.1440-1746.2006.04306.x
23. Shi D, Lv L, Fang D, Wu W, Hu C, Xu L, et al. Administration of Lactobacillus salivarius LI01 or Pediococcus pentosaceus LI05 prevents CCl4-induced liver cirrhosis by protecting the intestinal barrier in rats. Sci Rep. (2017) 7:6927. doi: 10.1038/s41598-017-07091-1
24. Moratalla A, Gomez-Hurtado I, Santacruz A, Moya A, Peiro G, Zapater P, et al. Protective effect of Bifidobacterium pseudocatenulatum CECT7765 against induced bacterial antigen translocation in experimental cirrhosis. Liver Int. (2014) 34:850–8. doi: 10.1111/liv.12380
25. Peng X, Jiang Y. Protective effects of Lactobacillus plantarum NDC 75017 against lipopolysaccharide-induced liver injury in mice. Inflammation. (2014) 37:1599–607. doi: 10.1007/s10753-014-9886-1
26. Jin P, Chen Y, Lv L, Yang J, Lu H, Li L. Lactobacillus fermentum ZYL0401 attenuates lipopolysaccharide-induced hepatic TNF-α expression and liver injury via an IL-10- and PGE2-EP4-dependent mechanism. PLoS One. (2015) 10:e0126520. doi: 10.1371/journal.pone.0126520
27. Liu J, Fu Y, Zhang H, Wang J, Zhu J, Wang Y, et al. The hepatoprotective effect of the probiotic Clostridium butyricum against carbon tetrachloride-induced acute liver damage in mice. Food Funct. (2017) 8:4042–52. doi: 10.1039/C7FO00355B
28. Chen X, Zhang J, Yi R, Mu J, Zhao X, Yang Z. Hepatoprotective effects of Lactobacillus on carbon tetrachloride-induced acute liver injury in mice. Int J Mol Sci. (2018) 19:2212. doi: 10.3390/ijms19082212
29. Jang SE, Jeong JJ, Kim JK, Han MJ, Kim DH. Simultaneous amelioratation of colitis and liver injury in mice by Bifidobacterium longum LC67 and Lactobacillus plantarum LC27. Sci Rep. (2018) 8:7500. doi: 10.1038/s41598-018-25775-0
30. Cui Y, Qi S, Zhang W, Mao J, Tang R, Wang C, et al. Lactobacillus reuteri ZJ617 culture supernatant attenuates acute liver injury induced in mice by lipopolysaccharide. J Nutr. (2019) 149:2046–55. doi: 10.1093/jn/nxz088
31. Ding L, Gong Y, Yang Z, Zou B, Liu X, Zhang B, et al. Lactobacillus rhamnosus GG ameliorates liver injury and hypoxic hepatitis in rat model of CLP-induced sepsis. Dig Dis Sci. (2019) 64:2867–77. doi: 10.1007/s10620-019-05628-0
32. Li Y, Lv L, Ye J, Fang D, Shi D, Wu W, et al. Bifidobacterium adolescentis CGMCC 15058 alleviates liver injury, enhances the intestinal barrier and modifies the gut microbiota in D-galactosamine-treated rats. Appl Microbiol Biotechnol. (2019) 103:375–93. doi: 10.1007/s00253-018-9454-y
33. Wang K, Lv L, Yan R, Wang Q, Jiang H, Wu W, et al. Bifidobacterium longum R0175 protects rats against d-Galactosamine-induced acute liver failure. mSphere. (2020) 5. doi: 10.1128/mSphere.00791-19
34. Peters JL, Sutton AJ, Jones DR, Abrams KR, Rushton L. Contour-enhanced meta-analysis funnel plots help distinguish publication bias from other causes of asymmetry. J Clin Epidemiol. (2008) 61:991–6. doi: 10.1016/j.jclinepi.2007.11.010
35. Tripathi A, Debelius J, Brenner DA, Karin M, Loomba R, Schnabl B, et al. The gut-liver axis and the intersection with the microbiome. Nat Rev Gastroenterol Hepatol. (2018) 15:397–411. doi: 10.1038/s41575-018-0011-z
36. Stärkel P, Schnabl B. Bidirectional communication between liver and gut during alcoholic liver disease. Semin Liver Dis. (2016) 36:331–9. doi: 10.1055/s-0036-1593882
37. Roodposhti PM, Dabiri N. Effects of probiotic and prebiotic on average daily gain, fecal shedding of Escherichia coli, and immune system status in newborn female calves. Asian Aust J Anim Sci. (2012) 25:1255–61. doi: 10.5713/ajas.2011.11312
38. Markowiak P, Slizewska K. Effects of probiotics, prebiotics, and synbiotics on human health. Nutrients. (2017) 9:1021. doi: 10.3390/nu9091021
39. Yan F, Cao H, Cover TL, Washington MK, Shi Y, Liu L, et al. Colon-specific delivery of a probiotic-derived soluble protein ameliorates intestinal inflammation in mice through an EGFR-dependent mechanism. J Clin Invest. (2011) 121:2242–53. doi: 10.1172/JCI44031
40. Wang Y, Liu L, Moore DJ, Shen X, Peek RM, Acra SA, et al. An LGG-derived protein promotes IgA production through upregulation of APRIL expression in intestinal epithelial cells. Mucosal Immunol. (2017) 10:373–84. doi: 10.1038/mi.2016.57
41. Yan F, Cao H, Cover TL, Whitehead R, Washington MK, Polk DB. Soluble proteins produced by probiotic bacteria regulate intestinal epithelial cell survival and growth. Gastroenterology. (2007) 132:562–75. doi: 10.1053/j.gastro.2006.11.022
42. Inokuchi S, Tsukamoto H, Park E, Liu ZX, Brenner DA, Seki E. Toll-like receptor 4 mediates alcohol-induced steatohepatitis through bone marrow-derived and endogenous liver cells in mice. Alcohol Clin Exp Res. (2011) 35:1509–18. doi: 10.1111/j.1530-0277.2011.01487.x
43. Lin MY, Yen CL. Reactive oxygen species and lipid peroxidation product-scavenging ability of yogurt organisms. J Dairy Sci. (1999) 82:1629–34. doi: 10.3168/jds.S0022-0302(99)75391-9
44. Wang Y, Liu Y, Kirpich I, Ma Z, Wang C, Zhang M, et al. Lactobacillus rhamnosus GG reduces hepatic TNFα production and inflammation in chronic alcohol-induced liver injury. J Nutr Biochem. (2013) 24:1609–15. doi: 10.1016/j.jnutbio.2013.02.001
Keywords: acute liver injury, probiotics, prebiotics, gut microbiota, gut-liver axis, meta-analysis
Citation: Xu S, Zhao M, Wang Q, Xu Z, Pan B, Xue Y, Dai Z, Wang S, Xue Z, Wang F and Xu C (2021) Effectiveness of Probiotics and Prebiotics Against Acute Liver Injury: A Meta-Analysis. Front. Med. 8:739337. doi: 10.3389/fmed.2021.739337
Received: 10 July 2021; Accepted: 11 August 2021;
Published: 21 September 2021.
Edited by:
Pradeep Kumar Shukla, University of Tennessee Health Science Center (UTHSC), United StatesReviewed by:
Geetha Samak, DVS College of Arts and Science, IndiaAmal Mondal, Jawaharlal Nehru University, India
Copyright © 2021 Xu, Zhao, Wang, Xu, Pan, Xue, Dai, Wang, Xue, Wang and Xu. This is an open-access article distributed under the terms of the Creative Commons Attribution License (CC BY). The use, distribution or reproduction in other forums is permitted, provided the original author(s) and the copyright owner(s) are credited and that the original publication in this journal is cited, in accordance with accepted academic practice. No use, distribution or reproduction is permitted which does not comply with these terms.
*Correspondence: Changlong Xu, xchlong@163.com; Fangyan Wang, wzyxywfy@126.com
†These authors have contributed equally to this work and share first authorship