- 1Department of Clinical Research, University of Southern Denmark, Odense, Denmark
- 2Department of Clinical Microbiology, Odense University Hospital, Odense, Denmark
- 3Department of Biochemistry and Molecular Biology, University of Southern Denmark, Odense, Denmark
- 4Department of Regional Health Research, University of Southern Denmark, Odense, Denmark
- 5Regional Department of Clinical Microbiology, Zealand University Hospital, Slagelse, Denmark
A new easy-to-use online bioinformatic tool analyzing whole genome sequences of healthcare associated bacteria was used by a local infection control unit to retrospectively map genetic relationship of isolates of E. faecium carrying resistance genes to vancomycin in a hospital. Three clusters of isolates were detected over a period of 5 years, suggesting transmission between patients. Individual relatedness between isolates within each cluster was established by SNP analyses provided by the system. Genetic antimicrobial resistance mechanisms to antibiotics other than vancomycin were identified. The results suggest that the system is suited for hospital surveillance of E. faecium carrying resistance genes to vancomycin in settings with access to next Generation Sequencing without bioinformatic expertise for interpretation of the genome sequences.
Introduction
Surveillance of specific microorganisms in hospitals and evaluation of genetic relationship of isolates is fundamental in detecting and interrupting transmission. Whole Genome Sequencing (WGS) offers a unique tool for analyzing relationship of vancomycin resistant enterococci (1–3), and may serve as a method for typing the bacteria for local surveillance. While bioinformatic analyses of WGS data have generally been hampered by complicated procedures, tools requiring no bioinformatic skills are now available to support infection control. Here, a local infection control unit retrospectively analyzed all available genome sequences of Enterococcus faecium carrying resistance genes to vancomycin isolated at a hospital from 2014 to 2018 by a new online tool, 1928 Diagnostics. Based on WGS data 1928 Diagnostics analyze core genome genetic relationship and genetic antibiotic resistance mechanisms in individual bacterial isolates (4).
Materials and Methods
Clinical Isolates
The study was carried out at Odense University Hospital, which is a >1,000 bed university hospital placed at two geographical locations. Isolates of E. faecium carrying vanA and/or vanB gene from clinical (non-screening) samples were analyzed. The first isolate from each patient in the years 2014 to 2018 was included. Date and place of sampling was recorded. No patient information was included. Core genome Multi Locus Sequencing Typing (cgMLST) of some of the included isolates have previously been reported (5, 6).
Whole Genome Sequencing Data Analyses
WGS was carried out by 2 ×150 bp paired end sequencing using a MiSeq instrument (Illumina, San Diego, CA, USA). Compressed unassembled sequence files were uploaded to the 1,928 Diagnostics pipeline (https://www.1928diagnostics.com/). The system initially performed a quality check based on sequencing depth/coverage. Results for individual isolates processed by 1,928 Diagnostics were presented with MLST sequence type (ST) and antibiotic resistance gene profiles. A cgMLST based Unweighted Pair Group Method with Arithmetic mean (UPGMA) dendrogram showing number of allelic differences was generated by the system. One thousand nine hundred twenty-eight Diagnostics uses the resqu database for identification of horizontally transferred antibiotic resistance genes (https://www.1928diagnostics.com/resdb/).
Isolates with 20 or less allelic differences were considered genetically related (7). Clusters of more than three genetically related isolates were studied in detail by the single nucleotide polymorphism (SNP) analysis function in 1928 Diagnostics. Closest Genbank complete chromosome sequence, identified by KmerResistance (https://cge.cbs.dtu.dk/services/kmerresistance/) using sequence files from a randomly selected isolate from each cluster, served as reference. The exclusion distance was set to 10.
Results
MLST
A total of 64 genomes from E. faecium were available for analyses.
Fifty-eight isolates carried the vanA gene, five carried vanB gene and one isolate from December 2018 carried both vanA and vanB gene (Figure 1). One isolate was not typeable by MLST. This was confirmed by MLST-2.0 (https://cge.cbs.dtu.dk/services/MLST/), which failed to detect hits in the pstS locus. The most common MLST type was ST80, including 30 isolates of which 26 carried vanA gene, three carried vanB gene, and the one isolate carrying both vanA and vanB genes. The UPGMA dendrogram showed large allelic variation in the core genome of the ST80 isolates (Figure 1). Twelve isolates were ST203 and eight were ST1421.
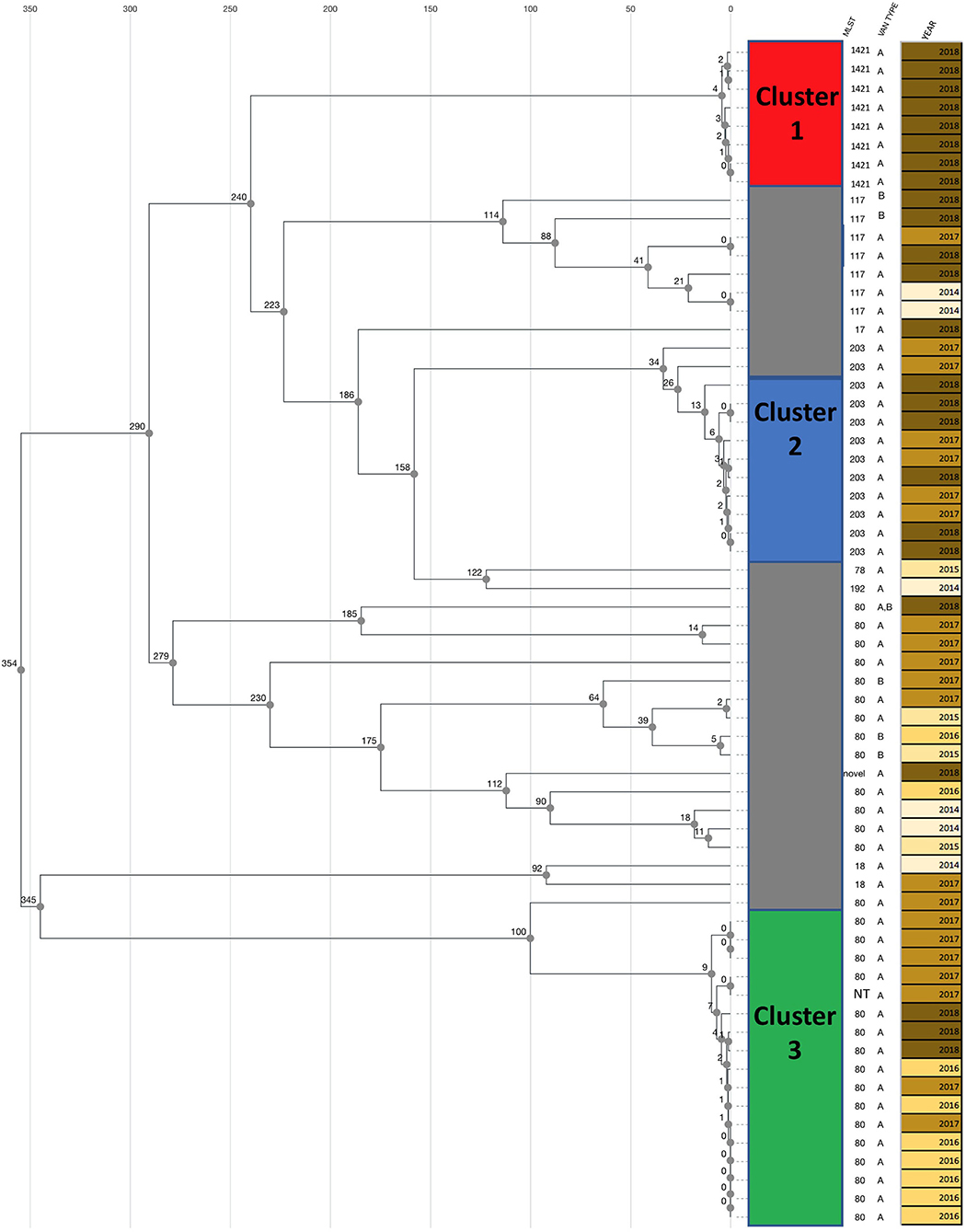
Figure 1. cgMLST based UPGMA clustering dendrogram of clinical (non-screening) E. faecium carrying resistance genes to vancomycin isolated 2014–2018 isolated at a large university hospital. Three clusters of genetically related isolates were identified. The number of allelic differences between individual isolates are indicated at each node. The MLST sequence type and the van gene (vanA or vanB gene) is indicated for each isolate next to the dendrogram. One isolate was not typable (NT). One isolate carried both vanA and vanB gene. Year of isolation is indicated for individual isolates.
Core Genome MLST, Epidemiology, and Antibiotic Resistance Gene Profiles
Three clusters were evident form the cgMLST based UPGMA dendrogram (Figure 1). Cluster 1 consisted of eight isolates of vanA gene positive E. faecium ST1421. All isolates in this cluster were from the second half of 2018 (Figure 2). The SNP analyses (Figure 3) showed few SNP differences between isolates. In addition to the vanA gene, the isolates all carried resistance genes to aminoglycosides [aac(6'), ant(9')-la, aph(3')-IIIa, macrolide-lincosamide-streptogramin B (MLS), erm(A), erm(B), msr(C), and trimethoprim dfr(G)]. All resistance genes had more than 98.5% amino acid match and 100% length match compared to the reference sequence. Six isolates were cultured from patients at five hospital departments and two isolates were from patients consulting General Practitioners.
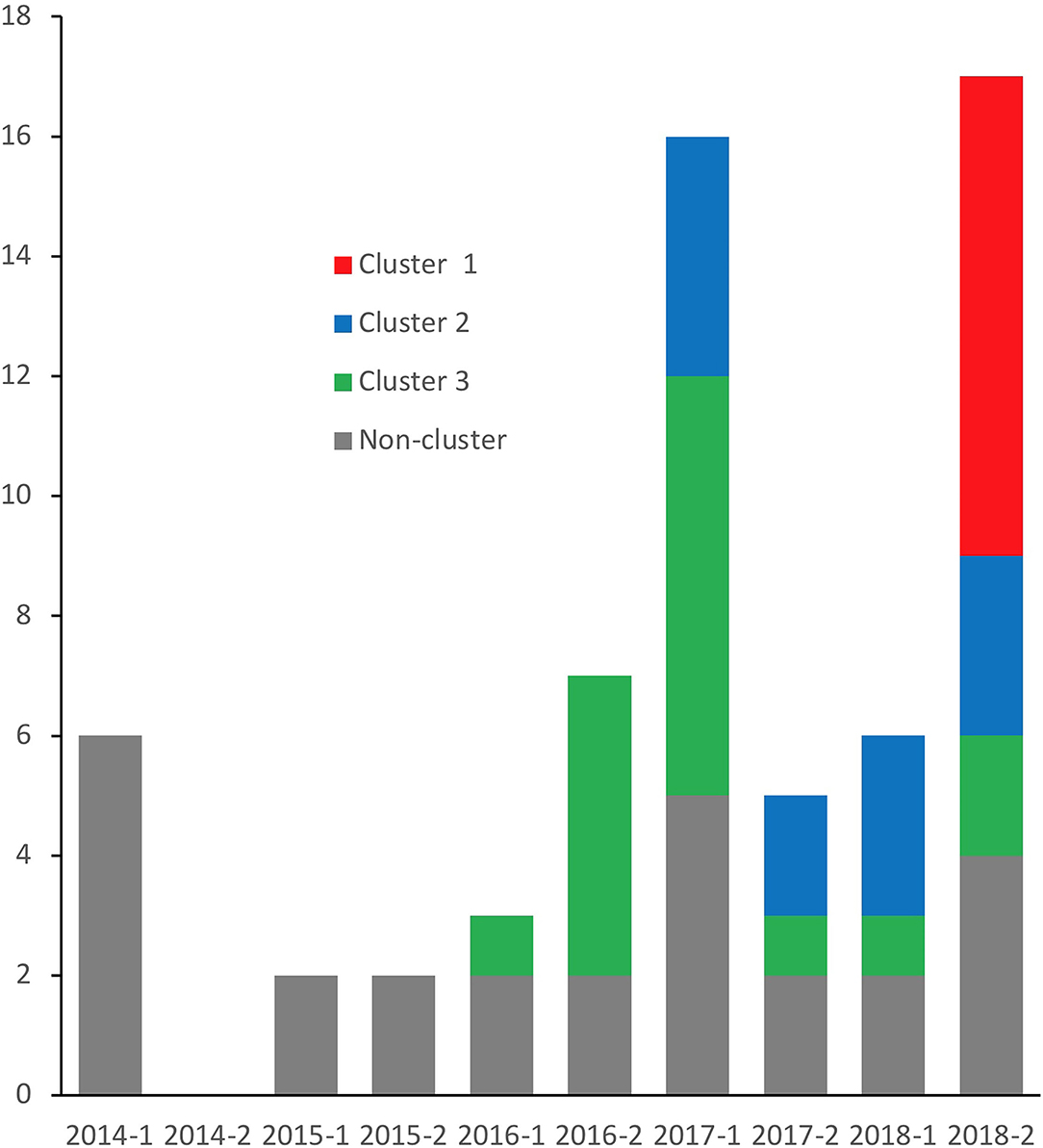
Figure 2. Number of isolates per half a year. Isolates of the three clusters identified from Figure 1 are indicated by color.
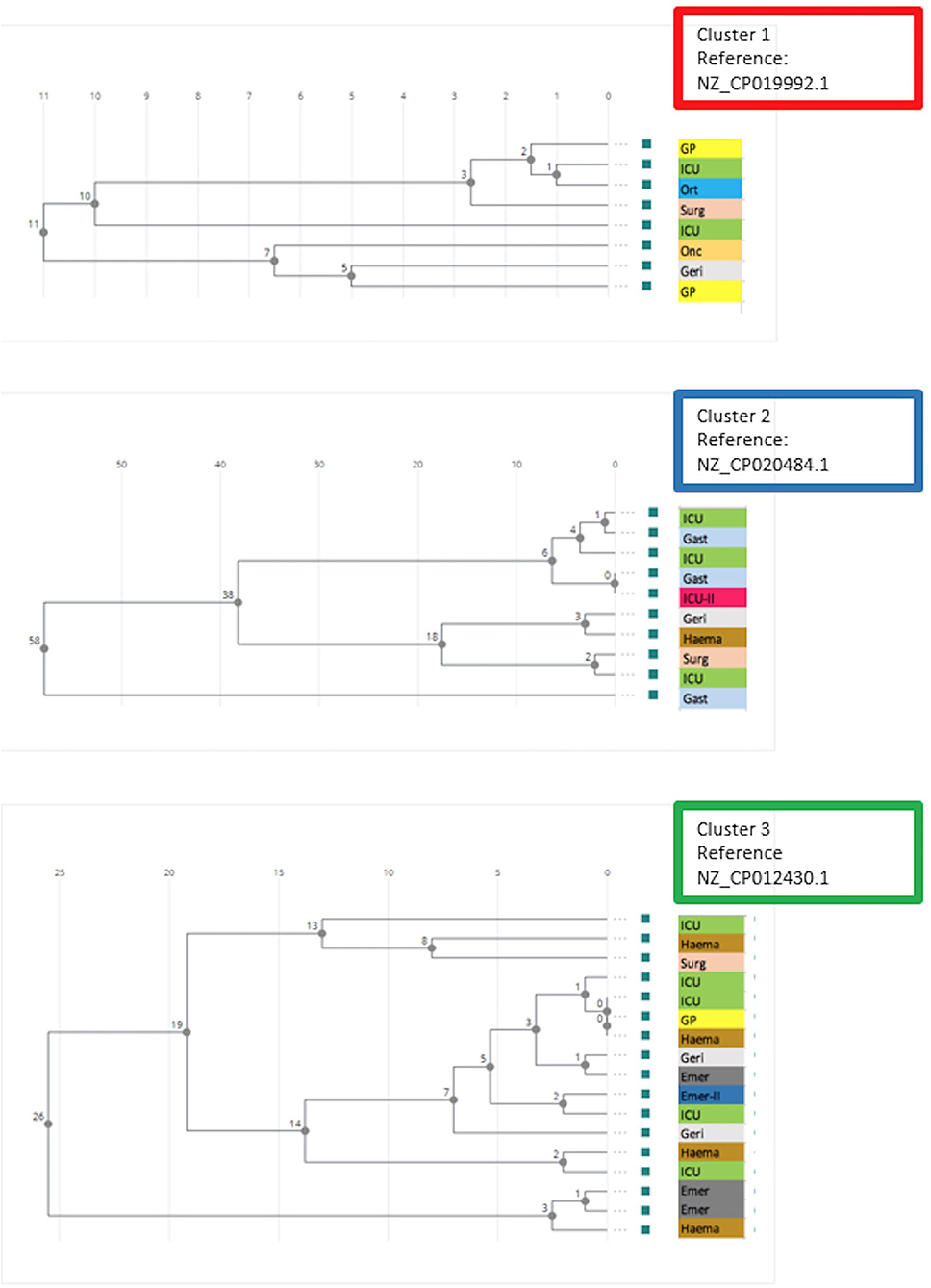
Figure 3. Phylogenetic trees based on SNPs in the core genome of E. faecium carrying vanA gene in the three clusters from Figure 1. Reference chromosome genome used for the analysis is indicated for each cluster. Location for sampling (General Practitioner or hospital department) is indicated for each isolate. ICU, Intensive Care Unit (hospital location 1); Ort, orthopedic surgery; Surg, abdominal surgery; Onc, oncology; Geri, geriatrics; GP, General Practitioner; Gast, gastroenterology; ICU-II, Intensive Care Unit at second hospital location; Haema, hematology; Emer, emergency; Emer-II, emergency at second location.
Cluster 2 included 10 ST203 isolates carrying vanA gene. The bacteria were cultured from first half of 2017 onwards (Figure 2). The isolates were cultured from patients at seven hospital departments. The isolates in Cluster 2 SNP analyses showed larger variation compared to the isolates in cluster 1 (Figure 3). All isolates in this cluster carried resistance genes to aminoglycosides [aac(6'), ant(6')-la, MLS erm(B), msr(C), trimethoprim (dfrG), and tetracycline tet(M), tet(U)]. Except for one isolate with a length fraction of 94.3% of the reference sequences, all resistance genes had more than 98.5% amino acid identity and 100% length to the references. The catA gene, an effector of chloramphenicol resistance, was detected in seven of the isolates. The amino acid sequence and length were both 100% identical to the reference sequence.
Cluster 3 included 17 vanA gene positive isolates. Sixteen of the isolates were typed as ST80, while one was not typeable. MLST 2.0 (https://cge.cbs.dtu.dk/services/MLST/) also failed to type this isolate. The isolates in cluster 3 were cultured from 2016 onwards and showed numbers of SNP differences between those detected in Cluster 1 and Cluster 2. The isolates carried resistance genes to aminoglycosides [aac(6'), aph(3')-IIIa, MLS erm(B), msr(C), and tetracyclines tet(M)]. With three exceptions both identity and fragment length were more than 98% of the reference sequences for all of these resistance genes. The E. faecium in this cluster were collected from six departments and one GP from 2016 onwards.
Discussion
WGS offers a unique method for typing and monitoring healthcare associated bacteria such as enterococci carrying resistance genes to vancomycin. For the technique to be useful in clinical practice, the bioinformatic handling of sequence data should be easy and fast. Access to external sequencing facilities may provide high quality genome sequences without data analyses and interpretation. We used 1928 Diagnostics, a new online tool for bioinformatic analyses of transmissible bacteria, to review the genetic characteristics of E. faecium carrying genes encoding resistance to vancomycin at the hospital during a period of 5 years.
The genomes of the ST80 isolates were highly heterogenous, including isolates carrying vanA gene and isolates with vanB gene. One isolate of ST80 E. faecium carried both vanA and vanB genes as did 5% of vancomycin-resistant E. faecium in Denmark in 2018 (5). Heterogenous ST80 vancomycin-esistant E. faecium has been reported from Denmark (8). Recent studies of ST80 vancomycin-resistant E. faecium in Sweden showed comparable epidemiological discrimination by 1,928 Diagnostics cgMLST and SNP analyses (9).
Three major clusters of related isolates were evident. The clusters replaced each other over time as the most frequently isolated strain and isolates of the strains within each cluster continued to be detected afterwards. The Cluster 1 strain of E. faecium ST1421 carried the vanA gene without necessarily expressing resistance to vancomycin in vitro (10). This strain was frequently detected in Denmark in 2018 and 2019 (5). Cluster 2 and Cluster 3 also reflected the nation-wide occurrence of dominating strains of E. faecium carrying vanA gene during the 5 years (5). Genes encoding resistance to antibiotics other than vancomycin varied between clusters and were largely identical in isolates within each cluster. Knowledge on mechanisms of resistance to antimicrobials other than vancomycin may potentially contribute to treatment guidance and to tracking routes of transmission of antimicrobial resistance.
The detailed relatedness between isolates within each cluster was established by SNP analyses. No association between place of sampling and relatedness of isolates was evident. However, location of sampling does often not reflect place of transmission. It is noteworthy, that bacteria of all three clusters were isolated from patients referred to the same Intensive Care Unit and that 10 of the isolates were from patients admitted to this unit. This may be due to patient factors, use of broad-spectrum antimicrobials, extensive use of indwelling foreign body materials, and frequent sampling practice.
In conclusion, this retrospective study showed that a rapid and easy-to-use bioinformatic pipeline clearly separated isolates of E. faecium with resistance genes to vancomycin into clusters and sporadic strains and allowed detailed analyses of clusters of related isolates. Prospective studies should establish the usefulness of 1928 Diagnostics and similar systems for real time surveillance of vancomycin resistant E. faecium and other hospital-associated bacteria.
Data Availability Statement
The original contributions presented in the study are publicly available. This data can be found at: https://www.ncbi.nlm.nih.gov/sra/?term=PRJNA767758.
Author Contributions
MK, LM, and LA conceived and designed the study. MK, LM, CS, S-AS, AT, and LW analyzed the data. MK, LM, CS, S-AS, AT, LA, TA, and BK critically revised the manuscript. All authors read and approved the manuscript.
Funding
The study was supported by a research grant from Odense University Hospital (Grant No.: 72-A3862).
Conflict of Interest
The authors declare that the research was conducted in the absence of any commercial or financial relationships that could be construed as a potential conflict of interest.
Publisher's Note
All claims expressed in this article are solely those of the authors and do not necessarily represent those of their affiliated organizations, or those of the publisher, the editors and the reviewers. Any product that may be evaluated in this article, or claim that may be made by its manufacturer, is not guaranteed or endorsed by the publisher.
Acknowledgments
Statens Serum Institut, Copenhagen, Denmark is thanked for sharing genome sequence data.
References
1. Neumann B, Bender JK, Maier BF, Wittig A, Fuchs S, Brockmann D, et al. Comprehensive integrated NGS-based surveillance and contact-network modeling unravels transmission dynamics of vancomycin-resistant enterococci in a high-risk population within a tertiary care hospital. PLoS ONE. (2020) 15:e0235160. doi: 10.1371/journal.pone.0235160
2. Xanthopoulou K, Peter S, Tobys D, Behnke M, Dinkelacker AG, Eisenbeis S, et al. Vancomycin-resistant Enterococcus faecium colonizing patients on hospital admission in Germany: prevalence and molecular epidemiology. J Antimicrob Chemother. (2020) 75:2743–51. doi: 10.1093/jac/dkaa271
3. Pinholt M, Gumpert H, Bayliss S, Nielsen JB, Vorobieva V, Pedersen M, et al. Genomic analysis of 495 vancomycin-resistant Enterococcus faecium reveals broad dissemination of a vanA plasmid in more than 19 clones from Copenhagen, Denmark. J Antimicrob Chemother. (2017) 2017:7240–7. doi: 10.1093/jac/dkw360
4. Slott Jensen ML, Chen M, Klitgaard JK, Andersen TE, Kemp M. Whole genome sequence analyses by a new easy-to-use software solution support suspicion of a neonatal ward outbreak of MRSA ST22 and transmission between hospitals. Infect Control Hospital Epidemiol. (2021) 2021:123. doi: 10.1017/ice.2021.123
5. Hammerum AM, Justesen US, Pinholt M, Roer L, Kaya H, Worning P, et al. Surveillance of vancomycin-resistant enterococci reveals shift in dominating clones and national spread of a vancomycin-variable vanA Enterococcus faecium ST1421-CT1134 clone, Denmark, 2015 to March 2019. Euro Surveill. (2019) 24:1900503. doi: 10.2807/1560-7917.ES.2019.24.34.1900503
6. Hansen SK, Andersen L, Detlefsen M, Holm A, Roer L, Antoniades P, et al. Use of core genomes MLST for infection control during a Danish outbreak of vancomycin-resistant Enterococcus faecium. J Glob Antimicrob Resist. (2021) 24:418–23. doi: 10.1016/j.jgar.2021.02.007
7. de Been M, Pinholt M, Top J, Bletz S, Mellmann A, van Schaik W, et al. Core genome multilocus sequence typing scheme for high- resolution typing of Enterococcus faecium. J Clin Microbiol. (2015) 53:3788–97. doi: 10.1128/JCM.01946-15
8. Pinholt M, Bayliss SC, Gumpert H, Worning P, Jensen VVS, Pedersen M, et al. WGS of 1058 Enterococcus faecium from Copenhagen, Denmark, reveals rapid clonal expansion of vancomycin-resistant clone ST80 combined with widespread dissemination of a vanA-containing plasmid and acquisition of a heterogeneous accessory genome. J Antimicrob Chemother. (2019) 74:1776–85. doi: 10.1093/jac/dkz118
9. Dyrkell F, Giske CG, Fang H. Epidemiological typing of ST80 vancomycin-resistant Enterococcus faecium: core-genome multi-locus sequence typing vs. single nucleotide polymorphism-based typing. J Glob Antimicrob Resist. (2021) 25:119–23. doi: 10.1016/j.jgar.2021.03.005
Keywords: vancomycin resistant enterococci, whole genome sequencing, bioinformatics, genomic epidemiology, infection control, surveillance
Citation: Marbjerg L, Stougaard CL, Sørensen S-AG, Thomsen AV, Wang L, Andersen L, Andersen TE, Kallipolitis B and Kemp M (2021) A New Tool for Analyses of Whole Genome Sequences Reveals Dissemination of Specific Strains of Vancomycin-Resistant Enterococcus faecium in a Hospital. Front. Med. 8:733676. doi: 10.3389/fmed.2021.733676
Received: 30 June 2021; Accepted: 05 October 2021;
Published: 27 October 2021.
Edited by:
Yann Felix Boucher, National University of Singapore, SingaporeReviewed by:
Paulo J. M. Bispo, Harvard Medical School, United StatesAndrei Nicoli Gebieluca Dabul, University of São Paulo, Brazil
Copyright © 2021 Marbjerg, Stougaard, Sørensen, Thomsen, Wang, Andersen, Andersen, Kallipolitis and Kemp. This is an open-access article distributed under the terms of the Creative Commons Attribution License (CC BY). The use, distribution or reproduction in other forums is permitted, provided the original author(s) and the copyright owner(s) are credited and that the original publication in this journal is cited, in accordance with accepted academic practice. No use, distribution or reproduction is permitted which does not comply with these terms.
*Correspondence: Michael Kemp, mkemp@regionsjaelland.dk