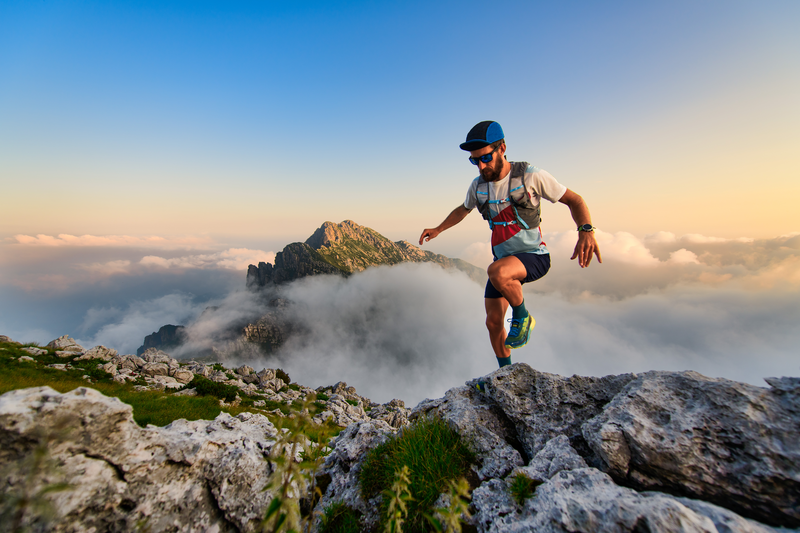
95% of researchers rate our articles as excellent or good
Learn more about the work of our research integrity team to safeguard the quality of each article we publish.
Find out more
SYSTEMATIC REVIEW article
Front. Med. , 22 July 2021
Sec. Pulmonary Medicine
Volume 8 - 2021 | https://doi.org/10.3389/fmed.2021.704222
This article is part of the Research Topic From Development to Senescence, Bridging the Gap in Lung Fibrosis View all 8 articles
Pulmonary fibrosis (PF) is a serious lung disease which can result from known genetic or environmental exposures but is more commonly idiopathic (IPF). In familial PF (FPF), the majority of identified causal genes play key roles in the maintenance of telomeres, the protective end structures of chromosomes. Recent evidence suggests that short telomeres may also be implicated causally in a significant proportion of idiopathic cases. The possible involvement of herpes viruses in PF disease incidence and progression has been examined for many years, with some studies showing strong, statistically significant associations and others reporting no involvement. Evidence is thus polarized and remains inconclusive. Here we review the reported involvement of herpes viruses in PF in both animals and humans and present a summary of the evidence to date. We also present several possible mechanisms of action of the different herpes viruses in PF pathogenesis, including potential contributions to telomere attrition and cellular senescence. Evidence for antiviral treatment in PF is very limited but suggests a potential benefit. Further work is required to definitely answer the question of whether herpes viruses impact PF disease onset and progression and to enable the possible use of targeted antiviral treatments to improve clinical outcomes.
A role for herpes viruses in pulmonary fibrosis remains controversial. Herpes viruses are DNA viruses and include herpes simplex (HSV) 1 & 2, Epstein Barr Virus (EBV), cytomegalovirus (CMV) and human herpes viruses (HHV6, HHV7 and HHV8 or Kaposi's Sarcoma-associated virus). Herpes viruses are ubiquitous; HSV affects up to 95% (1), EBV up to 90% (2) and CMV about 60% (3) of European populations, its prevalence rising with age. In contrast, other herpes viruses, such as HHV8 have high prevalence only in certain populations, such as Sub-Saharan Africans (4). Once herpes virus is established in a host, infection becomes latent and persists for life. Latent virus localizes within the nucleus and generally persists in episomal form, periodically reactivating as lytic virus, with different cell tropism and protein expression.
Lytic viral expression is often subclinical but may also be responsible for typical clinical manifestations such as cold sores (HSV1) or infectious mononucleosis (EBV or CMV), or in severely immunosuppressed people necrotising retinitis, encephalitis or pneumonitis (5). Periodic reactivation is universal but frequency and clinical consequences depend on multiple genetic and environmental factors, many of which are poorly understood. Herpes viruses can also integrate into chromosomal DNA and herpes viral DNA can be found in around 1% of people (6). In some cases, this integrated material can reactivate, raising the possibility of functional consequences (7).
Pulmonary fibrosis (PF) is a feature of the interstitial lung diseases, marking a progressive disorder characterized by alveolar-interstitial inflammation, accumulation of extracellular matrix, distortion of normal architecture and impairment of gas exchange. Slow deterioration in lung function is interspersed with acute exacerbations; these episodes of rapid decline in lung function, associated with high mortality, may or may not be associated with triggers such as infection. For Idiopathic PF (IPF), the most common form of PF, overall mortality is high with median survival of 3 years from diagnosis (8). Familial PF (FPF) has an established association with telomere maintenance (9) and evidence for a causal role for short telomeres in IPF is also growing (10, 11). Many groups report increased prevalence of herpes virus in IPF, FPF and animal models of PF (12–25) although this is not a universal finding (26–30).
Some herpes viruses appear able to augment other insults to the lung epithelium in causing and exacerbating fibrosis from both the lytic and latent states. In addition to the established role of these viruses in destruction of cells, there appear to be several potential herpes virus-associated mechanisms for enhancement of telomere shortening in alveolar epithelial cells (AEC). These include: induction of a pro-fibrotic cytokine environment; virally-induced endoplasmic reticulum stress which induces inflammatory signaling via NFκB and AP-1 pathways; enhanced cell proliferation regulated by viral DNA; an influence over telomeric repeat-containing RNA (TERRA) regulation on both maintenance of telomere integrity and degradation of shelterin; and a possible impact on two of the key genes implicated in FPF via the GA binding protein GABP. Given the recognized significance of telomere shortening in IPF, described in more detail below, it would be reasonable to postulate a potential role for herpes viruses in contributing to telomere attrition.
Herpes viruses may have different roles in PF, depending on whether they are latent (DNA viral genome only) or lytic. Various studies have looked at possible roles for herpes viruses in the initiation, maintenance and acute exacerbation of pulmonary fibrosis. The results of studies in humans are summarized in Table 1.
A large meta-analysis of case-control studies showed significant association between herpes virus infection and IPF (14). Presence of herpes viruses CMV, EBV, HHV7, and HHV8 all correlated with the risk of IPF (p = 0.001), although interestingly, not with acute exacerbation (p = 0.988) where there are less data. Not all studies have shown associations between herpes viruses and PF. In particular, the evidence linking acute exacerbations with herpes viruses is limited.
Vergnon et al. were first to suggest a link, showing higher anti-EBV antibody titres and higher prevalence of EBV VCA (viral capsid antigen) IgA in serum of IPF patients compared with controls with PF of known cause (23). Egan and colleagues found EBV lytic phase protein expression (gp340/220 and viral capsid antigen, VCA) on immunohistochemistry of lung biopsies from 14 of 20 patients with IPF, including 9 of 14 who were not on immunosuppressive drugs compared with 2 of 21 control lung biopsies; p = 0.0001 (46). In agreement with earlier studies, Tsukamoto et al. showed EBV latent membrane protein (LMP) by PCR in AEC in 24 of 25 patients with idiopathic and 5 of 5 with systemic sclerosis-associated PF (SSc), compared with 10 of 14 controls. The detection ratio was higher in IPF patients than controls (p = 0.047). Immunohistochemistry showed LMP protein in 9 of 29 patients with IPF, but no immunoreactivity in SSc (with PF) or healthy control biopsies. LMP detection was significantly associated with PF itself and with disease progression (p = 0.022) (16).
A study in 2003 found that lung tissue from 97% of PF patients (8 FPF and 25 IPF) contained PCR-amplifiable DNA from at least one Herpes virus, compared with 36% of controls (p < 0.0001) (15). The dominant virus differed between IPF and FPF (EBV and CMV, respectively). Subsequent work showed CMV seropositivity with patchy CMV RNA detectable by in situ PCR of lung biopsies from 9 of 19 PF patients. CMV RNA detection was focal, affecting endothelial cells and alveolar macrophages (24).
Other studies again showed increased prevalence of serum EBV IgA, possibly implying a mucosal process, and EBV DNA in bronchoalveolar lavage fluid from IPF patients. Sixty percent of 17 PF patients compared with 22% of 50 healthy controls and 24% of 46 controls with other interstitial lung diseases had detectable serum EBV IgA to early, nuclear or capsid antigens (p = 0.01). Three antibody positive PF patients but none of the controls had detectable EBV DNA, confirmed by sequencing (p = 0.02) (25). The following year, Lawson et al. found EBV (LMP1), CMV (immediate, early and late) or HHV8 (K-cyclin) antigens, by immunohistochemistry (39). Herpes virus antigens were co-localized with ER stress markers, in type II alveolar epithelial cells (AEC2) in 15 of 23 PF lungs but not in 10 control lungs (p = 0.0005), implying a functional consequence of infection. Calabrese et al. found CMV, EBV, HHV6, 7 or 8, but not other viruses by PCR in bronchoalveolar lavage in 40% of PF vs. 7.3% of disease control, non-IPF diffuse parenchymal, lung transplant explants (p = 0.0003), and 0% of healthy lungs (32). EBV was the most common, accounting for 60% of IPF-related infections, but was not detected in controls. RNA in situ hybridization detected EBV EBER (Epstein–Barr virus-encoded small RNAs) in AEC in 40% of positive PF cases.
A 2014 investigation found DNA of Herpesvirus saimiri, a virus closely related to HHV8, in the regenerating epithelial cells of 21/21 IPF cases and 0/21 controls (17). The authors detected four proteins known to be encoded in the genome of several γ-herpes viruses (cyclin D, thymidylate synthase, dihydrofolate reductase, and interleukin-17) that were strongly co-expressed in the regenerating epithelial cells of each of the 21 idiopathic pulmonary fibrosis cases and not in controls. Furthermore, they showed that the source of the cyclin D RNA, a key regulator of cell proliferation in active IPF was Herpesvirus saimiri and not human, implying that cell cycle regulation was virally-controlled. Such virally-controlled differences in cell turnover could contribute to fibrosis, perhaps via telomere shortening and premature replicative senescence. However, in the absence of further data, this remains speculative. Although its primary host is the squirrel monkey, up to 7% of humans may be infected with H. saimiri, likely depending on exposure (12). Association of IPF with H. saimiri has not been replicated elsewhere, although, to our knowledge H. saimiri has not been specifically sought, except in one other study which found no herpes virus genetic material in PF patients or controls (20). Of note, squirrel monkeys are permitted as pets in some US states, and it is conceivable that this data represented a locally increased prevalence of H. saimiri infection, with pathological consequences for some of those infected.
Among the negative studies, that of Yin et al. is most compelling: they looked specifically, by RNA next generation sequencing, at viral RNA and found no increase of herpes virus gene transcripts in 28 IPF lung transplant explants from different sources, compared with 20 healthy lungs. However, they did find some evidence of human endogenous retrovirus (HERV)-K, the expression of which is potentiated by EBV. Since only 1 EBV-associated RNA (EBER) was sought it remains possible that this latency-associated gene is only expressed at levels below the limit of detection in infected lung cells (29). A very recent study from Le Hingrat et al. found a similarly high prevalence of herpes viruses in both groups of 19 IPF and 9 control lung samples and no evidence of H. saimiri in either group (30). Likewise earlier studies have failed to show any evidence of EBER RNA by in situ hybridization in PF lungs (26). A study of CMV failed to show difference in seroprevalence or bronchoalveolar lavage copy number between 16 untreated PF patients and 16 healthy volunteers, although leukocyte CMV copy number was higher in PF patients (27). HHV8 and EBV proteins NCL-HHV8-LNA, LMP1, EBV RNA (EBER) by immunohistochemistry and EBV DNA by PCR were not found in IPF lung biopsies in another study (28), and HSV1 and EBV were not detectable by PCR of pan-microarray, generated from bronchoalveolar lavage samples from 40 Japanese or Korean patients with stable IPF (37).
Since patients in the studies discussed above were stable at the time the lung material was obtained, the results cannot address any role for herpes viruses in acute exacerbation, including subclinical exacerbation, which may nevertheless contribute to functional decline. Investigation of acute exacerbation has necessarily been limited by barriers in obtaining suitable material in very sick patients. Acute exacerbations were previously defined as deterioration in the absence of infection or other known factor. The role of infection, often cryptic, in triggering acute decline is now well-accepted but the definition has made it difficult to assess the role of virus in such decline.
In 2010, Huie et al. noted the presence of CMV or HSV by PCR or culture in bronchoalveolar lavage of 4 of 27 patients with IPF and other fibrotic diseases. In the patients who had biopsy material available, no CMV or other viral inclusions were seen, nor was there detectable viraemia, suggesting that the viruses were either commensals, or at least were not exerting any direct cytopathic effect (48).
Wootton et al. investigated bronchoalveolar lavage samples from PF patients undergoing acute exacerbation and found PCR, array or deep sequencing evidence of virus presence in only 19 of 43 cases, of which 3 were herpes viruses (EBV in 2 cases and HSV in 1) (37). Ushiki et al. examined bronchoalveolar lavage fluid from 14 patients with acute exacerbations of interstitial lung disease for CMV by PCR. Seven patients fulfilled the criteria for IPF and the others were classified as idiopathic interstitial pneumonia, but did not fulfill CT criteria for IPF; one of the latter was taking immunosuppressive drugs. Two patients, neither of whom had definite IPF, had detectable CMV (44). Given that the control group here may have had earlier stages of IPF, it is difficult to draw firm conclusions. Weng et al. detected HHV in 15 of 30 nasopharyngeal swabs from subjects experiencing an acute exacerbation of IPF (AE-IPF) vs. four of 30 individuals with stable IPF (47), while Santos et al. detected CMV infection in over a third of histological sections from 37 AE-IPF subjects (43). In a recent review discussing the contribution of infection and the microbiome in acute exacerbations of IPF, Invernizzi and Molyneaux provide a further summary of the studies cited here, linking HHV infection to AE-IPF (49).
Overall epidemiological evidence appears to be in favor of a role for herpes viruses, at least in stable disease (14), but a lack of data, poorly defined control groups and the exclusion of those with overt infection makes it difficult to draw any conclusion on the role of herpes viruses in acute exacerbations (14). Apparent inconsistencies between studies, particularly in the prevalence and types of herpes virus are troubling. These differences may have arisen because of the patchy nature of herpes virus infection, as a result of different handling of the technical approaches (50), differences in and limited range of the herpes virus DNA, RNA, proteins, or serology assessed and/or major differences in patient/control location, clinical status and material. Ethnicity may also influence the prevalence of exacerbations (51) and since host genetics influences the immune response to herpesviruses [e.g., (52)] and most of the genetic variation is ethnic and population specific, these influences also need to be controlled. Newer next generation techniques, such as used by Yin et al., are likely to be more sensitive than older methods. Development of less invasive techniques with methods that sample a wider lung region and larger patient numbers, and conversely analytical techniques that provide cell-level data, but require invasive sampling, will be important in resolving these conflicting data and answering the question as to whether herpes viruses are important in IPF. Collaborative initiatives such as the IPF cell atlas maximize the benefit of these difficult-to-obtain data (53).
An extensive study of asymptomatic relatives of patients with FPF showed that patients with PF had increased prevalence of herpes virus DNA in cell-free bronchoalveolar lavage fluid and evidence of herpes virus antigen expression in AEC (18); asymptomatic relatives also had elevated herpes virus DNA levels, overlapping with control and PF groups. These data suggest that herpes virus infection may precede onset of symptomatic pulmonary fibrosis. Alternatively, if PF patients were more permissive to herpes virus infection they might be more likely to infect relatives. Longitudinal data are required to address this question.
In contrast, observations in other animals provide more compelling evidence of a role for herpes virus in PF (19, 21, 54). The results of studies in animals are summarized in Table 2. Spontaneously acquired herpes virus is associated with PF in a variety of domestic mammals (20). Experimental infection of free living, outbred horses with a native horse herpes virus induced pulmonary fibrosis in 3 of 5 horses infected, compared with neither of 2 sham infection control horses (21). Other studies found that gammaHV-68 induced fibrosis in aged mice (55, 56), in STING gain-of-function mice (61) and in mice subject to sub-fibrotic bleomycin challenge (19). Mice with established PF experienced histological and clinical exacerbation when challenged with herpes virus (57, 58, 62). All of these studies establish mechanistic links between herpes virus infection of the lung and the subsequent development of PF.
Respiratory viruses can cause damage via direct cytopathic effects, by potentiation of secondary bacterial infections or via associated inflammation (12, 63). Presence of detectable infection is associated with higher short-term mortality in patients with critical illness (64–66). Lytic herpes viruses are thought to cause pulmonary fibrosis in baboons and cats (54, 67). Mouse models have shown alveolar damage and PF exacerbation in the presence of lytic γHV (19, 57, 58, 61). Herpes viruses have cytopathic effects when in the lytic/reactivation phase (68–70) and EBV is known to replicate in type 2 alveolar cells of PF patients (46). While single, mild or infrequent pulmonary infection typically heals without scarring, severe or persistent infection is often associated with aberrant healing and structural damage, including fibrosis. EBV virus can produce Zta protein in the lung, which enhances production of MMP9, damaging alveoli and allowing increased penetration of inflammatory cells (71). Increased prevalence of persistent viral infection and age-related waning of immunity might therefore predispose to more fibrogenic outcomes after viral infection, consistent with the observed increasing incidence of PF in older people. However, not all viruses are fibrogenic and some may even be protective: respiratory syncytial virus (RSV) can protect mice from vanadium pentoxide-induced fibrosis (72).
The microbiome is increasingly recognized as an important modulator of disease, including in pulmonary fibrosis (12, 73, 74) - although data concerning the pulmonary virome is lacking, as is understanding of the mechanisms whereby the microbiome exerts its influence. However, the recognition that the lung is not a sterile environment and that extensive cross-talk exists between lung, gut and other organs opens up a whole area of fruitful enquiry [e.g., (75)].
Direct herpes virus infection cannot be the only factor in induction of PF. Herpes viruses are common, whereas pulmonary fibrosis is not, implying an essential role for other genetic or environmental factors in its aetiology. Moreover, animal models suggest that latent herpes virus can induce PF with appropriate genetic or environmental background. Murine γHV-68, a γ-herpes virus analogous to EBV, H. saimiri or HHV8 (76), given to mice between 14 and 70 days prior to an otherwise subclinical fibrotic insult, causes fibrosis and increased collagen deposition (59). This work expanded on the earlier studies by Lok et al. who noted exacerbation of fibrosis in bleomycin-resistant mice infected with γHV68 7 days prior to bleomycin challenge. Whereas, Lok hypothesized that lytic herpes-virus infection was likely to be responsible, other work demonstrated induction of PF even after resolution of lytic infection, or when a modified γHV-68 incapable of lytic infection was used (19, 60). Subsequent studies showed that mice experienced increased fibrotic response even if the herpes challenge was administered after the fibrotic insult, a finding of potential importance to the human situation, where fibrotic insults, such as smoking or environmental pollution may well-precede the herpes virus infection (60).
AEC2, alveolar macrophages, neutrophils, fibroblasts and T cells all contribute to the fibrogenic environment in PF. Repeated environmental alveolar micro-injury, in the context of a Th2 (IL4, IL13) or Th17 (IL6, TGFβ)-polarized lymphocyte response can induce epithelial-mesenchymal transition of AEC2, their differentiation to myofibroblasts, activation of a type 2 cytokine-mediated immune response, profibrotic macrophages, fibroblast-myofibroblast transformation and neutrophil influx, in a complex manner which incorporates several paracrine amplification loops. Extracellular matrix, such as collagen, hyaluran or fibronectin, laid down by myofibroblasts causes architectural distortion and impaired gas transfer of clinical pulmonary fibrosis, and will itself contribute to fibrotic progression (77, 78). TGFβ is a key cytokine in induction of fibrosis and is itself found at higher levels in the presence of herpes virus. Increased TGFβ expression has been detected in alveolar macrophages and metaplastic AEC of human PF lung tissue, including in association with herpes viruses (32). Vascular endothelial growth factor (VEGF) may also play a role. VEGF is expressed by hypoxic cells, including alveolar epithelia, macrophages, lymphocytes and fibroblasts. VEGF is overexpressed in PF, with reports suggesting both damaging and protective/compensatory roles for differentially spliced isoforms (79).
Chemokines, particularly CCL2 (MCP1) mRNA and protein, as well as platelet-derived growth factor (PDGF), are expressed by alveolar epithelium in IPF but not normal human lung biopsies (80). CCL2 attracts fibroblasts and “alternatively-activated” M2 macrophages, which promote fibrosis via secretion of cytokines, particularly TGFβ (77, 81, 82). Only one human study has sought to link herpes viruses to potentially profibrotic cytokines. H. saimiri is known to promote expression of human IL-17. Folcik et al. demonstrated co-expression of H. Saimiri and IL-17 in regenerating AEC2 from human PF lung biopsies (17).
Animal studies demonstrate association of herpes viruses with a pro-fibrotic immunological environment. γHV-68 infection of Th2-biased mice increases secretion of TGFβ and cysteinyl leukotriene synthesis in AEC2 (59), hyperplasia of AEC2, increased myofibroblast transformation, higher levels of MMP7 and increased collagen deposition, with decline in forced vital capacity and airway remodeling (83), all features typical of PF. As well as its direct role in pulmonary fibrosis, TGFβ has multiple other effects, including an immunosuppressive, tolerogenic role, and is associated with increased susceptibility to γHV in mouse models (84), thus creating a potentially deleterious feedback loop between local herpes virus infection and TGFβ production.
Another study showed that aged, but not younger mice developed γHV-associated pulmonary fibrosis, with TGFβ production by alveolar macrophages and AEC2 and increased sensitivity of fibrocytes to TGFβ, despite similar levels of viral DNA and Th1/Th2 cytokine ratio (55). Use of aged mice may be particularly relevant to the observed human situation, where herpes virus infections are contracted throughout life, but pulmonary fibrosis is largely a disease of the elderly.
In a Th2-biased (IFNγ knockout) mouse model, PF was initiated by γ-herpes virus infection and was associated with high levels of lung VEGF, which were reduced when lytic infection was controlled or prevented by infection with lytic-phase-incompetent virus (81).
Alveolar macrophages and other immune cells in γHV-68-infected mice also produce chemokines such as CCL3, 4 & 5 (85), and more importantly CCL12, even after resolution of lytic phase. CCL12 has high homology with human MCP1/CCL2, known to be profibrotic (59). Thus, the available evidence implies that lytic herpes virus infection is not always required for initiation of PF. Latent infection is also associated with AEC2 production of cysteinyl leukotrienes, TNF and TGFβ. Leukotriene D4 increases TGFβ production in human lung epithelial cells, attracts profibrotic monocytes and neutrophils, and can cause fibrocyte/blast proliferation, with increased collagen production (86).
Two mouse studies demonstrate relevance of γHV as just one of multiple cofactors in the development of PF. Latent γHV infection post allogeneic transplant in a Th1-biased mouse model was associated with pneumonitis and pulmonary fibrosis, despite similar γHV levels in transplanted and untransplanted control mice, who did not develop PF. The PF was associated with increased TGFβ of non-lymphocyte, presumed lung, origin. Alternatively, activated macrophages, with a profibrotic, TGFβ-secreting phenotype were prominent (84). Severity of PF correlated with previous levels of lytic virus, implying that prior structural damage caused during the lytic phase might be important (84). Cook et al. showed that pulmonary CMV reactivation was universal when Th2-biased mice were subject to sepsis induced by caecal puncture. CMV reactivation under these circumstances was associated with increased and prolonged secretion of TNFα, IL1β, neutrophil chemokine KC and MIP2/CCL4 with collagen deposition and pulmonary fibrosis, which did not occur in uninfected mice (87). A requirement for coexistence of multiple profibrogenic factors would be consistent with the development of pulmonary fibrosis in only a fraction of those infected with herpes viruses.
Acute PF exacerbations may require different cytokine stimuli: a mouse model of γHV PF (57) demonstrated that acute lytic γHV induced pulmonary fibrosis. PF is usually associated with a Th2 environment, but this model demonstrated that herpes virus infections could produce fibrosis and acute clinical decline, reminiscent of acute PF exacerbation, even in Th1-biased, and Th2-knockout (IL4/IL13−/−) mice. As well as increased AEC and macrophage CCL2/12 production, there were increased levels of the type I cytokines, IFNγ and TNFα. Type 1 cytokines are usually considered protective against PF, but, as illustrated by this model, may be associated with acute alveolar damage and be deleterious early in the course of an exacerbation, particularly since TNFα can induce production of profibrotic cytokines such as TGFβ and PDGF (88). Of note, a double-blind randomized clinical trial of etanercept, an anti-TNF agent, did not show significant differences in progression in patients with PF after 48 weeks (89).
Lytic, but not latent γHV infection also resulted in pulmonary fibrosis in Th1-biased, STING gain of function mice. STING mutations are associated with increased production of type 1 antiviral cytokines, IFNγ and IFNβ, and in humans are associated with SAVI, an autoinflammatory disorder, known to be triggered by viral infection. This work further hints at a possible role of Th1 cytokines as mediators in initiation of acute virally-induced PF exacerbations (61). Th17 pathways were implicated in another mouse bleomycin model of acute exacerbation, where intra-tracheal HSV1 was found to induce ER stress and acute lung injury via an IL17-dependent pathway. HSV1-exposed mice showed high mortality compared with saline-challenged mice (62).
A role in exacerbations may be γHV-specific, since CMV, a betaherpes virus, and non-herpes viruses influenza, MAV-1 or the bacterium Pseudomonas were not associated with exacerbation in another mouse model (58).
Thus, with respect to modulation of cytokines, there exists a body of evidence suggesting that the presence of lytic or latent herpes viruses can play a range of key roles in creating a cell signaling environment conducive to the development of fibrosis from prior or subsequent lung injury.
Herpes virus infection may exacerbate other PF-associated pathologies. Pulmonary hypertension is common in PF, affecting up to 40% of patients (90) and associated with worse outcomes (91). Gamma herpes viruses are vasculotropic and there is some evidence of their direct involvement in pulmonary hypertension (32). Herpes virus, particularly EBV, in explant lungs of patients with PF undergoing transplantation was associated with vascular remodeling. There was increased TGFβ expression and arterial intimal thickening, compared with disease and healthy control lungs, along with higher mean pulmonary arterial pressure and worse clinical outcomes (32).
Alveolar injury results in AEC2 proliferation and increased expression of telomerase activity in healthy lung (92). Telomeres are non-protein-coding nucleotide sequences at the end of each chromosome, stabilized by their looped tertiary structure and telomere-related protein complexes (“shelterins”). With each cell division, telomere length becomes progressively shorter, until a critical length is reached whereby the host cell becomes apoptotic, senescent or potentially undergoes malignant transformation. Telomerase lengthens the telomeres and can mitigate, but not prevent, proliferation-associated telomere shortening. Thus, aged mammals tend to have shorter telomeres than young mammals, particularly in proliferating cells (93). Senescence results in dysfunctional but apoptosis-resistant cells, with a TGFβ-dominated, profibrotic cytokine profile (94). Defects of telomere repair are associated with cellular senescence and pulmonary fibrosis in humans (93, 95) and pulmonary fibrosis is predominantly a disorder of older people (96).
In families with multiple cases of PF the majority of causal variants found to date, occur in genes involved in telomere maintenance, e.g., (97). Recent evidence also supports a causal role for prematurely short telomeres in idiopathic PF. One study showed very short telomere lengths, similar in length to those found in patients with familial PF, in biopsies from the lungs of 15 of 28 IPF patients (10) and our team reported results inferring a causal effect of short telomeres in IPF using the statistical method of Mendelian randomization in large cohorts with genetic data (11). Studies in mouse models have shown that it is the AEC2 that are key to the short telomeric cause of lung remodeling and fibrosis (98) with dysfunctional chromosomal replication leading to cellular senescence and triggering the pro-fibrotic senescence-associated secretory phenotype (SASP) (99).
Herpes virus infection alone can induce pulmonary fibrosis in aged but not in young mice (which have very long telomeres), associated with increased CXCL12, a fibrocyte-attracting chemokine, and TGFβ expression (55, 56) suggesting the possibility of telomere-related senescence.
A study of 400 healthy individuals over 3 years reported a correlation between seropositivity for herpes virus infections and significant telomere attrition (with seropositivity to more herpes viruses being additively associated with greater leukocyte telomere length attrition), suggesting exposure to such infectious agents should be an important consideration in studies of telomere dynamics (100). A very recent study of 1,708 individuals aged 20–49 showed shorter telomeres in a small subgroup (3%) with latent pathogen burden characterized by high probabilities of infection with HSV1, CMV and H. pylori (101).
Latent herpes virus infection may impact telomere length by various mechanisms. In human diploid fibroblasts, HSV1 rearranges telomeres, is able to induce transcription of TERRA, a non-coding RNA essential for telomere homeostasis and degrades TPP1, a component of shelterin, a protein complex critical for stabilizing telomeres. Increased TERRA dampens the DNA damage response, and together with the enhanced telomere loss associated with TPP1 reduction, promotes cellular senescence, rather than apoptosis of aged cells, facilitating further HSV1 replication (102).
Human herpes virus 6A and 6B, and possibly human herpes virus 7 can integrate into telomeres, enabling somatic and sometimes vertical transmission (50). Indeed, evidence suggests that components of the shelterin complex such as TRF1 and TRF2 are recruited by the viral genome to aid chromosomal integration (103) and optical mapping highlights the way in which the viral genome hijacks and mimics the cellular telomere maintenance mechanisms (104). The presence of a large foreign genome may well interfere with telomere function, risking profibrotic cellular consequences such as senescence. Reactivation of latent telomere-integrated HHV6, is postulated to occur by one of several excision mechanisms, each of which result in loss of telomere sequence, and can therefore in some cases result in cellular telomere failure (105). In the event of a systemic trigger for HHV-6 reactivation, such as intercurrent infection, reactivation from multiple cells could trigger a clinically relevant telomere failure, perhaps resulting in acute exacerbation of pulmonary fibrosis. HHV6 reactivation itself could provide or exacerbate such a stimulus. Presence of genetic variants in helicases or shelterin components could amplify the deleterious effect of HHV-6 excision (105) and contribute to the molecular and clinical phenotypes observed in some telomere biology disorders (95).
Stem cell transplant is known to be associated with reactivation of chromosomally-integrated HHV6, e.g., (106). People with telomere biology disorders have a high prevalence of pulmonary fibrosis and other fibrotic disease after stem cell transplantation. While there are several possible mechanisms for this observation, it is feasible that reactivation of chromosomally-integrated viruses might be one factor in exacerbating the fibrotic process. Wood and Royle highlight the importance of longitudinal studies of HHV6 in those undergoing stem cell transplantation in order to address this question (105).
Cellular competition for transcription factor binding can be impacted by herpes viruses, which could decrease telomere length by “micro-competition.” After establishing a latent infection, the viral N-box (a core binding sequence contained by many viruses in their promoter /enhancer regions), binds the cellular transcription factor GA binding protein (GABP) p300 transcription complex, causing production of abnormal levels of cellular proteins. Viruses with strong N-boxes in their promoters/enhancers include herpes viruses EBV, CMV, HSV, VZV, Hepatitis B & C (HBV, HCV) and Human Papillomavirus (HPV). CMV has the strongest promoter/enhancer of these. The micro-competition theory suggests that a latent CMV binds the host GABP-binding N box, decreasing the availability of GABP to the promoter of TERF2, which encodes a shelterin protein, essential for telomere maintenance (107). Herpes virus infection-associated proliferation will further accelerate telomere loss leading to death or lack of function in AEC2 with consequent failure to replace the lost AEC1 responsible for gas exchange (56, 83). Gene set enrichment analysis of fibroblasts from rapidly progressing IPF lung compared with controls also showed that the most significantly down-regulated pathways included checkpoint signaling, DNA replication and telomere maintenance (108).
To summarize for ageing, telomere attrition and cellular senescence, there is growing evidence for the causal role of compromised telomere maintenance or short telomeres in PF pathogenesis, certainly in a substantial subset of patients. Here we present several reports of herpes virus interactions with cellular DNA that could also affect telomere maintenance.
Ageing individuals have higher levels of endoplasmic reticular (ER) stress. Increased ER stress and apoptotic markers are evident in explants of idiopathic PF patients, perhaps related to generically shorter telomeres, higher cellular senescence and higher TGFβ levels (109, 110). CMV can activate the unfolded protein response in cultured human fibroblasts, modifying ER stress proteins to favor viral persistence (111). ER stress alone is associated with reactivation of herpes virus, raising the possibility of a deleterious feedback loop (112).
Herpes virus antigens co-localize with ER stress markers in AEC2 in IPF lung biopsies as evidenced by markers of the unfolded protein response in the AEC (39) and correspond with presence of EBV or CMV DNA in samples or AEC2 from at-risk first degree relatives of PF patients, suggesting that herpes virus infection and ER stress predate overt lung fibrosis in many cases (18).
Aged, but not young, Th1-biased mice infected with γHV developed prolonged pulmonary inflammation and PF, compared with young mice who showed resolution without long-term fibrosis. Aged mice showed marked increase of ER stress markers and apoptosis of AEC2, prior to onset of fibrosis, despite controlling herpes virus to the same extent as young mice (56). Thus, again, herpes viruses can impact a characteristic that is associated with the development and progression of fibrosis, including PF (113).
In summary, there appear to be several plausible mechanisms by which herpes viruses could also enable or promote lung fibrosis via ER stress.
Several antiviral drugs with activity against lytic herpes viruses are currently available (Val)acyclovir and (val)ganciclovir are widely available, and well-tolerated even in long-term use for treatment and prophylaxis of herpes simplex, zoster, and cytomegalovirus disease, respectively (114, 115). Foscarnet and Cidofovir are alternatives for resistant viruses, limited by toxicity and the need for parenteral administration (116, 117). After preferential conversion by viral thymidine kinases, these drugs selectively inhibit viral DNA polymerases and have broad spectrum anti-herpes virus activity. Both lytic and latent herpes virus may be important in progression of PF. Antivirals target only lytic virus, but in doing so, indirectly reduce levels of latent virus by reducing ongoing infection of susceptible cells (81).
Despite our inability to cure latent herpes virus, or to prevent infection by vaccination, several animal studies have demonstrated clinical benefit from antivirals. Cidofovir administered to γ-herpes virus-infected IFNγR deficient (Th2-biased) mice, reduced the detection of herpes-related protein in lung and spleen, prevented progression of fibrosis and loss of lung function, and reduced production of TGFβ, inflammatory cytokines IFNγ, TNFα, and Th2 cytokines IL5 and IL13 (81). Treatment given early could prevent onset of pulmonary symptoms, but was beneficial in reducing mortality even after onset of symptomatic pulmonary fibrosis, although established fibrosis was not reversed. Interestingly, the associated rise in VEGF levels was controlled by early but not by late treatment. Cidofovir showed no benefit when PF was induced by bleomycin rather than by viral infection (81). Cidofovir was also effective in blocking CMV reactivation, attenuating the TNFα response and preventing PF in a caecal-puncture sepsis model (87).
Evidence of clinical benefit has also been demonstrated in humans. A patient with pulmonary fibrosis and a family history of rapid progression experienced clearance of pulmonary EBV expression, as measured by sputum PCR viral load and stabilization of lung function over 9 months, while on valaciclovir. A second patient treated with valaciclovir experienced clinical deterioration. However, this patient continued to have detectable sputum HHV7, despite EBV clearance (15).
In a small open-label study of 2 weeks' intravenous ganciclovir, 9 of 14 patients were classified as “responders” with improvement in a composite outcome of FVC, DTPA clearance, shuttle-walk test and reduction in prednisolone dose. Ganciclovir was well-tolerated without significant adverse events. Of those treated, 3 were alive without transplant, 2 had died and 3 had had lung transplant at 12 months follow up, compared with “non-responders” all of whom had died (3) or were post-transplant (2 subjects) (118).
Interferon gamma also has antiviral properties. In human PF and normal lung fibroblast cultures, IFNγ downregulates proliferation and differentiation to myofibroblasts in response to TGFβ and to act in a synergistic manner with pirfenidone (110). Although studies of subcutaneous IFN in established PF showed no benefit, a pilot study of inhaled IFN showed sustained reversal of DLCO (diffusing capacity of the lung for carbon monoxide) decline when administered to PF patients over a period of 80 weeks (119).
Applying learning from mouse models to treatment of patients, the anti-fibrotic effect of inhibition of TGFβ-ALK5 signaling in bleomycin-induced pulmonary fibrosis in mice is attenuated in the presence of concurrent γ-herpes virus infection, suggesting that herpes virus control may be necessary in order to optimize benefit from licensed antifibrotic drugs such as Pirfenidone. Pirfenidone may act by inhibiting TGFβ, probably indirectly, and, in the absence of pharmacological virus control, could potentially enhance herpes virus burden via the immunosuppressive effect of TGFβ (120).
Given the high community prevalence of herpes virus infections, the significance of current diagnostic tests, whether serological, antigen-based or histological, in a patient with PF, is largely unknown.
Better identification of biomarkers for relevant herpes virus infection may enable targeting of treatment to those most likely to benefit, and along with other biomarker-targeted treatment could enable truly personalized treatments. Animal studies clearly show that herpes virus-associated changes occur prior to onset of clinical features and in some cases that early treatment provides more greater benefit than later (81). For this reason, it will be all the more important to identify and diagnose people in the early stages of PF and to conduct trials at this time, rather than at symptomatic presentation, which is likely to represent a more treatment-resistant “end-stage” situation (11, 121, 122).
Pulmonary fibrosis is an end-stage manifestation of many different and interacting processes. Although human and animal data suggests that herpes viruses are implicated in initiation, progression and exacerbation of pulmonary fibrosis, other studies suggest no such effect. Direct alveolar epithelial damage, induction of a profibrotic immunological environment, endoplasmic reticular stress, telomere attrition and cellular senescence are consequences of herpes virus infection, which, in concert with other environmental and genetic factors could increase the risk of pulmonary fibrosis. Techniques such as single cell RNA sequencing will provide important answers as to whether herpes viruses are implicated in pulmonary fibrosis. Cell specific data are required to understand the mechanisms by which herpes viruses could induce, maintain or exacerbate the fibrotic process. If herpes viruses are indeed relevant to pulmonary fibrosis, anti-herpes virus treatments could play a part in modifying or slowing pulmonary fibrosis progression, especially when used at an early stage.
The original contributions presented in the study are included in the article/supplementary material, further inquiries can be directed to the corresponding author/s.
AD conceived the paper and together with HL wrote the first draft. CS and JP reviewed the manuscript and contributed new sections. AD and JP compiled the tables. All authors contributed to the literature review and approve the final version.
AD was funded by the GW4 BioMed MRC Doctoral Training Partnership.
The authors declare that the research was conducted in the absence of any commercial or financial relationships that could be construed as a potential conflict of interest.
1. Chayavichitsilp P, Buckwalter JV, Krakowski AC, Friedlander SF. Herpes simplex. Pediatri Rev. (2009) 30:119–29. doi: 10.1542/pir.30-4-119
2. Epstein MA. Aspects of the EB virus. Adv Cancer Res. (1970) 13:383–411. doi: 10.1016/S0065-230X(08)60169-4
3. Zhang LJ, Hanff P, Rutherford C, Churchill WH, Crumpacker CS. Detection of human cytomegalovirus DNA, RNA, and antibody in normal donor blood. J Infect Dis. (1995) 171:1002–6. doi: 10.1093/infdis/171.4.1002
4. Borie R, Cadranel J, Guihot A, Marcelin AG, Galicier L, Couderc L-J. Pulmonary manifestations of human herpesvirus-8 during HIV infection. Eur Respir J. (2013) 42:1105–18. doi: 10.1183/09031936.00154212
5. Louten J. Herpesviruses. In: Louten J, editor. Essential Human Virology. Boston, MA: Academic Press (2016). p. 235–56. doi: 10.1016/B978-0-12-800947-5.00013-2
6. Morissette G, Flamand L. Herpesviruses and chromosomal integration. J Virol. (2010) 84:12100–9. doi: 10.1128/JVI.01169-10
7. Huang Y, Hidalgo-Bravo A, Zhang E, Cotton VE, Mendez-Bermudez A, Wig G, et al. Human telomeres that carry an integrated copy of human herpesvirus 6 are often short and unstable, facilitating release of the viral genome from the chromosome. Nucleic Acids Res. (2014) 42:315–27. doi: 10.1093/nar/gkt840
8. Ley B, Collard HR, King TE. Clinical course and prediction of survival in idiopathic pulmonary fibrosis. Am J Respir Crit Care Med. (2011) 183:431–40. doi: 10.1164/rccm.201006-0894CI
9. Armanios MY, Chen JJ, Cogan JD, Alder JK, Ingersoll RG, Markin C, et al. Telomerase mutations in families with idiopathic pulmonary fibrosis. N Engl J Med. (2007) 356:1317–26. doi: 10.1056/NEJMoa066157
10. van Batenburg AA, Kazemier KM, van Oosterhout MFM, van der Vis JJ, van Es HW, Grutters JC, et al. From organ to cell: Multi-level telomere length assessment in patients with idiopathic pulmonary fibrosis. PLoS ONE. (2020) 15:e0226785. doi: 10.1371/journal.pone.0226785
11. Duckworth A, Gibbons MA, Allen RJ, Almond H, Beaumont RN, Wood AR, et al. Telomere length and risk of idiopathic pulmonary fibrosis and chronic obstructive pulmonary disease: a mendelian randomisation study. Lancet Respir Med. (2020). doi: 10.1101/2020.02.05.20019653
12. Moore BB, Moore TA. Viruses in idiopathic pulmonary fibrosis. Etiology and exacerbation. Ann Am Thorac Soc. (2015) 2(Suppl. 12):S186–92. doi: 10.1513/AnnalsATS.201502-088AW
13. Azadeh N, Limper AH, Carmona EM, Ryu JH. The role of infection in interstitial lung diseases: a review. Chest. (2017) 152:842–52. doi: 10.1016/j.chest.2017.03.033
14. Sheng G, Chen P, Wei Y, Yue H, Chu J, Zhao J, et al. Viral infection increases the risk of idiopathic pulmonary fibrosis: a meta-analysis. Chest. (2019) 157:1175–87. doi: 10.1016/j.chest.2019.10.032
15. Tang Y-W, Johnson JE, Browning PJ, Cruz-Gervis RA, Davis A, Graham BS, Loyd JE, Stecenko AA, et al. Herpesvirus DNA is consistently detected in lungs of patients with idiopathic pulmonary fibrosis. J Clin Microbiol. (2003) 41:2633–40. doi: 10.1128/JCM.41.6.2633-2640.2003
16. Tsukamoto K, Hayakawa H, Sato A, Chida K, Nakamura H, Miura K. Involvement of Epstein-Barr virus latent membrane protein 1 in disease progression in patients with idiopathic pulmonary fibrosis. Thorax. (2000) 55:958–61. doi: 10.1136/thorax.55.11.958
17. Folcik VA, Garofalo M, Coleman J, Donegan JJ, Rabbani E, Suster S, et al. Idiopathic pulmonary fibrosis is strongly associated with productive infection by herpesvirus saimiri. Mod Pathol. (2014) 27:851–62. doi: 10.1038/modpathol.2013.198
18. Kropski JA, Pritchett JM, Zoz DF, Crossno PF, Markin C, Garnett ET, et al. Extensive phenotyping of individuals at risk for familial interstitial pneumonia reveals clues to the pathogenesis of interstitial lung disease. Am J Respir Crit Care Med. (2015) 191:417–26. doi: 10.1164/rccm.201406-1162OC
19. Lok SS, Haider Y, Howell D, Stewart JP, Hasleton PS, Egan JJ. Murine gammaherpes virus as a cofactor in the development of pulmonary fibrosis in bleomycin resistant mice. Eur Respir J. (2002) 20:1228–32. doi: 10.1183/09031936.02.00272902
20. Williams KJ. Gammaherpesviruses and pulmonary fibrosis: evidence from humans, horses, and rodents. Vet Pathol. (2014) 51:372–84. doi: 10.1177/0300985814521838
21. Williams KJ, Robinson NE, Lim A, Brandenberger C, Maes R, Behan A, et al. Experimental induction of pulmonary fibrosis in horses with the gammaherpesvirus equine herpesvirus 5. PLoS ONE. (2013) 8:e77754. doi: 10.1371/journal.pone.0077754
22. Yonemaru M, Kasuga I, Kusumoto H, Kunisawa A, Kiyokawa H, Kuwabara S, et al. Elevation of antibodies to cytomegalovirus and other herpes viruses in pulmonary fibrosis. Eur Respir J. (1997) 10:2040–5. doi: 10.1183/09031936.97.10092040
23. Vergnon JM, Vincent M, de Thé G, Mornex JF, Weynants P, Brune J. Cryptogenic fibrosing alveolitis and Epstein-Barr virus: an association? Lancet. (1984) 2:768–71. doi: 10.1016/S0140-6736(84)90702-5
24. Magro CM, Allen J, Pope-Harman A, Waldman WJ, Moh P, Rothrauff S, et al. The role of microvascular injury in the evolution of idiopathic pulmonary fibrosis. Am J Clin Pathol. (2003) 119:556–67. doi: 10.1309/0B06Y93EGE6TQ36Y
25. Manika K, Alexiou-Daniel S, Papakosta D, Papa A, Kontakiotis T, Patakas D, et al. Epstein-Barr virus DNA in bronchoalveolar lavage fluid from patients with idiopathic pulmonary fibrosis. Sarcoid Vasculi Diffuse Lung Dis. (2007) 24:134–40.
26. Wangoo A, Shaw RJ, Diss TC, Farrell PJ, du Bois RM, Nicholson AG. Cryptogenic fibrosing alveolitis: lack of association with Epstein-Barr virus infection. Thorax. (1997) 52:888–91. doi: 10.1136/thx.52.10.888
27. Dworniczak S, Ziora D, Kapral M, Mazurek U, Niepsuj G, Rauer R, et al. Human cytomegalovirus DNA level in patients with idiopathic pulmonary fibrosis. J Physiol Pharmacol. (2004) 3(Suppl. 55):67–75.
28. Zamò A, Poletti V, Reghellin D, Montagna L, Pedron S, Piccoli P, et al. HHV-8 and EBV are not commonly found in idiopathic pulmonary fibrosis. Sarcoid Vasculi Diffuse Lung Dis. (2005) 22:123–8.
29. Yin Q, Strong MJ, Zhuang Y, Flemington EK, Kaminski N, de Andrade JA, Lasky JA. Assessment of viral RNA in idiopathic pulmonary fibrosis using RNA-seq. BMC Pulmonary Med. (2020) 20:81. doi: 10.1186/s12890-020-1114-1
30. Le Hingrat Q, Ghanem M, Cazes A, Visseaux B, Collin G, Descamps D, et al. No association between human herpesvirus or herpesvirus saimiri and idiopathic pulmonary fibrosis. ERJ Open Res. (2020) 6:00243–2020. doi: 10.1183/23120541.00243-2020
31. Lasithiotaki I, Antoniou KM, Vlahava VM, Karagiannis K, Spandidos DA, Siafakas NM, et al. Detection of herpes simplex virus type-1 in patients with fibrotic lung diseases. PLoS ONE. (2011) 6:e27800. doi: 10.1371/journal.pone.0027800
32. Calabrese F, Kipar A, Lunardi F, Balestro E, Perissinotto E, Rossi E, et al. Herpes virus infection is associated with vascular remodeling and pulmonary hypertension in idiopathic pulmonary fibrosis. PLoS ONE. (2013) 8:e55715. doi: 10.1371/journal.pone.0055715
33. Tang YW, Johnson J, Cruz-Gervis R, Graham B, Brigham K, Oates J, et al. Increased detection of herpesvirus DNA in idiopathic pulmonary fibrosis. Chest. (2001) 120(1 Suppl.):74S–5S. doi: 10.1378/chest.120.1_suppl.s74-a
34. Stewart JP, Egan JJ, Ross AJ, Kelly BG, Lok SS, Hasleton PS, et al. The detection of Epstein-Barr virus DNA in lung tissue from patients with idiopathic pulmonary fibrosis. Am J Respir Crit Care Med. (1999) 159(4 Pt 1):1336–41. doi: 10.1164/ajrccm.159.4.9807077
35. Bando M, Takahashi M, Ohno S, Hosono T, Hironaka M, Okamoto H, et al. Torque teno virus DNA titre elevated in idiopathic pulmonary fibrosis with primary lung cancer. Respirology. (2008) 13:263–9. doi: 10.1111/j.1440-1843.2007.01217.x
36. Pozharskaya V, Torres-González E, Rojas M, Gal A, Amin M, Dollard S, et al. Twist: a regulator of epithelial-mesenchymal transition in lung fibrosis. PLoS ONE. (2009) 4:e7559. doi: 10.1371/journal.pone.0007559
37. Wootton SC, Kim DS, Kondoh Y, Chen E, Lee JS, Song JW, et al. Viral infection in acute exacerbation of idiopathic pulmonary fibrosis. Am J Respir Crit Care Med. (2011) 183:1698–702. doi: 10.1164/rccm.201010-1752OC
38. Pulkkinen V, Salmenkivi K, Kinnula VL, Sutinen E, Halme M, Hodgson U, et al. A novel screening method detects herpesviral DNA in the idiopathic pulmonary fibrosis lung. Ann Med. (2012) 44:178–86. doi: 10.3109/07853890.2010.532151
39. Lawson WE, Crossno PF, Polosukhin VV, Roldan J, Cheng DS, Lane KB, et al. Endoplasmic reticulum stress in alveolar epithelial cells is prominent in IPF: association with altered surfactant protein processing and herpesvirus infection. Am J Physiol Lung Cell Mol Physiol. (2008) 294:L1119–26. doi: 10.1152/ajplung.00382.2007
40. Kelly BG, Lok SS, Hasleton PS, Egan JJ, Stewart JP. A rearranged form of Epstein-Barr virus DNA is associated with idiopathic pulmonary fibrosis. Am J Respir Crit Care Med. (2002) 166:510–3. doi: 10.1164/rccm.2103058
41. Miyake Y, Sasaki S, Yokoyama T, Chida K, Azuma A, Suda T, et al. Case-control study of medical history and idiopathic pulmonary fibrosis in Japan. Respirology. (2005) 10:504–9. doi: 10.1111/j.1440-1843.2005.00742.x
42. Rabea AEM, Zidan M, Daabis R, El Sayed P, Samir S. Prevalence of chronic hepatitis C virus (HCV) infection in patients with idiopathic pulmonary fibrosis. Egypt. J. Chest Dis. Tuberculosis. (2015) 64:907–13. doi: 10.1016/j.ejcdt.2015.07.003
43. Santos GC, Parra ER, Stegun FW, Cirqueira CS, Capelozzi VL. Immunohistochemical detection of virus through its nuclear cytopathic effect in idiopathic interstitial pneumonia other than acute exacerbation. Brazil J Med Biol Res. (2013) 46:985–92. doi: 10.1590/1414-431X20132885
44. Ushiki A, Yamazaki Y, Hama M, Yasuo M, Hanaoka M, Kubo K. Viral infections in patients with an acute exacerbation of idiopathic interstitial pneumonia. Respir Investig. (2014) 52:65–70. doi: 10.1016/j.resinv.2013.07.005
45. Saraya T, Kimura H, Kurai D, Tamura M, Ogawa Y, Mikura S, et al. Clinical significance of respiratory virus detection in patients with acute exacerbation of interstitial lung diseases. Respir Med. (2018) 136:88–92. doi: 10.1016/j.rmed.2018.02.003
46. Egan JJ, Stewart JP, Hasleton PS, Arrand JR, Carroll KB, Woodcock AA. Epstein-Barr virus replication within pulmonary epithelial cells in cryptogenic fibrosing alveolitis. Thorax. (1995) 50:1234–9. doi: 10.1136/thx.50.12.1234
47. Weng D, Chen XQ, Qiu H, Zhang Y, Li QH, Zhao MM, et al. The role of infection in acute exacerbation of idiopathic pulmonary fibrosis. Mediators Inflamm. (2019) 2019:5160694. doi: 10.1155/2019/5160694
48. Huie TJ, Olson AL, Cosgrove GP, Janssen WJ, Lara AR, Lynch DA, et al. A detailed evaluation of acute respiratory decline in patients with fibrotic lung disease: aetiology and outcomes. Respirology. (2010) 15:909–17. doi: 10.1111/j.1440-1843.2010.01774.x
49. Invernizzi R, Molyneaux PL. The contribution of infection and the respiratory microbiome in acute exacerbations of idiopathic pulmonary fibrosis. Eur Respir Rev. (2019) 28:190045. doi: 10.1183/16000617.0045-2019
50. Prusty BK, Gulve N, Rasa S, Murovska M, Hernandez PC, Ablashi DV. Possible chromosomal and germline integration of human herpesvirus 7. J Gene Virol. (2017) 98:266–74. doi: 10.1099/jgv.0.000692
51. Leuschner G, Behr J. Acute exacerbation in interstitial lung disease. Front Med. (2017) 4:176. doi: 10.3389/fmed.2017.00176
52. Sezgin E, An P, Winkler CA. Host genetics of cytomegalovirus pathogenesis. Front Genet. (2019) 10:616. doi: 10.3389/fgene.2019.00616
53. Neumark NCC, Rose K-A, Kaminski N. The idiopathic pulmonary fibrosis cell atlas. Am J Physiol Lung Cell Mol Physiol. (2020) 319:L887–92. doi: 10.1152/ajplung.00451.2020
54. Love DN. Feline herpesvirus associated with interstitial pneumonia in a kitten. Vet Rec. (1971) 89:178–81. doi: 10.1136/vr.89.7.178
55. Naik PN, Horowitz JC, Moore TA, Wilke CA, Toews GB, Moore BB. Pulmonary fibrosis induced by γ-herpesvirus in aged mice is associated with increased fibroblast responsiveness to transforming growth factor-β. J Gerontol A Biol Sci Med Sci. (2012) 67:714–25. doi: 10.1093/gerona/glr211
56. Torres-González E, Bueno M, Tanaka A, Krug LT, Cheng D-S, Polosukhin VV, et al. Role of endoplasmic reticulum stress in age-related susceptibility to lung fibrosis. Am J Respir Cell Mol Biol. (2012) 46:748–56. doi: 10.1165/rcmb.2011-0224OC
57. McMillan TR, Moore BB, Weinberg JB, Vannella KM, Fields WB, Christensen PJ, et al. Exacerbation of established pulmonary fibrosis in a murine model by gammaherpesvirus. Am J Respir Crit Care Med. (2008) 177:771–80. doi: 10.1164/rccm.200708-1184OC
58. Ashley SL, Jegal Y, Moore TA, van Dyk LF, Laouar Y, Moore BB. γ-Herpes virus-68, but not Pseudomonas aeruginosa or influenza A (H1N1), exacerbates established murine lung fibrosis. Am J Physiol Lung Cell Mol Physiol. (2014) 307:L219–30. doi: 10.1152/ajplung.00300.2013
59. Vannella KM, Luckhardt TR, Wilke CA, van Dyk LF, Toews GB, Moore BB. Latent herpesvirus infection augments experimental pulmonary fibrosis. Am J Respir Crit Care Med. (2010) 181:465–77. doi: 10.1164/rccm.200905-0798OC
60. Stoolman JS, Vannella KM, Coomes SM, Wilke CA, Sisson TH, Toews GB, et al. Latent infection by γherpesvirus stimulates profibrotic mediator release from multiple cell types. Am J Physiol Lung Cell Mol Physiol. (2011) 300:L274–85. doi: 10.1152/ajplung.00028.2010
61. Bennion BG, Ingle H, Ai TL, Miner CA, Platt DJ, Smith AM, et al. A human gain-of-function STING mutation causes immunodeficiency and gammaherpesvirus-induced pulmonary fibrosis in mice. J Virol. (2019) 93:e01806–18. doi: 10.1128/JVI.01806-18
62. Chen T, Qiu H, Zhao M-M, Chen S-S, Wu Q, Zhou N-Y, et al. IL-17A contributes to HSV1 infection-induced acute lung injury in a mouse model of pulmonary fibrosis. J Cell Mol Med. (2019) 23:908–19. doi: 10.1111/jcmm.13992
63. Bosch AA, Biesbroek G, Trzcinski K, Sanders EA, Bogaert D. Viral and bacterial interactions in the upper respiratory tract. PLoS Pathog. (2013) 9:e1003057. doi: 10.1371/journal.ppat.1003057
64. Blivet S, Philit F, Sab JM, Langevin B, Paret M, Guérin C, et al. Outcome of patients with idiopathic pulmonary fibrosis admitted to the ICU for respiratory failure. Chest. (2001) 120:209–12. doi: 10.1378/chest.120.1.209
65. Saydain G, Islam A, Afessa B, Ryu JH, Scott JP, Peters SG. Outcome of patients with idiopathic pulmonary fibrosis admitted to the intensive care unit. Am J Respir Crit Care Med. (2002) 166:839–42. doi: 10.1164/rccm.2104038
66. Stern JB, Mal H, Groussard O, Brugière O, Marceau A, Jebrak G, et al. Prognosis of patients with advanced idiopathic pulmonary fibrosis requiring mechanical ventilation for acute respiratory failure. Chest. (2001) 120:213–9. doi: 10.1378/chest.120.1.213
67. Brack M, Eichberg JW, Heberling RL, Kalter SS. [Experimentally induced herpesvirus SA 8-pneumonia (bronchopneumonia) in Kenya baboons (papio cynocephalus) (author's transl)]. Virchows Archiv Pathol Anatomy Histol. (1981) 392:81–96. doi: 10.1007/BF00430551
68. Fonseca Brito L., Brune W, Stahl FR. Cytomegalovirus (CMV) pneumonitis: cell tropism, inflammation, and immunity. Int J Mol Sci. (2019) 20:63865. doi: 10.3390/ijms20163865
69. Temple RM, Zhu J, Budgeon L, Christensen ND, Meyers C, Sample CE. Efficient replication of Epstein-Barr virus in stratified epithelium in vitro. Proc Natl Acad Sci USA. (2014) 111:16544–9. doi: 10.1073/pnas.1400818111
70. Karasneh GA, Shukla D. Herpes simplex virus infects most cell types in vitro: clues to its success. Virol J. (2011) 8:481. doi: 10.1186/1743-422X-8-481
71. Guenther JF, Cameron JE, Nguyen HT, Wang Y, Sullivan DE, Shan B, et al. Modulation of lung inflammation by the Epstein-Barr virus protein Zta. Am J Physiol Lung Cell Mol Physiol. (2010) 299:L771–84. doi: 10.1152/ajplung.00408.2009
72. Turpin EA, Antao-Menezes A, Cesta MF, Mangum JB, Wallace DG, Bermudez E, et al. Respiratory syncytial virus infection reduces lung inflammation and fibrosis in mice exposed to vanadium pentoxide. Respir Res. (2010) 11:20. doi: 10.1186/1465-9921-11-20
73. Invernizzi R, Barnett J, Rawal B, Nair A, Ghai P, Kingston S, et al. Bacterial burden in the lower airways predicts disease progression in idiopathic pulmonary fibrosis and is independent of radiological disease extent. Eur Respir J. (2020) 55:1901519. doi: 10.1183/13993003.01519-2019
74. O'Dwyer DN, Ashley SL, Gurczynski SJ, Xia M, Wilke C, Falkowski NR, et al. Lung microbiota contribute to pulmonary inflammation and disease progression in pulmonary fibrosis. Am J Respir Crit Care Med. (2019) 199:1127–38. doi: 10.1164/rccm.201809-1650OC
75. Anand S, Mande S, Diet S. Microbiota and gut-lung connection. Front Microbiol. (2018) 9:2147. doi: 10.3389/fmicb.2018.02147
76. Efstathiou S, Ho YM, Hall S, Styles CJ, Scott SD, Gompels UA. Murine herpesvirus 68 is genetically related to the gammaherpesviruses Epstein-Barr virus and herpesvirus saimiri. J Gene Virol. (1990) 71:1365–72. doi: 10.1099/0022-1317-71-6-1365
77. Kolahian S, Fernandez IE, Eickelberg O, Hartl D. Immune mechanisms in pulmonary fibrosis. Am J Respir Cell Mol Biol. (2016) 55:309–22. doi: 10.1165/rcmb.2016-0121TR
78. Zhang J, Wang D, Wang L, Wang S, Roden AC, Zhao H, et al. Profibrotic effect of IL-17A and elevated IL-17RA in idiopathic pulmonary fibrosis and rheumatoid arthritis-associated lung disease support a direct role for IL-17A/IL-17RA in human fibrotic interstitial lung disease. Am J Physiol Lung Cell Mol Physiol. (2019) 316:L487–97. doi: 10.1152/ajplung.00301.2018
79. Barratt SL, Flower VA, Pauling JD, Millar AB. VEGF (Vascular Endothelial Growth Factor) and fibrotic lung disease. Int J Mol Sci. (2018) 19:1269. doi: 10.3390/ijms19051269
80. Antoniades HN, Neville-Golden J, Galanopoulos T, Kradin RL, Valente AJ, Graves DT. Expression of monocyte chemoattractant protein 1 mRNA in human idiopathic pulmonary fibrosis. Proc Natl Acad Sci USA. (1992) 89:5371–5. doi: 10.1073/pnas.89.12.5371
81. Mora AL, Torres-González E, Rojas M, Xu J, Ritzenthaler J, Speck SH, et al. Control of virus reactivation arrests pulmonary herpesvirus-induced fibrosis in IFN-gamma receptor-deficient mice. Am J Respir Crit Care Med. (2007) 175:1139–50. doi: 10.1164/rccm.200610-1426OC
82. Murray LA, Argentieri RL, Farrell FX, Bracht M, Sheng H, Whitaker B, et al. Hyper-responsiveness of IPF/UIP fibroblasts: interplay between TGFbeta1, IL-13 and CCL2. Int J Biochem Cell Biol. (2008) 40:2174–82. doi: 10.1016/j.biocel.2008.02.016
83. Mora AL, Woods CR, Garcia A, Xu J, Rojas M, Speck SH, et al. Lung infection with γ-herpesvirus induces progressive pulmonary fibrosis in Th2-biased mice. Am J Physiol Lung Cell Mol Physiol. (2005) 289:L711–21. doi: 10.1152/ajplung.00007.2005
84. Coomes SM, Wilke CA, Moore TA, Moore BB. Induction of TGF-beta 1, not regulatory T cells, impairs antiviral immunity in the lung following bone marrow transplant. J Immunol. (2010) 184:5130–40. doi: 10.4049/jimmunol.0901871
85. Weinberg JB, Lutzke ML, Efstathiou S, Kunkel SL, Rochford R. Elevated chemokine responses are maintained in lungs after clearance of viral infection. J Virol. (2002) 76:10518–23. doi: 10.1128/JVI.76.20.10518-10523.2002
86. Bossé Y, Thompson C, McMahon S, Dubois CM, Stankova J, Rola-Pleszczynski M. Leukotriene D4-induced, epithelial cell-derived transforming growth factor beta1 in human bronchial smooth muscle cell proliferation. Clin Experi Allergy. (2008) 38:113–21. doi: 10.1111/j.1365-2222.2007.02873.x
87. Cook CH, Zhang Y, Sedmak DD, Martin LC, Jewell S, Ferguson RM. Pulmonary cytomegalovirus reactivation causes pathology in immunocompetent mice. Crit Care Med. (2006) 34:842–9. doi: 10.1097/01.CCM.0000201876.11059.05
88. Warshamana GS, Corti M. Brody ATNF-alpha R, PDGF, and TGF-beta(1) expression by primary mouse bronchiolar-alveolar epithelial and mesenchymal cells: tnf-alpha induces TGF-beta(1). Exp Mol Pathol. (2001) 71:13–33. doi: 10.1006/exmp.2001.2376
89. Raghu G, Brown KK, Costabel U, Cottin V. R.Bois Md, Lasky JA, et al. Treatment of idiopathic pulmonary fibrosis with etanercept. Am J Respir Crit Care Med. (2008) 178:948–55. doi: 10.1164/rccm.200709-1446OC
90. Collum SD, Amione-Guerra J, Cruz-Solbes AS, DiFrancesco A, Hernandez AM, Hanmandlu A, et al. Pulmonary hypertension associated with idiopathic pulmonary fibrosis: current and future perspectives. Canad Respir J. (2017) 2017:1430350. doi: 10.1155/2017/1430350
91. Patel NM, Lederer DJ, Borczuk AC, Kawut SM. Pulmonary hypertension in idiopathic pulmonary fibrosis. Chest. (2007) 132:998–1006. doi: 10.1378/chest.06-3087
92. Garcia O, Hiatt MJ, Lundin A, Lee J, Reddy R, Navarro S, et al. Targeted type 2 alveolar cell depletion. A dynamic functional model for lung injury repair. Am J Respir Cell Mol Biol. (2016) 54:319–30. doi: 10.1165/rcmb.2014-0246OC
93. Niewisch MR, Savage SA. An update on the biology and management of dyskeratosis congenita and related telomere biology disorders. Expert Rev Hematol. (2019) 12:1037–52. doi: 10.1080/17474086.2019.1662720
94. Hecker L, Logsdon NJ, Kurundkar D, Kurundkar A, Bernard K, Hock T, et al. Reversal of persistent fibrosis in aging by targeting Nox4-Nrf2 redox imbalance. Sci Transl Med. (2014) 6:231ra47. doi: 10.1126/scitranslmed.3008182
95. Dokal I, Vulliamy T, Mason P, Bessler M. Clinical utility gene card for: Dyskeratosis congenita - update 2015. Eur J Hum Genet. (2015) 23:170. doi: 10.1038/ejhg.2014.170
96. Raghu G, Collard HR, Egan JJ, Martinez FJ, Behr J, Brown KK, et al. An official ATS/ERS/JRS/ALAT statement: idiopathic pulmonary fibrosis: evidence-based guidelines for diagnosis and management. Am J Respir Crit Care Med. (2011) 183:788–824. doi: 10.1164/rccm.2009-040GL
97. Newton CA, Batra K, Torrealba J, Kozlitina J, Glazer CS, Aravena C, et al. Telomere-related lung fibrosis is diagnostically heterogeneous but uniformly progressive. Eur Respir J. (2016) 48:1710–20. doi: 10.1183/13993003.00308-2016
98. Naikawadi RP, Disayabutr S, Mallavia B, Donne ML, Green G, La JL, et al. Telomere dysfunction in alveolar epithelial cells causes lung remodeling and fibrosis. JCI Insight. (2016) 1:e86704. doi: 10.1172/jci.insight.86704
99. Schafer MJ, White TA, Iijima K, Haak AJ, Ligresti G, Atkinson EJ, et al. Cellular senescence mediates fibrotic pulmonary disease. Nat Commun. (2017) 8:14532. doi: 10.1038/ncomms14532
100. Dowd JB, Bosch JA, Steptoe A, Jayabalasingham B, Lin J, Yolken R, et al. Persistent herpesvirus infections and telomere attrition over 3 years in the whitehall ii cohort. J Infect Dis. (2017) 216:565–72. doi: 10.1093/infdis/jix255
101. Noppert GA, Feinstein L, Dowd JB, Stebbins RC, Zang E, Needham BL, et al. Pathogen burden and leukocyte telomere length in the United States. Immun Ageing. (2020) 17:36. doi: 10.1186/s12979-020-00206-9
102. Deng Z, Kim ET, Vladimirova O, Dheekollu J, Wang Z, Newhart A, et al. HSV-1 remodels host telomeres to facilitate viral replication. Cell Rep. (2014) 9:2263–78. doi: 10.1016/j.celrep.2014.11.019
103. Gilbert-Girard S, Gravel A, Collin V, Wight DJ, Kaufer BB, Lazzerini-Denchi E, et al. Role for the shelterin protein TRF2 in human herpesvirus 6A/B chromosomal integration. PLoS Pathog. (2020) 16:e1008496. doi: 10.1371/journal.ppat.1008496
104. Wight DJ, Aimola G, Aswad A, Jill Lai CY, Bahamon C, Hong K, et al. Unbiased optical mapping of telomere-integrated endogenous human herpesvirus 6. Proc Natl Acad Sci USA. (2020) 117:31410–6. doi: 10.1073/pnas.2011872117
105. Wood ML, Royle NJ. Chromosomally integrated human herpesvirus 6: models of viral genome release from the telomere and impacts on human health. Viruses. (2017) 9:184. doi: 10.3390/v9070184
106. Ogata M, Kikuchi H, Satou T, Kawano R, Ikewaki J, Kohno K, et al. Human herpesvirus 6 DNA in plasma after allogeneic stem cell transplantation: incidence and clinical significance. J Infect Dis. (2006) 193:68–79. doi: 10.1086/498531
107. Polansky H, Javaherian A. The latent cytomegalovirus decreases telomere length by microcompetition. Open Med (Wars). (2015) 10:294–6. doi: 10.1515/med-2015-0042
108. Peng R, Sridhar S, Tyagi G, Phillips JE, Garrido R, Harris P, et al. Bleomycin induces molecular changes directly relevant to idiopathic pulmonary fibrosis: a model for “active” disease. PLoS ONE. (2013) 8:e59348–e59348. doi: 10.1371/journal.pone.0059348
109. Korfei M, Ruppert C, Mahavadi P, Henneke I, Markart P, Koch M, et al. Epithelial endoplasmic reticulum stress and apoptosis in sporadic idiopathic pulmonary fibrosis. Am J Respir Crit Care Med. (2008) 178:838–46. doi: 10.1164/rccm.200802-313OC
110. Dickens JA, Malzer E, Chambers JE, Marciniak SJ. Pulmonary endoplasmic reticulum stress-scars, smoke, and suffocation. FEBS J. (2019) 286:322–41. doi: 10.1111/febs.14381
111. Isler JA, Skalet AH, Alwine JC. Human cytomegalovirus infection activates and regulates the unfolded protein response. J Virol. (2005) 79:6890–9. doi: 10.1128/JVI.79.11.6890-6899.2005
112. Zhou X-c, Dong S-h, Liu Z-s, Liu S, Zhang C-c, Liang X-z. Regulation of gammaherpesvirus lytic replication by endoplasmic reticulum stress-induced transcription factors ATF4 CHOP. J Biol Chem. (2018) 293:2801–14. doi: 10.1074/jbc.M117.813675
113. Burman A, Tanjore H, Blackwell TS. Endoplasmic reticulum stress in pulmonary fibrosis. Matrix Biol. (2018) 69:355–65. doi: 10.1016/j.matbio.2018.03.015
118. Egan JJ, Adamali HI, Lok SS, Stewart JP, Woodcock AA. Ganciclovir antiviral therapy in advanced idiopathic pulmonary fibrosis: an open pilot study. Pulm Med. (2011) 2011:240805. doi: 10.1155/2011/240805
119. Skaria SD, Yang J, Condos R, Smaldone GC. Inhaled Interferon and Diffusion Capacity in Idiopathic Pulmonary Fibrosis (IPF). Sarcoid Vascul Diffuse Lung Dis. (2015) 32:37–42. Available online at: https://mattioli1885journals.com/index.php/sarcoidosis/article/view/3516
120. Smoktunowicz N, Alexander RE, Franklin L, Williams AE, Holman B, Mercer PF, et al. The anti-fibrotic effect of inhibition of TGFβ-ALK5 signalling in experimental pulmonary fibrosis in mice is attenuated in the presence of concurrent γ-herpesvirus infection. Dis Models Mech. (2015) 8:1129–39. doi: 10.1242/dmm.019984
121. Salisbury ML, Hewlett JC, Ding G, Markin CR, Douglas K, Mason W, et al. Development and progression of radiologic abnormalities in individuals at risk for familial interstitial lung disease. Am J Respir Crit Care Med. (2020) 201:1230–9. doi: 10.1164/rccm.201909-1834OC
Keywords: pulmonary fibrosis, herpes virus, CMV, EBV, telomeres, senescence, endoplasmic reticulum stress, antiviral treatment
Citation: Duckworth A, Longhurst HJ, Paxton JK and Scotton CJ (2021) The Role of Herpes Viruses in Pulmonary Fibrosis. Front. Med. 8:704222. doi: 10.3389/fmed.2021.704222
Received: 02 May 2021; Accepted: 24 June 2021;
Published: 22 July 2021.
Edited by:
Elena Lopez-Rodriguez, Charité-Universitätsmedizin Berlin, GermanyReviewed by:
Raquel Arroyo, Cincinnati Children's Hospital Medical Center, United StatesCopyright © 2021 Duckworth, Longhurst, Paxton and Scotton. This is an open-access article distributed under the terms of the Creative Commons Attribution License (CC BY). The use, distribution or reproduction in other forums is permitted, provided the original author(s) and the copyright owner(s) are credited and that the original publication in this journal is cited, in accordance with accepted academic practice. No use, distribution or reproduction is permitted which does not comply with these terms.
*Correspondence: Anna Duckworth, YWQ2NTNAZXhldGVyLmFjLnVr
†These authors have contributed equally to this work
Disclaimer: All claims expressed in this article are solely those of the authors and do not necessarily represent those of their affiliated organizations, or those of the publisher, the editors and the reviewers. Any product that may be evaluated in this article or claim that may be made by its manufacturer is not guaranteed or endorsed by the publisher.
Research integrity at Frontiers
Learn more about the work of our research integrity team to safeguard the quality of each article we publish.