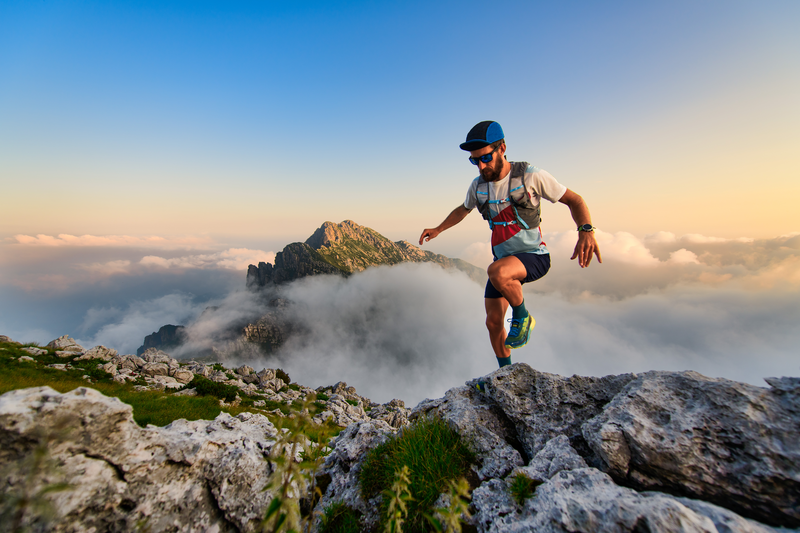
94% of researchers rate our articles as excellent or good
Learn more about the work of our research integrity team to safeguard the quality of each article we publish.
Find out more
METHODS article
Front. Med. , 08 June 2021
Sec. Infectious Diseases – Surveillance, Prevention and Treatment
Volume 8 - 2021 | https://doi.org/10.3389/fmed.2021.685926
This article is part of the Research Topic Infection and Control of Vector-Borne Diseases View all 34 articles
Several methods exist to collect and assess the abundance of dengue vector mosquitoes, i.e., morning adult collection, pupal collection, ovitraps, human landing, and larval collection. Several of these methods are officially implemented to monitor mosquito density and make decisions on treatments for dengue control. This monitoring is also constrained by the need to conduct this assessment on a “one point/one day” process, meaning that once the threshold of 100 households is reached, the assessment is made, and the collectors teams move to another place, thus preventing the use of long-term sampling methods. This diversity of methods might be a source of variability and lack of statistical significance. There is also a lack of published data regarding the efficacy of these methods. Furthermore, the Stegomyia indices are shown to be not reliable for assessing the risk of dengue outbreaks. A mosquito survey was, thus, conducted in 39 locations corresponding to 15 dengue endemic provinces in Indonesia by using the different adult and larval collection methods recommended nationwide. A total of 44,675 mosquitoes were collected. The single larva method was the most efficient. Out of a total of 89 dengue-positive pools, the most frequently encountered virus was DENV2, which made up half of the positive samples, followed by DENV3 and DENV1, respectively. Factor analysis of mixed data showed that no correlation could be found between any methods and the presence of dengue virus in mosquitoes. Moreover, no correlation could be found between any methods and the incidence of dengue. There was no consistency in the efficacy of a given method from one site to another. There was no correlation between any of the parameters considered, i.e., method, incidence of dengue, location, and the presence of dengue virus in mosquitoes.
Dengue is the most rapidly spreading arboviral disease worldwide (1). Recent studies estimate that 55–100 million dengue cases are reported annually with 3.9 billion people at risk (2, 3). Indonesia is an hyperendemic dengue country; i.e., all four serotypes are circulating with the highest number of dengue cases in Southeast Asia (4, 5). Dengue incidence in Indonesia has increased significantly over the last four decades from 0.05/100,000 in 1968 to 78.8/100,000 in 2016 (6). The dengue virus is transmitted to humans by the bite of infected Aedes aegypti mosquitoes, the main vector, and Ae. albopictus, the secondary vector. These species are anthropophilic; i.e., they live in human environments and breed in various sites, such as water containers, flowerpots, birdbaths, disposed water-holding vessels, waste disposal areas, small containers, discarded tires, natural holes in vegetation, etc. (7–10). Both are present in urban and suburban areas. With no treatment and while an effective vaccine is still under study, vector control remains the only effective way to prevent and control dengue.
Vector surveillance methods have remained mostly unchanged for more than three decades (11). Larval survey is the most widely adopted dengue vector surveillance method to locate larval habitats and to measure the abundance of Ae. aegypti and Ae. albopictus (12, 13). The Stegomyia indices, i.e., house index (HI), container index (CI), and Breteau index (BI), to which a specific free larval index (FLI) is added in Indonesia, are used for calculating mosquito abundance and for predicting the risk of dengue transmission (11). The FLI is defined as the number of houses without larva x 100/total number houses. The FLI is, thus, the reverse of the HI. However, previous studies demonstrated the lack of correlation between Stegomyia indices and the risk of dengue outbreak (12, 14–18), although a correlation was found between human population density and incidence of dengue (18). This sounds logical owing to the anthropophilic behavior of these vectoring mosquitoes. Several methods for collecting mosquitoes are officially recommended by the Ministry of Health, i.e., morning adult collection, pupal collection, ovitraps, whole night collection using human landing, and larval collection. Because the Stegomyia indices, exclusively based on larval collection, are not reliable predictors, other predictors must be sought. The diversity of methods endorsed by the Ministry might be a source of variability. Furthermore, there is a lack of published data regarding the effectiveness of these methods (12, 19–22). A major constraint associated with the monitoring of mosquitoes is that the decision for treatment or absence of treatment is made on a one point/one day basis, meaning that agents in charge of the survey issue the assessment conclusion once the threshold of 100 households is reached and move to another place. They do not conduct long-term capture and sampling. Methods of insect collection influence the reliability of entomological indices. We report here a large-scale comparative analysis of various methods of insect collection to assess their relative effectiveness and reliability to determine whether entomological descriptors can be envisioned to determine the risk of dengue outbreak or if other kinds of descriptors should be considered.
The study was conducted in 39 locations corresponding to 39 districts/municipalities in 15 dengue endemic provinces in Indonesia (Figure 1). These provinces include Aceh, West Sumatra, Lampung, Bangka-Belitung, Banten, West Kalimantan, South Kalimantan, North Sulawesi, West Java, East Java, Southeast Sulawesi, Maluku, West Nusa Tenggara, East Nusa Tenggara, and North Maluku. This study is part of the Indonesia national project, Rikhus Vektora led by the Ministry of Health which started in 2016.
A mosquito survey was performed in all study sites from July to August 2016 during the rainy season. Larva collection was performed in at least 100 households taken at random in each study site according to the recommendations for the calculation of the Stegomyia indices. Adult collection of Aedes mosquitoes were performed in the morning (morning resting) on mosquitoes resting inside houses using manual aspirators. Adult mosquitoes were also collected outside using standard procedures for all night human-landing collection methods from 6 p.m. to 6 a.m. All methodologies used in this study are described in the Ministry of Health guidelines (22). All methodologies investigated must be compatible with the “one point/one day” process of decision making. This “one point/one day” concept means that collectors do not stay in the same sampling site over a long period of time. As soon as the threshold of 100 households is reached, they calculate the Stegomyia indices and move to another sampling site. Long-term sampling methods are not suitable. Field data collections for larva and adult Aedes mosquitoes were performed by trained collectors in collaboration with local volunteers, local authorities, and staff from district/municipality dengue control programs.
At least 100 households were taken at random in each study site for larval and pupal sampling. All artificial and natural water containers in and around each household were inspected for mosquito larvae and pupae. At least one larva (second, third, and/or fourth instars) and pupae (if any) from each positive container were collected with pipettes and tea strainers. Water from large containers was first removed with a water hose and then sieved with tea strainers. Larvae or pupae were then collected on a white plastic tray. Immature stages were pipetted and placed in a plastic clip with water, labeled, and taken to the field laboratory. Data on infected containers and households were used for calculating the Stegomyia indices. This corresponds to the single larva method routinely implemented for mosquito monitoring. For the rearing method, immature mosquitoes (pupae and larvae) were reared in 250-ml plastic cups covered with gauze under room temperature. Larvae were fed on tetrabit fish food until adult mosquitoes emerged. Emerged adult mosquitoes from the same household and species were then killed in a freezer (−20°C) or by using ethyl acetate for 5–10 min and immediately stored in 1.5-ml vial tubes with RNALater (Qiagen, Hilden, Germany) by pools of up to 25 specimens. Mosquitoes from different households or different species were not pooled together. Immature stages that did not yield adults by the fourth day were pooled based on location (maximum 25 larvae per tube).
Two adult mosquito sampling methods were conducted simultaneously in all study sites, i.e., morning resting and human landing collection, to which a third one, an animal-baited trap, was added in one location (Malang, East Java).
(1) Morning resting collections were made by eight collectors using hand nets and aspirators. Collections were conducted from 7 to 9 a.m. and included any resting locations within the house. All adult mosquitoes were placed into labeled paper caps and taken to the field laboratory for further analysis.
(2) Human landing collections were performed by eight local volunteers as collectors in three selected houses in each study site for sampling adult mosquitoes using mouth aspirators. They were all trained before collecting mosquitoes. Three teams of two people sampled outdoors (up to 5 m from the house) and indoors. Each collector sat on a chair while exposing the legs. Sampling was conducted all night from 6 p.m. to 6 a.m. The teams rotated and changed roles regularly every 2 h with a 2-h break. Although the targeted Aedes mosquitoes are diurnal, Indonesia law does not allow human landing collection during daytime. Therefore, collections had to be conducted at night. This introduces a strong bias in the sampling, but because it is what surveillance teams do in accordance with the law, this method was nevertheless considered. Mosquitoes that have been collected per hour were then taken to the field laboratory for species identification and further analysis.
(3) An animal-baited trap was conducted by using tame animals, i.e., cows, placed inside a net all night. Mosquito collections were carried out for 15 min/h inside the nets by three collectors. Collected mosquitoes were then similarly preserved as for the human landing collection method.
All mosquitoes from these three collecting methods identified as Ae. aegypti and Ae. albopictus were then killed with ethyl acetate, pooled up to 25 mosquitoes in labeled 1.5-ml vial tubes with RNAlater (Qiagen, Hilden, Germany) and preserved based on the same cold chain management as above for larvae.
The Ae. aegypti and Ae. albopictus mosquito pools were homogenized in 1.5-ml tubes containing 200 μl PBS 1x by using pellet pestles. RNA was extracted using QIAamp®Viral RNA Mini Kit (Qiagen®, Courtaboeuf, France). RNA was extracted from 200-μl homogenized samples following the manufacturer's instructions. All RNA extracted samples were analyzed for dengue detection using Lanciotti's protocol (23). The nested RT-PCR for dengue was performed using SimpliAmp Thermal Cycler Applied Biosystems™ (ThermoFisher Scientific®, United States). Amplification of dengue RNA was carried out with following specific primers: D1 (5′-TCA ATA TGC TGA AAC GCG CGA GAA ACC G-3′), D2 (5′-TTG CAC CAA CAG TCA ATG TCT TCA GGT TC-3′), TS1 (5′-CGT CTC AGT GAT CCG GGG G-3′), TS2 (5′-CGC CAC AAG GGC CAT GAA CAG-3′), TS3 (5′-TAA CAT CAT CAT GAG ACA GAG C- 3′), and TS4 (5′-CTC TGT TGT CTT AAA CAA GAG A-3′). The first amplification of dengue virus was performed using Superscript III one-step RT-PCR kit (Invitrogen, Carlsbad, CA). The cycling conditions consisted of an initial 95°C denaturation step for 2 min, followed by 40 cycles of 95°C denaturation for 30 s, 60°C annealing for 1 min, and 72°C extension for 1 min 30 s, and a final extension step 72°C for 10 min. Samples were then stored at 4°C. First-step PCR products were run on 2% agarose gel under 120 V current for 1 h. Visualization was done using SYBR® safe DNA gel stain (Invitrogen, Carlsbad, CA, USA) under UV condition in a GelDoc system. The presence of the 511-bp control band indicated a dengue virus (DENV) positive sample. Subsequent genotyping was conducted by using the first step PCR product with thermal cycle setting as follow: initial denaturation step at 95°C for 2 min, followed by 10 cycles of denaturation step at 95°C for 30 s, 60°C annealing for 1 min, and an extension step at 72°C for 1 min and 30 s. The final extension step was conducted at 72°C for 10 min. Subsequently, samples were stored at 4°C. Multiplex genotyping reactions yielded a single specific band with the size of 482 bp for DENV-1, 119 bp for DENV-2, 290 bp for DENV-3, and 389 bp for DENV-4. All field samples were tested for the presence of dengue virus after being pooled by 25 individuals of the same species.
The incidence, number of new dengue cases per total population for the time of the study, was obtained from the district health center in each district.
A first factor analysis of mixed data (FAMD) (24) was conducted using the incidence data, the number of mosquitoes, and the number of positive pools for each dengue serotype as quantitative parameters and mosquito species, methods of collection, and provinces as qualitative parameters. The effectiveness of the collection methods (qualitative data) against mosquito species (quantitative data) was assessed using a second FAMD. These analyses were performed using the R software with FactoMineR (25).
A total of 44,675 mosquitoes were collected from 39 locations (Figure 1, Supplementary Table 1). Out of these 44,675 mosquitoes collected, 32,525 (72.8%) were Ae. aegypti and 10,300 (23.1%) were Ae. albopictus, and 1,850 (4.1%) were undetermined. When considering the method of capture, the highest number of captured individuals was, as expected, obtained when targeting larvae. The single larva method was the most efficient in terms of number of individuals collected. A total of 36,500 larvae were collected with this method out of which 27,475 were Ae. aegypti, 7,775 were Ae. albopictus and 1,250 were not identified. Out of 6,450 larvae collected and reared, 4,325 were Ae. aegypti, 1,575 were Ae. albopictus, and 550 were not identified. With both larval methods, a bias was observed in favor of Ae. aegypti, which represented 75.27% and 37.05% of all samples for the single lava and rearing methods, respectively. Very different results were obtained with the adult capture method. From the three methods used, human landing was the most efficient even though a bias was introduced by the legal obligation to perform this approach by night. Out of 1,325 adult mosquitoes captured, 325 were Ae. aegypti, 975 were Ae. albopictus and 25 were not identified. The animal-baited trap method yielded only 25 mosquitoes, all being Ae. albopictus. The ratio between Ae. aegypti and Ae. albopictus was reversed with this time a bias in favor of Ae. albopictus. It represented 73.58% and 53.33% for the human landing and morning resting methods, respectively. The animal baited trap method yielded only Ae. albopictus, but considering the very low number of mosquitoes captured, i.e., 25, this is not significant.
A total of 89 pools were positive for dengue virus. The most frequently encountered virus was DENV2 (n = 44), which made up half of the positive samples. DENV3 and DENV1 followed with 20 and 17 positive pools, respectively. DENV4 was detected in only one pool. Combinations were also detected. Eight pools contained a combination of DENV1 and DENV2, whereas the combination of DENV1 and DENV3 was found in only one pool. Another single pool contained the triple combination DENV1–DENV2–DENV3. With respect to the geographic distribution, a strong imbalance was observed. A large part of the detected dengue viruses, i.e., 56 (63%), were found in mosquitoes collected in the province of Aceh. All four dengue virus serotypes and all positive combinations were found in this province. The other provinces where positive pools were detected were West Sumatra (n = 5), Lampung (n = 6), Bangka-Belitung (n = 4), West Kalimantan (n = 2), South Kalimantan (n = 1), North Sulawesi (n = 2), East Java (n = 7), and Maluku (n = 6). A strong imbalance was also observed when considering the nature of the positive samples. Mosquito larvae were almost the exclusive source of virus, i.e., 93.3% (n = 83) with 70.8% (n = 63) found with the single larva method and 22.5% (n = 20) for the rearing method. Only six pools (6.7%) of adult mosquitoes were found positive with the human landing method totalizing two pools (2.3%), and four pools (4.4%) were found positive in mosquitoes collected with the morning resting method. An imbalanced result was also found regarding the mosquito species with 76.4% (n = 68) of the positive pools corresponding to Ae. aegypti and 23.6% (n = 21) corresponding to Ae. albopictus.
A FAMD was performed to determine the potential correlation between the various parameters considered: mosquito species, province, number of mosquitoes, collection method, dengue virus, and dengue incidence (Figure 2A). The only correlation that could be found was between the province and the incidence (Figure 2A). However, the global level of explanation was low (20%) indicating a lack of correlation between any of the parameters with the exception of province and incidence of dengue. A similar result was found when comparing the different collection methods with the mosquito species. The only, but rather weak, correlation that could be found was the preferred association of the larval methods with Ae. aegypti and the adult methods with Ae. albopictus (Figure 2B, Supplementary Table 1).
Figure 2. Multivariate analysis of parameters. (A) Global factor analysis of mixed data. (B) FAMD assessment of the effectiveness of the collection methods.
In the absence of commercialized vaccines and of any medical treatment, the management of dengue relies only on mosquito control and on prevention. Finding efficient and reliable descriptors for assessing the risk of dengue outbreaks is, thus, a priority in all dengue-endemic countries. The main tools currently in use for assessing this risk of dengue outbreak are the Stegomyia indices (15, 26), which rely on the calculation of the relative density of mosquito larvae present in containers and in households through the CI, HI, and BI (26, 27). However, these indices were shown to display no correlation with dengue infection rates and are, thus, not reliable descriptors (16, 18, 28, 29).
Because the Stegomyia indices are not reliable descriptors, they must be replaced by other descriptors. They could be replaced by other entomological indices provided that these other entomological indices are reliable. Entomological indices are, by definition, based on the capture of insects. Therefore, it is essential to assess whether the collection methods are reliable and reproducible and do not generate biases. This is independent from the calculation model applied. It is an intrinsic trait of the collection method itself. If not, entomological indices cannot be used as predictors. They must also be compatible with the logistical and administrative constraint of the “one point/one day” nature of the operational monitoring and decision process. This operational constraint is essential. Agents conducting mosquito surveys do not spend all their time at the same place and make their calculations and assessments and release their conclusions usually within 1 day. They move to another place as soon as the threshold of 100 households needed for calculation of the official Stegomyia indices is reached. The window for deciding on treatment is also narrow because treatments must be effective before outbreaks occur. Long-term assessments within a single place are scientific experimentations for the purpose of understanding biological processes or developing techniques and methodology but are not adapted to fast decision making. They are not suitable for use under such conditions. This constraint is even more important in large countries such as Indonesia. As a consequence, trapping methods, which are long-term methods based on cumulated data, are not favored.
The methods implemented being used to generate descriptors must be reliable regardless of the location and local conditions. We, therefore, conducted this large-scale study to assess the procedures endorsed by the Ministry of Health guidelines within a very large study involving many sampling sites corresponding to different urbanistic and geographical conditions. This work is, to our knowledge, the most extensive one with 44,675 mosquitoes collected in 39 different sampling locations over Indonesia within a short 2-month period, allowing thus a robust statistical analysis. The main conclusion of this work is that it is not only the Stegomyia indices, but any kind of entomological indices that might be at best of very limited use. Not only could no correlation be found between any methods and the presence of dengue virus in mosquitoes, but no correlation could be found between any of the methods and the incidence of dengue. The higher proportion of Ae. albopictus found in the human landing method might be related to the fact that this species is more crepuscular than Ae. aegypti. No consistency was found for any given method from one place to another. Finally, there was no consistency in the efficiency of a given method for detecting dengue. The single-larva and rearing methods yielded 63% of all dengue-positive samples in the sole province of Aceh. However, the incidence of dengue in Aceh is not the highest among all provinces and is rather the average. Provinces displaying the highest incidence, such as Bangka-Belitung, South Kalimantan, or North Sulawesi, did not yield any dengue-positive larvae. The only single positive pool in these provinces was found in South Kalimantan among morning resting adults. This lack of correlation between incidence and dengue infection rate in mosquitoes is also a drawback for methods associating the capture of adults and the direct detection of dengue virus in the sampled mosquitoes (30–32).
The use of Stegomyia indices and the monitoring of mosquitoes are today the main means of assessment of the risk of dengue outbreaks and efficiency of mosquito control. Previous studies demonstrated the lack of correlation of the Stegomyia indices with the risk of dengue outbreaks and dengue incidence (12, 14–18). In this work, we further demonstrate the lack of consistency of the various collection methods officially recommended and the lack of correlation with the recorded dengue incidence. Altogether, this indicates that entomological approaches do not provide reliable indicators of risks of dengue outbreak. The risk with these methods is mostly misleading interpretation and misguided decisions and allocation of resources. The assessment of the risk of dengue outbreaks should be reconsidered from a different angle.
A previous study found that a factor positively correlated with the incidence of dengue was the human population density (18). This provides an angle for further research. Ae. aegypti and Ae. albopictus are both anthropophilic species, and the human environment is, thus, a major driver of their demography and distribution. The measurement and prediction of the risk of dengue outbreaks should be considered from the angle of urbanism and human societal factors. Efforts should be devoted to the development of novel societal indices. It is even more important to communicate on this issue because dengue endemic countries worldwide, as well as WHO, still base their recommendations and dengue management procedures on entomological indices.
The datasets presented in this study can be found in online repositories. The names of the repository/repositories and accession number(s) can be found in the article/Supplementary Material.
The studies involving human participants were reviewed and approved by the Ethical Commission Board of the NIHRD, Ministry of Health, Indonesia (No. LB.02.01/5.2/KE.003/2016 and No. LB.02.01/5.2/KE.020/2017). The patients/participants provided their written informed consent to participate in this study.
TG, LS, TS, RW, and MP conceived and designed the field studies. MujiyonoM, SN, and DS prepared samples. TG and MP ran molecular analyses and laboratory experiments. TG, LG, and RF analyzed the data. MujiyantoM prepared the map. TG and RF wrote the manuscript. SM, LG, and RF provided critiques and significant revisions to the manuscript. All authors contributed to the article and approved the submitted version.
The research was supported by the Institute for Vector and Reservoir Control Research and Development, National Institute of Health Research and Development, Ministry of Health Indonesia. SM, LG, and RF were supported by the Université de Montpellier, IRD, Espace-Dev, and CIRAD, Montpellier, France. The project was supported in part by the French-Indonesian Nusantara projects Zika & Co and SOCIAL.
The authors declare that the research was conducted in the absence of any commercial or financial relationships that could be construed as a potential conflict of interest.
Authors express their gratitude to all field mosquito collectors, the experts, and technical team and validators of the Rikhus Vektora project for great support. The authors would also like to thank the head of Laboratory Data Management NIHRD, MoH Indonesia who have permitted to do further analyses. The authors also gratefully acknowledge especially Widiarti, Mochammad Choirul Hidajat, Dhian Prastowo, Riyani Setyaningsih, Yusnita Mirna Anggraeny, Siti Alfiah, Evi Sulistyorini, Lasmiati, Sapto Prihasto Siswoko, Warido, Valentinus Widiratno, Rima Tunjungsari Dyah Ayuningtyas, Fahmay Dwi Ayuningrum, Ary Octsari Yanti, Sekar Negari Kusumaningtyas, Jeri Cahyandaru, Indira Diah Utami, Happy Anita Putri, Syarifah Nurhayati, Senja Rahayu Kinanti, Wahyu Sri Kunto, and other members of the IVRCRD-NIHRD, MoH Indonesia for all support of fieldwork and laboratory work.
The Supplementary Material for this article can be found online at: https://www.frontiersin.org/articles/10.3389/fmed.2021.685926/full#supplementary-material
Supplementary Table 1. Sampling localities and characteristics of samples.
HI, House Index; CI, Container Index; BI, Breteau Index; FAMD, Factor Analysis of Mixed Data.
1. World Health Organization. Global Strategy for Dengue Prevention and Control 2012-2020. World Health Organization, Geneva (2012).
2. Ritchie SA. Dengue vector bionomics: why Aedes aegypti is such a good vector. In: Gubler DJ, Ooi EE, Subhash Vasudevan JF, editor. Dengue and Dengue Hemorrhagic Fever. 2nd ed. Boston, MA: CAB International (2014). p. 455–80. doi: 10.1079/9781845939649.0455
3. Nadjib M, Setiawan E, Putri S, Nealon J, Beucher S, Hadinegoro R, et al. Economic burden of dengue in Indonesia. PLoS Negl Trop Dis. (2019) 13:e0007038. doi: 10.1371/journal.pntd.0007038
4. Maula AW, Utarini A. Ten-years trend of dengue research in Indonesia and South-east Asian countries: a bibliometric analysis. Glob Health Action. (2018) 11:1504398. doi: 10.1080/16549716.2018.1504398
5. World Health Organization. Treatment, Prevention and Control Global Strategy for Dengue Prevention and Control 2012-2020. (2012). Available online at: http://apps.who.int/iris/bitstream/10665/75303/I/9789241504034_eng.pdf
6. MoH-Indonesia. Situasi Demam Berdarah Dengue di Indonesia. Pusat data dan Informasi Kementerian Kesehatan RI (2018). Available online at: https://pusdatin.kemkes.go.id/download.php?file=download/pusdatin/infodatin/InfoDatin-Situasi-Demam-Berdarah-Dengue.pdf
7. Sutherst RW. Global change and human vulnerability to vector-borne diseases. Clin Microbiol Rev. (2004) 17:136–73. doi: 10.1128/CMR.17.1.136-173.2004
8. Mulligan K, Elliott SJ, Schuster-Wallace C. The place of health and the health of place: Dengue fever and urban governance in Putrajaya, Malaysia. Health Place. (2012) 18:613–20. doi: 10.1016/j.healthplace.2012.01.001
9. de Mendonca HFMS, Ferreira AL, dos Santos CB, Rezende HR, Ferreira GEM, Leite GR, et al. Breeding sites of Aedes aegypti in metropolitan vacant lots in Greater Vitória, State of Espírito Santo, Brazil. Rev Soc Bras Med Trop. (2011) 44:243–6. doi: 10.1590/S0037-86822011000200022
11. Kusriastuti R, Sutomo S. Evolution of dengue prevention and control programme in Indonesia DF / DHF Disease Burden. Dengue Bull. (2005) 29:1–7.
12. Resende MC De, Silva IM, Ellis BR, Eiras ÁE. A comparison of larval, ovitrap and MosquiTRAP surveillance for Aedes (Stegomyia) aegypti. Mem Inst Oswaldo Cruz. (2013) 108:1024–30. doi: 10.1590/0074-0276130128
13. Nathan MB. Critical review of Aedes aegypti control programs in the Caribbean and selected neighboring countries. J Am Mosq Control Assoc. (1993) 9:1–7.
14. Focks D. A Review of Entomological Sampling Methods and Indicators for dengue Vectors. WHO (2003).
15. Bowman LR, Runge-Ranzinger S, McCall PJ. Assessing the relationship between vector indices and dengue transmission: a systematic review of the evidence. PLoS Negl Trop Dis. (2014) 8:e2848. doi: 10.1371/journal.pntd.0002848
16. Chang FS, Tseng YT, Hsu PS, Chen CD, Lian IB, Chao DY. Re-assess vector indices threshold as an early warning tool for predicting dengue epidemic in a dengue non-endemic country. PLoS Negl Trop Dis. (2015) 9:e0004043. doi: 10.1371/journal.pntd.0004043
17. Cromwell EA, Stoddard ST, Barker CM, Van Rie A, Messer WB, Meshnick SR, et al. The relationship between entomological indicators of Aedes aegypti abundance and dengue virus infection. PLoS Negl Trop Dis. (2017) 11:e0005429. doi: 10.1371/journal.pntd.0005429
18. Garjito TA, Hidajat MC, Kinansi R, Setyaningsih R, Anggraeni YM, Mujiyanto M, et al. Stegomyia indices and risk of dengue transmission: a lack of correlation. Front Public Health. (2020). 8:328. doi: 10.3389/fpubh.2020.00328
19. Morrison AC, Astete H, Chapilliqun F, Ramirez-Prada G, Diaz G, Getis A, et al. Evaluation of a sampling methodology for rapid assessment of Aedes aegypti infestation levels in Iquitos, Peru. J Med Entomol. (2004) 41:502–10. doi: 10.1603/0022-2585-41.3.502
20. Focks D, Chadee DD. Pupal survey at epidemioogically significant surveillance method for Aedes aegypti: an example using data from Trinidad. Am J Trop Med Hyg. (1997) 56:159–67. doi: 10.4269/ajtmh.1997.56.159
21. WHO. Malaria Entomology and Vector Control: Tutor's Guide. (2013). Available online at: http://whqlibdoc.who.int/hq/2003/WHO_CDS_CPE_SMT_2
22. IVRCRD. Manual on Mosquitoes Field Sampling Methods (In Bahasa Indonesia). Available online at: http://www.b2p2vrp.litbang.kemkes.go.id/publikasi/download/59
23. Lanciotti RS, Calisher CH, Gubler DJ, Chang GJ, Vorndam AV. Rapid detection and typing of dengue viruses from clinical samples by using reverse transcriptase-polymerase chain reaction. J Clin Microbiol. (1992) 30:545–51. doi: 10.1128/JCM.30.3.545-551.1992
25. Lê S, Josse J, Husson F. FactoMineR: an R package for multivariate analysis. J Stat Softw. (2008) 25:1–18. doi: 10.18637/jss.v025.i01
26. World Health Organization. Dengue: Guidelines for Diagnosis, Treatment, Prevention and Control. (2009). Available online at: https://www.who.int/rpc/guidelines/9789241547871/en/
27. Pan American Health Organization. Dengue and Dengue Hemorrhagic Fever in the Americas: Guidelines for Prevention and Control (1994).
28. Wijayanti SP, Sunaryo S, Suprihatin S, McFarlane M, Rainey SM, Dietrich I, et al. Dengue in Java, Indonesia: relevance of mosquito indices as risk predictors. PLoS Negl Trop Dis. (2016) 10:e0004500. doi: 10.1371/journal.pntd.0004500
29. Agha SB, Tchouassi DP, Bastos AD, Sang R. Assessment of risk of dengue and yellow fever virus transmission in three major Kenyan cities based on Stegomyia indices. PLoS Negl Trop Dis. (2017) 11:e0005858. doi: 10.1371/journal.pntd.0005858
30. Muller DA, Frentiu FD, Rojas A, Moreira LA, O'Neill SL, Young PR. A portable approach for the surveillance of dengue virus-infected mosquitoes. J Virol Methods. (2012) 183:90–3. doi: 10.1016/j.jviromet.2012.03.033
31. Lau SM, Chua TH, Sulaiman WY, Joanne S, Lim YAL, Sekaran SD, et al. A new paradigm for Aedes spp. surveillance using gravid ovipositing sticky trap and NS1 antigen test kit. Parasites Vectors. (2017). 10:151. doi: 10.1186/s13071-017-2091-y
Keywords: Aedes aegypti, Aedes albopictus, dengue, collection methods, dengue incidence, Indonesia
Citation: Garjito TA, Susanti L, Mujiyono M, Prihatin MT, Susilo D, Nugroho SS, Mujiyanto M, Wigati RA, Satoto TBT, Manguin S, Gavotte L and Frutos R (2021) Assessment of Mosquito Collection Methods for Dengue Surveillance. Front. Med. 8:685926. doi: 10.3389/fmed.2021.685926
Received: 26 March 2021; Accepted: 27 April 2021;
Published: 08 June 2021.
Edited by:
Xiaoxiao Wang, Zhejiang Center for Disease Control and Prevention (Zhejiang CDC), ChinaReviewed by:
Rafael Maciel-de-Freitas, Oswaldo Cruz Foundation (Fiocruz), BrazilCopyright © 2021 Garjito, Susanti, Mujiyono, Prihatin, Susilo, Nugroho, Mujiyanto, Wigati, Satoto, Manguin, Gavotte and Frutos. This is an open-access article distributed under the terms of the Creative Commons Attribution License (CC BY). The use, distribution or reproduction in other forums is permitted, provided the original author(s) and the copyright owner(s) are credited and that the original publication in this journal is cited, in accordance with accepted academic practice. No use, distribution or reproduction is permitted which does not comply with these terms.
*Correspondence: Triwibowo Ambar Garjito, dHJpd2lib3dvQGxpdGJhbmcua2Vta2VzLmdvLmlk; Roger Frutos, cm9nZXIuZnJ1dG9zQGNpcmFkLmZy
Disclaimer: All claims expressed in this article are solely those of the authors and do not necessarily represent those of their affiliated organizations, or those of the publisher, the editors and the reviewers. Any product that may be evaluated in this article or claim that may be made by its manufacturer is not guaranteed or endorsed by the publisher.
Research integrity at Frontiers
Learn more about the work of our research integrity team to safeguard the quality of each article we publish.