- 1Radiology Department, University of Tennessee Graduate School of Medicine, Knoxville, TN, United States
- 2Lucerno Dynamics LLC, Cary, NC, United States
- 3Department of Molecular Imaging, Carilion Clinic, Roanoke, VA, United States
Background: The patient benefit from a diagnostic nuclear medicine procedure far outweighs the associated radiation risk. This benefit/risk ratio assumes a properly administered radiopharmaceutical. However, a significant diagnostic radiopharmaceutical extravasation can confound the procedure in many ways. We identified three current extravasation hypotheses espoused by medical societies, advisory committees, and hundreds of individual members of the nuclear medicine community: diagnostic extravasations do not cause harm, do not result in high absorbed dose to tissue, and require complex dosimetry methods that are not readily available in nuclear medicine centers. We tested these hypotheses against a framework of current knowledge, recent developments, and original research. We conducted a literature review, searched regulatory databases, examined five clinical cases of extravasated patients, and performed dosimetry on those extravasations to test these globally accepted hypotheses.
Results: A literature review found 58 peer-reviewed documents suggesting patient harm. Adverse event/vigilance report database reviews for extravasations were conducted and revealed 38 adverse events which listed diagnostic radiopharmaceutical extravasation as a factor, despite a regulatory exemption for required reporting. In our own case material, assessment of care was evaluated for five extravasated patients who underwent repeat imaging. Findings reflected results of literature review and included mis- or non-identification of lesions, underestimation of Standardized Uptake Values (SUVs) by 19–73%, classification of scans as non-diagnostic, and the need to repeat imaging with the associated additional radiation exposure, inconvenience, or delays in care. Dosimetry was performed for the same five cases of diagnostic radiopharmaceutical extravasation. Absorbed doses to 5 cm3 of tissue were between 1.1 and 8.7 Gy, and shallow dose equivalent for 10 cm2 of skin was as high as 4.2 Sv.
Conclusions: Our findings suggest that significant extravasations can or have caused patient harm and can irradiate patients' tissue with doses that exceed medical event reporting limits and deterministic effect thresholds. Therefore, diagnostic radiopharmaceutical injections should be monitored, and dosimetry of extravasated tissue should be performed in certain cases where thresholds are thought to have been exceeded. Process improvement efforts should be implemented to reduce the frequency of extravasation in nuclear medicine.
Introduction
Diagnostic nuclear medicine procedures have been consistently and appropriately viewed through the lens that the benefits from these procedures demonstrably exceed the inherent radiation risk of the procedure. In 2002, fifty nuclear medicine leaders emphasized in the Guide for Diagnostic Nuclear Medicine (1) “more than one-third of a billion doses have been administered to patients, with a track record for safety that is unparalleled. The radiation doses associated with diagnostic nuclear medicine procedures average 4.4 mSv effective dose equivalent according to a National Council on Radiation Protection and Measurement study published in 1991.” The authors also describe that deterministic effects can “occur only after relatively high dose levels that exceed the threshold for those effects, usually a dose on the order of 100 roentgen equivalent man (rem) (1 Sv)” and “the risk of stochastic effects increases as a function of radiation dose.” They state that “the risk of deterministic effects attributed to the exposures likely to be encountered in diagnostic nuclear medicine procedures is insignificant.” In diagnostic nuclear medicine procedures where the radiopharmaceutical is assumed to be injected properly, the absorbed dose to patient tissue would be <1 mGy or 1 mSv dose equivalent.
An infiltration is the inadvertent injection of a pharmaceutical into the tissue instead of the vein, as intended. Although an extravasation is typically defined as an infiltration of a vesicant (2), an infiltration of a radiopharmaceutical can be considered an extravasation due to the effects of ionizing radiation on patient tissue. In 2020, the Nuclear Regulatory Commission (NRC) which regulates the use of radioactive isotopes in the United States requested public comments on a petition that the NRC eliminate a policy. The policy exempts extravasations from medical event reporting, even if they meet reporting requirements. The NRC's Advisory Committee on the Medical Uses of Isotopes (ACMUI), medical societies, and leading members of the nuclear medicine and radiology communities have provided their position on diagnostic extravasations to the NRC.
In a September 2019 meeting with the NRC regarding extravasations, ACMUI members reported they were “unaware of any cases where there has been patient harm due to extravasation” (3). The public comments submitted to the NRC regarding the extravasation petition also support the commonly held hypothesis. In 396 submitted comments, medical societies or individual members stated there is no clinical data that diagnostic radiopharmaceutical extravasation is a patient safety issue. Other comments provide additional insight into these hypotheses regarding diagnostic extravasations. The American College of Radiology (ACR) stated: “A study into reports of extravasation found that <0.001% of diagnostic nuclear medicine extravasations result in temporary symptoms of any kind.”1 One member stated: “Gamma ray emitting radiotracer which has very negligible effects on the skin if infiltrated and is not at risk of being harmful.” Further, the ACR compared contrast media and chemotherapeutic administrations to nuclear medicine administrations as follows when stating: “They are not analogous to administrations of radiopharmaceuticals, which are typically small volume, without inherent properties harmful to tissue.” In discussing diagnostic extravasations, the ACR also stated: “it would be unlikely if not impossible to meet the §35.3045(b) standard with typical diagnostic nuclear medicine agents.” Leading members of the Society of Nuclear Medicine and Molecular Imaging (SNMMI) when referencing diagnostic extravasations stated “An extravasated radiopharmaceutical administration would never deliver 0.5 Sv (50 rem) to any whole organ or tissue, including the skin.”2 And the Health Physics Society stated: “there is no evidence that infiltration of radiopharmaceuticals carries any health consequences for the patient or the general public” and “designating extravasations as a Medical Event would call for a dose estimation, which is far from a trivial process. Use of simplified techniques do not effectively account for removal of the pharmaceutical from the injection site and the time varying geometry of the source, though they can be modified to do so with additional data collection.”3 These positions reveal commonly held hypotheses regarding diagnostic extravasations—they do not cause patient harm, their resulting absorbed dose cannot exceed regulatory reporting limits and threshold limits that can lead to adverse tissue reactions, and dosimetric characterization is beyond the capabilities of most nuclear medicine centers.
We analyzed the three hypotheses considering data from: (1) Current literature, (2) Databases of adverse events and vigilance reports of patient harm, and (3) Five patient cases of significant extravasations in our centers for whom dosimetry calculations were performed. Our findings suggest new hypotheses related to the impact of nuclear medicine extravasations. Diagnostic radiopharmaceutical extravasations can result in patient harm, can result in absorbed doses that exceed current regulatory reporting limits and threshold limits that can lead to adverse tissue reactions, and appropriate dosimetry methods can be applied with minimal resources to provide effective characterization of extravasations across most centers.
Methods
Literature Review
We conducted a review of the medical literature looking for evidence of patient consequences from diagnostic extravasations. We used search word(s) such as: radiopharmaceutical administration, infiltration, extravasation, paravenous injections, interstitial injections, and dose tissuing. We then searched for evidence of harm or potential for harm as a result of diagnostic extravasations. Harm or potential harm included: unnecessary additional exposure to radiation through repeat imaging, delays in treatment, false positives or negatives that led to additional patient intervention, incorrect diagnosis, or incorrect treatment assessment, and deterministic effects or adverse tissue reactions.
Database Search Strategy
We conducted a systematic search of the Food and Drug Administration's (FDA) Adverse Event Reporting System (FAERS) (4) Public Dashboard for U.S. adverse events and the EudraVigilance (EV) (5) database for adverse drug reactions in the European Economic Area (EEA) to find relevant reports of diagnostic nuclear medicine extravasations through November 2019.
FAERS is a compilation of medication error reports voluntarily submitted to FDA by healthcare professionals, consumers, and manufacturers. The FAERS search included adverse drug reaction reports from 1968 to 2019, based on FDA-approved radiopharmaceuticals (6) or trade names. The search methodology begins with “Search.” Select “Search by Product” and insert FDA-approved radiopharmaceutical or trade name. Initiate search. Select “listing of cases.” Results were filtered by selecting “reaction group.” These results were further filtered by selecting the group: “General Disorders and Administration Site Conditions.” Within this group, additional filtering was employed by selecting “Reaction” and then expanding the “General Disorders and Administration Site Conditions.” In “Number of Cases” the results were further filtered by selecting the terms: “extravasation,” “administration site extravasation,” “application site extravasation,” “catheter site extravasation,” “infusion site extravasation,” “injection site extravasation,” “medical device site extravasation,” and “tissue infiltration.” Results were manually exported, duplicates were removed, and findings summarized.
EV is the pharmacovigilance database to manage the collection and analysis of suspected adverse drug reactions authorized within the EEA. Reports are submitted electronically by national regulatory authorities and by pharmaceutical companies that hold licenses for medicines. The search methodology begins with “Search.” Select “Suspected adverse drug reaction reports for Products” and select a letter or number from which to browse a radiopharmaceutical name or trade name. Initiate the search by selecting the name. Select the “Line Listing” tab to access the filtering conditions for individual cases identified in EV for the radiopharmaceutical. Within filtering conditions, from the “Reaction Groups” dropdown list, select “General disorders and administration site conditions. From the “Reported Suspected Reaction” dropdown list, select terms “extravasation,”, “administration site extravasation,” “application site extravasation,” “catheter site extravasation,” “infusion site extravasation,” “injection site extravasation,” “medical device site extravasation,” and “tissue infiltration.” Using the “Gateway Date” dropdown list, select a year and select “Run Line Listing Report.” If individual case reports were input into the database for the given year, reports will be listed. This search process was repeated for each year from 1994 to 2019 for each radiopharmaceutical. Results were manually exported, duplicates were removed, and findings were summarized.
Patient Management Effects
We also analyzed data from two nuclear medicine centers – Carilion Clinic, Roanoke, VA and the University of Tennessee Medical Center, Knoxville, TN. In both centers, injection quality is routinely monitored by technologists using bilateral, external radiation detectors (Lara® System, Lucerno Dynamics, Cary, NC, USA) that provide a real time display of counts after injection and prior to imaging. Once the administration is complete, nuclear medicine physicians also review this information now in the form of a time-activity curve (TAC) to evaluate the quality of radiopharmaceutical administration. Repeat imaging in these centers is routinely ordered for patients with extravasations that the interpreting nuclear medicine physician suspects would negatively affect the diagnostic value of the imaging study. For this report we selected five representative cases involving extravasated diagnostic radiopharmaceuticals: 18F-Fluorodeoxyglucose (18F-FDG) for Positron Emission Tomography/Computed Tomography (PET/CT) (N = 4) and 99mTc-Methylene diphosphonate (99mTc-MDP) for Single Photon Emission Computed Tomography (SPECT) (N = 1). Acquisition and reconstruction parameters for each site are described below.
At Carilion Clinic, when performing PET/CT the standard injected activity was 10mCi 18F-FDG using a standard step and shoot acquisition routine with axial range matched to the CT FOV. For CT Acquisition, data were acquired using 120 kVp with CareDose 4D. Data were acquired using a 20 × 0.6 mm detector setting with 5.0 mm slice thickness. For Bone SPECT the standard injected activity was 25mCi 99mTc-MDP using a LEHR collimator and matrix size of 256 × 256 with a zoom of 1. SPECT data were acquired using 30 views in a non-circular (body contour) orbit with an acquisition time of ~20 s per view. For CT Acquisition, data were acquired using 130 kVp with CareDose 4D and pitch of 1.5 and detector settings of 16 × 1.2 mm with 3 mm slice thickness. For visualization, both B80s AC for ECT and B50s medium sharp with bone window kernels were employed.
At University of Tennessee Medical Center, when performing PET/CT the standard injected activity was 10 mCi of 18F-FDG ±20%. Data were acquired using continuous bed motion acquisition with a bed speed of 1.5 mm/s. Reconstruction was performed using point spread function resolution recovery with time of flight (UltraHD PET, Siemens Healthineers, Malvern, PA). A matrix size of 200 × 200 was used with 3 iterations and 21 subsets and a Gaussian filter of 5 mm FWHM was applied. For CT, data were acquired using 120 kVp with CareDose 4D using a 5 mm acquisition technique reconstructed to 4 mm slice thickness and using a standard abdomen kernel.
All patients consented to publication of their images or were granted a waiver of consent by the local institutional review board. Attempts were made during the repeat studies to ensure that imaging parameters and patient preparation were as consistent as possible with the extravasated procedure to help assess extravasation effects. PET/CT scans were performed on the Biograph mCT camera (Siemens Healthineers, Knoxville, TN). SPECT scans were performed on the Symbia Intevo Bold™ camera (Siemens Healthineers, Knoxville, TN). Extravasated and repeated images were interpreted on the day of the procedure by a trained nuclear medicine physician and findings from the scan were recorded in the standard department reporting system.
Tissue Dosimetry
We calculated absorbed tissue dose using methods previously described in the literature (7). When considering extravasations, the calculated dose is for that tissue which contains residual radiopharmaceutical, and the absorbed energy comes from the radiopharmaceutical itself—self dose. As the radiopharmaceutical decays, it releases energy in the form of photons, x-rays, electrons, positrons, etc., with specific energy deposition characteristics dependent on the particular isotope. Furthermore, these primary emissions lead to secondary emissions through excitation of the surrounding tissue. Unlike the emissions used for imaging (i.e., 511 keV gammas for PET), many of these emissions will not penetrate the local tissue; their energy may be absorbed completely within a short distance.
To calculate self-dose to tissue from extravasation, three pieces of information are needed: absorbed energy per radioactive decay, initial source activity, and the rate at which the activity is decreasing due to the combination of physical half-life and biological clearance.
Using the methods described by Osborne et al. (7), we used the GATE Monte-Carlo framework to simulate absorbed energy per decay for both 18F and 99mTc. Simulations consisted of 5-gram spheres of water uniformly containing 1 MBq of each isotope as an ion source. Spheres with a mass of 5 g were chosen to strike a balance between accuracy of localized dosimetry vs. very high absorbed doses in very small tissue volumes. Absorbed energy within the sphere was then converted to absorbed dose rate in Gy/MBq-min.
Effective half-life is the combination of the isotope-specific physical half-life and the rate of biological clearance. This combination can be found using multiple image-based measurements of the area (8–13), or through measurements with a scintillation counter or other radiation detection system (14). We used data from external radiation detectors to estimate the effective half-life as described previously by Osborne et al. (7). The external detector data indicates the rate of change of localized radioactivity over time, to which we fit a mono-exponential function to obtain the effective half-life. The initial activity within the extravasation can be estimated by extrapolating a quantitative measurement of the injection site backwards to time zero using the effective half-life. We used quantitative PET imaging or qualitative image assessments for the activity estimate at imaging time and extrapolated to time zero using the effective half-life.
With the above values determined, calculation of absorbed dose is straight forward (Equations 1 and 2). Total absorbed energy in Joules is found by the multiplication of the initial activity in Bq, the absorbed energy per decay in Joules (1 keV = 1.602 × 10−16 J), the effective half-life in seconds, and one divided by the natural logarithm of two. Total absorbed dose is then the ratio of total absorbed energy divided by tissue mass in kilograms.
where A0 is the initial activity in Bq, Q is the absorbed energy per decay in J, and T1/2, eff is the effective half-life in sec.
where E is the total absorbed energy in J and M is the tissue mass in kg.
For skin, we used the computer code VARSKIN 6 (15) to calculate the shallow dose equivalent to 10 cm2 of skin overlaying the extravasation.
Results
Literature Review
We found 58 peer-reviewed articles that show how diagnostic extravasation can harm or have harmed patients. Extravasations have a negative effect on the sensitivity of PET/CT. The clinical implications of an extravasation on a PET/CT study for the management of cancer patients include under staging the disease (16–23), over staging the disease (16–18, 20, 24–45), therapeutic procedure planning errors (46), and therapy assessment errors (22, 45, 47–57). Extravasations have negative patient management implications also in PET/CT procedures that are used for indications other than oncology. For example, an extravasation that occurred during a myocardial perfusion study can lead to either a false positive or false negative misinterpretation with serious consequence for patient management (19, 58–61); an extravasation during an FDG neurological function study would adversely affect the reported results by limiting the FDG uptake in the brain (62); an extravasation during amyloid plaque imaging for Alzheimer's disease and dementia diagnosis can cause poor image quality (63) and during fever of unknown origin study may compromise imaging sensitivity and diagnostic capability.
Extravasations of diagnostic radiopharmaceuticals during gamma camera procedures have similar implications to those found in extravasated PET/CT procedures: misinterpretation of results may lead to patient harm, unnecessary invasive procedures, and additional exposure to radiation from repeat scans. In a renal scan/glomerular filtration rate studies extravasations can cause false-positive findings, can require repeat procedures (32), and may not be visible in the imaging FOV (64, 65). In Tc-99m Sestamibi studies an extravasated injection can result in a scan with poor-quality images, mask ischemia, and can lead to an inappropriate investigation for malignancy (58). In multigated acquisition (MUGA) studies used to assess the impact of a patient's chemotherapy treatment on myocardial function, an extravasation can result in suboptimal radiolabeling of blood cells with corresponding increased amounts of residual, unreacted free pertechnetate (66) and lead to inappropriate cessation of chemotherapy treatment. In dopamine transporter imaging studies assessing Parkinson's disease an extravasation of Ioflupane I-123 can confound the dopamine transporter study results (67, 68). In Ventilation Perfusion (V/Q) studies an extravasation creates the opportunity for false negative interpretations (69) with potential serious patient implications (70, 71). In planar bone scanning an injection issue can lead to misinterpreting an extravasation for pathologic findings, false positive lymph node uptake, and “Compton scatter” possibly resulting in misinterpretation of significant breast abnormality (72). Additionally, patients can experience adverse tissue reactions from significant diagnostic extravasations (20, 39, 73).
Database Search
Examining the FAERS database, we found 21 adverse events which included diagnostic radiopharmaceutical extravasation as a factor, despite the NRC's reporting exemption in the United States. Table 1 contains a listing of these cases.
Examining the EV database, we found 17 diagnostic radiopharmaceutical extravasation safety reports in Europe as seen in Table 2. For the EEA, seriousness includes: “death,” “life-threatening,” “requires hospitalization/prolongation of hospitalization,” “results in disability/incapacity,” “congenital anomaly/birth defect,” and “other.”
Examples of Patient Management Effects
Between April 1 and September 30, 2019, Carilion Clinic enrolled 1,023 patients. We identified 34 cases of extravasation (3.3%). Of these, 11 were assessed to be moderate to significant based on TAC data and visual assessment of the images. We estimated that all 11 of these patients had more than 5% of their injected activity extravasated. Three of the 11 patients were asked to provide consent to publish their extravasated and non-extravasated images and results.
For University of Tennessee Medical Center (UTMC) patients, we performed a retrospective analysis of more than 3,534 patients with monitored injections under an exempt protocol that was reviewed and approved by the University of Tennessee Graduate School of Medicine Institutional Review Board (IRB # 4607). Of those reviewed, 100 imaging studies (2.8%) were determined to have residual radioactivity at the site of injection. Of those, thirteen were moderate to significant based on TAC information and visual image assessment. Two cases were selected for inclusion in this work.
Patient A
A 79-year-old female presented with stage 2B T3N1M0 pancreatic cancer. The tumor arose in the pancreatic head but was felt to be unresectable due to neurovascular involvement. During an FDG-PET/CT scan for staging, the patient experienced a significant radiopharmaceutical extravasation and was reimaged the following day. The patient subsequently received chemoradiation since she was not considered a surgical candidate for tumor resection. Procedure parameters and images are compared in Table 3 and Figure 1.
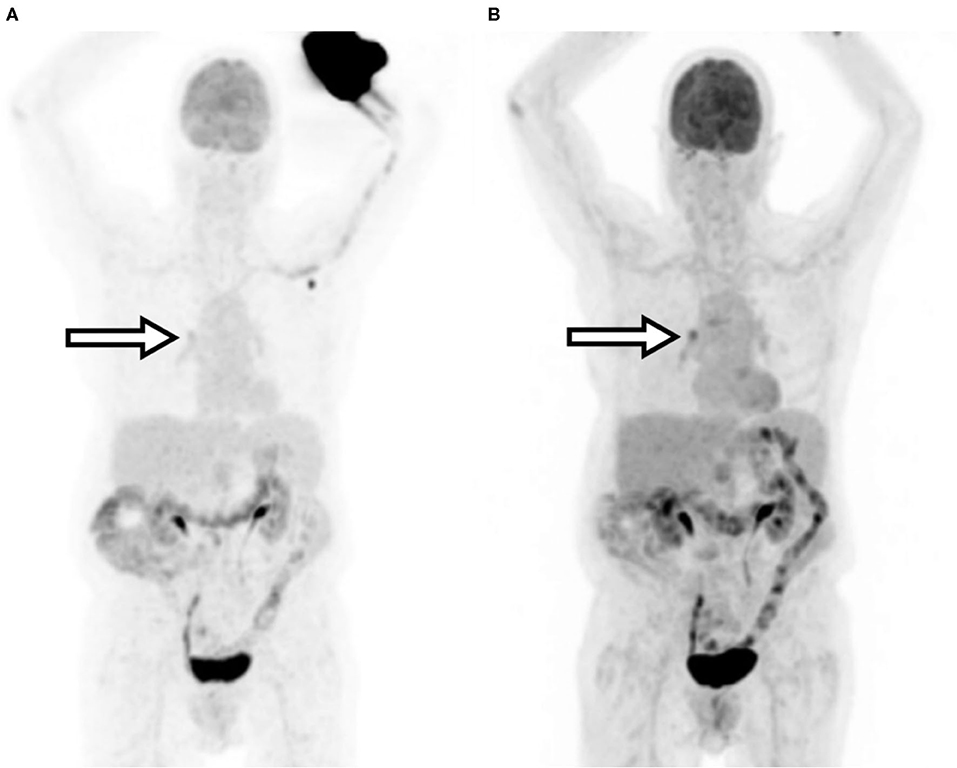
Figure 1. Patient A maximum intensity projection (MIP) images. (A) Extravasated injection. (B) Repeated imaging. Arrows indicate the clinically relevant area of uptake.
Patient B
A 69-year-old female with a history of a T2N2aM0 breast cancer who subsequently developed metastatic skeletal disease. The patient received their first re-staging 18F-FDG PET/CT and was noted to have oligometastatic skeletal metastases. The patient received radiotherapy and then a second re-staging FDG PET/CT 15 weeks later. This second re-staging study was compromised by a significant radiopharmaceutical extravasation and was repeated 3 days later. Procedure parameters and images are compared in Table 4 and Figure 2. The extravasated image SUVmax values were understated by 53–73%.
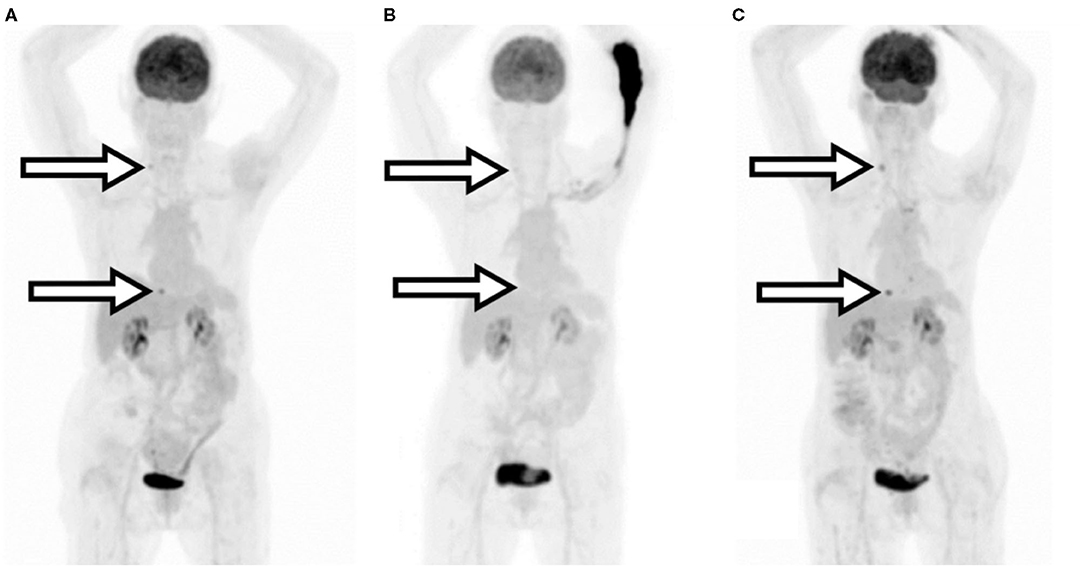
Figure 2. Patient B MIP images. (A) Initial re-staging imaging. (B) Second re-staging extravasated injection. (C) Repeated imaging. Arrows indicate the areas of relevant uptake which were not visible after extravasation.
Patient C
An 81-year-old male presented with a history of clinical stage T1cN0M0 prostate cancer. Post-prostatectomy, the patient had persistently elevated prostate-specific antigen levels and a 99mTc-MDP bone scan was ordered to look for metastatic disease. The bone scan image was deemed non-diagnostic by the radiologist due to significant extravasation and was not interpreted. The study was repeated 2 days later. Benign degenerative disease was noted, but no metastatic bone lesions were found. Procedure parameters and images are compared in Table 5 and Figure 3.
Patient D
An 80-year-old male presented with a history of bladder carcinoma that had metastasized to the liver. Initial follow-up imaging was determined to be non-diagnostic due to a significant extravasation observed on imaging and TAC data. Repeat 18F-FDG PET/CT imaging of the patient was performed the following day. Repeat imaging confirmed disease progression and identified additional uptake not seen in the prior extravasated scan—including an upper liver lesion, increased hilar node activity, and prostate uptake. Quantitative results showed an average increase in SUVmax calculations of ~25%. Procedure parameters and images are compared in Table 6 and Figure 4.
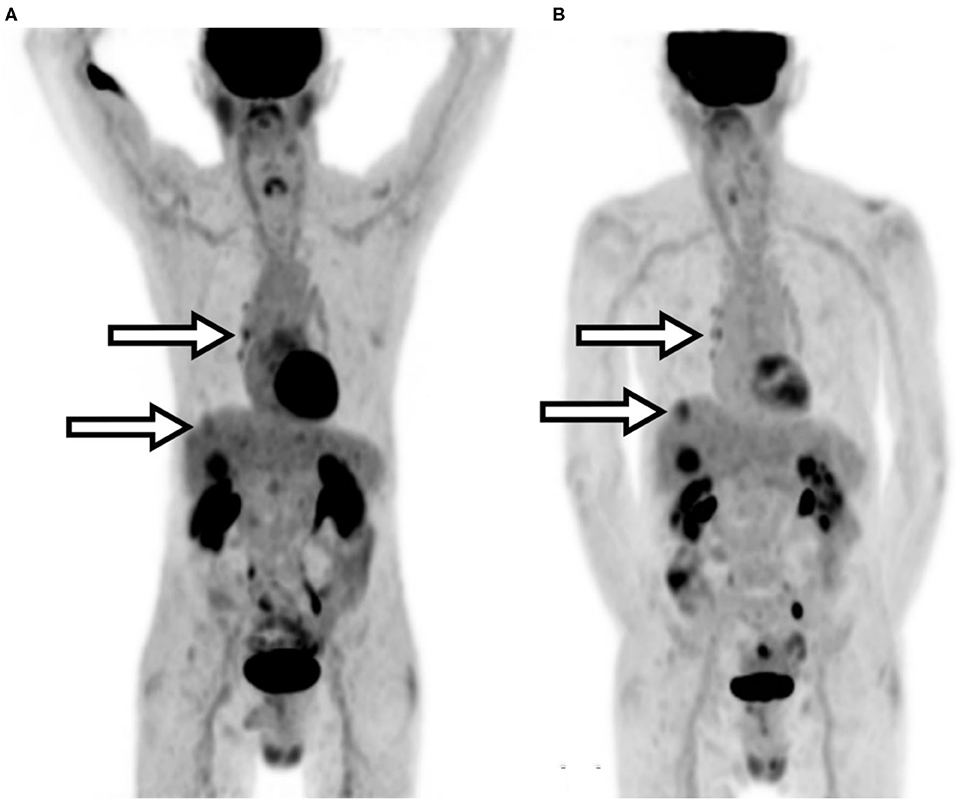
Figure 4. Patient D MIP images. (A) Extravasated injection. (B) Repeated imaging. Arrows indicate the areas of clinically relevant increased uptake.
Patient E
A 61-year-old female presented with a history of breast cancer and malignant right pleural effusion. Follow-up imaging identified possible bone involvement and additional 18F-FDG PET/CT imaging was ordered. The restaging PET/CT images were deemed non-diagnostic due to a significant extravasation. Repeat PET/CT imaging was ordered, and the patient was imaged 5 days later. Repeat imaging showed evidence of a minor extravasation which was supported by TAC data but indicated diffuse metastatic disease with bone involvement—confirming further disease progression. Additionally, the repeated imaging clearly identified a left adrenal lesion that had appeared equivocal on the previous extravasated image and increased SUV values. Procedure parameters and images are compared in Table 7 and Figure 5.
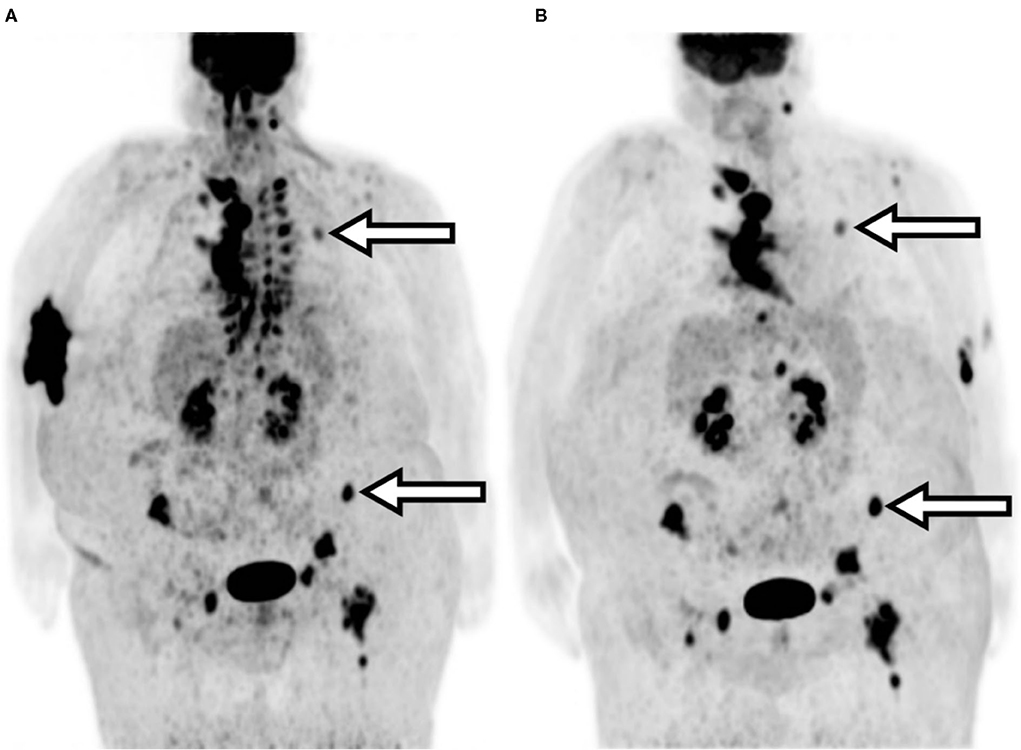
Figure 5. Patient E MIP images. (A) Extravasated injection. (B) Repeated imaging. Arrows indicate areas of relevant uptake between the two scans.
Tissue Dose
Dosimetry results for the five extravasations are shown in Table 8. Skin and tissue effects were not observed post-imaging procedure, as expected due to the latent effects of ionizing radiation on skin and tissue.
Discussion
Reasons for the Commonly Held Hypotheses
Several potential reasons could explain these commonly held hypotheses regarding diagnostic extravasations. In the United States, there are no mandatory requirements to report extravasations. Furthermore, the primary accreditation bodies do not audit the frequency of extravasations. As a result, centers do not routinely follow extravasated patient, nor do they routinely perform dosimetry on extravasated patients. This hesitancy to perform dosimetry may not be exclusive to the United States. In a comprehensive literature review of the consequences of extravasations, van der Pol et al.found that in 3,016 reported cases of diagnostic extravasations, only three cases reported dosimetry and patient follow-up. All three cases resulted in adverse tissue reactions. The authors stated that “lack of clinical follow-up after diagnostic nuclear medicine scans, but also conservative attitude toward reporting and publishing of complications may have possible lead [sic] to under-reporting of skin lesions” (20). Submitted public comments to the NRC also suggest a lack of awareness of the non-penetrating energy emissions associated with routinely used isotopes like 99mTc and 18F. It is often overlooked in standard nuclear medicine practice, that conversion electrons, Auger electrons, low-energy photons, and positrons that result in a minimal dose to tissue when properly administered and circulating in the entire body, can result in extremely high absorbed doses when localized during an extravasation. It also may be easy to summarily dismiss the possibility of localized damage, given the standard assumptions based on whole-body exposures during diagnostic procedures involving ideal radiopharmaceutical administrations. However, low-energy and low-intensity emissions must be considered when calculating dose due to extravasation sites, even if they are routinely ignored in the context of diagnostic nuclear imaging. Many comments note no evidence of harm to patients immediately after an extravasation. These comments indicated a lack of awareness of the typical time required for radiation injury to manifest in patient skin and tissue. Skin reactions can take days and often the skin may not be affected, even when the tissue dose is high. And tissue reactions can take months or years and would not be easily attributed to extravasations, especially when the patient and their treating physician may not be experienced in radiation protection principles.
ACMUI statements are another important reason why these commonly held hypotheses exist. Over 300 public comments regarding the extravasation petition to the NRC refer to the published ACMUI positions. These positions were announced after three separate meetings on extravasations (2008, 2009, and 2019) (3, 74, 75). The ACMUI position in all three cases—extravasations should remain exempted from reporting. However, a detailed review of the transcripts of these meetings reveal that some members understood the patient safety implications, but still recommended retaining the exemption. One ACMUI member when discussing an absorbed dose to tissue of 3–5 Gy (exceeding the 0.5 Sv reporting limit between 6 and 10 times) stated the following: “However, the first thing before us is, should NRC consider it as a medical event. Now if we consider this as a medical event, if we go through all the procedures and identify whatever- 3 or 4 or 5 - the patient will have to be informed; the physician have to be informed, blah blah blah [sic], and then - you have to go into all the reporting mechanisms. And therefore, I am thoroughly against this being reported as a medical event” (76).
Why Review These Hypotheses Now?
As a result of the petition, the NRC is considering evidence on whether diagnostic extravasations can exceed reporting limits. Current NRC reporting requirements in the United States state that a misadministration that results in a dose equivalent to tissue that exceeds 0.5 Sv (over 500× the dose the patient tissue receives during a proper administration) is indicative that an authorized user may be having issues handling radioactive material. Today, if a radiopharmaceutical is spilled on a patient and exposes patient tissue to >0.5 Sv, it is reportable.4 However, if an extravasation results in a tissue dose >0.5 Sv or significantly higher, this is NOT a reportable event and the patient is not required to be informed. Additionally, in Europe there is a new focus on safety and quality regarding diagnostic ionizing radiation. In the European Union, the Commission Staff Working Document on a Strategic Agenda for Medical Ionising Radiation Applications (SAMIRA) has a dedicated effort focused on “Quality and Safety of Medical Radiation Applications” (77). The Commission's objectives are to ensure “that citizens receive the best possible protection from the carcinogenic effects of ionizing radiation, while fully benefiting from the advantages it offers in battling cancer and other diseases. Notwithstanding recent developments in the European regulatory framework, there remains significant room for improvement of the quality and safety of medical radiation applications” (77). The Commission goes on to state that they intend “to launch a European Initiative on quality and safety aiming to ensure that the main diagnostic and therapeutic applications of ionizing radiation in Member States operate in line with high standards for quality and safety, in the interest of patients.”
Another important reason to address the commonly held hypotheses now, is the rapidly growing and important field of theranostics.5 As new therapeutic radiopharmaceuticals prove effective and are approved, administration volume will grow rapidly. This growing volume will result in more and more technologists administering these higher activity therapeutics. As a result, extravasations of therapies could increase. During the initial 44 administrations of Lutathera at a major US medical facility, 6 patients were extravasated (13.6%).6 Additionally, it has never been easier to characterize extravasations than today. A recent article describes using patient-specific biological clearance and reference tissue volumes (5cc) to quickly assess absorbed dose to tissue (7).
Benefits of the New Hypotheses
We believe that once centers recognize that diagnostic radiopharmaceuticals extravasations can matter to patients and can be more easily characterized, patient care will improve, and inadvertent irradiation and wasteful healthcare spending may be reduced. Our findings from the literature and the five clinical case studies presented in this study support our belief. Patient A's lesion SUVmax was understated by 59% due to extravasation. Using the underestimation for comparison with a follow-up study could contribute to an incorrect interpretation of the treatment response. For example, a properly administered injection in a follow-up study could produce a lesion SUV higher than the Day 0 extravasated baseline SUV. The increase in SUV could be interpreted as stable or progressive disease when the proper interpretation may be partial response. Patient B's second restaging results appear to indicate a significant treatment improvement as compared to their first re-staging results; however, results from repeating the extravasated second re-staging procedure suggest stable disease. Patient C, like Patients A and B, required additional imaging and subsequent radiation exposure due to injection extravasation. Patient D showed lesions in the repeat scan that were not readily visible in the initial extravasated study. This may suggest that the extravasation resulted in limited bioavailability of the tracer over time causing a marked change in uptake for that patient. Patient E also showed significant differences in biodistribution between the initial and repeat imaging studies.
As precision medicine and quantification continues to become more important in the future, as ALARA principles grow more important, and as lower radiation doses are used in imaging, it is critical to reduce the frequency and severity of extravasation. Patient care and the field of nuclear medicine will benefit by reducing these misadministrations. While some questions remain unanswered about extravasations and while reporting of significant extravasations will involve some additional burden until extravasation rates are reduced, the recent advances in monitoring, detecting, and performing dosimetry justify this additional work to maximize the safety of our patients.
There were limitations to our work. Based on language skills and familiarity with the EV and FAERS databases, we only accessed two surveillance system databases. While they are a valuable source of information, surveillance systems do have limitations. By searching for certain terms, we only captured a sample of the available data. Our searches were also limited to information from submitted reports, likely due to the same conservative attitude toward reporting of extravasations noted by van der Pol et al. (20). The data available through these searches may comprise only a part of the surveillance data and do not necessarily confirm a causal relationship between the radiopharmaceutical and the reported adverse event. With respect to our local patient data, in our attempts to assess the impact of extravasations on patient management, efforts were made to minimize imaging parameter differences between procedures. While significant efforts were taken to ensure consistency of equipment and biological factors between scans, these patients were not part of a rigorous test-retest imaging protocol. As a result, some of the quantification differences may be attributable to factors other than extravasation. Additionally, because patient review was retrospective, we have no insight on possible adverse tissue and skin reactions. Future prospective work should include long-term patient follow-up to address radiation latent effects.
Conclusions
Our findings suggest that significant extravasations can or have caused patient harm and can irradiate patients' tissue with doses that exceed medical event reporting limits and deterministic effect thresholds (78). In these cases, reports should be made, a patient follow-up plan should be devised, and the event should be analyzed with a goal toward reducing extravasations in the future. Further study is needed to fully assess the impact of significant nuclear medicine extravasations on patient imaging, dose to tissue, and possible patient harm.
Data Availability Statement
The original contributions presented in the study are included in the article/supplementary material, further inquiries can be directed to the corresponding author/s.
Ethics Statement
For Carilion Clinic, ethics committee approval was not necessary for this work. All participants consented to participation in the investigation. For UTMC the study was performed under an exempt protocol (IRB # 4607). All participants provided either written or telephone consent for their de-identified data to be used for teaching and publication purposes or were exempted from providing consent by the local institutional review board. Consent was documented in the patient files.
Author Contributions
DO and JK contributed by obtaining, analyzing, interpreting patient data, and preparation of the manuscript. RL contributed to data analysis, interpretation, and preparation of the manuscript. JK contributed to data analysis, interpretation, and algorithm development. TB contributed to data collection, data analysis, interpretation, and preparation of the manuscript. IB contributed to data analysis and preparation of the manuscript. YF contributed by analyzing, interpreting patient data, and preparation of the manuscript. All authors contributed to manuscript review and editing and have read and approved the final manuscript.
Conflict of Interest
RL, JK, TB, and IB are employed by the company Lucerno Dynamics LLC, the manufacturer of the Lara System described in this manuscript.
The remaining authors declare that the research was conducted in the absence of any commercial or financial relationships that could be construed as a potential conflict of interest.
Abbreviations
ACMUI, Advisory Committee on the Medical Use of Isotopes; EEA, European Economic Area; EV, EudraVigilance; FAERS, FDA's Adverse Event Reporting System; FDA, Food and Drug Administration; FDG, Fluorodeoxyglucose; Gy, Gray; IRB, Institutional Review Board; MDP, Methylene diphosphonate; MIP, Maximum intensity projection; mSv, Millisievert; NRC, Nuclear Regulatory Commission; PET/CT, Positron Emission Tomography/Computed Tomography; rem, Roentgen equivalent man; SPECT, Single Photon Emission Computed Tomography; SUV, Standardized Uptake Value; Sv, Sievert; TAC, Time-activity curve.
Footnotes
1. ^Available online at: https://www.regulations.gov/comment/NRC-2020-0141-0444 (accessed March 1, 2021).
2. ^Available online at: https://www.regulations.gov/comment/NRC-2020-0141-0428 (accessed March 1, 2021).
3. ^Available online at: https://www.regulations.gov/document/NRC-2020-0141-0137 (accessed March 1, 2021).
4. ^NRC Event Number 53434. Available online at: https://www.nrc.gov/reading-rm/doc-collections/event-status/event/2018/20180611en.html (accessed March 1, 2021).
5. ^Available online at: https://www.globenewswire.com/news-release/2020/10/26/2114163/0/en/Global-Theranostics-Markets-Report-2020-Oncology-Segment-Market-for-Theranostics-Should-Grow-from-73-1-Billion-in-2020-to-119-7-Billion-by-2025.html (accessed March 1, 2021).
6. ^Available online at: https://ons.confex.com/ons/2019/cp/eposter.cgi?eposterid=442 (accessed March 1, 2021).
References
1. Siegel JA. Guide for Diagnostic Nuclear Medicine. Reston, VA: Society of Nuclear Medicine (2002).
2. Helm RE, Klausner JD, Klemperer JD, Flint LM, Huang E. Accepted but unacceptable: peripheral IV catheter failure. J Infus Nurs. (2015) 38:189–203. doi: 10.1097/NAN.0000000000000100
3. ACMUI. ACMUI Subcommittee on Extravasation, Final Report. Washington, DC: US Nuclear Regulatory Commission (2019).
4. Food and Drug Administration. Adverse Event Reporting System (FAERS) Public Dashboard. (2019). Available online at: https://fis.fda.gov/sense/app/d10be6bb-494e-4cd2-82e4-0135608ddc13/sheet/7a47a261-d58b-4203-a8aa-6d3021737452/state/analysis (accessed September 24, 2019).
5. Agency EM. EudraVigilance - European Database of Suspected Adverse Durg Reaction Reports. (2019). Available online at: http://www.adrreports.eu/en/search.html# (accessed September 27, 2019).
6. Health C. FDA-Approved Radiopharmaceuticals. (2018). Available online at: https://www.cardinalhealth.com/content/dam/corp/web/documents/fact-sheet/cardinal-health-fda-approved-radiopharmaceuticals.pdf (accessed March 22, 2021).
7. Osborne D, Kiser JW, Knowland J, Townsend D, Fisher DR. Patient-specific extravasation dosimetry using uptake probe measurements. Health Phys. (2021) 120:339–43. doi: 10.1097/HP.0000000000001375
8. Breen SL, Dreidger AA. Radiation injury from interstitial injection of iodine-131-iodocholesterol. J Nucl Med. (1991) 32:892.
9. Williams G, Palmer MR, Parker JA, Joyce R. Extravazation of therapeutic yttrium-90-ibritumomab tiuxetan (zevalin): a case report. Cancer Biother Radiopharm. (2006) 21:101–5. doi: 10.1089/cbr.2006.21.101
10. Bonta DV, Halkar RK, Alazraki N. Extravasation of a therapeutic dose of 131I-metaiodobenzylguanidine: prevention, dosimetry, and mitigation. J Nucl Med. (2011) 52:1418–22. doi: 10.2967/jnumed.110.083725
11. Terwinghe C, Binnebeek SV, Bergans N, Haustermans K, Van Custem E, Verbruggen A, et al. Extravasation of Y-DOTATOC : case report and discussion of potential effects, remedies and precautions in PRRT. Eur J Nuclear Med Mol Imaging. (2012) 39:155–303. doi: 10.1007/s00259-012-2221-x
12. Kawabe J, Higashiyama S, Kotani K, Yoshida A, Tsushima H, Yamanaga T, et al. Subcutaneous extravasation of Sr-89: Usefulness of bremsstrahlung imaging in confirming Sr-89 extravasation and in the decision making for the choice of treatment strategies for local radiation injuries caused by Sr-89 extravasation. Asia Ocean J Nucl Med Biol. (2013) 1:56–9.
13. Tylski P, Vuillod A, Goutain-Majorel C, Jalade P. Abstract 58, dose estimation for an extravasation in a patient treated with 177Lu-DOTATATE. Phys. Med. (2018) 56:32–3. doi: 10.1016/j.ejmp.2018.09.071
15. Hamby DM, Mangini CD. VARSKIN 6: A Computer Code for Skin Contamination Dosimetry (NUREG/CR-6918, Rev. 3). Corvallis, OR: U.S. Government Publishing Office (2018).
16. Bogsrud TV, Lowe VJ. Normal variants and pitfalls in whole-body PET imaging with 18F FDG. Appl Radiol. (2006) 35:16–30.
17. Sonoda LI, Ghosh-Ray S, Sanghera B, Dickson J, Wong WL. FDG injection site extravasation: potential pitfall of misinterpretation and missing metastases. Clin Nucl Med. (2012) 37:1115–6. doi: 10.1097/RLU.0b013e318266cbdb
18. Ozdemir E, Poyraz NY, Keskin M, Kandemir Z, Turkolmez S. Hot-clot artifacts in the lung parenchyma on F-18 fluorodeoxyglucose positron emission tomography/CT due to faulty injection techniques: two case reports. Korean J Radiol. (2014) 15:530–3. doi: 10.3348/kjr.2014.15.4.530
19. Schaefferkoetter JD, Osman M, Townsend DW. The importance of quality control for clinical PET imaging. J Nucl Med Technol. (2017) 45:265–6. doi: 10.2967/jnmt.117.198465
20. van der Pol J, Voo S, Bucerius J, Mottaghy FM. Consequences of radiopharmaceutical extravasation and therapeutic interventions: a systematic review. Eur J Nucl Med Mol Imaging. (2017) 44:1234–43. doi: 10.1007/s00259-017-3675-7
21. Bennett P. Dose Infiltration. (2018). Available online at: https://www.instagram.com/p/BespZWGjUxy/?hl=en&taken-by=nuclear_radiology (accessed March 22, 2021).
22. Bennett PA, Mintz A, Perry B, Trout A, Vergara-Wentland P. Specialty Imaging: PET Positron Emission Tomography With Correlative CT and MR. Philadelphia, PA: Elsevier (2018).
23. Kiser JW, Crowley JR, Wyatt DA, Lattanze RK. Impact of an 18F-FDG PET/CT radiotracer injection infiltration on patient management – a case report. Front Med. (2018) 5:143. doi: 10.3389/fmed.2018.00143
24. Penney HF, Styles CB. Fortuitous lymph node visualization after interstitial injection of Tc-99m-MDP. Clin Nucl Med. (1982) 7:84–85. doi: 10.1097/00003072-198202000-00012
25. Vieras F. Serendipitous lymph node visualization during bone imaging. Clin Nucl Med. (1986) 11:434. doi: 10.1097/00003072-198606000-00018
26. Wallis JW, Fisher S, Wahl RL. 99Tcm-MDP uptake by lymph nodes following tracer infiltration: clinical and laboratory evaluation. Nucl Med Commun. (1987) 8:357–63. doi: 10.1097/00006231-198705000-00006
27. Dogan AS, Rezai K. Incidental lymph node visualization on bone scan due to subcutaneous infiltration of Tc-99m MDP. A potential for false positive interpretation. Clin Nucl Med. (1993) 18:208–9. doi: 10.1097/00003072-199303000-00004
28. Peller PJ, Ho VB, Kransdorf MJ. Extraosseous Tc-99m MDP uptake: a pathophysiologic approach. Radiographics. (1993) 13:715–34. doi: 10.1148/radiographics.13.4.8356264
29. Ongseng F, Goldfarb CR, Finestone H. Axillary lymph node uptake of technetium-99m-MDP. J Nucl Med. (1995) 36:1797–9.
30. Andrich MP, Chen CC. Bone scan injection artifacts. Clin Nucl Med. (1996) 21:260–2. doi: 10.1097/00003072-199603000-00021
31. Shih WJ, Wierzbinski B, Magoun S. Lymph node visualization in the elbow region. J Nucl Med. (1996) 37:1913.
32. Slavin JDJr, Jung WK, Spencer RP. False-positive renal study with Tc-99m DTPA caused by infiltration of dose. Clin Nucl Med. (1996) 21:978–80. doi: 10.1097/00003072-199612000-00019
33. Shih WJ, Han JK, Coupal J, Wierzbinski B, Magoun S, Gross K. Axillary lymph node uptake of Tc-99m MIBI resulting from extravasation should not be misinterpreted as metastasis. Ann Nucl Med. (1999) 13:269–71. doi: 10.1007/BF03164904
34. Shih WJ, Collins J, Kiefer V. Visualization in the ipsilateral lymph nodes secondary to extravasation of a bone-imaging agent in the left hand: a case report. J Nucl Med Technol. (2001) 29:154–5.
35. Pitman AG, Binns DS, Ciavarella F, Hicks RJ. Inadvertent 2-deoxy-2-[18F]fluoro-D-glucose lymphoscintigraphy: a potential pitfall characterized by hybrid PET-CT. Mol Imaging Biol. (2002) 4:276–8. doi: 10.1016/S1536-1632(02)00013-6
36. Chiang SB, Rebenstock A, Guan L, Burns J, Alavi A, Zhuang H. Potential false-positive FDG PET imaging caused by subcutaneous radiotracer infiltration. Clin Nucl Med. (2003) 28:786–8. doi: 10.1097/01.rlu.0000082677.96653.22
37. Stauss J, Treves ST, Connolly LP. Lymphatic Tc-99m DMSA localization after partial-dose extravasation. Clin Nucl Med. (2003) 28:618–9. doi: 10.1097/00003072-200307000-00026
38. Farsad M, Ambrosini V, Nanni C, Castellucci P, Boschi S, Rubello D, et al. Focal lung uptake of 18F-fluorodeoxyglucose (18F-FDG) without computed tomography findings. Nucl Med Commun. (2005) 26:827–30. doi: 10.1097/01.mnm.0000175786.27423.42
39. Vallabhajosula S, Killeen RP, Osborne JR. Altered biodistribution of radiopharmaceuticals: role of radiochemical/pharmaceutical purity, physiological, and pharmacologic factors. Semin Nucl Med. (2010) 40:220–41. doi: 10.1053/j.semnuclmed.2010.02.004
40. Long NM, Smith CS. Causes and imaging features of false positives and false negatives on (18)F-PET/CT in oncologic imaging. Insights Imaging. (2011) 2:679–98. doi: 10.1007/s13244-010-0062-3
41. Manohar K, Agrawal K, Bhattacharya A, Mittal BR. New axillary lymph nodal F-18 fluoro-deoxy glucose uptake in an interim positron emission tomography scan - not always a sign of disease progression. Indian J Nucl Med. (2011) 26:192–3. doi: 10.4103/0972-3919.106703
42. Wagner T, Brucher N, Julian A, Hitzel A. A false-positive finding in therapeutic evaluation: hypermetabolic axillary lymph node in a lymphoma patient following FDG extravasation. Nucl Med Rev Cent East Eur. (2011) 14:109–11. doi: 10.5603/NMR.2011.00025
43. Liu Y. Fluorodeoxyglucose uptake in absence of CT abnormality on PET-CT: What is it? World J Radiol. (2013) 5:460–7. doi: 10.4329/wjr.v5.i12.460
44. Giron J, Lacout A, Marcy P.-Y. Accuracy of positron emission tomography may be improved when combined with postcontrast high-resolution computed tomography scanIn Regard to Pepek et al. Pract Radiat Oncol. (2014) 5:e549–50. doi: 10.1016/j.prro.2014.12.008
45. Simpson DL, Bui-Mansfield LT, Bank KP. FDG PET/CT: artifacts and pitfalls. Contemporary Diagnostic Radiol. (2017) 40:108. doi: 10.1097/01.CDR.0000513008.49307.b7
46. Agency IAE. The Role of PET/CT in Radiation Treatment Planning for Cancer Patient Treatment. (2008). Available online at: https://www-pub.iaea.org/books/iaeabooks/8016/The-Role-of-PET-CT-in-Radiation-Treatment-Planning-for-Cancer-Patient-Treatment (accessed March 22, 2021).
47. Weber WA. Use of PET for monitoring cancer therapy and for predicting outcome. J Nucl Med. (2005) 46:983–95.
49. Hall N, Zhang J, Reid R, Hurley D, Knopp M. Impact of FDG extravasation on SUV measurements in clinical PET/CT. Should we routinely scan the injection site? J Nucl Med. (2006) 47:115P.
50. Teymouri C, Botkin C, Osman M. FDG dose extravasation in PET/CT: frequency and impact on SUV measurements. J Nuclear Med. (2007) 48:475P.
51. Fernolendt H, Bundschuh R, Winter A, Scheidhauer K, Schwaiger M. Paravenous activity in PET/CT – Influence on SUV and correction. J Nuclear Med. (2008) 49:416P.
52. Boellaard R. Standards for PET image acquisition and quantitative data analysis. J Nuclear Med. (2009) 50 (Suppl. 1):11S−20S. doi: 10.2967/jnumed.108.057182
53. Kelly M. SUV: Advancing Comparability and Accuracy. (2009). Available online at: https://www.mpcphysics.com/documents/SUV_Whitepaper_Final_11.17.09_59807428_2.pdf (accessed March 22, 2021).
54. Agency IAE. IAEA Human Health Series No. 27. PET/CT Atlas on Quality Control and Image Artefacts. Vienna, Austria: IAEA (2014). Available: https://www.iaea.org/publications/10424/pet/ct-atlas-on-quality-control-and-image-artefacts (accessed March 22, 2021).
55. Ghesani M, Ghesani N, DePuey EG, Kashefi A, Zhang YC. Nuclear Medicine: A Case-based Approach. New York, NY: Jaypee Brothers Medical Publishers (2016).
56. Lee JJ, Chung JH, Kim S.-Y. Effect of (18)F-fluorodeoxyglucose extravasation on time taken for tumoral uptake to reach a plateau: animal and clinical PET analyses. Ann Nucl Med. (2016) 30:525–33. doi: 10.1007/s12149-016-1090-y
57. Lee JJ, Chung JH, Kim S.-Y. Effect of extravasation on optimal timing of oncologic FDG PET. J Nuclear Med. (2016) 57:1413.
58. Burrell S, MacDonald A. Artifacts and pitfalls in myocardial perfusion imaging. J Nucl Med Technol. (2006) 34:193–211.
59. Erthal L, Erthal F, Beanlands RSB, Ruddy TD, deKemp RA, Dwivedi G. False-positive stress PET-CT imaging in a patient with interstitial injection. J Nucl Cardiol. (2017) 24:1447–50. doi: 10.1007/s12350-016-0634-9
60. Murthy LV, Bateman TM, Beanlands RS, Berman DS, Borges-Neto S, Chareonthaitawee P, et al. Clinical quantification of mycardial blood flow using PET: joint position paper of the SNMMI cardiovascular council and the ASNC. J Nuc Med. (2018) 59:269–97. doi: 10.2967/jnumed.117.201368
61. Qutbi M. Masking effect of radiopharmaceutical dose extravasation during injection on myocardial perfusion defects during SPECT myocardial perfusion imaging: a potential source of false negative result. Mol Imaging Radionucl Ther. (2018) 27:141–3. doi: 10.4274/mirt.88942
62. Waxman AD, Herholz K, Lewis DH, Herscovitch P, Minoshima S, Ichise M, et al. Society of Nuclear Medicine Procedure Guideline for FDG PET Brain Imaging. (2009). Available online at: http://snmmi.files.cms-plus.com/docs/Society%20of%20Nuclear%20Medicine%20Procedure%20Guideline%20for%20FDG%20PET%20Brain%20Imaging.pdf (accessed March 22, 2021).
63. Minoshima S, Drzezga AE, Barthel H, Bohnen N, Djekidel M, Lewis DH, et al. SNMMI procedure standard/EANM practice guideline for amyloid PET imaging of the brain 1.0. J Nucl Med. (2016) 57:1316–22. doi: 10.2967/jnumed.116.174615
64. Fleming JS, Zivanovic MA, Blake GM, Burniston M, Cosgriff PS. Guidelines for the measurement of glomerular filtration rate using plasma sampling. Nucl Med Commun. (2004) 25:759–69. doi: 10.1097/01.mnm.0000136715.71820.4a
65. Murray AW, Barnfield MC, Waller ML, Telford T, Peters AM. Assessment of glomerular filtration rate measurement with plasma sampling: a technical review. J Nucl Med Technol. (2013) 41:67–75. doi: 10.2967/jnmt.113.121004
66. Ponto JA. Preparation and Dispensing Problems Associated With Technetium Tc-99m Radiopharmaceuticals. (2004). Available online at: https://pharmacyce.unm.edu/nuclear_program/freelessonfiles/Vol11Lesson1.pdf (accessed March 22, 2021).
67. European Medicines Agency. DaTSCAN, INN- Ioflupane (123I) Injection Issues. Amsterdam: European Medicines Agency (2004).
68. Alliance QIB. QIBA Profile: Quantifying Dopamine Transporters With 123Iodine Labeled Ioflupane in Neurodegenerative Diseases. Oak Brook, IL: QIBA (2017).
69. Hur S, Bauer A, McMillan N, Krupinski EA, Kuo PH. Optimizing the ventilation–perfusion lung scan for image quality and radiation exposure. J Nuclear Med Technol. (2014) 42:51–4. doi: 10.2967/jnmt.113.128900
70. Mallick S, Petkova D. Investigating suspected pulmonary embolism during pregnancy. Respir Med. (2006) 100:1682–7. doi: 10.1016/j.rmed.2006.02.005
71. Goel S, Bhargava P, Depuey EG. Recognition of dose infiltration on pulmonary ventilation-perfusion scintigraphy. Radiol Case Rep. (2011) 6:562. doi: 10.2484/rcr.v6i4.562
72. Naddaf SY, Collier BD, Elgazzar AH, Khalil MM. Technical errors in planar bone scanning. J Nuclear Med Technol. (2004) 32:148–53.
73. Vano-Galvan S, Rodriguez-Rey C, Vano-Galvan E, Jaen P. Technetium and blood extravasation before gammagraphy: a case report. Cases J. (2009) 2:141. doi: 10.1186/1757-1626-2-141
74. Nuclear Regulatory Commission. Advisory Commitee on the Medical Uses of Isotopes. (2008). Available online at: https://www.nrc.gov/docs/ML0903/ML090340745.pdf (accessed March, 22, 2021).
75. US Nuclear Regulatory Commission (2009). Advisory Committee on the Medical Uses of Isotopes. Washington, DC: Nuclear Regulatory Commission.
76. US Nuclear Regulatory Commission. Official Transcript of Proceedings, Advisory Comittee on the Medical Use of Isotopes. Washington, DC: US Nuclear Regulatory Commission (2009).
77. European Commission. SAMIRA: Strategic Agenda for Medical Ionising Radiation Applications. Brussels: European Commission (2021).
Keywords: extravasation, infiltration, patient harm, adverse events, quality, dosimetry
Citation: Osborne D, Lattanze R, Knowland J, Bryant TE, Barvi I, Fu Y and Kiser JW (2021) The Scientific and Clinical Case for Reviewing Diagnostic Radiopharmaceutical Extravasation Long-Standing Assumptions. Front. Med. 8:684157. doi: 10.3389/fmed.2021.684157
Received: 22 March 2021; Accepted: 02 June 2021;
Published: 28 June 2021.
Edited by:
Francesco Cicone, University of Catanzaro, ItalyReviewed by:
Arnaud Dieudonné, Centre Henri Becquerel Rouen, FranceSusanne Asenbaum-Nan, Lower Landeskliniken-Holding, Austria
Silvano Gnesin, Centre Hospitalier Universitaire Vaudois (CHUV), Switzerland
Copyright © 2021 Osborne, Lattanze, Knowland, Bryant, Barvi, Fu and Kiser. This is an open-access article distributed under the terms of the Creative Commons Attribution License (CC BY). The use, distribution or reproduction in other forums is permitted, provided the original author(s) and the copyright owner(s) are credited and that the original publication in this journal is cited, in accordance with accepted academic practice. No use, distribution or reproduction is permitted which does not comply with these terms.
*Correspondence: Dustin Osborne, RG9zYm9ybmUmI3gwMDA0MDt1dG1jay5lZHU=