- 1Department of Microbiology, College of Medicine, Kosin University, Busan, South Korea
- 2Department of Ophthalmology, College of Medicine, Kosin University, Busan, South Korea
Purpose: To establish in vitro and in vivo ocular co-culture models of Staphylococcus epidermidis and Enterococcus faecalis and to study how various concentrations of moxifloxacin affect the survival of these two endophthalmitis-causing bacteria.
Methods: Standard strains of S. epidermidis and E. faecalis were used. Color detection agar plates were employed to distinguish their colonies. To establish the in vitro and in vivo co-culture models, S. epidermidis and E. faecalis were co-cultivated at different ratios for various periods. For the in vivo model, various volumes and concentrations of either a mono-culture or co-culture were inoculated into the lower conjunctival sac of rabbits. Finally, the newly developed in vitro and in vivo co-culture models were subjected to the moxifloxacin treatment to access its effect on S. epidermidis and E. faecalis.
Results: When S. epidermidis and E. faecalis were cultured separately in tryptic soy broth, their growth peaked and plateaued at approximately 16 and 6 h, respectively. When they were co-cultured, the growth peak of S. epidermidis got delayed, whereas the growth peak of E. faecalis did not change. The number of E. faecalis was significantly higher in the co-culture than that in the mono-culture. Treatment with moxifloxacin in the in vitro co-culture model rapidly decreased the number of S. epidermidis cells at doses ≥ 0.125 μg/ml. In contrast, the number of E. faecalis did not change significantly up to 16 μg/ml moxifloxacin. In in vivo co-culture (at 1:1), the S. epidermidis count decreased in a pattern similar to that seen in in vivo mono-culture and was barely detectable at 24 h after inoculation. In contrast, the of E. faecalis count increased up to 16 h and then decreased. When moxifloxacin was applied (zero, one, or two times) to this model, the S. epidermidis count decreased in proportion to the number of treatments. In contrast, the E. faecalis count increased with moxifloxacin treatment.
Conclusions: The in vitro and in vivo co-culture models of S. epidermidis and E. faecalis were established to determine the influence of moxifloxacin eye drops on these bacteria. The results clearly show that the moxifloxacin eye drops can make E. faecalis dominant on the ocular surface.
Introduction
Cataract surgery is one of the most common surgical procedures worldwide. Owing to advancements in surgical techniques and materials, cataract surgery is now faster and safer (1). Nonetheless, various complications, including posterior capsular tear, loss of endothelial cells, corneal stromal burn, retinal detachment, and postoperative endophthalmitis (PE), still occur (2). Acute PE is a rare prevalence 0.023–0.26% but devastating complication of cataract surgery (3–5).
PE is initiated by the entry of microorganisms into the intraocular space, and the type of invading microorganism is a critical determinant of visual outcomes (6, 7). Because most of the bacteria causing PE come from commensal conjunctival microbes (8), it is important to confirm the presence of certain bacteria on the ocular surface and to monitor changes in this bacterial community.
Recently, it has been reported that the distribution of the causative bacteria of postoperative PE changed in Korea, Sweden, and Taiwan (9–11). These changes may reflect alterations of conjunctival microflora in the PE patients. Nonetheless, it would be difficult to determine the changes in this ocular surface microbial community by human clinical trials because the incidence of endophthalmitis is too low to meet statistical power requirements of a study population. Therefore, we decided to investigate the changes in the causative bacteria of PE by constructing an experimental co-culture model using representative bacteria occurring on the ocular surface. In vitro and in vivo co-culture models based on various microbial species have enabled research into cell-cell interactions among eukaryotic and bacterial species and helped to mimic complex in vivo environment (12).
A healthy and balanced microbial community is essential for maintaining metabolic, physiological, and immunological functions. Commensal microorganisms of the ocular surface provide defense against competitors, by inhibiting the growth of more virulent bacterial strains (13). Nevertheless, a variety of factors, such as age, dry eye, contact lens use, immunocompromising diseases, medications, and environmental factors can shift commensal-microflora composition on the ocular surface (13–16). Such a shift may occur in response to increased use of topical antibiotics intended to prevent ocular infection. Previous studies reporting that the distribution of isolates causing PE is changing from coagulase-negative staphylococci (CNS) to enterococci suggest that external factors have altered the relationship between the two most common bacterial taxa in the conjunctiva (9–11).
Fluoroquinolones are bactericidal antibiotics that directly inhibit bacterial DNA synthesis (17). Topical fluoroquinolones are the prophylactic antibiotics most commonly used in ophthalmic surgery (18). Although no randomized clinical trials of surgical prophylaxis for controlling ocular infection have been conducted, topical fluoroquinolones are routinely employed perioperatively in eye clinics (19). The first three topical fluoroquinolones introduced as topical eye drops were 0.3% ciprofloxacin, 0.3% ofloxacin, and 0.3% norfloxacin. Subsequently, next generation fluoroquinolones, including 0.5% levofloxacin, 0.3% gatifloxacin, and 0.5% moxifloxacin, have been introduced. The most important attribute of a later generation fluoroquinolones is their enhanced activity against gram-positive bacteria relative to that of the previous generation of fluoroquinolones (17). Currently, a moxifloxacin solution, a fourth-generation fluoroquinolone, is the most popular topical eye antibiotic for ophthalmic surgery. Of note, the fluoroquinolones possess high antimicrobial activity against staphylococci, as confirmed in some studies; however, these drugs are known to have poor antimicrobial activity against Enterococcus faecalis (20–23). The selective antimicrobial activity of a fluoroquinolone may have an effect on the alteration of microbial community on the ocular surface and induce changes in causative isolates of PE from coagulase-negative staphylococci to enterococci.
At present, however the experimental studies have not been attempted to elucidate the mechanism underlying the changes in causative bacteria of PE. Here, we hypothesized that the major factor altering of causative isolates of PE is perioperative application of ophthalmic antibiotics such as moxifloxacin to the conjunctiva of patients (24). To test this hypothesis, we constructed in vitro and in vivo co-culture models by representative causative pathogens of PE: S. epidermidis and E. faecalis. Then, the models were subjected to a fluoroquinolone ophthalmic antibiotic, and responses of the two bacterial species to the antibiotic were examined.
Methods
Bacterial Strains and Growth Conditions
Standard strains of E. faecalis (ATCC29212) and S. epidermidis (KCTC1917; equivalent to ATCC12228) were purchased from the American Type Culture Collection and the Korean Collection of Type Cultures, respectively. Both strains were cultured either in tryptic soy broth (TSB) or on tryptic soy agar (TSA) at 37°C. These standard strains were used to set up the in vitro and in vivo co-culture models.
In vitro Mono- and Co-cultures of S. epidermidis and E. faecalis
A single bacterial colony was streaked on a TSA plate and incubated for 24 h at 37°C. Then, colonies were collected and dispersed in TSB, and the suspension was diluted to optical density at 600 nm (OD600) of 0.1. The suspensions of the standard S. epidermidis or E. faecalis strains were prepared at equal concentrations (2.5 × 107 CFU/mL) in 5 ml of TSB.
For the in vitro mono-culture model, each bacterial suspension was incubated for 24 h, and then 100 μl aliquots were collected at 2 h intervals for 12 and at 4 h intervals thereafter. The samples were diluted 1:105 and 1:106 with a 0.85% NaCl solution, and 100 μl of each diluted bacterial suspension was spread on a TSA plate. After incubation for 24 h, the colonies on the plates were counted by means of a colony counter.
For the in vitro co-culture model, the prepared bacterial suspensions of S. epidermidis and E. faecalis (2.5 × 107 CFU/ml each) were mixed in equal volumes and co-cultured for 42 h. The mixture was cultivated in two media in parallel (TSB and PBS). Then, 100 μl of the bacterial suspension was collected at 2 h intervals for 16 h and at 4 h intervals thereafter. The samples were serially diluted, and 100 μl of the diluted suspension was spread on a color detection agar (CDA) plate (MB-C1611; MB-cell, Korea). On such a plate, colonies of E. faecalis and S. epidermidis can be distinguished by color: E. faecalis colonies are blue, whereas S. epidermidis colonies are white. After 24 h of incubation, the colonies of each bacterium were counted using the colony counter.
Cultivation of S. epidermidis and E. faecalis With Various Concentrations of a Culture Supernatant
A single bacterial colony was streaked on a TSA plate and incubated for 24 h at 37°C. After that, colonies were collected and dispersed and diluted in TSB to an OD600 of 0.1. The bacterial suspension was cultivated in a shaking incubator (N-Biotek, South Korea). After cultivation for 24 h, a cell-free culture supernatant was prepared by centrifuging the culture media and passing the supernatant through a 0.2 μm syringe filter (Jet biofil, China). The culture supernatants of E. faecalis and S. epidermidis were next diluted to various concentrations (50, 80, and 100%) with TSB. To measure the effects of a culture supernatant on bacterial growth, 15 μl of the bacterial suspension (2.5 × 107 CFU/mL) and 135 μl of each culture supernatant (separately) were mixed in a 96 well plate and incubated at 37°C with shaking. OD600 was measured on a microplate reader (Infinite M200; Tecan, USA) wih 30 min intervals for 24 h to build a growth curve.
Analysis of the Impact of Moxifloxacin on S. epidermidis and E. faecalis in the in vitro Co-culture Models
S. epidermidis and E. faecalis suspensions were prepared at equal concentrations (2.5 × 107 CFU/ml) in TSB and mixed at a ratio of either 1:1 or 95:5 (S. epidermidis: E. faecalis). The co-culture prepared at the ratio of 1:1 was incubated for 18 h, and 100 μl of the resultant suspension was withdrawn and mixed with 900 μl of TSB containing various concentrations of moxifloxacin (0–16 μg/ml; Tokyo Chemical Industry, Japan), and incubated for 14 h. Next, the suspension was serially diluted and plated on CDA. The co-culture prepared at the ratio of 95:5, S. epidermidis 95 ul and E. faecalis 5 ul, was mixed with TSB (900 ul) containing various concentrations of moxifloxacin and incubated for 18 h. After that, colonies were counted in the same manner as described above.
In vivo Mono- and Co-cultures of S. epidermidis and E. faecalis
New Zealand white rabbits (4–5 kg; Hyochang Science, Korea) were used to establish in vivo mono- and co-culture models of S. epidermidis and E. faecalis infection. The rabbits were anesthetized by intramuscular injection of tiletamine-zolazepam (Virbac Korea, France) and xylazine (Bayer, Germany). Each group consisted of five rabbits, and only the right eye was subjected to the experiment.
Either a prepared bacterial suspension or topical antibiotic solution was inoculated into the lower conjunctival sac (Figures 1A,B). To avoid a loss of the solution, tarsorrhaphy was performed using continuous 6–0 nylon (Ethicon, USA) (Figure 1C). To compare the numbers of bacterial cells among the different treatment groups, the bacteria in the inferior cul-de-sac were collected with a sterile, cotton-tipped swab at different time points (4–24 h) after the inoculation (Figure 1D). The swabs were immediately soaked in 1 ml of normal saline in an Eppendorf tube and shaken at 2,800 rpm for 60 s in a microtube homogenizer (BeadBug, Benchmark Scientific, USA) to extract the bacterial cells from the swabs (Figure 1E). Next, the suspension was serially diluted and plated on CDA for differential colony counting (Figures 1F,G). After an overnight incubation at 37°C, the number of E. faecalis and S. epidermidis colonies were determined as described above (Figure 1H). The study protocol was approved by the Institutional Animal Care and Use Committee of Kosin University College of Medicine (KMAP-18-19). All experiments involving animal subjects were conducted in accordance with the guidelines and regulations of the Association for Research in Vision and Ophthalmology.
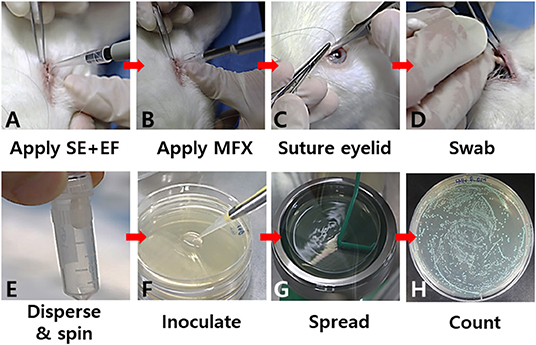
Figure 1. Key steps in the in vivo co-culture model of S. epidermidis (SE) and E. faecalis (EF). A prepared bacterial suspension (A) or a topical antibiotic (moxifloxacin; MFX) (B) was applied to the lower conjunctival sac. To avoid loss of the bacterial suspension, tarsorrhaphy was performed (C). The bacteria in the inferior cul-de-sac were collected with a sterile cotton-tipped swab (D). The bacterial cells on the swabs were immediately dispersed into a medium in an Eppendorf tube (E). Serial dilutions of the bacterial suspension were seeded on a CDA plate for differential colony counting (F,G). After overnight incubation at 37°C, the numbers of colonies were determined (H).
For in vivo mono-cultures of S. epidermidis or E. faecalis, 25 μl of the prepared bacterial suspension (109 CFU/ml) was applied to the lower conjunctival sac. For the in vivo co-culture model of S. epidermidis and E. faecalis, various conditions with different ratios of the two bacteria [1:1, 8:2, or 9:1 (S. epidermidis: E. faecalis)], volumes (50, 25, or 12.5 μl), and concentrations (1 × 109, 2 × 109, or 4 × 109 CFU/ml) were applied. Then, the bacteria were collected at 4, 8, and 16 h after the administration as described above.
Evaluation of the Effects of Topical Moxifloxacin Administration on the in vivo Co-culture Model of S. epidermidis and E. faecalis
To this end, 9 μl of a S. epidermidis suspension (4 × 109 CFU/ml) and 1 μl of an E. faecalis suspension (4 × 109 CFU/ml) were employed. Moxifloxacin (5 μl at 0.5% concentration; Tokyo Chemical Industry Co., Japan) was applied to the lower conjunctival sac once immediately after the inoculation of the bacterial suspension or twice (additional treatment 1 h later). The number of bacterial cells in the lower conjunctiva was determined 4 h after the inoculation of the bacterial solution.
Statistics
All in vitro experiments were conducted three times independently and animal experiments were conducted at least three to five times with a group of five animals. Paired Student's t-test was performed to assess the significance of differences between two groups. All statistical analyses were performed using GraphPad Prism (version 6.03). Statistical significance was defined as a p-value < 0.05.
Results
Growth Curves of in vitro Mono-Cultures and Co-cultures of S. epidermidis and E. faecalis
When S. epidermidis was cultivated alone in TSB, its growth peaked and plateaued at approximately 16 h (Figure 2A). When E. faecalis was cultured alone in TSB, its growth peaked and plateaued at ~6 h, which was faster than that of S. epidermidis. When S. epidermidis and E. faecalis were cocultivated, peak growth of S. epidermidis got delayed to approximately 24 h (Figure 2B). In addition, the concentration of E. faecalis cells was 1.86-fold higher in the co-culture with S. epidermidis than that in mono-culture.
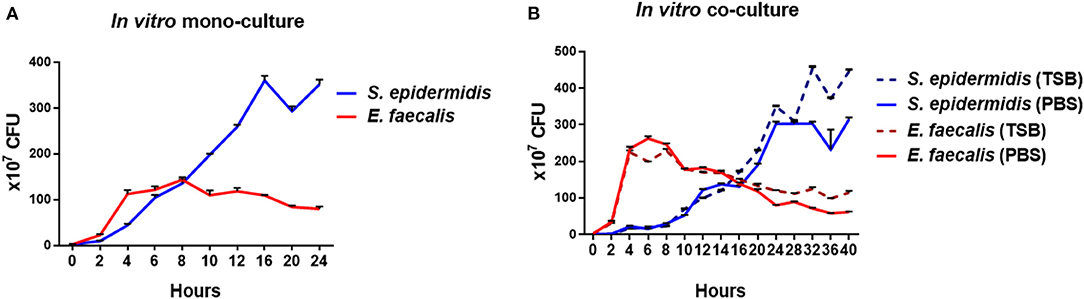
Figure 2. Growth curves of in vitro mono-cultures and co-cultures of S. epidermidis and E. faecalis. For the mono-cultures, S. epidermidis and E. faecalis were cultured separately in TSB, and the numbers of bacterial cells were determined by quantifying CFUs every 2-4 h (A). For the co-cultures, the two bacterial species were mixed at the ratio of 1:1 and cocultivated in either TSB or PBS. The CFUs on the CDA plates were counted every 2-4 h (B).
Growth Patterns of S. epidermidis and E. faecalis Cultivated With Various Concentrations of a Culture Supernatant
To further analyze the difference in the growth patterns between the mono-cultures and co-cultures of S. epidermidis and E. faecalis, these microbes were cultivated alone with various concentrations of a culture supernatant from S. epidermidis or E. faecalis mono-cultures or co-cultures (Figure 3). When E. faecalis was cultured with a supernatant from mono-cultures or co-culture of S. epidermidis or E. faecalis, the proliferation rate decreased in a supernatant concentration-dependent manner (Figures 3A–C, Supplementary Figures 1A-C). In the case of S. epidermidis, when cultivated with a S. epidermidis, E. faecalis, or co-culture supernatant, the initial growth rates (first 4 h) were similar to that of the control except for samples with the 100% supernatant. In contrast, the growth rate of a later phase slowed down, taking a long time to reach the maximum cell concentration (Figures 3D–F, Supplementary Figures 1D-F). Notably, when E. faecalis was cultured with a supernatant from S. epidermidis mono-culture or co-culture, the growth slowdown and the delay of maximum cell concentration shown in Figure 3B were overcome: we noticed a slow increase of cell proliferation over time (Figures 3A,C). In other words, although the maximum cell concentration decreased as the concentration of the supernatant increased, the growth of E. faecalis cultivated with media containing the supernatant of S. epidermidis was found to recover and reached the final OD600 higher than that of the mono-culture in TSB. These results suggested that the S. epidermidis culture contains some substances that induce the increase in the cell concentration of E. faecalis, which may explain the significant increase of maximum cell concentration of E. faecalis in the co-culture with S. epidermidis.
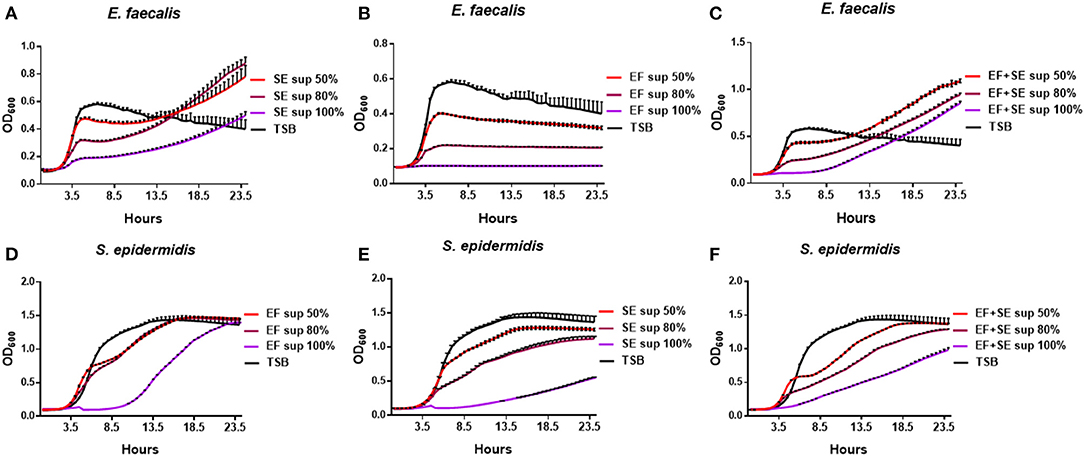
Figure 3. Growth patterns of S. epidermidis (SE) and E. faecalis (EF) cultivated with various concentrations of a culture supernatant (sup). E. faecalis was cultured with media containing a supernatant (0, 50, 80, or 100%) of S. epidermidis mono-culture (A), of E. faecalis mono-culture (B), or of the S. epidermidis + E. faecalis co-culture (C) and the growth rates were measured every 30 min. In the same way, S. epidermidis was cultivated with a supernatant of E. faecalis mono-culture (D), S. epidermidis mono-culture (E), or of the co-culture (F) and growth curves were constructed.
Effects of Moxifloxacin on in vitro Co-cultures of S. epidermidis and E. faecalis
When S. epidermidis and E. faecalis were cocultivated at the ratio of 1:1 or 95:5 for 18 h, the final cellular concentration of the two species was different (Figures 4A,B). The concentrations of S. epidermidis and E. faecalis cells were 1,925 ± 195 × 105 and 338 ± 68 × 105 CFU /ml, respectively, when cocultured at 1:1 (p < 0.0001, Figure 4A) and 408 ± 158 x 105 and 268 ± 55.0 x 105, respectively, when cocultured at 95:5 (p = 0.028, Figure 4B). This difference was similar to the result shown in Figure 2B, in which the numbers of colonies of the cocultured bacteria, mixed in the ratio of 1:1, are compared over time. In the co-culture, the E. faecalis count initially increased sharply at 4–6 h and then decreased, while the growth of S. epidermidis was initially delayed and then was good between 12 and 20 h. For this reason, the 1:1 co-culture had a significantly smaller number of E. faecalis cells than S. epidermidis cells after 18 h. Of note, the numbers of S. epidermidis colonies were markedly different between the 1:1 and 95:5 co-cultures, whereas the numbers of E. faecalis colonies were similar.
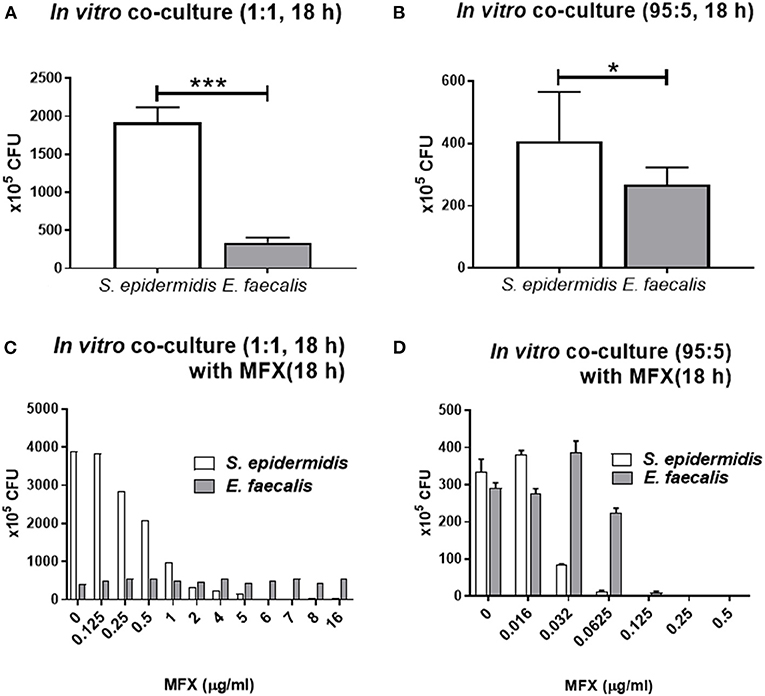
Figure 4. In vitro co-culture modeling of S. epidermidis (SE) and E. faecalis (EF) and their responses of bacteria to moxifloxacin treatment. S. epidermidis and E. faecalis were mixed at the ratio of 1:1 and cocultured for 18 h, and their CFUs were quantified (A). Similarly, a mixture in the 95:5 ratio (SE:EF) was cultivated for 18 h (B). For the co-culture with moxifloxacin (MFX) treatment, the bacterial mixture in the 1:1 ratio was cocultured for 18 h and then treated with various concentrations of moxifloxacin. The CFUs were counted after 14 h moxifloxacin treatment (C). When S. epidermidis and E. faecalis were mixed at the ratio of 95:5, the moxifloxacin treatment was started immediately, without the 18 h culturing period, and the CFUs were counted after 18 h moxifloxacin treatment (D). Means and S.D. (n = 3) are shown as columns and error bars, respectively. Asterisks at the top of bars denote statistically significant differences (*, p < 0.05; ***, p < 0.001; student t-test).
When 1:1 co-cultures were treated with moxifloxacin at various concentrations for 18 h, the numbers of S. epidermidis cells rapidly decreased at 2 μg/ml moxifloxacin, while the numbers of E. faecalis cells, did not significantly change even at a moxifloxacin concentration of 16 μg/ml (Figure 4C). On the contrary, when S. epidermidis and E. faecalis were mixed at 95:5 and immediately treated with moxifloxacin for 18 h (without co-cultivation for 18 h beforehand), the numbers of S. epidermidis colonies stared to decline at 0.032 μg/ml moxifloxacin, and no S. epidermidis colonies were detectable at 0.125 μg/ml. The decrease of E. faecalis colonies were observed at 0.125 μg/ml and no colonies at 0.25 μg/ml (Figure 4D). Therefore, when the two bacteria were cocultured for 18 h before the exposure to moxifloxacin, the resistance to this drug strengthened for bacteria. In particular, E. faecalis co-cultured with S. epidermidis survived with moxifloxacin above 16 μg/ml.
In vivo Co-culture Modeling With Different Volumes and Ratios of S. epidermidis and E. faecalis Cell Suspensions
In the in vivo mono-cultures (25 μl) with the inoculum concentration of 109 CFU/ml, the number of S. epidermidis colonies gradually diminished at every time point, whereas the number of E. faecalis colonies increased until 8 h and then decreased (Figure 5A). When these bacteria were co-cultured, the number of S. epidermidis colonies decreased at every time point and was close to zero at 24 h, whereas the number of E. faecalis increased until 16 h and then decreased (Figure 5B). In comparison to in vivo mono-culture, E. faecalis had higher number of cells for longer time in the in vivo co-culture. When the inoculum concentration was doubled to 2 × 109 CFU/ml by reducing the inoculum volume in half from 25 to 12.5 μl, the number of S. epidermidis colonies were low at 4 h, slightly increased at 8 h and then declined, which differed from the result above, and the E. faecalis count went up until 8 h and then decreased (Figure 5C).
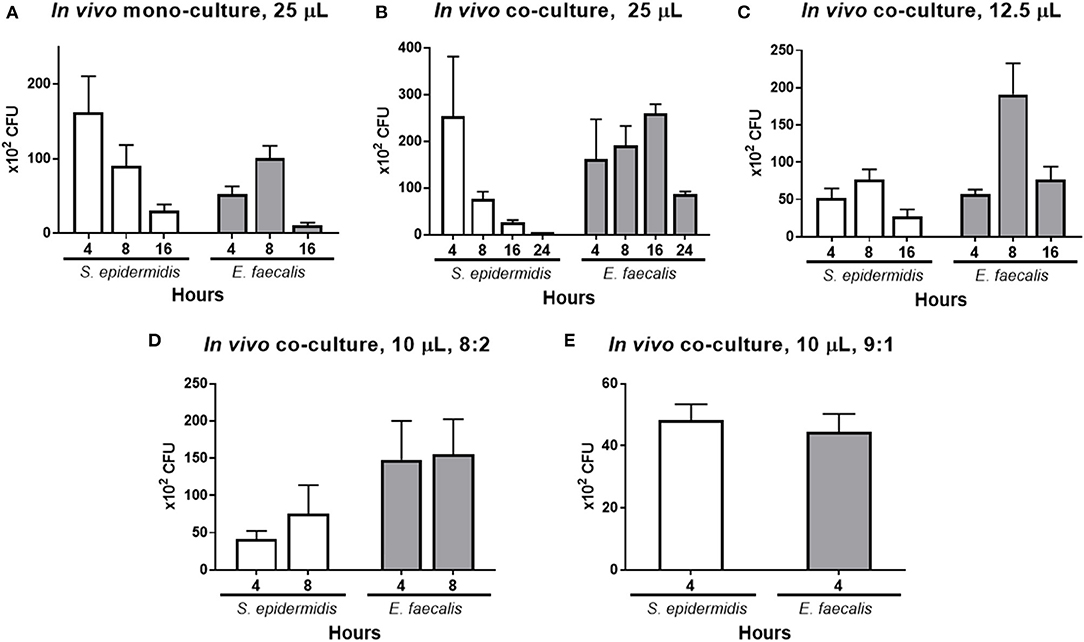
Figure 5. In vivo co-culture modeling and effects of the mixing ratio and volume of the numbers of S. epidermidis (SE) and E. faecalis (EF) cells. As a control, the mono-cultures of each bacterium were inoculated (25 μl of a 1 × 109 CFU/ml suspension) into the lower conjunctival sac of a rabbit, and the bacteria CFUs were quantified at 4, 8, and 16 h post-inoculation (A). Under the same conditions, co-culture inoculum was prepared by mixing the two bacteria at the 1:1 ratio (25 μl with total volume, with a total bacterial concentration of 1 × 109 CFU/ml). After inoculation, the CFUs were counted at 4, 8, 16, and 24 h (B). Half volume with a double-concentrated suspension was prepared by reducing the inoculum volume to 12.5 μl and raising the cell concentration to 2 × 109 CFU/ml. After inoculation, the CFUs were counted at 4, 8, and 16 h (C). Next, the total inoculum volume was reduced to 10 μl at a concentration of 4 × 109 CFU/ml, and different ratios of S. epidermidis to E. faecalis were tested. First, the microbes in the ratio of 8:2 (SE:EF) were inoculated, and the CFUs were counted at 4 and 8 h after the inoculation (D). Finally, the bacteria in the ratio of 9:1 (SE:EF) were inoculated and the CFU were counted at 4 h after inoculation (E), and this ratio was chosen for the test of moxifloxacin treatment. Means and S.D. (n = 3) are shown as columns and error bars, respectively.
In order to implement the antibiotic treatment, the volume of the suspension of inoculated bacteria was reduced further, and the ratio of the bacteria cells was adjusted. The total volume of the bacterial inoculum was reduced to 10 μl (4 × 109 CFU/ml), and the ratios of S. epidermidis and E. faecalis cells were 8:2 and 9:1 (Figures 5D,E). When the ratio was 8:2, the S. epidermidis count increased until 8 h, whereas the E. faecalis count was stable for 8 h. When the initial ratio was 9:1, the numbers of S. epidermidis and E. faecalis cells were almost identical at 4 h.
Survival of in vivo Co-cultures After Moxifloxacin Treatment
The effects of moxifloxacin eye drops were tested on the in vivo co-culture model of S. epidermidis and E. faecalis. These microbes (4 × 109 CFU/ml each) were inoculated (9 and 1 μl, respectively), and moxifloxacin was administered zero, one, or two times. The numbers of S. epidermidis and E. faecalis colonies were determined at 4 h post inoculation (Figure 6). The number of S. epidermidis colonies diminished significantly as the number of moxifloxacin treatment increased. In contrast, the number of E. faecalis colonies remained constant, regardless of the moxifloxacin administration scheme. Moreover, when moxifloxacin was administered once, the number of E. faecalis colonies tended to go up.
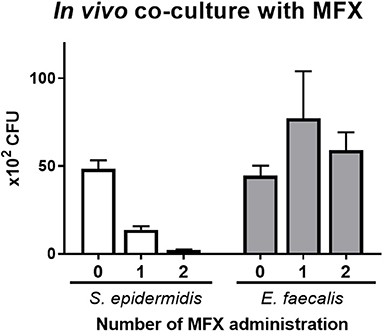
Figure 6. The influence of moxifloxacin on the in vivo co-culture model of S. epidermidis (SE) and E. faecalis (EF). A bacterial suspension was prepared in the ratio of 9:1 (SE:EF) in a total volume of 10 μl (4 × 109 CFU/ml) and was inoculated into a lower conjunctival sac of rabbits. A moxifloxacin solution (5 μl at a 0.5% concentration) was administered zero, one, or two times. The first treatment with moxifloxacin was administered immediately after the bacterial suspension inoculation, and the second treatment was performed 1 h later. The CFUs were counted at 4 h after bacterial inoculation. Means and S.D. (n = 3) are shown as bars and error bars, respectively.
Discussion
In this study, we developed in vitro and in vivo co-culture models of S. epidermidis and E. faecalis to evaluate the impact of moxifloxacin on the survival of these two bacteria. E. faecalis has significantly higher resistance to moxifloxacin than S. epidermidis does, and administration of moxifloxacin likely allows for selective survival of E. faecalis on the ocular surface. The in vitro and in vivo co-culture models clearly showed that moxifloxacin treatment reduce the number of S. epidermidis but not the number of E. faecalis. These suggest that if there is a small number of E. faecalis cells in the conjunctiva, then administration of moxifloxacin promotes not only the selective survival of E. faecalis but also its domination in conjunctival sac microflora.
Recently, administration of prophylactic antibiotics before and after intraocular surgery became a common practice, even though there is no clear evidence that it prevents infection. If fluoroquinolone is utilized as a prophylactic antibiotic perioperatively, it is expected to be effective against S. epidermidis present on the ocular surface but not against E. faecalis, hence changing conjunctiva microflora. The changed conjunctiva microflora provides more opportunities for E. faecalis to enter the intraocular space through the surgical wounds, resulting PE.
In our study, in vitro co-culture of S. epidermidis and E. faecalis increased the maximum cell concentration of E. faecalis in contrast to the mono-cultures. The different growth patterns between mono- and co-cultures is likely related to direct co-culture of the two bacteria. In other words, it is highly likely that the proliferation of E. faecalis is induced in the presence of S. epidermidis. To explain this difference, the bacteria were cultivated with various concentrations of a culture supernatant from each bacterial species. The experiments using culture supernatants have many limitations to represent the direct co-culture condition. For example, using the culture supernatant of stationary phase may exclude some components of exponential phase, and the nutrient-reduced culture supernatant may hinder normal growth of bacteria. The supernatant of stationary phase rather than exponential phase was chosen because we thought that bacterial growth stage in the in vivo condition, where bacteria inhabit on the ocular surface, may be closer to the stationary phase than exponential phase. When the supernatant of stationary phase is used, the effect of nutrient reduction can be an issue. When 0, 50, 80, 100% of supernatant with culture media TSB was used, the effect of nutrient-reduced supernatant to the bacterial growth was shown (Figure 3). However, the purpose of this experiment is to observe the changes in the growth pattern of bacteria affected by bacterial culture supernatant. For this purpose, we were able to observe that the supernatant of S. epidermidis culture induced the increase of the cell density of E. faecalis above their maximum cell concentration in the standard culture.
The growth patterns shown in the in vitro co-culture model were also observed in the in vivo co-culture model. When E. faecalis and S. epidermidis were administered to the lower conjunctival sac of rabbits, the number of S. epidermidis cells decreased with time, whereas the number of E. faecalis cells increased for up to 16 h. These data suggest that if E. faecalis enters the conjunctival sac before or after intraocular surgery, it is likely to proliferate in the conjunctival sac for at least 16 h. Therefore, great care should be taken not to contaminate the ocular surface with the bacteria before and after intraocular surgery.
We next sought to determine whether there is a change in antibiotic sensitivity when the two bacteria were co-cultured. When the bacteria were mixed (at a ratio of 95:5) and immediately treated with moxifloxacin, without a co-culturing period, no S. epidermidis colonies were detectable in the presence of 0.125 μg/ml moxifloxacin, and there were no E. faecalis colonies in the presence of 0.25 μg/ml moxifloxacin. Of note, when S. epidermidis and E. faecalis were mixed (at a ratio of 1:1), co-cultured for 14 h and only then treated with moxifloxacin, the antibiotic concentration effective against S. epidermidis was much higher, 6 μg/ml and, moreover, E. faecalis became resistant even to 16 μg/ml moxifloxacin. This phenomenon was observed in the in vivo co-culture model too. In the in vivo model, when 0.5% moxifloxacin was administered twice with an 1 h interval, S. epidermidis almost disappeared, but E. faecalis persisted. These findings also imply that not only in the conjunctival sac but also in the cases of endophthalmitis, where the bacteria co-proliferate in the vitreous cavity, E. faecalis may become more resistant to fluoroquinolone antibiotics.
Fluoroquinolone eye drops are widely used before and after intraocular surgery to prevent PE. It is still debatable whether these antibiotics reduces the risk of infectious endophthalmitis. Although these antibiotics are very likely to effectively eliminate CNS, including S. epidermidis, in the conjunctival microflora, our data suggest that moxifloxacin may have little antimicrobial activity toward the Enterococcus spp. that are present in the conjunctiva.
Most of the bacteria that cause PE are those already present in the conjunctiva. Because the most prevalent bacteria in the conjunctiva are CNS, including S. epidermidis, they have been the major causative agent of PE after surgery. On the other hand, several recent reports on PE in Korea, Sweden, and Taiwan indicated that E. faecalis has become the top causative agent of PE (6, 9–11). Enterococcus spp. have been relatively rare but are of special clinical importance because they cause a fulminant and destructive disease course, and poor visual outcomes of infected patients (7, 25–27). In one study, only 17% of patients with E. faecalis endophthalmitis achieved a final visual acuity of 20/400 or better (28). Therefore, the emergence of E. faecalis as a cause of PE may affect visual outcomes after treatment of PE.
This reshuffling of major causative isolates of PE is especially obvious in a series of nationwide, multicenter, prospective studies in Sweden. In the first report, covering the 1998 national prospective survey, the most common bacteria that caused PE were CNS (15/41, 41.37%) (29). In the second report, covering the 1999–2001 survey, CNS were still the most common isolates at 32.97% (30/91), followed by Enterococcus spp. at 25.27% (23/91) (30). In the third report, covering the 2002–2004 survey, CNS were the most common isolates at 39.08% (34/87), again followed by Enterococcus spp. at 28.73% (25/87) (31). In the most recent report, covering the 2005–2010 survey, Enterococcus spp. were the most common isolates at 39.13% (42/115), followed by CNS at 30.43% (35/115) (10). Looking at the results of these four surveys covering 12 years, we can see that the percentage of PE cases caused by Enterococcus spp. gradually increased. Those authors hypothesized that the selection pressure applied by antibiotics has caused the other species to become the leading causative microorganisms of PE (32).
One of key tasks for the researchers in this field is establishing a proper experimental model to investigate the shifts of the major causative isolates of PE. Challenges for the studies on this complex environment of the ocular surface are not limited to the presence of many cell types and bacterial species: there is also substantial variations of the microbiota among individuals. Mimicking such complex ocular surface conditions is difficult in an in vitro model. Therefore, creating in vivo model is essential for researching these changes of ocular pathological processes. Our proposed in vivo animal model may be suitable for studying the shifts of the major causative isolates for understanding PE pathogenesis, and for evaluating novel therapeutics.
In conclusion, we successfully created in vitro and in vivo co-culture models of S. epidermidis and E. faecalis. By means of these models, we confirmed that moxifloxacin, a fourth-generation fluoroquinolone, is effective against S. epidermidis, but not E. faecalis. Our results provide supporting evidences that the administration of fluoroquinolone as perioperative ophthalmic treatment is an important contributing factor of the change in the major causative agent of PE from S. epidermidis to E. faecalis. The co-culture models of this study can be a useful tool to develop an effective antibiotic that can control PE caused by E. faecalis.
Data Availability Statement
The original contributions presented in the study are included in the article/Supplementary Material, further inquiries can be directed to the corresponding authors.
Ethics Statement
The animal study was reviewed and approved by the Institutional Animal Care and Use Committee of Kosin University College of Medicine (KMAP-18-19).
Author Contributions
SL conceived of the project and created all instructional materials. SL and HK prepared all figures and wrote the manuscript. JK and IP performed data analysis and critical review the manuscript. All the authors contributed to the article and approved the submitted version.
Conflict of Interest
The authors declare that the research was conducted in the absence of any commercial or financial relationships that could be construed as a potential conflict of interest.
Publisher's Note
All claims expressed in this article are solely those of the authors and do not necessarily represent those of their affiliated organizations, or those of the publisher, the editors and the reviewers. Any product that may be evaluated in this article, or claim that may be made by its manufacturer, is not guaranteed or endorsed by the publisher.
Supplementary Material
The Supplementary Material for this article can be found online at: https://www.frontiersin.org/articles/10.3389/fmed.2021.670199/full#supplementary-material
Supplementary Figure S1. Logarithmic growth curves of in vitro mono-cultures and co-cultures of S. epidermidis and E. faecalis. Growth patterns of S. epidermidis (SE) and E. faecalis (EF) cultivated with various concentrations of a culture supernatant (sup). E. faecalis was cultured with media containing a supernatant (0, 50%, 80%, or 100%) of S. epidermidis mono-culture (A), of E. faecalis mono-culture (B), or of the S. epidermidis + E. faecalis co-culture (C) and the growth rates were measured every 30 min. In the same way, S. epidermidis was cultivated with a supernatant of E. faecalis mono-culture (D), S. epidermidis mono-culture (E), or of the co-culture (F) and growth curves were constructed.
References
1. Weikert MP. Update on bimanual microincisional cataract surgery. Curr Opin Ophthalmol. (2006) 17:62–7. doi: 10.1097/01.icu.0000193069.32369.e1
3. Wong TY, Chee S-P. The epidemiology of acute endophthalmitis after cataract surgery in an Asian population. Ophthalmology. (2004) 111:699–705. doi: 10.1016/j.ophtha.2003.07.014
4. Greenberg PB, Tseng VL, Wu W-C, Liu J, Jiang L, Chen CK, et al. Prevalence and predictors of ocular complications associated with cataract surgery in United States veterans. Ophthalmology. (2011) 118:507–14. doi: 10.1016/j.ophtha.2010.07.023
5. Nam KY, Lee JE, Lee JE, Jeung WJ, Park JM, Park JM, et al. Clinical features of infectious endophthalmitis in South Korea: a five-year multicenter study. BMC Infect Dis. (2015) 15:177. doi: 10.1186/s12879-015-0900-5
6. Kim HW, Kim SY, Chung IY, Lee JE, Lee JE, Park JM, et al. Emergence of Enterococcus species in the infectious microorganisms cultured from patients with endophthalmitis in South Korea. Infection. (2014) 42:113–8. doi: 10.1007/s15010-013-0530-z
7. Han DP, Vine AK, Blodi BA, Elner SG, Johnson MW, Khanderia S, et al. Microbiologic factors and visual outcome in the endophthalmitis vitrectomy study. Am J Ophthalmol. (1996) 122:830–46. doi: 10.1016/S0002-9394(14)71959-2
8. Bannerman TL, Rhoden DL, McAllister SK, Miller JM, Wilson LA. The source of coagulase-negative Staphylococci in the Endophthalmitis vitrectomy study. Arch Ophthalmol. (1997) 115:357. doi: 10.1001/archopht.1997.01100150359008
9. Kim JK, Nam KY, Chung IY, Jeung WJ, Kwon YH, Park JM, et al. Emerging Enterococcus isolates in postoperative endophthalmitis by selection pressure of fluoroquinolones: an 11-year multicenter and experimental study. Emerg Microbes Infect. (2020) 9:1892–9. doi: 10.1080/22221751.2020.1810134
10. Friling E, Lundström M, Stenevi U, Montan P. Six-year incidence of endophthalmitis after cataract surgery: Swedish national study. J Cataract Refract Surg. (2013) 39:15–21. doi: 10.1016/j.jcrs.2012.10.037
11. Teng YT, Teng MC, Kuo HK, Fang PC, Wu PC, Chen CH, et al. Isolates and antibiotic susceptibilities of endophthalmitis in postcataract surgery: a 12-year review of culture-proven cases. Int Ophthalmol. (2017) 37:513–8. doi: 10.1007/s10792-016-0288-2
12. Mountcastle SE, Cox SC, Sammons RL, Jabbari S, Shelton RM, Kuehne SA. A review of co-culture models to study the oral microenvironment and disease. J Oral Microbiol. (2020) 12:1773122. doi: 10.1080/20002297.2020.1773122
13. Iskeleli G, Bahar H, Eroglu E, Torun MM, Ozkan S. Microbial changes in conjunctival flora with 30-day continuous-wear silicone hydrogel contact lenses. Eye Contact Lens. (2005) 31:124–6. doi: 10.1097/01.ICL.0000141923.63458.DF
14. Yamauchi Y, Minoda H, Yokoi K, Maruyama K, Kumakura S, Usui M, et al. Conjunctival flora in patients with human immunodeficiency virus infection. Ocul Immunol Inflamm. 13:301–4. doi: 10.1080/09273940590951106
15. Kusbeci T, Kusbeci OY, Aktepe OC, Yavas G, Ermis SS. Conjunctival flora in patients with Parkinson's disease. Curr Eye Res. (2009) 34:251–6. doi: 10.1080/02713680902725970
16. Adam M, Balci M, Bayhan HA, Inkaya AÇ, Uyar M, Gürdal C. Conjunctival flora in diabetic and nondiabetic individuals. Turkish J Ophthalmol. (2015) 45:193–6. doi: 10.4274/tjo.33230
17. Hwang DG. Fluoroquinolone resistance in ophthalmology and the potential role for newer ophthalmic fluoroquinolones. Surv Ophthalmol. (2004) 49:S79–83. doi: 10.1016/j.survophthal.2004.01.004
18. Chang DF, Braga-Mele R, Mamalis N, Masket S, Miller KM, Nichamin LD, et al. Prophylaxis of postoperative endophthalmitis after cataract surgery: results of the 2007 ASCRS member survey. J Cataract Refract Surg. (2007) 33:1801–5. doi: 10.1016/j.jcrs.2007.07.009
19. Liesegang TJ. Perioperative antibiotic prophylaxis in cataract surgery. Cornea. (1999) 18:383–402. doi: 10.1097/00003226-199907000-00001
20. Agardh C-DD, Agardh E, Torffvit O, Agardh C-DD, Torffvit O, Agardh E, et al. The prognostic value of albuminuria for the development of cardiovascular disease and retinopathy: a 5-year follow-up of 451 patients with type 2 diabetes mellitus. Diabetes Res Clin Pract. (1996) 32:35–44. doi: 10.1016/0168-8227(96)01218-1
21. Adhi M, Alwassia AA, Duker JS. Analysis of choroidal thickness in eyes treated with focal laser photocoagulation using SD-OCT. Can J Ophthalmol. (2013) 48:535–8. doi: 10.1016/j.jcjo.2013.05.010
22. Aderman CM, Chao DL, Obeid A, Schwartz DM, Bhisitkul RB, Chiu CS, et al. Bilateral Enterococcus faecalis endophthalmitis with multiple recurrences. Retin Cases Brief Rep. (2021) 15:38–42. doi: 10.1097/ICB.0000000000000722
23. Abbasi J. A retinal scan for Alzheimer disease. JAMA. (2017) 318:1314. doi: 10.1001/jama.2017.15192
24. Dave SB, Toma HS, Kim SJ, Toma HS, Kim SJ, Dave SB, et al. Changes in ocular flora in eyes exposed to ophthalmic antibiotics. Ophthalmology. (2013) 120:937–41. doi: 10.1016/j.ophtha.2012.11.005
25. Benz MS, Scott IU, Flynn HW, Unonius N, Miller D, Flynn Jr HW, et al. Endophthalmitis isolates and antibiotic sensitivities: a 6-year review of culture-proven cases. Am J Ophthalmol. (2004) 137:38–42. doi: 10.1016/S0002-9394(03)00896-1
26. Choi S, Hahn TW, Osterhout G, O'Brien TP. Comparative intravitreal antibiotic therapy for experimental Enterococcus faecalis endophthalmitis. Arch Ophthalmol. (1996) 114:61. doi: 10.1001/archopht.1996.01100130057009
27. Chen K-J, Lai C-C, Sun M-H, Chen T-L, Yang K-J, Kuo Y-H, et al. Postcataract endophthalmitis caused by Enterococcus faecalis. Ocul Immunol Inflamm. (2009) 17:364–9. doi: 10.3109/09273940903105110
28. Scott IU, Loo RH, Flynn HW, Miller D. Endophthalmitis caused by Enterococcus faecalis: antibiotic selection and treatment outcomes. Ophthalmology. (2003) 110:1573–7. doi: 10.1016/S0161-6420(03)00502-5
29. Montan P, Lundström M, Stenevi U, Thorburn W. Endophthalmitis following cataract surgery in Sweden.The 1998 national prospective survey. Acta Ophthalmol Scand. (2002) 80:258–61. doi: 10.1034/j.1600-0420.2002.800305.x
30. Wejde G, Montan P, Lundström M, Stenevi U, Thorburn W. Endophthalmitis following cataract surgery in Sweden: national prospective survey 1999-2001. Acta Ophthalmol Scand. (2005) 83:7–10. doi: 10.1111/j.1600-0420.2005.00377.x
31. Wejde G, Stenevi U, Lundström M, Thorburn W, Montan P, Lundström M, et al. Endophthalmitis after cataract surgery. Ophthalmology. (2007) 114:866–70. doi: 10.1016/j.ophtha.2006.11.025
Keywords: Enterococcus, Staphylococcus, fluoroquinolone, endophthalmitis, topical eye drops, co-culture model
Citation: Kim HW, Kim JK, Park I and Lee SJ (2021) Establishing in vitro and in vivo Co-culture Models of Staphylococcus epidermidis and Enterococcus faecalis to Evaluate the Effect of Topical Fluoroquinolone on Ocular Microbes. Front. Med. 8:670199. doi: 10.3389/fmed.2021.670199
Received: 26 February 2021; Accepted: 02 December 2021;
Published: 20 December 2021.
Edited by:
Xiaohui Zhou, Fudan University, ChinaCopyright © 2021 Kim, Kim, Park and Lee. This is an open-access article distributed under the terms of the Creative Commons Attribution License (CC BY). The use, distribution or reproduction in other forums is permitted, provided the original author(s) and the copyright owner(s) are credited and that the original publication in this journal is cited, in accordance with accepted academic practice. No use, distribution or reproduction is permitted which does not comply with these terms.
*Correspondence: Sang Joon Lee, aGhpYXR1c0BnbWFpbC5jb20=