- 1Department of Critical Care Medicine, Zhongshan Hospital, Fudan University, Shanghai, China
- 2Wuhan JinYinTan Hospital, Wuhan, China
- 3Department of Cardiology, Zhongshan Hospital, Shanghai Institute of Cardiovascular Diseases, Fudan University, Shanghai, China
- 4Institute of Biomedical Sciences, Fudan University, Shanghai, China
- 5Intensive Care Unit of the Six Floor of South Building, Wuhan JinYinTan Hospital, Wuhan, China
- 6Department of Critical Care Medicine, GuangZhou Institute of Respiratory Health, The First Affiliated Hospital of GuangZhou Medical University, Guangzhou, China
- 7Department of Critical Care Medicine, The First Affiliated Hospital of Zhejiang University, Hangzhou, China
Object: To evaluate the clinical efficacy and safety of α-Lipoic acid (ALA) for critically ill patients with coronavirus disease 2019 (COVID-19).
Methods: A randomized, single-blind, group sequential, active-controlled trial was performed at JinYinTan Hospital, Wuhan, China. Between February 2020 and March 2020, 17 patients with critically ill COVID-19 were enrolled in our study. Eligible patients were randomly assigned in a 1:1 ratio to receive either ALA (1200 mg/d, intravenous infusion) once daily plus standard care or standard care plus equal volume saline infusion (placebo) for 7 days. All patients were monitored within the 7 days therapy and followed up to day 30 after therapy. The primary outcome of this study was the Sequential Organ Failure Estimate (SOFA) score, and the secondary outcome was the all-cause mortality within 30 days.
Result: Nine patients were randomized to placebo group and 8 patients were randomized to ALA group. SOFA score was similar at baseline, increased from 4.3 to 6.0 in the placebo group and increased from 3.8 to 4.0 in the ALA group (P = 0.36) after 7 days. The 30-day all-cause mortality tended to be lower in the ALA group (3/8, 37.5%) compared to that in the placebo group (7/9, 77.8%, P = 0.09).
Conclusion: In our study, ALA use is associated with lower SOFA score increase and lower 30-day all-cause mortality as compared with the placebo group. Although the mortality rate was two-folds higher in placebo group than in ALA group, only borderline statistical difference was evidenced due to the limited patient number. Future studies with larger patient cohort are warranted to validate the role of ALA in critically ill patients with COVID-19.
Clinical Trial Registration: http://www.chictr.org.cn/showproj.aspx?proj=49534.
Introduction
Since December 2019, the novel coronavirus pneumonia 2019 (COVID-19) induced by severe acute respiratory coronavirus 2 (SARS-CoV-2) has become a worldwide pandemic and is overwhelming health care systems globally. Thus far, no therapeutics have yet been proven effective for the treatment of severe COVID-19, and the mortality rate of severe COVID-19 patients is high (1–5). Pathological results suggested that the lung displayed diffuse alveolar damage. In addition, interstitial mononuclear inflammatory infiltrates, mainly lymphocytes, were observed in both lungs (6). Moreover, several studies have shown that C reactive protein (CRP), D-dimer and serum proinflammatory cytokines (IL-6, IL-1β, etc.) was increased in patients with severe COVID-19 (6–9). These findings suggested that the cytokine release syndrome (CRS) may be associated with COVID-19. CRS is a systemic inflammatory response that can be induced by infection and certain drugs. The sharp increase of pro-inflammatory cytokines is the main manifestation, which can cause damage to the heart, lungs and other organs (10). Therefore, regulating systemic inflammatory response may be a key method for treating patients with COVID-19, and several clinical studies targeting CRS have been conducted (11). Viral infection can also cause the production of reactive oxygen species (ROS), and ROS plays an important role in virus replication and invasion (12), organ damage (13, 14), and systemic inflammatory response (15–20).
α-Lipoic acid (ALA), as an antioxidant, has been confirmed to reduce systemic inflammatory response in patients with acute coronary syndromes, liver transplantation patients, and kidney-pancreas combined transplantation patients (21–24). Therefore, the aim of this study was to investigate whether ALA could alleviate systemic inflammatory response by reducing ROS production, thereby improve organ function and the prognosis of critically ill patients with COVID-19.
Methods
Study Design and Participants
This study was a randomized, single-blind, group sequential, active-controlled trial. Participants were recruited from February 2020 to March 2020. 17 participants with critically ill COVID-19 hospitalized in JinYinTan Hospital, Wuhan, China were enrolled. Ethical approval was obtained from the Institutional Ethics Committee of Zhongshan Hospital(B2020-030). The informed consent was signed by all participants. The trial has been registered in the Chinese Clinical Trial Registry (ChiCTR2000029851). The inclusion criteria were: 1. Patients diagnosed with critically ill COVID-19. It complies with the COVID-19 Critical and Critical Diagnostic Standards, namely “Pneumonitis Diagnosis and Treatment Scheme for Novel Coronavirus Infection (Trial Version 5); 3. Sign written informed consent. Patients who cannot sign informed consent must obtain informed consent from the independent authorized nurse. The exclusion criteria were: (1) Patients who are participating in other clinical trials; (2) Pregnant or breastfeeding women; (3) There are other life-threatening diseases such as cancer; (4) Expected survival time <24 h; (5) Patients who are allergic to ALA or similar drugs (B vitamins), and intolerant to the recommended dosage of ALA in the past; (6). A history of immune system diseases or diseases closely related to the immune system (Figure 1).
Randomization and Blinding
We used mixed randomization for unpredictability in allocation sequences (25). The allocation sequences were placed in the sealed opaque envelopes. We randomly assigned critically ill patients with COVID-19 to the treatment group and control group in a 1:1 ratio. Blinding is saved until research have been completed and the database is finally unlocked. Participants and study personnel were aware of the study-group assignments, but outcome adjudicators were not.
Procedures
Patients in the treatment group were treated with ALA (1200 mg/d, intravenous infusion) once daily plus for 7 days on top of standard medical care. Patients in the control group were treated with equal volume saline infusion on top of standard medical care for 7 days. ALA is discontinued when the treatment plan is completed or the patient meets the halfway withdrawal criteria. Withdrawal criteria: (1) The subject himself requested to withdraw from the trial; (2) An intolerable adverse event or an adverse event that the investigator believes must be withdrawn from the study; (3) Those who cannot be treated according to the protocol, have poor compliance, or the physician believes that they should withdraw from the trial.
Clinical and Laboratory Monitoring
Initial investigations included onset time, CT results, nucleic acid test results, previous history, heart-related symptoms (chest tightness, etc.), NYHA classification, personal history, comorbidities and treatment plans, comprehensive physical examination (including blood pressure, heart rate, weight, oxygen Saturation measurement), Sequential Organ Failure Estimate (SOFA) Score, electrocardiogram results, echocardiogram results and laboratory test results (blood routine, liver and kidney function, electrolytes, blood glucose, blood lipids, CRP, urine pregnancy test (women), brain natriuretic peptide, myocardial enzymes, troponin, coagulation function, serum inflammatory factor tests). During the clinical follow-up period (days 1, 2, 3, 4, 5, 6, 7), the following data were collected from patients each day: symptoms, blood pressure, heart rate, fingertip oxygen saturation (recorded twice daily: 9 am and 3 pm), SOFA score, major and minor endpoint event records, adverse event records and laboratory tests (blood routine, Liver and kidney function electrolytes, myocardial enzymes, troponin, NT-proBNP, CPR, coagulation).
Primary Outcomes and Secondary Outcomes
The primary outcome of this study was the SOFA Score, and the secondary outcome was the all-cause mortality up to 30 days. All outcomes were determined by an independent clinical endpoint determination committee.
Statistical Analysis
We estimated that with 10 deaths the study would have 68% power to detect a 50% improvement in survival with ALA vs. standard control group (from admission to discharge), at a two-sided alpha level of 0.05. Primary and secondary endpoints were measured in the intention-to-treat population, which included all patients who underwent randomization. The Kaplan–Meier method was used to estimate time-to-event end points. Two-sided stratified log-rank tests and 2Log (LR) test were used. Cox regression models were applied to estimate hazard ratios. Safety evaluations were included all patients who received at least one dose of study medication. Continuous variables were described using mean, median, and standard deviation, and examined using Analysis of Variance (ANOVA) or Wilcoxon rank sum test or Kruskal-Wallis test. Categorical variables were described by frequency, and assessed using chi-square test or Fisher exact test. Changes in laboratory data, signs and examination items, and adverse events before and after treatment in the two groups will be analyzed as safety indicators. This study does not estimate missing data. Statistical analysis was performed using SAS 9.13. Values of P < 0.05 were considered statistically significant, and the two-sided test is represented by a 95% confidence interval.
Results
Characteristics of the Patients: Groups Were Balanced With Respect to Baseline Characteristics
A total of 17 eligible patients with critically ill COVID-19 were enrolled in our study. 8 patients were assigned to receive ALA and 9 patients to standard care alone. A total of 13 patients (76.5%) were men, the age range was 51 to 91 years, and the median age of patients was 63 years (interquartile range [IQR], 59 to 66 years) (Table 1). There were no significant differences between groups in demographic characteristics, baseline laboratory test results and SOFA Score at enrollment.
Primary Outcomes: No Significant Difference in SOFA Score
From day 0 to day 7, the average SOFA Score in the placebo group increased by 1.7 points from 4.3 to 6.0. In the same time period, the average SOFA Score in the ALA treatment group increased by 0.2 points from 3.8 to 4.0 (Figure 2, Table 2). There was no significant difference in SOFA Score between the placebo group and the ALA group (P = 0.36).
Secondary Outcomes: A Borderline Statistical Significance in All-Cause Mortality
After a 30-day follow-up, the 30-day all-cause mortality was 77.8% (7/9) in the placebo group, and 37.5% (3/8) in the ALA group (Figure 3, Table 2). The 30-day all-cause mortality was numerically lower in the ALA group than in the placebo group, a borderline statistical significance was observed (P = 0.09).
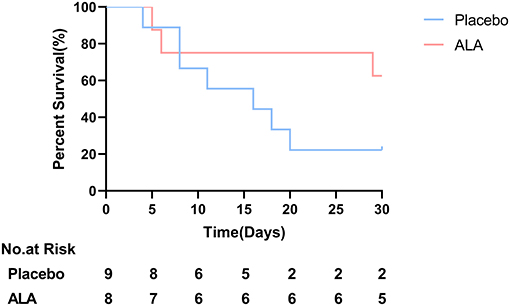
Figure 3. Overall survival; Borderline significance was observed between ALA group and placebo group (P = 0.09) at day 30.
Adverse Events: No Adverse Events
Patients were followed up for adverse events. No unexpected drugs-related adverse events occurred during the trial.
Discussion
Our study enrolled 17 patients with critically ill COVID-19 in Wuhan JinYinTan Hospital. The results suggested ALA treatment might be able to improve 30-day survival rate of patients with critically ill COVID-19 and slow down the increase of SOFA score, both parameters did not reach statistical significance due to the limited patient number, but the tendency of improvement is clear to see.
The protection efficiency of ALA in critically ill COVID-19 patients could be mainly contributed by its antioxidant and anti-inflammation effects. Firstly, ALA could ameliorate virus-induced organ dysfunction by counteracting ROS. ROS serves as important mediator for virus infection and subsequent organ failure. Xian (12) and Ross (14) revealed that ROS induced by influenza virus infection is responsible for the replication of influenza virus and could cause organ injury, which could be alleviated by inhibiting ROS production. Recent study also prove that virus infection could increase ROS production and facilitate subsequent proinflammatory response (26). ALA could directly interact with and scavenge ROS such as superoxide radicals, hydroxyl radicals, hypochlorous acid, peroxyl radicals, and singlet oxygen (21). In addition, ALA contributes to the recycling of vitamin-E and vitamin-C, which could also attenuate oxidative damage (21). Secondly, the anti-inflammation effect of ALA was verified by a series of studies. MERS-CoV infection could upregulate series of proinflammatory cytokines in endothelial cells, which might induce cytokine storms and cause severe organ damage (26). Some studies proved that ALA could mitigate infiltration of inflammatory cells, decline the upregulation of inflammatory cytokines (IL-1B, IL-6) after harmful stimuli (22). It is also known that ALA could significantly reduce levels of serum inflammatory cytokines and improve symptoms of patients with acute coronary syndrome, etc. (23, 24). The anti-inflammatory effects of ALA can be attributed to two aspects. Firstly, lipoic acid can inhibit the NF-κB translocation, thereby attenuating the release of more free radicals and cytotoxic cytokines (27); secondly, lipoic acid can eliminate reactive oxygen species and alleviate cell death, thereby reducing the release of damage associated molecular patterns (DAMPs) and further activation of downstream inflammatory pathway (28). Some clinical trials have been designed to dampen inflammatory responses. One clinical trial (ChiCTR2000029765) reported that neutralizing IL-6 with tocilizumab could quickly control the high fever and respiratory symptoms in 21 patients with COVID-19 (11), which revealed the feasibility of anti-inflammation treatment option for COVID-19. Our data demonstrated that ALA could alleviate the increase of CRP to some extent, but no significant difference was observed due to limited number of enrolled patients (Supplementary Figure 1).
The clinical trials of hydroxychloroquine combined with azithromycin in the treatment of COVID-19 by Molina et al. (29) (N = 11) focused on patients with mild COVID-19. In another clinical trial focused on patients with severe COVID-19, there was no significant difference in viral RNA loads or duration of viral RNA detectability between the lopinavir-ritonavir combination treatment group and the control group, but lopinavir-ritonavir treatment group presented with lower all-cause mortality on day 28 (P > 0.05) (30). Thus, in addition to the anti-virus treatment strategy, searching for other treatment strategies are of clinical importance aiming to alleviate the complications of COVID patients and reduce the mortality rate of patients with severe and critically ill COVID-19 was also essential.
This trial has several limitations. In particular, the trial did not have sufficient number of cases. Because the epidemic situation in Wuhan was rapidly under control during the trial period, and critically ill COVID patients participating other clinical trials could not be enrolled in this study, only 17 critically ill COVID patients were enrolled in this study. Another limitation is that we use all-cause mortality as our secondary outcome, which could not distinguish death due to primary SARS-COV-2 insult from secondary bacterial infection, which was common in late phrase of critically ill patients with COVID-19. Further clinical studies are needed to verify the promising efficacy of ALA injection in patients with critically ill COVID-19.
In conclusion, our results derived from the small number of critically ill COVID patients are promising and hope this report might stimulate the initiation of further clinical studies in larger patient cohort to validate the role of ALA on reducing the short-term mortality rate among critically ill COVID-19 patients.
Data Availability Statement
The raw data supporting the conclusions of this article will be made available by the authors, without undue reservation.
Ethics Statement
The studies involving human participants were reviewed and approved by Institutional Ethics Committee of Zhongshan Hospital (B2020-030). The patients/participants provided their written informed consent to participate in this study.
Author Contributions
AS, JZ, and WY analysed data and wrote the manuscript. KH, XJ, and TX analysed data and reviewed the manuscript. PW and XZ reviewed the manuscript. MZ, TX, GY, and LS reviewed the manuscript and contributed to the discussion. JG and DZ are the guarantor of this work and, as such, had full access to all the data in the study and takes responsibility for the integrity of the data and the accuracy of the data analysis. All authors contributed to the article and approved the submitted version.
Conflict of Interest
The authors declare that the research was conducted in the absence of any commercial or financial relationships that could be construed as a potential conflict of interest.
Publisher's Note
All claims expressed in this article are solely those of the authors and do not necessarily represent those of their affiliated organizations, or those of the publisher, the editors and the reviewers. Any product that may be evaluated in this article, or claim that may be made by its manufacturer, is not guaranteed or endorsed by the publisher.
Acknowledgments
We thank Mr. Huirong Zhang from YAOPHARMA CO., LTD, for the donation of α-Lipoic acid used in this study.
Supplementary Material
The Supplementary Material for this article can be found online at: https://www.frontiersin.org/articles/10.3389/fmed.2021.566609/full#supplementary-material
Supplementary Figure 1. CRP.
Supplementary Figure 2. SaO2.
References
1. Epidemiology Working Group for NCIP Epidemic Response, Chinese Center for Disease Control and Prevention. The epidemiological characteristics of an outbreak of 2019 novel coronavirus diseases (COVID-19) in China. Chin J Epidemiol. (2020) 41:145–1510. doi: 10.46234/ccdcw2020.032
2. Arentz M, Yim E, Klaff L, Lokhandwala S, Riedo FX, Chong M, et al. Characteristics and outcomes of 21 critically ill patients with COVID-19 in Washington state. Jama. (2020) 323:1612–4. doi: 10.1001/jama.2020.4326
3. Wang D, Hu B, Hu C, Zhu F, Liu X, Zhang J, et al. Clinical characteristics of 138 hospitalized patients with 2019 novel coronavirus-infected pneumonia in Wuhan, China. Jama. (2020) 323:1061–9. doi: 10.1001/jama.2020.1585
4. Zhou F, Yu T, Du R, Fan G, Liu Y, Liu Z, et al. Clinical course and risk factors for mortality of adult inpatients with COVID-19 in Wuhan, China: a retrospective cohort study. Lancet. (2020) 395:1054–62. doi: 10.1016/S0140-6736(20)30566-3
5. Bhatraju PK, Ghassemieh BJ, Nichols M, Kim R, Jerome KR, Nalla AK, et al. Covid-19 in critically ill patients in the seattle region - case series. N Engl J Med. (2020) 382:2012–22. doi: 10.1056/NEJMoa2004500
6. Xu Z, Shi L, Wang Y, Zhang J, Huang L, Zhang C, et al. Pathological findings of COVID-19 associated with acute respiratory distress syndrome. Lancet Respir Med. (2020) 8:420–2. doi: 10.1016/S2213-2600(20)30076-X
7. Qin C, Zhou L, Hu Z, Zhang S, Yang S, Tao Y, et al. Dysregulation of immune response in patients with coronavirus 2019 (COVID-19) in Wuhan, China. Clin Infect Dis. (2020) 71:762–8. doi: 10.1093/cid/ciaa248
8. Tan M, Liu Y, Zhou R, Deng X, Li F, Liang K, et al. Immunopathological characteristics of coronavirus disease 2019 cases in Guangzhou, China. Immunology. (2020) 160:261–8. doi: 10.1111/imm.13223
9. Huang C, Wang Y, Li X, Ren L, Zhao J, Hu Y, et al. Clinical features of patients infected with 2019 novel coronavirus in Wuhan, China. Lancet. (2020) 395:497–506. doi: 10.1016/S0140-6736(20)30183-5
10. Shimabukuro-Vornhagen A, Gödel P, Subklewe M, Stemmler HJ, Schlößer HA, Schlaak M, et al. Cytokine release syndrome. J Immunother Cancer. (2018) 6:56. doi: 10.1186/s40425-018-0343-9
11. Cao X. COVID-19: immunopathology and its implications for therapy. Nat Rev Immunol. (2020) 20:269–70. doi: 10.1038/s41577-020-0308-3
12. Lin X, Wang R, Zou W, Sun X, Liu X, Zhao L, et al. The influenza virus H5N1 infection can induce ROS production for viral replication and host cell death in A549 cells modulated by human Cu/Zn superoxide dismutase (SOD1) overexpression. Viruses. (2016) 8:13. doi: 10.3390/v8010013
13. Mittal M, Siddiqui MR, Tran K, Reddy SP, Malik AB. Reactive oxygen species in inflammation and tissue injury. Antioxid Redox Signal. (2014) 20:1126–67. doi: 10.1089/ars.2012.5149
14. Vlahos R, Stambas J, Selemidis S. Suppressing production of reactive oxygen species (ROS) for influenza A virus therapy. Trends Pharmacol Sci. (2012) 33:3–8. doi: 10.1016/j.tips.2011.09.001
15. Yoo SK, Starnes TW, Deng Q, Huttenlocher A. Lyn is a redox sensor that mediates leukocyte wound attraction in vivo. Nature. (2011) 480:109–12. doi: 10.1038/nature10632
16. Shimada K, Crother TR, Karlin J, Dagvadorj J, Chiba N, Chen S, et al. Oxidized mitochondrial DNA activates the NLRP3 inflammasome during apoptosis. Immunity. (2012) 36:401–14. doi: 10.1016/j.immuni.2012.01.009
17. Singh DK, Kumar D, Siddiqui Z, Basu SK, Kumar V, Rao KV. The strength of receptor signaling is centrally controlled through a cooperative loop between Ca2+ and an oxidant signal. Cell. (2005) 121:281–93. doi: 10.1016/j.cell.2005.02.036
18. Devadas S, Zaritskaya L, Rhee SG, Oberley L, Williams MS. Discrete generation of superoxide and hydrogen peroxide by T cell receptor stimulation: selective regulation of mitogen-activated protein kinase activation and fas ligand expression. J Exp Med. (2002) 195:59–70. doi: 10.1084/jem.20010659
19. Hara-Chikuma M, Chikuma S, Sugiyama Y, Kabashima K, Verkman AS, Inoue S, et al. Chemokine-dependent T cell migration requires aquaporin-3-mediated hydrogen peroxide uptake. J Exp Med. (2012) 209:1743–52. doi: 10.1084/jem.20112398
20. Kumar A, Wu H, Collier-Hyams LS, Hansen JM, Li T, Yamoah K, et al. Commensal bacteria modulate cullin-dependent signaling via generation of reactive oxygen species. EMBO J. (2007) 26:4457–66. doi: 10.1038/sj.emboj.7601867
21. Packer L, Witt EH, Tritschler HJ. alpha-Lipoic acid as a biological antioxidant. Free Radic Biol Med. (1995) 19:227–50. doi: 10.1016/0891-5849(95)00017-R
22. Hu QF, Sun AJ. Cardioprotective effect of alpha-lipoic acid and its mechanisms. Cardiol Plus. (2020) 5:109. doi: 10.4103/cp.cp_16_20
23. Casciato P, Ambrosi N, Caro F, Vazquez M, Müllen E, Gadano A, et al. α-lipoic acid reduces postreperfusion syndrome in human liver transplantation - a pilot study. Transpl Int. (2018) 31:1357–68. doi: 10.1111/tri.13314
24. Bacchetti P, Leung JM. Sample size calculations in clinical research. Anesthesiology. (2002) 97:1028–9. doi: 10.1097/00000542-200210000-00050
26. Shao Y, Saredy J, Xu K, Sun Y, Saaoud F, Drummer Ct, et al. Endothelial Immunity Trained by Coronavirus Infections, DAMP Stimulations and Regulated by Anti-Oxidant NRF2 May Contribute to Inflammations, Myelopoiesis, COVID-19 Cytokine Storms and Thromboembolism. Front Immunol. (2021) 12:653110. doi: 10.3389/fimmu.2021.653110
27. Bierhaus A, Chevion S, Chevion M, Hofmann M, Quehenberger P, Illmer T, et al. Advanced glycation end product-induced activation of NF-kappaB is suppressed by alpha-lipoic acid in cultured endothelial cells. Diabetes. (1997) 46:1481–90. doi: 10.2337/diabetes.46.9.1481
28. Deng C, Sun Z, Tong G, Yi W, Ma L, Zhao B, et al. α-Lipoic acid reduces infarct size and preserves cardiac function in rat myocardial ischemia/reperfusion injury through activation of PI3K/Akt/Nrf2 pathway. PLoS ONE. (2013) 8:e58371. doi: 10.1371/journal.pone.0058371
29. Molina JM, Delaugerre C, Le Goff J, Mela-Lima B, Ponscarme D, Goldwirt L, et al. No evidence of rapid antiviral clearance or clinical benefit with the combination of hydroxychloroquine and azithromycin in patients with severe COVID-19 infection. Med Mal Infect. (2020) 50:384. doi: 10.1016/j.medmal.2020.03.006
Keywords: pneumonia, SARS-CoV-2, COVID-19, α-Lipoic acid, SOFA score
Citation: Zhong M, Sun A, Xiao T, Yao G, Sang L, Zheng X, Zhang J, Jin X, Xu L, Yang W, Wang P, Hu K, Zhang D and Ge J (2022) A Randomized, Single-Blind, Group Sequential, Active-Controlled Study to Evaluate the Clinical Efficacy and Safety of α-Lipoic Acid for Critically Ill Patients With Coronavirus Disease 2019 (COVID-19). Front. Med. 8:566609. doi: 10.3389/fmed.2021.566609
Received: 02 June 2020; Accepted: 15 November 2021;
Published: 04 February 2022.
Edited by:
Daihai He, Hong Kong Polytechnic University, Hong Kong SAR, ChinaReviewed by:
Andrey V. Kuznetsov, Innsbruck Medical University, AustriaXiaofeng Yang, Temple University, United States
Copyright © 2022 Zhong, Sun, Xiao, Yao, Sang, Zheng, Zhang, Jin, Xu, Yang, Wang, Hu, Zhang and Ge. This is an open-access article distributed under the terms of the Creative Commons Attribution License (CC BY). The use, distribution or reproduction in other forums is permitted, provided the original author(s) and the copyright owner(s) are credited and that the original publication in this journal is cited, in accordance with accepted academic practice. No use, distribution or reproduction is permitted which does not comply with these terms.
*Correspondence: Dingyu Zhang, 1813886398@qq.com; Junbo Ge, jbge@zs-hospital.sh.cn
†These authors have contributed equally to this work