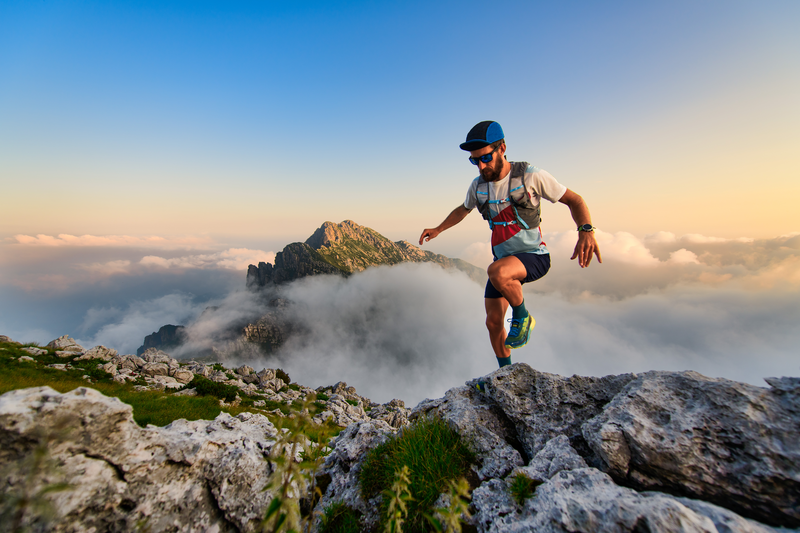
94% of researchers rate our articles as excellent or good
Learn more about the work of our research integrity team to safeguard the quality of each article we publish.
Find out more
MINI REVIEW article
Front. Med. , 17 May 2021
Sec. Infectious Diseases – Surveillance, Prevention and Treatment
Volume 8 - 2021 | https://doi.org/10.3389/fmed.2021.553965
This article is part of the Research Topic Innovative Approaches In The Management Of Bone and Joint Infection View all 20 articles
The genus Staphylococcus is the main causative agent of bone and joint infections (BJI) in which outcomes are impacted by both effective surgical and appropriate antimicrobial management. In this context, methicillin resistance (MR) detection is a microbiological challenge to optimize the anti-staphylococcal drug coverage and to secure the surgical procedure. During the last decade, molecular tools have been developed to rapidly detect bacterial-resistant strains in clinical samples. The GeneXpert MRSA/SA SSTI® assay (Cepheid, Sunnyvale, CA, USA) is a real-time PCR method aimed at detecting methicillin-resistant Staphylococcus aureus (MRSA) in skin and soft tissues infections. In the literature, this test has been reported to be diverted from its original purpose to be evaluated in surgical samples. Within the current review, we update the GeneXpert MRSA/SA SSTI® assay performance in staphylococcal species determination (i.e., S. aureus vs. coagulase-negative species) together with MR genotype detection, when performed in osteoarticular infections.
Bone and joint infections (BJI) encompass a heterogenous group combining native joints and device-associated infections, covering children osteomyelitis, adults' septic arthritis, spondylodiscitis, and prosthetic joint infections (PJI). They require a complex management involving a multidisciplinary approach associating orthopedic surgeons, infectiologists, and microbiologists (1). Staphylococcus spp., the main bacterial genus involved in BJI, is reported to be a risk factor associated with inpatient mortality (2). Today, methicillin-resistant coagulase-negative staphylococci (MRCoNS) have become unavoidable in chronic PJI (3, 4) justifying empirical use of glycopeptides (5); while efficiently targeting methicillin-sensitive Staphylococcus aureus (MSSA) in native BJI is the key to avoid recurrence and complications (6, 7). In this context, providing rapid bacterial susceptibility results is crucial to guide efficient antimicrobial adaptation in the peri-operative time. Until now, the “gold-standard” method still relies on conventional microbial cultures that require 2–15 days to identify bacterial strains (8) and additional 24–72 h longer to ascertain the antibiotic susceptibility pattern. Moreover, the efficacy of culture methods is partly questionable with a 62.6% sensitivity (9) in PJI cases, and importantly, methicillin resistance (MR) determination may be delicate to rapidly discriminate a heterogenous phenotypical feature and a “borderline” resistance with conventional methods. Hence, there is a need to improve diagnosis methods to reduce the time frame for appropriate antimicrobial management, since total hip and total knee revisions are anticipated to increase by 137 and 601%, respectively, between 2005 and 2030 (10) with 25% attributed to infection (11). During the last decade, the global bacterial resistance burden, including the spread of the virulent community-acquired methicillin-resistant S. aureus (CA-MRSA) clone US300 (12), triggered the development of molecular tools aimed at targeting bacterial pathogen DNA and their main resistance determinants. In this attempt, the GeneXpert MRSA/SA SSTI® test (Cepheid, Sunnyvale, CA, USA) was originally designed to detect S. aureus (SA) and methicillin-resistant SA (MRSA) directly in clinical samples of skin and soft tissue infections (SSTI). This test was efficiently evaluated in wound and blood culture specimens (13) engaging to divert its former use for osteoarticular applications by distinct clinical units worldwide. Beyond SA and MRSA identification, the assay allows for the specific detection of the genetic support of MR, the mecA gene, and interestingly provides the possibility to detect the presence of an MR staphylococcal (MRS) strain from the surgical site, whatever the species. We propose herein to review the performance of the GeneXpert MRSA/SA SSTI® assay for SA, MRSA, and MRCoNS detection and discuss the reliability of such use in several BJI contexts throughout recent articles.
MR is acquired by horizontal transfer and chromosomal integration of a mobile genetic element designated staphylococcal cassette chromosome mec (SCCmec) (14). The mecA gene encodes an alternative penicillin-binding protein (PBP2a), an enzyme responsible for crosslinking the peptidoglycans in the bacterial cell wall, resulting in poor affinity for β-lactams and global resistance to this class of antibiotics (15). The GeneXpert MRSA/SA SSTI® assay is a commercial real-time PCR-based method which relies on the simultaneous detection of three targets: the SA protein A (spa) gene, the gene supporting MR (mecA), and the SA SCCmec chromosomal insertion site which is located at the 3′ end of an unknown function open reading frame, orfX (16). All PCR steps (i.e., extraction, amplification, and detection) take place in a single-use cartridge which contains all the reagents necessary for the detection of the three abovementioned bacterial targets together with an internal sample processing control (SPC) (Bacillus globigii spores). According to the manufacturer's recommendations, clinical samples may be collected on Copan swabs, discharged in elution buffer, vortexed for 10 s, and then transferred into Xpert MRSA/SA cartridges. The overall analysis is complete in <1 h, and amplification curves are automatically read by the GeneXpert Dx System in terms of MRSA and SA positive or negative, respectively. A comprehensive look may lead to additional interpretations: (i) an isolated amplification of the spa gene assesses the presence of MSSA, (ii) the simultaneous detection of the three targets (i.e., spa, mecA, and SCCmec) attests the presence of MRSA, (iii) a unique amplification of the mecA gene can be interpreted as the presence of MRCoNS, (iv) the simultaneous detection of both the spa and the mecA genes supposes a mixed infection containing both MSSA and MRCoNS strains, and (v) the amplification of the spa gene and SCC insertion site without mecA signal may be interpreted as an MSSA empty cassette variant. The limits of detection reported by the manufacturer are 150 and 300 CFU/swab for positive SA and MRSA results, respectively.
The literature points out only seven publications dealing with the performances of the MRSA/SA SSTI® real-time PCR assay (Cepheid, Sunnyvale, CA) in BJI diagnosis according to distinct protocols. Four out of seven were prospective studies; all but one (17) were led on adult cohorts. These studies were mainly conducted on PJI patients (18–21); unspecified BJI (22); a combination between PJI, spondylodiscitis, and septic arthritis (23); and children suffering from musculoskeletal infections (17). The number of patients included varied from 30 to 213 patients tested for one (21) to at least three distinct samples (18, 19), including joint aspiration, tissue or bone specimens, and prosthetic sonicates in one case (21). Tests of patients diagnosed with staphylococcal BJI were performed either on fresh samples (18, 19, 23) or frozen stored ones (−80°C) (17, 20–22). Biopsies were either directly vortexed (20), grinded, or crushed in saline buffer (17, 22, 23) or even cultured according to beadmill processing (18, 19, 21, 24). In all studies, the liquid phase of the samples was absorbed onto a swab (Copan, Cepheid) from 5 s (23) to 1 min (22) and then discharged in the elution buffer according to the manufacturer's recommendations. Another strategy consisted of directly collecting one Eswab from the periprosthetic tissue during the surgery and vortexing it into a reagent vial from the Xpert kit (21). RT-PCR results obtained from those swabs were compared to identification and resistance patterns reached from corresponding standard (17, 18, 23) and enriched cultures in blood culture bottles (17, 20) in Schaedler (22) or Rosenow broth (19), which were incubated from 5 (17) up to 14 (18, 19, 21–23) or 15 days (20). The main evaluation criteria were the accuracy of MR detection (18–20), the ability of the GeneXpert MRSA-SA SSTI restricted to the identification of SA and MRSA (17, 22), or the latter associated with MRCoNS detection (21, 23).
SA is the predominant causal pathogen involved in native infections which represent the most frequent clinical form of BJI, accounting for 68% of cases (2). SA also remains the main cause of acute hematogenous BJI in children, representing >90% of methicillin-sensitive strains (25). On the one hand, while most of the studies focus on MRSA, MSSA native BJI are associated with a high rate of treatment failure and further functional sequalae (6), as reported by a three times higher risk of recurrence following vancomycin therapy than observed with β-lactam antibiotics (7). On the other hand, when considering device-associated infections, SA is more likely to be involved in early acute infections (4, 26), while MR phenotype is subject to geographical discrepancies (3). In the USA, SA accounts for 38.6% of surgical site infections following orthopedic surgery, including 38% of MR strains (27), leading to an evaluated cost of $107,264 per case in comparison with $68,053 in case of MSSA (28). In Europe, SA is involved in a similar proportion of PJI (31.9 and 38.7% of total hip and knee arthroplasty infections, respectively) (29), whereas MR proportion follows a downward trend, as confirmed within German (30) and French PJI cohorts (4). When compared to conventional culture (Table 1), the Xpert's test shows attractive performances in SA detection with high sensitivity and specificity ranging from 85.4% (17) to 100% (18, 21, 23) and 91.2% (18) to 100% (21, 22), respectively. Moreover, positive PCR cases related to sterile cultures had to be reconsidered in light of the patient's history (i.e., proven SA infection) supporting the demonstrated sensitivity of the molecular assay. Interestingly, MRSA detection displays an analogous level of efficiency and is associated with a high negative predictive value (NPV 98.9–100%) allowing unambiguous use of β-lactams when SA is detected in osteoarticular samples. Further studies considering both the clinical outcome and the economic impact of such early screening would be of major interest, as suggested by the recent report from AlQahtani et al. (31) in the context of SA bacteremia.
MRCoNS have gained global attention in recent years and are responsible for a large proportion of PJI in European countries (3). While CoNS are less pathogenic than SA, they are able to adhere and colonize orthopedic devices by producing a biofilm. This structure encloses low amounts of slow-growing bacteria and causes delayed infections characterized by subtle symptoms, making their clinical and microbiological diagnosis challenging. Moreover, these infections involving a large proportion of MRCoNS (3, 4) justify empirical use of vancomycin in combination with a broad-spectrum β-lactam (5, 32). High dosage regimens are required to ensure an effective bone diffusion of the antibiotics, but this strategy leads to a significant rate of adverse effect (33) that could be spared by directly detecting MRCoNS in the surgical site. Xpert's accuracy was addressed in this aim by targeting the mecA gene in solid and liquid osteoarticular samples (18–21, 23). The test was first validated by Dubouix-Bourandy et al. (23) on 25 samples isolated from various BJI conditions with sensitivity, specificity, positive predictive value (PPV), and NPV values of 100, 95.3, 85.2, and 100%, respectively. These results were further corroborated on chronic PJI patients (18, 22) with an acceptable sensitivity (76.5 and 87%) and a high NPV (95.4 and 96.4%). Nevertheless, Lourtet-Hascoëtt et al. (20) questioned these performances due to a significant number of false negative results (16/25) and highlighted inconsistent sensitivity and NPV, respectively, 36 and 74%. These 16 samples were all frozen and originated from 14 periprosthetic tissues and 2 articular fluids. In contrast, the three other series were performed on fresh tissue samples or extemporaneous periprosthetic tissue swabs directly processed in the peri-operative time. Furthermore, when applied to a larger prospective cohort of 213 patients and 639 osteoarticular specimens (19) and considering Xpert's performance from a patient point of view, only 6 out of 213 patients (2.8%) were misdiagnosed, among whom 5 were infected by MRCoNS strains. It is worth noting that rapid and appropriate antimicrobial adaptation could be delivered to 194 out of 213 patients (91%).
Reaching 100% accuracy for a diagnostic tool is unrealistic in routine practice, especially when the microbiologist must deal with all the bacterial subtleties related to BJI contexts. Although we highlighted the good performances of the MRSA/SA SSTI above, the limits of this test in terms of false positive and false negative results must be discussed, owing to their potential impact on the antimicrobial strategy. Indeed, a false positive assay may lead to an inappropriate prescription of a broad-spectrum molecule with individual and ecological side effects, and a loss of opportunity to heal in case of MSSA infection. Interestingly, false positive tests have rarely been observed in the seven studies (Table 1) including 5 and 10 reported cases of SA and MR detection, respectively. These cases were mainly related to bacterial DNA detection in patients with an anteriority of infection or who had prior antimicrobial therapy leading to sterile cultures. Finally, these false positive cases could be re-interpreted as a true positive one, highlighting the limit of the gold standard used for the evaluation of this molecular assay. Secondly, worse than a false positive result, a false negative one may jeopardize surgery and the implanted device, implying revision procedure and prolonged hospital cost and length of stay associated with a morbidity increase. These false negative cases were more frequent in MRCoNS infections (18–20). This slightly lower accuracy comparative to SA infections must be interpreted according to the assay's content (i.e., specific probes targeting SA and MRSA) and also physiopathological considerations relative to acute SA infections involving high inoculum of bacteria and chronic MRCoNS infections involving low amounts of biofilm embedded bacteria, not evenly distributed at the implant's surface. Consequently, in a chronic context, the number of samples analyzed should not be restricted to a single one for correct interpretation. Multiplying samples and PCR tests may entail a financial burden for clinical laboratories that should counterbalance the economic consequences of misdiagnosis for healthcare settings. So far, the number and the kind of osteoarticular specimens required for a contributive analysis have to be defined. Moreover, regarding the software's interpretation algorithm, the latter is configured for MRSA/SA detection in skin and soft tissue samples with positivity reports related to abundant bacterial load resulting in low PCR Ct values. Consequently, these criteria cannot be extrapolated to osteoarticular or MRCoNS infection contexts. Accordingly, in BJI indication, the microbiologist's appraisal is required to interpret amplification curves, and particularly late Ct values of the mecA gene, in order to not miss a positive sample (18, 19). Finally, other false negative results were also reported in cases of staphylococcal small colonies variants or polymicrobial infections, without any obvious explanations yet.
Although conventional culture is a perfectible gold standard, it remains the key method to document the infection and to provide an exhaustive antibiogram. The use of blood culture bottles has significantly reduced the time for micro-organism detection with a sensitivity increased to 87% (9) allowing, in the most favorable conditions, phenotypical antimicrobial data in 48 h. The aim of the MRSA/SA SSTI® assay is to reduce this time frame to a couple of hours by targeting the main resistance determinant, i.e., the mecA gene, which is decisive for empirical antimicrobial therapy adaptation. In contrast to the 16S rRNA PCR (34, 35) or other home-designed multiplex PCR panels (36), the Xpert's test targets a single bacterial genus but delivers results in 72 min (23) vs. 2 days and half a day, respectively. Xpert is also easily implementable in the routine workflow of a clinical laboratory, with a hand-on time of 2 min (23), and does not involve the use of complex molecular facilities nor dedicated technical supports. Alternatively, an equivalent concept is proposed by the automated multiplex PCR Unyvero i60 ITI (Curetis, Holzgerlingen, Germany). This cartridge system targets 52 pathogens at the genus level, among which are 15 bacteria and yeasts at the species level, and 19 antimicrobial resistance markers delivering available results in 5 h. This assay has been evaluated in PJI diagnosis in three studies (37–39), although MR detection accuracy was only addressed by Malandain et al. (38). Unfortunately, when tested on culture-positive samples, no more than 35% of blamecA gene amplifications were detected.
Collectively, these data fully support the relevance of the Xpert's assay in osteoarticular infections for rapid antimicrobial adaptation in the peri-operative time along with its applicability in routine practice. One may assume that this strategy of early and accurate diagnosis is cost-effective; however, this point has to be fully demonstrated for acute and chronic indications, respectively. Nowadays, the room for such new molecular methods in the diagnostic strategy of BJI remains to be clearly defined in clinical and microbiological guidelines.
MT drafted the manuscript. ES, CL, HM, J-TL, FD, TM, and HD reviewed the article and provided critical insights. All authors contributed to the article and approved the submitted version.
ES declares personal honoraria from Cepheid for speaker activity.
The remaining authors declare that the research was conducted in the absence of any commercial or financial relationships that could be construed as a potential conflict of interest.
We warmly thank Madeleine Engilof for her valuable advice.
1. Ferry T, Seng P, Mainard D, Jenny J-Y, Laurent F, Senneville E, et al. The CRIOAc healthcare network in France: a nationwide health ministry program to improve the management of bone and joint infection. Orthop Traumatol Surg Res. (2019) 105:185–90. doi: 10.1016/j.otsr.2018.09.016
2. Grammatico-Guillon L, Baron S, Gettner S, Lecuyer A-I, Gaborit C, Rosset P, et al. Bone and joint infections in hospitalized patients in France, 2008: clinical and economic outcomes. J Hosp Infect. (2012) 82:40–8. doi: 10.1016/j.jhin.2012.04.025
3. Aggarwal VK, Bakhshi H, Ecker NU, Parvizi J, Gehrke T, Kendoff D. Organism profile in periprosthetic joint infection: pathogens differ at two arthroplasty infection referral centers in Europe and in the United States. J Knee Surg. (2014) 27:399–406. doi: 10.1055/s-0033-1364102
4. Triffault-Fillit C, Ferry T, Laurent F, Pradat P, Dupieux C, Conrad A, et al. Microbiologic epidemiology depending on time to occurrence of prosthetic joint infection: a prospective cohort study. Clin Microbiol Infect. (2019) 25:353–8. doi: 10.1016/j.cmi.2018.04.035
5. Osmon DR, Berbari EF, Berendt AR, Lew D, Zimmerli W, Steckelberg JM, et al. Diagnosis and management of prosthetic joint infection: clinical practice guidelines by the Infectious Diseases Society of America. Clin Infect Dis. (2013) 56:e1 25. doi: 10.1093/cid/cis803
6. Valour F, Bouaziz A, Karsenty J, Ader F, Lustig S, Laurent F, et al. Determinants of methicillin-susceptible Staphylococcus aureus native bone and joint infection treatment failure: a retrospective cohort study. BMC Infect Dis. (2014) 14:443. doi: 10.1186/1471-2334-14-443
7. Tice AD, Hoaglund PA, Shoultz DA. Risk factors and treatment outcomes in osteomyelitis. J Antimicrob Chemother. (2003) 51:1261–8. doi: 10.1093/jac/dkg186
8. Schäfer P, Fink B, Sandow D, Margull A, Berger I, Frommelt L. Prolonged bacterial culture to identify late periprosthetic joint infection: a promising strategy. Clin Infect Dis. (2008) 47:1403–9. doi: 10.1086/592973
9. Peel TN, Dylla BL, Hughes JG, Lynch DT, Greenwood-Quaintance KE, Cheng AC, et al. Improved diagnosis of prosthetic joint infection by culturing periprosthetic tissue specimens in blood culture bottles. mBio. (2016) 7:e01776–15. doi: 10.1128/mBio.01776-15
10. Kurtz S, Ong K, Lau E, Mowat F, Halpern M. Projections of primary and revision hip and knee arthroplasty in the United States from 2005 to 2030. JBJS. (2007) 89:780–5. doi: 10.2106/JBJS.F.00222
11. Bozic KJ, Kurtz SM, Lau E, Ong K, Vail TP, Berry DJ. The epidemiology of revision total hip arthroplasty in the United States. JBJS. (2009) 91:128–33. doi: 10.2106/JBJS.H.00155
12. King MD, Humphrey BJ, Wang YF, Kourbatova EV, Ray SM, Blumberg HM. Emergence of community-acquired methicillin-resistant Staphylococcus aureus USA 300 clone as the predominant cause of skin and soft-tissue infections. Ann Intern Med. (2006) 144:309–17. doi: 10.7326/0003-4819-144-5-200603070-00005
13. Wolk DM, Struelens MJ, Pancholi P, Davis T, Della-Latta P, Fuller D, et al. Rapid detection of Staphylococcus aureus and methicillin-resistant S. aureus (MRSA) in wound specimens and blood cultures: multicenter preclinical evaluation of the Cepheid Xpert MRSA/SA skin and soft tissue and blood culture assays. J Clin Microbiol. (2009) 47:823–6. doi: 10.1128/JCM.01884-08
14. Katayama Y, Ito T, Hiramatsu K. A new class of genetic element, Staphylococcus Cassette Chromosome mec, Encodes Methicillin Resistance in Staphylococcus aureus. Antimicrob Agents Chemother. (2000) 44:1549–55. doi: 10.1128/AAC.44.6.1549-1555.2000
15. Hartman BJ, Tomasz A. Low-affinity penicillin-binding protein associated with beta-lactam resistance in Staphylococcus aureus. J Bacteriol. (1984) 158:513–6. doi: 10.1128/JB.158.2.513-516.1984
16. Hiramatsu K, Katayama Y, Yuzawa H, Ito T. Molecular genetics of methicillin-resistant Staphylococcus aureus. Int J Med Microbiol. (2002) 292:67–74. doi: 10.1078/1438-4221-00192
17. Searns JB, Robinson CC, Wei Q, Yuan J, Hamilton S, Pretty K, et al. Validation of a novel molecular diagnostic panel for pediatric musculoskeletal infections: integration of the Cepheid Xpert MRSA/SA SSTI and laboratory-developed real-time PCR assays for clindamycin resistance genes and Kingella kingae detection. J Microbiol Methods. (2019) 156:60–7. doi: 10.1016/j.mimet.2018.12.004
18. Titécat M, Loïez C, Senneville E, Wallet F, Dezèque H, Legout L, et al. Evaluation of rapid mecA gene detection versus standard culture in staphylococcal chronic prosthetic joint infections. Diagn Microbiol Infect Dis. (2012) 73:318–21. doi: 10.1016/j.diagmicrobio.2012.04.011
19. Titécat M, Wallet F, Robineau O, Valette M, Migaud H, Senneville E, et al. Focus on MRSA/SA SSTI assay failure in prosthetic joint infections: 213 consecutive patients later. J Clin Microbiol. (2017) 55:635–7. doi: 10.1128/JCM.01658-16
20. Lourtet-Hascoëtt J, Bicart-See A, Félicé MP, Giordano G, Bonnet E. Is Xpert MRSA/SA SSTI real-time PCR a reliable tool for fast detection of methicillin-resistant coagulase-negative staphylococci in periprosthetic joint infections? Diagn Microbiol Infect Dis. (2015) 83:59–62. doi: 10.1016/j.diagmicrobio.2015.04.009
21. Sambri A, Pignatti G, Romagnoli M, Donati D, Marcacci M, Cadossi M. Intraoperative diagnosis of Staphylococcus aureus and coagulase-negative Staphylococcus using Xpert MRSA/SA SSTI assay in prosthetic joint infection. New Microbiol. (2017) 4:130–4.
22. Valour F, Blanc-Pattin V, Freydière A-M, Bouaziz A, Chanard E, Lustig S, et al. Rapid detection of Staphylococcus aureus and methicillin resistance in bone and joint infection samples: evaluation of the GeneXpert MRSA/SA SSTI assay. Diagn Microbiol Infect Dis. (2014) 78:313–5. doi: 10.1016/j.diagmicrobio.2013.11.026
23. Dubouix-Bourandy A, Ladoucette A de, Pietri V, Mehdi N, Benzaquen D, Guinand R, et al. Direct detection of Staphylococcus osteoarticular infections by use of Xpert MRSA/SA SSTI real-time PCR. J Clin Microbiol. (2011) 49:4225–30. doi: 10.1128/JCM.00334-11
24. Roux A-L, Sivadon-Tardy V, Bauer T, Lortat-Jacob A, Herrmann J-L, Gaillard J-L, et al. Diagnosis of prosthetic joint infection by beadmill processing of a periprosthetic specimen. Clin Microbiol Infect. (2011) 17:447–50. doi: 10.1111/j.1469-0691.2010.03359.x
25. Lorrot M, Gillet Y, Gras Le Guen C, Launay E, Cohen R, Grimprel E. Antibiotic therapy of bone and joint infections in children: proposals of the French Pediatric Infectious Disease Group. Archives de Pédiatrie. (2017) 24(Suppl. 12):S36–41. doi: 10.1016/S0929-693X(17)30517-1
26. Metsemakers WJ, Kuehl R, Moriarty TF, Richards RG, Verhofstad MHJ, Borens O, et al. Infection after fracture fixation: current surgical and microbiological concepts. Injury. (2018) 49:511–22. doi: 10.1016/j.injury.2016.09.019
27. Weiner-Lastinger LM, Abner S, Edwards JR, Kallen AJ, Karlsson M, Magill SS, et al. Antimicrobial-resistant pathogens associated with adult healthcare-associated infections: summary of data reported to the National Healthcare Safety Network, 2015-2017. Infect Control Hosp Epidemiol. (2020) 41:1–18. doi: 10.1017/ice.2019.296
28. Parvizi J, Pawasarat IM, Azzam KA, Joshi A, Hansen EN, Bozic KJ. Periprosthetic joint infection: the economic impact of methicillin-resistant infections. J Arthroplasty. (2010) 25(Suppl. 6):103–7. doi: 10.1016/j.arth.2010.04.011
29. European Centre for Disease Prevention and Control. Healthcare-associated infections: surgical site infections. In: ECDC, editor. Annual Epidemiological Report for 2017. Stockholm: ECDC (2019).
30. Rosteius T, Jansen O, Fehmer T, Baecker H, Citak M, Schildhauer TA, et al. Evaluating the microbial pattern of periprosthetic joint infections of the hip and knee. J Med Microbiol. (2018) 67:1608–13. doi: 10.1099/jmm.0.000835
31. AlQahtani H, Alqahtani FY, Aleanizy FS, Baloch S, Tabb D. Impact of rapid identification of Staphylococcus species in positive blood culture using GeneXpert methicillin-resistant Staphylococcus aureus/ Staphylococcus aureus blood culture assay combined with antibiotic stewardship. Microb Drug Resist. (2021) doi: 10.1089/mdr.2020.0347. [Epub ahead of print].
32. Høiby N, Bjarnsholt T, Moser C, Bassi GL, Coenye T, Donelli G, et al. ESCMID guideline for the diagnosis and treatment of biofilm infections 2014. Clin Microbiol Infect. (2015) 21:S1–25. doi: 10.1016/j.cmi.2014.10.024
33. Triffault-Fillit C, Valour F, Guillo R, Tod M, Goutelle S, Lustig S, et al. Prospective cohort study of the tolerability of prosthetic joint infection empirical antimicrobial therapy. Antimicrob Agents Chemother. (2018) 62:e00163–18. doi: 10.1128/AAC.00163-18
34. Bémer P, Plouzeau C, Tande D, Léger J, Giraudeau B, Valentin AS, et al. Evaluation of 16S rRNA gene PCR sensitivity and specificity for diagnosis of prosthetic joint infection: a prospective multicenter cross-sectional study. J Clin Microbiol. (2014) 52:3583–9. doi: 10.1128/JCM.01459-14
35. Jacquier H, Fihman V, Amarsy R, Vicaut E, Bousson V, Cambau E, et al. Benefits of polymerase chain reaction combined with culture for the diagnosis of bone and joint infections: a prospective test performance study. Open Forum Infect Dis. (2019) 6:ofz511. doi: 10.1093/ofid/ofz511
36. Cazanave C, Greenwood-Quaintance KE, Hanssen AD, Karau MJ, Schmidt SM, Urena EOG, et al. Rapid molecular microbiologic diagnosis of prosthetic joint infection. J Clin Microbiol. (2013) 51:2280–7. doi: 10.1128/JCM.00335-13
37. Borde JP, Häcker GA, Guschl S, Serr A, Danner T, Hübner J, et al. Diagnosis of prosthetic joint infections using UMD-Universal Kit and the automated multiplex-PCR Unyvero i60 ITI® cartridge system: a pilot study. Infection. (2015) 43:551–60. doi: 10.1007/s15010-015-0796-4
38. Malandain D, Bémer P, Leroy AG, Léger J, Plouzeau C, Valentin AS, et al. Assessment of the automated multiplex-PCR Unyvero i60 ITI® cartridge system to diagnose prosthetic joint infection: a multicentre study. Clin Microbiol Infect. (2018) 24:83.e1–e6. doi: 10.1016/j.cmi.2017.05.017
Keywords: bone and joint infections, methicillin resistance, PCR, conventionnal culture, Xpert MRSA/SA SSTI®
Citation: Titécat M, Loïez C, Demaeght F, Leclerc J-T, Martin T, Dezèque H, Migaud H and Senneville E (2021) Challenging Methicillin Resistance Detection in Bone and Joint Infections: Focus on the MRSA/SA SSTI® Strategy. Front. Med. 8:553965. doi: 10.3389/fmed.2021.553965
Received: 20 April 2020; Accepted: 08 April 2021;
Published: 17 May 2021.
Edited by:
Alex Soriano, Hospital Clínic de Barcelona, SpainReviewed by:
Dolors Rodriguez-Pardo, Vall d'Hebron University Hospital, SpainCopyright © 2021 Titécat, Loïez, Demaeght, Leclerc, Martin, Dezèque, Migaud and Senneville. This is an open-access article distributed under the terms of the Creative Commons Attribution License (CC BY). The use, distribution or reproduction in other forums is permitted, provided the original author(s) and the copyright owner(s) are credited and that the original publication in this journal is cited, in accordance with accepted academic practice. No use, distribution or reproduction is permitted which does not comply with these terms.
*Correspondence: Marie Titécat, bWFyaWUudGl0ZWNhdEBjaHJ1LWxpbGxlLmZy
Disclaimer: All claims expressed in this article are solely those of the authors and do not necessarily represent those of their affiliated organizations, or those of the publisher, the editors and the reviewers. Any product that may be evaluated in this article or claim that may be made by its manufacturer is not guaranteed or endorsed by the publisher.
Research integrity at Frontiers
Learn more about the work of our research integrity team to safeguard the quality of each article we publish.