- Department of Pathology, University of Michigan Medical School, Ann Arbor, MI, United States
The wide use of the mouse model of polymicrobial sepsis has provided important evidence for events occurring in infectious sepsis involving septic mice and septic humans. Nearly 100 clinical trials in humans with sepsis have been completed, yet there is no FDA-approved drug. Our studies of polymicrobial sepsis have highlighted the role of complement activation products (especially C5a anaphylatoxin and its receptors C5aR1 and C5aR2) in adverse effects of sepsis. During sepsis, the appearance of these complement products is followed by appearance of extracellular histones in plasma, which have powerful proinflammatory and prothrombotic activities that cause cell injury and multiorgan dysfunction in septic mice. Similar responses occur in septic humans. Histone appearance in plasma is related to complement activation and appearance of C5a and its interaction with its receptors. Development of the cardiomyopathy of sepsis also depends on C5a, C5a receptors and histones. Neutralization of C5a with antibody or absence of C5aR1 blocks appearance of extracellular histones and cell and organ failure in sepsis. Survival rates in septic mice are greatly improved after blockade of C5a with antibody. We also review the various strategies in sepsis that greatly reduce the development of life-threatening events of sepsis.
Background
Development of infectious sepsis in humans often results in a series of events that can lead to death. In spite of nearly 100 clinical trials, no drug has been approved by the FDA for use in septic patients. The mouse model of infectious sepsis is polymicrobial sepsis produced by cecal ligation and puncture (CLP). This model has been widely used for four decades (1, 2) because it appears to mimic events occurring in septic humans. In the early phases of polymicrobial sepsis (first 1–3 days), there is hyperactivation of the innate immune system, releasing a flood of cytokines, chemokines, and extracellular histones, all of which cause injury to cells and organs (especially lungs, liver, spleen, heart, kidneys, and the central nervous system) (3–5). It is also established that aged septic mice (20–24 months of age) or septic humans (>60 years of age) have more severe sepsis and much greater lethality when compared to younger mice or humans, respectively (6–10). Reasons for these responses are not established, except, perhaps, for the fact that aged mice or humans have progressive impairments of the innate immune system (7, 11, 12). Beginning around 3 days after onset of sepsis, the innate immune system becomes progressively non-responsive, and immunosuppression sets in (5, 13). If the septic mouse or human is able to contain these responses, within about a week the innate immune system recovers, immunosuppression subsides and organ dysfunction is reversed, with return to the pre-septic state (14). It has recently been shown that, after discharge of patients from the hospital, there is evidence of residual medical problems in many individuals, especially the elderly (15, 16). For instance, nearly 50% of these individuals have a shortened life span over a 2-year period post-sepsis, resulting in a doubling of death rates when compared to a non-septic cohort. Obviously, there is a great deal to be learned about events in sepsis and how survival can be improved. Septic mice with various genetic manipulations appear to be critical resources for such studies. While the NIH/NIGMS has discouraged the use of mice for sepsis studies and is no longer engaged in supporting sepsis studies in humans, we have been able to identify mechanisms in septic mice that can be extrapolated to septic humans. Studies such as neutralization of histones or C5a have been shown to greatly improve survival in septic mice, employing interventions that could not be done in septic humans.
Polymicrobial Sepsis Model and Blockade Strategies Targeting C5aRs Or Extracellular Histones
Most of the septic studies in mice use the polymicrobial sepsis model with CLP as the standard procedure (17, 18). The polymicrobial experimental model mimics sepsis in humans and is helpful for understanding the sepsis process in the human body (19, 20). In human sepsis, there is excessive C5a generation associated with inflammatory responses. In 2009, Xu et al. described the role of histones in mice with polymicrobial sepsis, endotoxemia or infusion of TNF (21). We have shown the harmful effects of C5a in septic mice, resulting in cardiomyopathy and cardiac dysfunction (22, 23). Blockade of C5a or its receptors (mainly C5aR1) significantly preserved heart dysfunction in septic mice. The same result developed in septic mice lacking C5a receptors (C5aR1 or C5aR2) (23). In these studies, cardiovascular performance was measured by ECHO/Doppler technology in mice before and 8 h after induction of CLP. Echocardiograms were also obtained before and after sepsis, according to the recommendations of the American Society of Echocardiography (23). Cardiac performance (especially isovolumic relaxation time) showed preserved levels of this parameter in the septic mice which lacked either C5a receptor (especially C5aR1) compared to the septic wild type mice (23).
Another strategy for reducing the harmful effects of sepsis was neutralization of extracellular histones (with clone BWA3 antibody targeting H2A/H4). Our functional studies showed remarkably reduced cardiac dysfunction in septic mice. Septic mice receiving this neutralizing antibody against histones showed preserved cardiac function as measured by Echo/Doppler studies (24).
Phases of Polymicrobial Sepsis
It is now well-established in polymicrobial sepsis in mice that there is an early phase of robust activation of the innate immune system (1–3 days after CLP) during which time neutrophils (PMNs) and monocytes/macrophages release powerful proinflammatory mediators (TNF, IL-6, IL-1β, IL-17, etc.) in a “cytokine burst” that causes cell damage and multiorgan dysfunction, especially affecting kidneys, heart, liver, and other organs (13, 25, 26). As polymicrobial sepsis progresses, many of the proinflammatory responses are attenuated as immunosuppression develops at 3–7 days, resulting in reduced innate immune cellular responses which may compromise the natural protective responses that combat a variety of infectious agents (bacterial, fungal, viral) (5, 27, 28). If the protective immune responses are adequate, the inflammatory responses subside and mice are returned to health within 7 days, including recovery of organs from damage and reversion to the pre-sepsis state (14, 29).
The early phases of sepsis are associated with strong activation of the three complement pathways (classical, alternative, and lectin), generating a variety of strong proinflammatory peptides, especially anaphylatoxins C3a and C5a. C3a was originally identified by its ability to react with its receptor (C3aR), resulting in increased vascular permeability and smooth muscle contraction in a variety of tissues (3, 30, 31). The other anaphylatoxin, C5a, was recognized as a very powerful activator of PMNs and macrophages. Following its rapid binding to C5a receptors (C5aR1 and C5aR2), C5a·C5aR1 interaction causes activation of both PMNs and macrophages, the result being release of proinflammatory cytokines and chemokines, chemotaxis, generation of powerful and harmful oxygen-free radicals, and release of a variety of enzymes and lipid mediators that positively and negatively modify inflammatory responses (32–34).
Sepsis-Induced Release of Histones and Mechanisms of Cell and Organ Damage Related to Histones
It is commonly found that septic mice and septic humans develop activation of all three complement pathways (classical, alternative and lectin). As emphasized in Figure 1, early in sepsis in both mice and humans (over the first 24 h), via C5a·C5aR1 interactions, there is extensive cell and organ damage related to the surge in plasma of proinflammatory peptides. In addition, there is also an early (1–3 days) appearance in plasma of IL-1β associated with activation of the NLRP3 inflammasome in PMNs and macrophages (35, 36). TNF, IL-6, and the IL-17 family of factors are early proinflammatory peptides appearing in plasma (13, 37, 38). The role of complement and C5a and its receptors in activation of the NLRP3 inflammasome in septic mice has been described (39), as well as NLRP3-induced activation of CD4+ T cells (40). As time progresses, like the rest of the innate system, the NLRP3 inflammasome becomes functionally defective, resulting in diminished release of IL-1β. We have recently shown that the cardiac dysfunction and proinflammatory cytokines (including IL-1β) levels are significantly diminished in septic mice lacking NLRP3 (35). We also have shown the levels of extracellular histones were significantly lower in plasma from mice lacking NLRP3 or C5a receptors (24). It has been demonstrated that sepsis causes in PMNs the appearance of neutrophil extracellular traps (NETs) and in macrophages the appearance of macrophage extracellular traps (METs) (41–44). In both cases, these traps cause adherence of bacteria to the traps resulting in bacterial killing. At the same time, these traps contain a host of products from leukocyte granules, such as proteases, proinflammatory peptides as well as extracellular histones, which are strongly proinflammatory and prothrombotic, the composite resulting in extensive injury to cells and organs (41–43, 45).
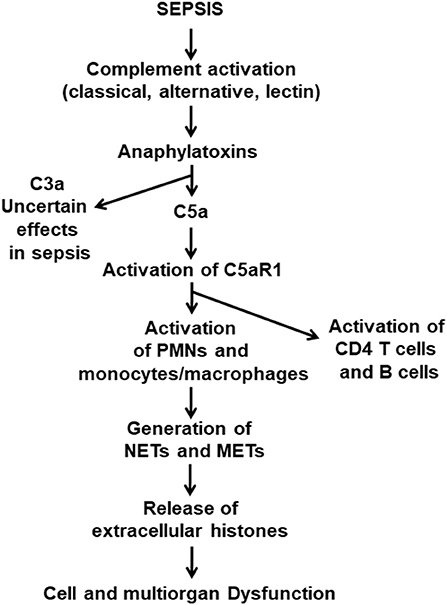
Figure 1. Pathophysiological consequences of sepsis. This figure is a composite of what is currently known about the pathophysiology of polymicrobial sepsis in mice. Onset of sepsis is associated with activation of the three pathways of complement activation, which results in appearance of the anaphylatoxins, C3a and C5a. Both anaphylatoxins have strong proinflammatory effects, and both interact with their receptors, C3aR and C5aR1 and C5aR2, especially on PMNs and macrophages. The literature indicates that the C5a axis of activation, involving C5aR1 and C5aR2, causes proinflammatory responses that are harmful to cells and organs. In addition, C5a interaction with C5aR1 activates PMNs and monocytes and macrophages which form NETs and METs that are strongly proinflammatory and prothrombotic. Similar events occur in septic humans.
Biological Roles of Extracellular Histones in Sepsis
In the setting of sepsis, many of the proinflammatory and thrombogenic events affecting cells and organs can be attributed to extracellular histones which derive from activated PMNs and macrophages (41–44). We have shown numerous biological responses, including vascular leakage, buildup of PMNs and macrophages in organs, cell swelling and cytotoxic outcomes, LDH release, increased [Ca2+]i in cells, and release of cytokines and chemokines (46). We have also shown the biological activities related to purified individual histones (H1, H2A, H2B, H3, H4) (46). We have not yet determined to what extent various biochemical changes that develop in histone proteins (acetylation, methylation and ubiquination) affect the biological function of extracellular histones, but this likely happens in a manner that amplifies or reduces biological responses, induced by histones.
Role of Toll-Like Receptors (TLRs) in Biological Responses in Sepsis
It has been known for some time that TLRs function as pattern recognition receptors and are critical for numerous responses of the innate immune system. TLRs are also involved in responses to LPS, with most evidence suggesting that TLR4 is critical for cell responses to LPS (47). We have recently shown that in septic mice, as early as 8 h after onset of sepsis, cardiomyocytes develop dysfunction and that such dysfunction can be linked to the role of complement activation, C5a and its receptors, as well as extracellular histones, causing cardiomyocyte dysfunction (24). We have seen decreased levels of [Ca2+]i in the cardiomyocytes from knockout (KO) of either TLR2 or TLR4 after infusion of the histones, suggesting these receptors may be linked to interactions with histones (24).
Regarding other TLRs, we have also shown that the full development of cardiac dysfunction developing in septic mice requires the presence of both TLR3 and TLR9 (48). Septic mice with KO of either TLR3 or TLR9 have attenuated cardiac dysfunction during sepsis, but the precise pathways responsible for such changes are not currently known. However, in recent studies, cardiomyocytes (from normal mice) exposed to the histone mix showed release of LDH and the septic hearts showed release of proinflammatory cytokines (TNF, IL-6, and IL-1β) that are greatly diminished in hearts from TLR3 or TLR9 KO mice (48). Obviously, much more data are needed in terms of roles of TLRs in sepsis.
Protective Interventions in Septic Mice and Septic Humans
Based on the information in Figure 1, there are numerous interventions that protect mice from the damaging effects of infectious sepsis.
Complement Blockade
This has been done in septic mice using a variety of strategies, including KO of key components (49–51) for each of the three pathways (classical, alternative, lectin) of complement activation. The problem with such interventions is that there are extensive interactions involving activation products from each pathway reacting positively with all three pathways of complement. Accordingly, specific and limited pathway blockade of a single complement pathway does not often occur under such circumstances. The exception may be the C1 esterase inhibitor which blocks C1 of the classical pathway (52). This inhibitor has been used in human septic patients (53, 54), but the results have not been especially impressive. C1 esterase inhibitor is currently not used to treat septic humans (55).
Blockade Involving C5 or C5a Receptors
The absence (by KO) of C5 or its blockade with a mAb blocks both C5a and C5b-9 generation as obvious targets (49–51). Blockade of C5 with mAb has been shown to block inflammatory responses in rheumatoid arthritis (56, 57). The use of mAb to C5 has also been approved in patients with paroxysmal nocturnal hemoglobinuria (58) as well as patients with myasthenia gravis (59). Using KO mice, the absence of C5aR1 has been shown to block many of the harmful outcomes in septic mice (23, 51). KO of C5aR1 substantially reduces the harmful effects of sepsis in mice, reducing multiorgan injury and greatly improving survival after CLP (23, 51, 60). There are several companies that have developed small molecular weight inhibitors for C5aR1, but to date none have been approved for use in septic humans. The second C5a receptor, C5aR2 was originally described as a C5a “default receptor,” functioning as a compound that binds C5a, resulting in the absence of any signal-transduction response. There is conflicting information in the literature about the biological role of C5aR2 (formerly known as C5L2) (61, 62). Until this conflict is resolved, it seems unlikely that C5aR2 will be a target for pharmaceutical companies.
Blockade of Proinflammatory Peptides or Their Receptors in Sepsis
While blockade of TNF or its receptor, or mAb to C5 has been effective in patients with paroxysmal nocturnal hemoglobinuria or myasthenia gravis, similar interventions have not been successful in the setting of sepsis in mice or humans. This is probably due to the presence of numerous other proinflammatory peptides appearing in septic humans with similar biological activities.
Blockade of Histones in the Setting of Sepsis
As indicated above, it is now clear that extracellular histones derived from activated PMNs or macrophages in septic mice (or septic humans) appear in the plasma of septic mice or septic humans in the early phases of sepsis (1–3 days) in substantial amounts (25 μg/ml) as determined by immunological assays or by mass spectrometry (24, 63, 64). In septic mice, PMNs and macrophages appear to be the chief source of the extracellular histones. As indicated above, these histones are intensely proinflammatory and prothrombotic. Our studies indicate that appearance of extracellular histones in sepsis is complement and C5aR1-dependent. This suggests an alternative strategy to block appearance of extracellular histones in the setting of sepsis. However, much more information will be needed before interventions of histones in septic humans can be considered.
Author Contributions
FZ and PW were involved in the work in mice with polymicrobial sepsis. Both participated in the writing and review of this report.
Funding
Funding provided by the National Institutes of Health, National Institute of Aging grant award R56-AG061207.
Conflict of Interest
The authors declare that the research was conducted in the absence of any commercial or financial relationships that could be construed as a potential conflict of interest.
References
1. Hubbard WJ, Choudhry M, Schwacha MG, Kerby JD, Rue LW, III, Bland KI, et al. Cecal ligation and puncture. Shock. (2005) 24:52–7. doi: 10.3791/2860
2. Wichterman KA, Baue AE, Chaudry IH. Sepsis and septic shock–a review of laboratory models and a proposal. J Surg Res. (1980) 29:189–201. doi: 10.1016/0022-4804(80)90037-2
3. Rittirsch D, Flierl MA, Ward PA. Harmful molecular mechanisms in sepsis. Nat Rev Immunol. (2008) 8:776–87. doi: 10.1038/nri2402
4. Bozza FA, Salluh JI, Japiassu AM, Soares M, Assis EF, Gomes RN, et al. Cytokine profiles as markers of disease severity in sepsis: a multiplex analysis. Crit Care. (2007) 11:R49. doi: 10.1186/cc5783
5. Boomer JS, To K, Chang KC, Takasu O, Osborne DF, Walton AH, et al. Immunosuppression in patients who die of sepsis and multiple organ failure. JAMA. (2011) 306:2594–605. doi: 10.1001/jama.2011.1829
6. Nacionales DC, Gentile LF, Vanzant E, Lopez MC, Cuenca A, Cuenca AG, et al. Aged mice are unable to mount an effective myeloid response to sepsis. J Immunol. (2014) 192:612–22. doi: 10.4049/jimmunol.1302109
7. Starr ME, Saito H. Sepsis in old age: review of human and animal studies. Aging Dis. (2014) 5:126–36. doi: 10.14336/AD.2014.0500126
8. Martin GS, Mannino DM, Moss M. The effect of age on the development and outcome of adult sepsis. Crit Care Med. (2006) 34:15–21. doi: 10.1097/01.CCM.0000194535.82812.BA
9. Angus DC, Linde-Zwirble WT, Lidicker J, Clermont G, Carcillo J, Pinsky MR. Epidemiology of severe sepsis in the united states: Analysis of incidence, outcome, and associated costs of care. Crit Care Med. (2001) 29:1303–10. doi: 10.1097/00003246-200107000-00002
10. Nasa P, Juneja D, Singh O. Severe sepsis and septic shock in the elderly: an overview. World J Crit Care Med. (2012) 1:23–30. doi: 10.5492/wjccm.v1.i1.23
11. Turnbull IR, Clark AT, Stromberg PE, Dixon DJ, Woolsey CA, Davis CG, et al. Effects of aging on the immunopathologic response to sepsis. Crit Care Med. (2009) 37:1018–23. doi: 10.1097/CCM.0b013e3181968f3a
12. Brakenridge SC, Efron PA, Stortz JA, Ozrazgat-Baslanti T, Ghita G, Wang Z, et al. The impact of age on the innate immune response and outcomes after severe sepsis/septic shock in trauma and surgical intensive care unit patients. J Trauma Acute Care Surg. (2018) 85:247–55. doi: 10.1097/TA.0000000000001921
13. Chaudhry H, Zhou J, Zhong Y, Ali MM, McGuire F, Nagarkatti PS, et al. Role of cytokines as a double-edged sword in sepsis. In Vivo. (2013) 27:669–84.
14. Delano MJ, Ward PA. The immune system's role in sepsis progression, resolution, and long-term outcome. Immunol Rev. (2016) 274:330–53. doi: 10.1111/imr.12499
15. Shankar-Hari M, Rubenfeld GD. Understanding long-term outcomes following sepsis: implications and challenges. Curr Infect Dis Rep. (2016) 18:37. doi: 10.1007/s11908-016-0544-7
16. Cuthbertson BH, Elders A, Hall S, Taylor J, MacLennan G, Mackirdy F, et al. Mortality and quality of life in the five years after severe sepsis. Crit Care. (2013) 17:R70. doi: 10.1186/cc12616
17. Dejager L, Pinheiro I, Dejonckheere E, Libert C. Cecal ligation and puncture: the gold standard model for polymicrobial sepsis? Trends Microbiol. (2011) 19:198–208. doi: 10.1016/j.tim.2011.01.001
18. Rittirsch D, Huber-Lang MS, Flierl MA, Ward PA. Immunodesign of experimental sepsis by cecal ligation and puncture. Nat Protoc. (2009) 4:31–6. doi: 10.1038/nprot.2008.214
19. Efron PA, Darden DB, Wang Z, Nacionales DC, Lopez MC, Hawkins RB, et al. Transcriptomic responses from improved murine sepsis models can better mimic human surgical sepsis. FASEB J. (2020). doi: 10.1096/fj.202002150R. [Epub ahead of print].
20. Stortz JA, Hollen MK, Nacionales DC, Horiguchi H, Ungaro R, Dirain ML, et al. Old mice demonstrate organ dysfunction as well as prolonged inflammation, immunosuppression, and weight loss in a modified surgical sepsis model. Crit Care Med. (2019) 47:e919–29. doi: 10.1097/CCM.0000000000003926
21. Xu J, Zhang X, Pelayo R, Monestier M, Ammollo CT, Semeraro F, et al. Extracellular histones are major mediators of death in sepsis. Nat Med. (2009) 15:1318–21. doi: 10.1038/nm.2053
22. Niederbichler AD, Hoesel LM, Westfall MV, Gao H, Ipaktchi KR, Sun L, et al. An essential role for complement c5a in the pathogenesis of septic cardiac dysfunction. J Exp Med. (2006) 203:53–61. doi: 10.1084/jem.20051207
23. Kalbitz M, Fattahi F, Herron TJ, Grailer JJ, Jajou L, Lu H, et al. Complement destabilizes cardiomyocyte function in vivo after polymicrobial sepsis and in vitro. J Immunol. (2016) 197:2353–61. doi: 10.4049/jimmunol.1600091
24. Kalbitz M, Grailer JJ, Fattahi F, Jajou L, Herron TJ, Campbell KF, et al. Role of extracellular histones in the cardiomyopathy of sepsis. FASEB J. (2015) 29:2185–93. doi: 10.1096/fj.14-268730
25. Bone RC. Toward an epidemiology and natural history of sirs (systemic inflammatory response syndrome). JAMA. (1992) 268:3452–5. doi: 10.1001/jama.268.24.3452
26. Riedemann NC, Guo RF, Neff TA, Laudes IJ, Keller KA, Sarma VJ, et al. Increased c5a receptor expression in sepsis. J Clin Invest. (2002) 110:101–8. doi: 10.1172/JCI0215409
27. Hotchkiss RS, Monneret G, Payen D. Immunosuppression in sepsis: a novel understanding of the disorder and a new therapeutic approach. Lancet Infect Dis. (2013) 13:260–8. doi: 10.1016/S1473-3099(13)70001-X
28. Fattahi F, Ward PA. Understanding immunosuppression after sepsis. Immunity. (2017) 47:3–5. doi: 10.1016/j.immuni.2017.07.007
29. Xiao H, Siddiqui J, Remick DG. Mechanisms of mortality in early and late sepsis. Infect Immun. (2006) 74:5227–35. doi: 10.1128/IAI.01220-05
30. Foreman KE, Glovsky MM, Warner RL, Horvath SJ, Ward PA. Comparative effect of c3a and c5a on adhesion molecule expression on neutrophils and endothelial cells. Inflammation. (1996) 20:1–9. doi: 10.1007/BF01487740
31. Flierl MA, Rittirsch D, Nadeau BA, Day DE, Zetoune FS, Sarma JV, et al. Functions of the complement components c3 and c5 during sepsis. FASEB J. (2008) 22:3483–90. doi: 10.1096/fj.08-110595
32. Guo RF, Riedemann NC, Ward PA. Role of c5a-c5ar interaction in sepsis. Shock. (2004) 21:1–7. doi: 10.1097/01.shk.0000105502.75189.5e
33. Riedemann NC, Guo RF, Ward PA. A key role of c5a/c5ar activation for the development of sepsis. J Leukoc Biol. (2003) 74:966–70. doi: 10.1189/jlb.0403137
34. Huber-Lang MS, Sarma JV, McGuire SR, Lu KT, Padgaonkar VA, Younkin EM, et al. Structure-function relationships of human c5a and c5ar. J Immunol. (2003) 170:6115–24. doi: 10.4049/jimmunol.170.12.6115
35. Kalbitz M, Fattahi F, Grailer JJ, Jajou L, Malan EA, Zetoune FS, et al. Complement-induced activation of the cardiac nlrp3 inflammasome in sepsis. FASEB J. (2016) 30:3997–4006. doi: 10.1096/fj.201600728R
36. Grailer JJ, Canning BA, Kalbitz M, Haggadone MD, Dhond RM, Andjelkovic AV, et al. Critical role for the nlrp3 inflammasome during acute lung injury. J Immunol. (2014) 192:5974–83. doi: 10.4049/jimmunol.1400368
37. Bosmann M, Ward PA. Therapeutic potential of targeting il-17 and il-23 in sepsis. Clin Transl Med. (2012) 1:4. doi: 10.1186/2001-1326-1-4
38. Blackwell TS, Christman JW. Sepsis and cytokines: current status. Br J Anaesth. (1996) 77:110–7. doi: 10.1093/bja/77.1.110
39. Fattahi F, Grailer JJ, Parlett M, Lu H, Malan EA, Abe E, et al. Requirement of complement c6 for intact innate immune responses in mice. J Immunol. (2020) 205:251–60. doi: 10.4049/jimmunol.1900801
40. Arbore G, West EE, Spolski R, Robertson AAB, Klos A, Rheinheimer C, et al. T helper 1 immunity requires complement-driven nlrp3 inflammasome activity in cd4(+) t cells. Science. (2016) 352:aad1210. doi: 10.1126/science.aad1210
41. Fattahi F, Grailer JJ, Jajou L, Zetoune FS, Andjelkovic AV, Ward PA. Organ distribution of histones after intravenous infusion of fitc histones or after sepsis. Immunol Res. (2015) 61:177–86. doi: 10.1007/s12026-015-8628-2
42. Doster RS, Rogers LM, Gaddy JA, Aronoff DM. Macrophage extracellular traps: a scoping review. J Innate Immun. (2018) 10:3–13. doi: 10.1159/000480373
43. Brinkmann V, Reichard U, Goosmann C, Fauler B, Uhlemann Y, Weiss DS, et al. Neutrophil extracellular traps kill bacteria. Science. (2004) 303:1532–5. doi: 10.1126/science.1092385
44. Fattahi F, Zetoune FS, Ward PA. Complement as a major inducer of harmful events in infectious sepsis. Shock. (2020) 54:595–605. doi: 10.1097/SHK.0000000000001531
45. Saffarzadeh M, Juenemann C, Queisser MA, Lochnit G, Barreto G, Galuska SP, et al. Neutrophil extracellular traps directly induce epithelial and endothelial cell death: a predominant role of histones. PLoS ONE. (2012) 7:e32366. doi: 10.1371/journal.pone.0032366
46. Fattahi F, Grailer JJ, Lu H, Dick RS, Parlett M, Zetoune FS, et al. Selective biological responses of phagocytes and lungs to purified histones. J Innate Immun. (2017) 9:300–17. doi: 10.1159/000452951
47. Park BS, Lee JO. Recognition of lipopolysaccharide pattern by tlr4 complexes. Exp Mol Med. (2013) 45:e66. doi: 10.1038/emm.2013.97
48. Fattahi F, Russell MW, Malan EA, Parlett M, Abe E, Zetoune FS, et al. Harmful roles of tlr3 and tlr9 in cardiac dysfunction developing during polymicrobial sepsis. Biomed Res Int. (2018) 2018:4302726. doi: 10.1155/2018/4302726
49. Czermak BJ, Sarma V, Pierson CL, Warner RL, Huber-Lang M, Bless NM, et al. Protective effects of c5a blockade in sepsis. Nat Med. (1999) 5:788–792. doi: 10.1038/10512
50. Huber-Lang MS, Sarma JV, McGuire SR, Lu KT, Guo RF, Padgaonkar VA, et al. Protective effects of anti-c5a peptide antibodies in experimental sepsis. FASEB J. (2001) 15:568–70. doi: 10.1096/fj.00-0653fje
51. Rittirsch D, Flierl MA, Nadeau BA, Day DE, Huber-Lang M, Mackay CR, et al. Functional roles for c5a receptors in sepsis. Nat Med. (2008) 14:551–7. doi: 10.1038/nm1753
52. Singer M, Jones AM. Bench-to-bedside review: the role of c1-esterase inhibitor in sepsis and other critical illnesses. Crit Care. (2011) 15:203. doi: 10.1186/cc9304
53. Igonin AA, Protsenko DN, Galstyan GM, Vlasenko AV, Khachatryan NN, Nekhaev IV, et al. C1-esterase inhibitor infusion increases survival rates for patients with sepsis. Crit Care Med. (2012) 40:770–7. doi: 10.1097/CCM.0b013e318236edb8
54. Hirose T, Ogura H, Takahashi H, Ojima M, Jinkoo K, Nakamura Y, et al. Serial change of c1 inhibitor in patients with sepsis: a prospective observational study. J Intensive Care. (2018) 6:37. doi: 10.1186/s40560-018-0309-5
55. Schlapbach LJ, Schibler A, Jensenius JC. C1-esterase inhibitor treatment in sepsis-can we target the right patients? Crit Care Med. (2012) 40:2735–6; author reply 2736–2737. doi: 10.1097/CCM.0b013e318258eb7a
56. Wang Y, Rollins SA, Madri JA, Matis LA. Anti-c5 monoclonal antibody therapy prevents collagen-induced arthritis and ameliorates established disease. Proc Natl Acad Sci USA. (1995) 92:8955–9. doi: 10.1073/pnas.92.19.8955
57. Hornum L, Hansen AJ, Tornehave D, Fjording MS, Colmenero P, Watjen IF, et al. C5a and c5ar are elevated in joints of rheumatoid and psoriatic arthritis patients, and c5ar blockade attenuates leukocyte migration to synovial fluid. PLoS ONE. (2017) 12:e0189017. doi: 10.1371/journal.pone.0189017
58. Hillmen P, Young NS, Schubert J, Brodsky RA, Socie G, Muus P, et al. The complement inhibitor eculizumab in paroxysmal nocturnal hemoglobinuria. N Engl J Med. (2006) 355:1233–43. doi: 10.1056/NEJMoa061648
59. Albazli K, Kaminski HJ, Howard JF, Jr. Complement inhibitor therapy for myasthenia gravis. Front Immunol. (2020). 11:917. doi: 10.3389/fimmu.2020.00917
60. Atefi G, Zetoune FS, Herron TJ, Jalife J, Bosmann M, Al-Aref R, et al. Complement dependency of cardiomyocyte release of mediators during sepsis. FASEB J. (2011) 25:2500–8. doi: 10.1096/fj.11-183236
61. Huber-Lang M, Sarma JV, Rittirsch D, Schreiber H, Weiss M, Flierl M, et al. Changes in the novel orphan, c5a receptor (c5l2), during experimental sepsis and sepsis in humans. J Immunol. (2005) 174:1104–10. doi: 10.4049/jimmunol.174.2.1104
62. Li XX, Lee JD, Kemper C, Woodruff TM. The complement receptor c5ar2: A powerful modulator of innate and adaptive immunity. J Immunol. (2019) 202:3339–48. doi: 10.4049/jimmunol.1900371
63. Garcia-Gimenez JL, Roma-Mateo C, Carbonell N, Palacios L, Peiro-Chova L, Garcia-Lopez E, et al. A new mass spectrometry-based method for the quantification of histones in plasma from septic shock patients. Sci Rep. (2017) 7:10643. doi: 10.1038/s41598-017-10830-z
Keywords: anaphylatoxins, histones, NLRP3 inflammasome, ROS, NETs, METs, C5a, C5b-9
Citation: Zetoune FS and Ward PA (2020) Role of Complement and Histones in Sepsis. Front. Med. 7:616957. doi: 10.3389/fmed.2020.616957
Received: 13 October 2020; Accepted: 30 November 2020;
Published: 23 December 2020.
Edited by:
Alessandro Russo, University of Pisa, ItalyReviewed by:
Philip Alexander Efron, University of Florida, United StatesPeter Monk, The University of Sheffield, United Kingdom
Copyright © 2020 Zetoune and Ward. This is an open-access article distributed under the terms of the Creative Commons Attribution License (CC BY). The use, distribution or reproduction in other forums is permitted, provided the original author(s) and the copyright owner(s) are credited and that the original publication in this journal is cited, in accordance with accepted academic practice. No use, distribution or reproduction is permitted which does not comply with these terms.
*Correspondence: Peter A. Ward, cHdhcmQmI3gwMDA0MDt1bWljaC5lZHU=