- 1Department of Pediatrics, AIIMS Bhubaneswar, Bhubaneswar, India
- 2Evidence Based Health Informatics Unit, Department of Telemedicine, PGIMER, Chandigarh, India
- 3Department of Medicine, VMMC, Safdarjung Hospital, New Delhi, India
- 4Department of Microbiology, MM Institute of Medical Sciences and Research, MMU (Deemed to be University), Ambala, India
- 5Department of Obstetrics and Gynecology, Capital Hospital, Bhubaneswar, India
- 6Department of Pediatrics, AIIMS New Delhi, New Delhi, India
Background: Anti-malarial drugs inhibit coronaviruses in-vitro. Few published studies have evaluated the safety and efficacy of these drugs in the treatment of COVID-19 infection.
Materials and Methods: This is a systematic review and meta-analysis of clinical trials and observational studies. Major database searches were carried out up until June 5, 2020. Participants admitted with RT-PCR-confirmed SARS Cov-2 (COVID-19) infection were included. The “Intervention group” received anti-malarial drugs with or without other drugs (Azithromycin) administered as an adjunct to the standard treatment/care. The “Control group” received treatment except anti-malarial drugs. The primary outcome is “all-cause mortality.” Secondary outcome measures were effects on clinical and laboratory parameters and adverse events.
Results: Of 3,472 citations, 17 (six clinical trials and 11 observational studies) studies provided data of 8,071 participants. Compared to the control, Hydroxy-chloroquine (HCQ) has no significant effect on mortality [(OR 0.87; 95% CI 0.46–1.64); eight observational studies; N = 5,944]. Data from a single, small non-randomized trial (N = 42) also reached a similar conclusion (OR 1.94; 95% CI 0.07–50.57; p = 0.69). Compared to the control, HCQ plus Azithromycin (AZM) significantly increased mortality [(OR 2.84; 95% CI 2.19–3.69); four observational studies; N = 2,310]. Compared to the control, risk of any adverse event was significantly increased in HCQ group [(OR 3.35; 95% CI 1.58–7.13); four clinical trials; N = 263]. Compared to control, risk of adverse cardiac events (abnormal ECG, arrhythmia, or QT prolongation) were not significantly increased in HCQ group (but significantly increased in the HCQ plus AZM group). The GRADE evidence generated for all the outcomes was of “very low-quality.”
Conclusions: As very low quality evidence suggests an increased risk of mortality and adverse event with HCQ plus Azithromycin combination (not HCQ alone), caution should be exercised while prescribing this combination for treatment of hospitalized adults with COVID-19 infection. Good quality, multi-centric RCTs (including both hospitalized and non-hospitalized patients) are required for any firm recommendation to be made during the ongoing pandemic.
OSF Protocol Registration Link: https://osf.io/6zxsu.
Introduction
COVID-19, also known as severe acute respiratory syndrome coronavirus 2 (SARS-CoV-2), emerged in Wuhan, China, in late 2019. It is a highly contagious disease with a global average mortality rate of 4.6% (1). There have been ongoing efforts to develop effective treatment modalities for this dreaded pandemic. Currently, no specific therapies against SARS-CoV-2 infection exist, and a series of therapeutic agents (e.g., antiviral agents, antibiotics, immune-modulators, inhaled nitric oxide, and convalescent plasma) have been repurposed with negative to inconclusive evidence available (2). There has been an increased interest in two existing anti-malarial drugs belonging to amino-quinoline group (Chloroquine and Hydroxy-chloroquine) to treat COVID-19. This is because of the inhibitory effects of these two drugs on other coronaviruses, such as SARS-CoV-1 (2, 3). The plausible mechanism of actions includes inhibition of angiotensin converting enzyme 2 (ACE-2) present on the cell surface for virus entry (by reduction of glycosylation in the enzyme) (4, 5), inhibition of release of viral particles into intra-cellular space (6, 7), and an anti-inflammatory effect (inhibition of interleukin-6, the tumor necrosis factor, the aberrant interferon, and other pro-inflammatory cytokines that cause lung injury leading to acute respiratory distress syndrome) (6, 8). Chloroquine and Hydroxy-chloroquine (HCQ) are both cost-effective and considered safe as per their approved indications. Compared to Chloroquine (CQ), Hydroxy-chloroquine (HCQ) is more soluble and less toxic and is considered safer (9, 10). It has to be kept in mind that these drugs are not entirely safe because of the risk of some serious side-effects (e.g., neuro-psychiatric, retinal, cardiac, and hypoglycemia), and there have been reports of toxicities in people who are self-medicating (11, 12).
There have been published studies evaluating the safety and/or efficacy of these agents (alone or in combination) compared to a control arm or parallel intervention, to treat patients with COVID-19 (13–32). However, the results have been contradictory. Earlier published rapid systematic reviews have concluded the role of anti-malarial drugs in patients with COVID-19 is still uncertain, and its routine use should not be recommended until more evidence is available from ongoing studies (33, 34). However, these systematic reviews neither included larger observational studies and randomized clinical trials (RCTs) published recently nor provided quality (GRADE) of evidence in a more systematic manner. In addition, findings from an ORCHID (Outcomes Related to COVID-19 treated with hydrox-ychloroquine among In-patients with symptomatic Disease) study have shown that HCQ neither harms nor benefits patients with COVID-19 infection (35). The present systematic review is an endeavor in this direction to synthesize the available evidences to inform clinical practice and guide the international agencies to formulate recommendations.
Materials and Methods
This systematic review protocol is registered at the Open Science Forum (OSF) registration link: https://osf.io/6zxsu.
Types of Studies
Both clinical trials (randomized, quasi- randomized, and non-randomized) and observational studies comparing anti-malarial drugs (Chloroquine and Hydroxy-chloroquine) alone or in combination with other drugs vs. a control (standard of care) or other treatment were included. As a majority of the studies were published on pre-print servers (for rapid dissemination of knowledge) prior to publication in peer-reviewed journals, we planned to include these studies in the present meta-analysis after taking permission from the study authors.
Types of Participants
Children of 12–18 years of age and adults with RT-PCR-confirmed SARS Cov-2 (COVID-19) cases treated in the hospital were included. Exclusion criteria were an allergy to anti-malarial drugs [Chloroquine (CQ) and Hydroxy-chloroquine (HCQ)], retinopathy, hearing loss, and severe neuro-psychiatric diseases.
Types of Interventions
(a) Interventions included anti-malarial drugs (CQ and HCQ) provided in various formulations and dose schedules. Based on a previous study, the following dose schedules were considered: HCQ—a loading dose of 400 mg twice daily (BID) followed by a maintenance dose of 200 mg BID for 4 days; and CQ-−500 mg BID for 5 days (9). The intervention was administered as an adjunct to other treatment modalities [including Azithormycin (AZM)] to patients infected with SARS Cov-2 (COVID-19). Those in the control group received supportive treatments without CQ/HCQ. We also included trials comparing different doses (high dose vs. low-dose of anti-malarial drugs) to provide more information and urgent dissemination of knowledge during the current pandemic.
(b) Supportive and additional treatment included various methods. In hospitalized cases, it varied from bed rest, nebulization, and oxygen inhalation to invasive respiratory support (mechanical ventilation) and maintenance of vital parameters. In addition, additional treatment during the current pandemic included antibiotics, non-specific anti-viral drugs [Remdesivir, Lopinavir/Ritonavir, IFN-α/β, Umifenovir [Arbidol], Entecavir, Ribavirin, and/or Oseltamivir], Immuno-modulators (Immunoglobulin, Tocilizumab, and Sarilumab), steroids, and NSAIDs (including Aspirin). There is evidence that non-specific antiviral drugs may not benefit patients with Covid-19, though Remdesivir and immune modulators may have some role in severe or critical cases (36, 37).
Types of Outcome Measures
Primary
1. All-cause mortality: patients with Covid-19 dying from any cause.
Secondary
1. Time to clinical recovery: time taken for normalization of temperature, respiratory distress, and relief of cough or no cough for 72 h
2. Proportion of patients with clinical recovery: proportions of patients with normalization of temperature, respiratory distress, and relief of cough or no cough for 72 h
3. Proportion of patients requiring escalation of respiratory support (including mechanical ventilation) or requiring ICU transfer: escalation of respiratory support defined as progressive change in the requirement of respiratory support to maintain normal oxygen saturation (SpO2) and vital parameters
4. Proportion of patients developing severe disease: proportions of patients developing severe disease as defined as per the National Institute of Health (NIH) COVID-19 Treatment Guidelines (37)
5. Duration of hospitalization: the time from admission (days) to either discharge or death
6. Duration of ICU stay: the time from admission (days) to ICU to death or transfer back to non-critical areas
7. Time to negative PCR results for COVID-19: the time taken for two consecutive negative reports of a positive patient
8. Proportion of patients with negative PCR results for COVID-19 after day 3, 5, 7, 10, 14, 21, and 28: proportions of patients with two consecutive negative reports after a positive report
9. Proportion of patients with improved radiological features after day 3, 5, 7, 10, 14, 21, and 28: proportions of patients with improvement noted in either chest X ray or CT scan of chest compared to that done at baseline
10. Effect on hematological parameters (including inflammatory markers): these include the blood parameters (complete blood count, differential counts, and platelet count), acute phase reactants (ESR, CRP, and pro-calcitonin), and inflammatory markers (IL-6, TNF-α, etc.)
11. Adverse events: developing secondary to the use of anti-malarial drugs alone or in combination with other drugs.
Search Methodology
The following major databases were searched systematically from 1970 till June 5, 2020: Cochrane Central Register of Controlled Trials (CENTRAL), PubMed/MEDLINE, Google Scholar, and EMBASE (Appendix 1). We also searched the Pre-print servers (medRxiv, bioRxiv, OSF pre-prints, Pre-prints.org) till June 5, 2020. The PubMed/Medline search strategy used the various MeSH and free text terms for “novel corona virus,” “COVID 19,” “Hydroxychloroquine,” and “Chloroquine” combined using the Boolean operators. No language restrictions were applied. Three reviewers (RRD, NJ, and ND) reviewed the search results to identify relevant studies.
Data Extraction
Data extraction was done using a data extraction form that was designed and pilot tested a priori. Three authors (NJ, ND, and SSN) independently extracted the following information from each study: author; year; location (country); study design (clinical trial or observational study); setting (hospital or community); method of recruitment; inclusion criteria; unit of analysis; allocation ratio In case of RCT); risk of bias; participants (age, sex, sample size, and disease severity); intervention (dosage, duration, frequency, and co-intervention if any); outcomes (outcome definition, valid unit of measurement, time points of collection and reporting); loss to follow-up; and miscellaneous (key conclusions, references to other relevant trials, and additional data required).
Assessment of Risk of Bias in the Included Studies
Two review authors independently (NJ and SSN) assessed the methodological quality of the selected trials by using methodological quality assessment forms and the criteria outlined in the Cochrane Handbook for Systematic Reviews of Interventions (38). Quality assessment was undertaken using the Newcastle Ottawa Scale (NOS) for observational studies. This scale assesses the quality under three major headings, namely, selection of the studies (representativeness and the exposure assessment/control selection), comparability (adjustment for main/additional confounders), and outcome/exposure (adequacy of outcome measured, exposure measured vs. self-report) (39). Quality assessment was undertaken using the ROBINS-I tool for non-randomized trials (40). Any disagreements between the two review authors were resolved through discussion with a third author (JS).
Dealing With Missing Data
We described missing data, including dropouts in included studies. Differential dropout rates can lead to biased estimates of the effect size, and bias may arise if the reasons for dropping out differ across groups. We reported reasons participants dropped out of studies as mentioned by the authors. If data were missing, or if reasons for dropping out were not reported, we contacted the authors for further information.
Data Synthesis
Data were analyzed using Review Manager (RevMan) V.5.1 (The Nordic Cochrane Center, The Cochrane Collaboration, Copenhagen, Denmark) (41). The data from various studies were pooled and expressed as mean difference (MD) with 95% confidence interval (CI) in case of continuous data, and odds ratio (OR) with 95% CI in case of categorical data. Where data were expressed as a median (IQR), we calculated the mean and SD by the statistical formula described previously (42). The primary pooled analysis of all the reports was conducted using the Generic Inverse Variance method using random effects weighting (43), where the log RRs for cohort studies or log ORs for case–control studies were weighted by the inverse of the variance to obtain a pooled RR estimate. Since nested case-cohort and nested case–control studies are temporally prospective, we analyzed data from these studies with the prospective studies. A p < 0.05 was considered statistically significant. Inter-study heterogeneity was assessed by Cochrane's Q (χ2 p < 0.10) and quantified by I2. An I2 ≥ 50% indicated “substantial” heterogeneity and ≥75% indicated “considerable” heterogeneity (44). The cause of substantial and considerable heterogeneity was explored, and sensitivity and/or sub-group analyses were carried out.
Publication Bias
To evaluate for any possible publication bias, we constructed the funnel plot from primary outcome data (45).
Grade of Evidence
To assess the quality of evidence we used GRADE Profiler software (V.3.2) (46, 47). The software uses five parameters for rating the quality of evidence. The parameters used were limitations to design of randomized controlled trials, inconsistency of results or unexplained heterogeneity, indirectness of evidence, imprecision of results, and publication bias. The rating was determined as no, serious, or very serious limitations.
Results
Description of Studies
Of 3,472 total citations retrieved, the full texts of 49 papers were assessed for eligibility, and 29 were excluded for various reasons (Figure 1). Of the remaining 20 eligible studies, 14 were published in peer-reviewed journals (13–29) and six in pre-print servers (not peer-reviewed) (17, 28–32). We contacted the authors of these six studies to give us their permission to use their data in the meta-analysis, but only three authors gave their permission (17, 28, 29). We therefore included the data of three studies in the meta-analysis and described the characteristics of the remaining three studies using a separate table (Supplementary Table 1). Finally, we were able to conduct a meta-analysis of a total of 17 studies (six clinical trials and 11 observational studies) including 8,071 patients (Adults = 8,041; Adolescents = 30) (Table 1). Twenty-nine studies were excluded for the following reasons: 19 were case series (without having a control/comparator that is inclusion criteria of present review), nine studies mentioned about intervention but did not provide outcome data for them separately, and one study reported use of anti-malarial drugs with or without AZM in rheumatoid arthritis (RA) patients for non-RA indications (including viral and other infections).
Of 17 published peer-reviewed studies included, six clinical trials provide data of 381 patients, and the 11 observational studies provided data of 8,071 patients. A total of 4,009 patients received HCQ or CQ (clinical trials = 226, observational studies = 3,783), and 1,255 received a combination of HCQ plus Azithromycin (clinical trials = 06, observational studies = 1,249). The studies were conducted in following countries: USA (five studies, 3,985 patients), Spain (two studies, 2,185 patients), China (four studies, 752 patients), France (two studies, 217 patients), Brazil (one study, 81 patients), and the UAE (one study, 34 patients). One trial compared high vs. low-dose of Chloroquine (18). One clinical trial (13) and six observational studies (19–22, 25, 27); each had three arms of comparison (HCQ, HCQ+AZM, and Control). Two studies included data on adolescents (<18 years) (13, 21). Two studies used Azithromycin but did not provide separate outcome data for both the groups (19, 20). Of the six clinical trials, three were described as double blinded, two were open label, and one was a non-randomized trial.
As shown in Table 1, the age of included participants, severity of illness, dose schedule, and timing of administration of intervention (HCQ/CQ) varied widely among the studies. Majority of the participants in the clinical trials were ≤ 50 yr of age, whereas, majority of the participants in the observational studies (except one) were ≥60 yr of age. Around 72% of participants in the clinical trials were having mild and moderate illness, whereas <40% of the participants in the observational studies were having mild and moderate illness. One study included only cancer patients (27). The dose of CQ was nearly uniform (except one RCT comparing high and low-dose) with duration varying from 5 to 10 days. The dose of HCQ varied widely with the lowest dose being 200 mg/d−1,200 mg on day 1 followed by variable doses for variable period (sometime till discharge/death). Two studies did not provide any information on dose schedule of HCQ (26, 27). The median time from onset of symptom to admission or treatment initiation was ≤ 8 days in all but two studies (one RCT has 17 days, and one observational study has 10 days). Two studies did not provide any information on the timing of initiation of HCQ (26, 27). Except one study (17), no other study was able to start the intervention (HCQ/CQ) in the early phase of illness (within 48 h of symptom onset), which is regarded as the golden window for antiviral treatment (e.g., in influenza) (48).
Risk of Bias in Included Studies
The details have been provided in Appendix 2. Except two trials (17, 18), others had low to high-risk of bias in different domains. One non-randomized trial had a serious risk of bias overall (13). Of the 11 observational studies, five were at a high risk of bias for selection of cases (22, 23, 25, 28, 29). Except for one study (27), the remaining 10 studies were at a high risk of bias for selection of controls and a low risk of bias for the exposure parameters.
Effect of Interventions
Primary Outcomes (All-Cause Mortality)
HCQ vs. control
(i) overall results: Three trials reported no mortality in any of the groups. One Non-RCT (N = 42) found no significant difference in the mortality rate between HCQ and control group (OR 1.94; 95% CI 0.07–50.57; p = 0.69) (13) (Supplementary Figure 1). Eight observational studies (N = 5,944) reported mortality rate, and found no significant difference between the HCQ and control group (OR 0.87; 95% CI 0.46–1.64; p = 0.66; I2 = 92%) (Figure 2) (19–21, 23, 25–27, 29).
(ii) Subgroup analysis (data from observational studies): Mortality rate was found to be significantly increased in the HCQ group in the studies from USA (OR 1.71; 95% CI 1.38–2.13; p < 0.001; I2 = 0%; N = 3,036) (20, 21, 25, 27), whereas a significantly decreased mortality rate was found in the studies conducted outside USA (N = 2,908) population (OR 0.38; 95% CI 0.23–0.63; p < 0.001; I 2 = 56%) (19, 23, 26, 29). The heterogeneity was not significant once we separated studies conducted in USA vs. outside USA. Two studies (20, 23) compared mortality rate in participants aged ≤ 60 vs. >60 yr and found significantly increased risk in those >60 yr age [data provided as hazard ratio [not raw data]]. One study used HCQ after 48 h of admission and two studies had no information on timing; when these two studies were omitted, no difference in mortality was found (OR 1.24; 95% CI 0.7–2.18; p = 0.46; I2 = 82%) (23, 26, 27). When studies with median time from onset of symptom to admission or treatment initiation of >8 days were excluded, and no significant difference was found (OR 1.24; 95% CI 0.7–2.18; p = 0.46; I2 = 82%). We could not carry out subgroup analyses of mortality rate in participants with and without co-morbidity, as these data were not provided separately by the included studies. When studies that did not follow the recommended dose schedule of HCQ/CQ were excluded, still no significant difference was found (OR 0.83; 95% CI 0.36–1.88; p = 0.65; I2 = 90%).
HCQ plus azithromycin (AZM)
Four studies (N = 2,310) reported a significant increase in the mortality rate in the HCQ plus AZM group compared to the control group (OR 2.84; 95% CI 2.19–3.69; p < 0.001) (19, 21, 25, 27) (Figure 3). Another study used Azithromycin in treatment but did not provide separate data (19).
HCQ vs. HCQ plus AZM
Five studies (N = 1,988) reported mortality rate, and found a significant decrease in HCQ group (OR 0.7; 95% CI 0.54–0.9; p = 0.006; I2 = 0%) (19, 21, 22, 25, 27).
High-dose vs. low-dose CQ
One RCT (N = 81) found a significantly higher mortality rate in the high-dose group (OR 3.63; 95% CI 1.24–10.58; p = 0.02) (Supplementary Figure 2) (18).
Secondary Outcomes
Details have been provided in Table 2. A majority of the outcome measures favored the Control group (i.e., the Control was better than HCQ±AZM), and these were the occurrence of adverse events [any events (Supplementary Figure 3), or only cardiac events, or only vomiting], development of severe disease, and duration of hospitalization. Those favored HCQ group (i.e., HCQ±AZM was better than Control) were resolution of cough, proportion of patients with negative COVID-19 PCR after days 5, 10, and 14, proportion of patients with improved radiological features after day 5, change in IL-6 level (pg/mL), and change in total leukocyte count (/cumm). The outcomes that favored HCQ over HCQ plus AZM were mortality rate and the development of severe disease. Contrary to common belief, no difference between HCQ and HCQ plus AZM was found for any type of adverse cardiac events.
Publication Bias
The funnel plot was asymmetrical showing publication bias (Supplementary Figure 4). The reasons for publication bias were heterogeneity among studies, poor methodological design, and selective outcome reporting.
Grade of Evidence
The evidence generated was of “very low quality” for all the outcomes (primary and secondary). A detailed analysis of the summary of evidence is provided in Table 3.
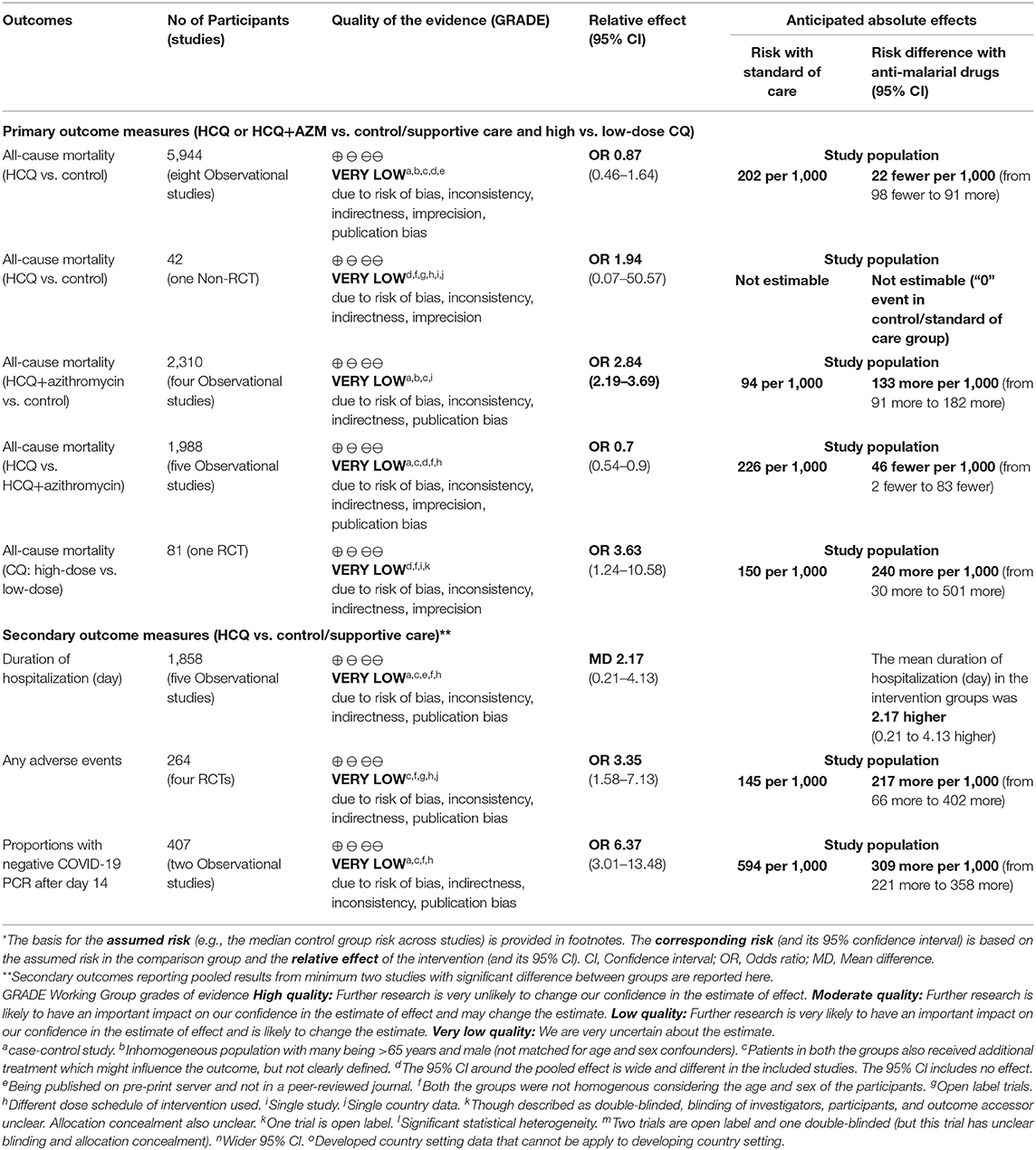
Table 3. GRADE evidence (anti-malarial drugs ± azithromycin vs. standard of care for patients with COVID-19 infection).
Discussion
Summary of Evidence
After an extensive search of the literature, we included 17 studies with data of 8,071 participants. Compared to control, HCQ alone (not HCQ+AZM combination) has no significant effect on mortality or risk of adverse cardiac events. The evidence for all the outcomes was of “very low quality.”
The high mortality and increased risk of adverse events with anti-malarial drugs noted by some studies may be overestimated because of the inclusion of an older population with underlying co-morbidities (including cardiac conditions) and simultaneous use of other cardiotoxic drugs (e.g., Azithromycin, and Oseltamivir). The same may be difficult to know during the current pandemic as there is no definitive treatment, and healthcare professionals all over the world want to administer these experimental drugs with the hope of saving some lives. The use of HCQ+AZM has drawn attention, and there are differences in opinion regarding use of this combination. Compared to control, HCQ+AZM combination was found to increase the mortality rate significantly, in contrast to HCQ alone. Compared to HCQ+AZM combination, HCQ alone was significantly decreasing the mortality rate. These indirect evidences suggest that HCQ+AZM might increase the mortality rate, and caution should be exercised while using this combination in vulnerable population (e.g., those with advanced age, underlying cardiac conditions, and those receiving medication with cardiac side-effects, as noted in the included studies).
It has to be kept in mind that, the anti-viral action of anti-malarial drugs against COVID-19 is still largely unknown (49, 50). An acute systemic inflammatory reaction/cytokine storm (besides the viral infection itself) is the hallmark of COVID-19 infection (51). This reaction, once well-established, can cause rapid disease progression leading to death (52, 53). However, except for three studies (15, 23, 28), no studies have reported the effect of anti-malarial drugs on the inflammatory markers and blood counts (lymphocyte, neutrophil). As supportive treatments were not uniform across the included studies, one may argue that simultaneous use of other drugs (anti-viral drugs, and/or interferon-α) as a part of supportive treatment might have confounded (increased or decreased) the efficacy of the anti-malarial drugs (15). This possibility, however, seems less likely, as few studies have found no difference after excluding patients receiving these drugs (15).
An interesting observation was that, studies from USA showed a significantly increased risk of mortality compared to those from outside USA. The same could be explained by the following points in the USA study cohort: inclusion of a higher proportion of patients with severe or critical illness, advanced age, and co-morbidities. Among the included studies in the present review, marked variation (high heterogeneity) was noted in the age group (in the clinical trials majority were ≤ 50 yr of age, whereas, in the observational studies majority were ≥60 yr of age), severity of COVID-19 illness (around 72% of participants in the clinical trials were having mild and moderate illness, whereas <40% of the participants in the observational studies exhibited mild and moderate illness), and inclusion of patients with co-morbidities (diabetes, cardio-vascular disease, chronic lung disease, etc.) among the study cohorts. We could not, however, carry out sub-group analyses as per severity illness because of paucity of data. Except for the severity of COVID-19 illness, the remaining two characteristics (age group and inclusion of patients with co-morbidities) of the study cohort could increase mortality that is independent of the effect of CQ/HCQ (±Azithromycin). This emphasizes the role of randomized double-blind trials in establishing the actual efficacy (if any) of anti-malarial drugs, as the chance of selection bias would be very low, and the groups would be comparable. The dose schedule of CQ was nearly uniform; however, the dose schedule of HCQ varied widely among the studies (except for one large study, the cumulative dose was equal or higher than the recommended schedule in the remaining studies). There was, however, no difference in the mortality rate. The median time from onset of symptom to admission or treatment initiation was nearly ≤ 8 days in all but two studies, and, apart from one study, others used CQ/HCQ within 48 h of admission/hospitalization (not symptom onset). There was no significant difference in the mortality rate between exposure/interventions and controls in these sub-groups. This might be due to the fact that starting anti-viral drugs (including HCQ/CQ) after 48 h of symptom onset might not be beneficial as the golden window for antiviral treatment (e.g., in influenza) is lost (48). This is difficult in a hospitalized setting (may be possible in outpatient or community setting); however, one RCT could able to use it within 48 h of symptom onset (found a significantly shorter time to clinical recovery and pneumonia resolution without any mortality) (17).
Limitations
The studies were variable in many aspects (blinding of participants and outcome assessors, patient selection, severity of illness, dose schedule of the anti-malarial drugs, timing of administration, measurement of inflammatory markers and effect of the drugs on these markers, outcome definition, and measurements). We could not determine the effect of anti-malarial drugs in Covid-19 infection in pediatric and adolescent population. As there were few studies, results from all the secondary outcomes could not be pooled.
Future Areas of Research
Future clinical trials should include good quality RCTs with adequate sample size, should ideally be multi-centric, and should focus on the variability noted in the present review. Pediatric and adolescent population also need to be included in the ongoing studies to guide recommendation in this group of patients. Both CQ/HCQ should also be evaluated in non-hospitalized patients with COVID-19 infection.
Conclusions
As very low quality evidence suggests an increased risk of mortality and adverse event with HCQ plus Azithromycin combination (not HCQ alone); caution should be exercised while prescribing this combination for treatment of hospitalized adults with COVID-19 infection. Multi-centric RCTs (including both hospitalized and non-hospitalized patients) of a good quality are required for any firm recommendation to be made during the ongoing pandemic.
Data Availability Statement
All datasets generated for this study are included in the article/Supplementary Material.
Author Contributions
RD developed the concept, abstracted data from papers, managed data for the review, performed the statistical analysis, interpreted data, made statistical inferences, wrote the review, and serves as guarantor for the review. NisJ, ND, NikJ, and SN abstracted data from papers, managed data for the review, and wrote the review. JS developed the concept, performed the statistical analysis and made inferences, and wrote the review. RD and JS jointly act as guarantors. All the authors have approved the version to be published.
Conflict of Interest
The authors declare that the research was conducted in the absence of any commercial or financial relationships that could be construed as a potential conflict of interest.
Acknowledgments
The authors acknowledge ICMR center for advanced research in evidence based child health, PGIMER, and ICMR Headquarters.
Supplementary Material
The Supplementary Material for this article can be found online at: https://www.frontiersin.org/articles/10.3389/fmed.2020.00482/full#supplementary-material
Supplementary Figure 1. All-cause mortality (HCQ vs. control; Non-RCT).
Supplementary Figure 2. All-cause mortality (High-dose vs. low-dose CQ; RCT).
Supplementary Figure 3. Any adverse events (HCQ vs. control; RCTs).
Supplementary Figure 4. Funnel plot (primary outcome data from observational studies).
References
1. WHO Coronavirus Disease (COVID-19) Dashboard. Available online at: covid19.who.int (accessed July 9, 2020).
2. Zhang J, Xie B, Hashimoto K. Current status of potential therapeutic candidates for the COVID-19 crisis. Brain Behav Immun. (2020) 87:59–73. doi: 10.1016/j.bbi.2020.04.046
3. Vincent MJ, Bergeron E, Benjannet S, Erickson BR, Rollin PE, Ksiazek TG, et al. Chloroquine is a potent inhibitor of SARS coronavirus infection and spread. Virol J. (2005) 2:69. doi: 10.1186/1743-422X-2-69
4. Wan Y, Shang J, Graham R, Baric RS, Li F. Receptor recognition by the novel coronavirus from Wuhan: an analysis based on decade-long structural studies of SARS coronavirus. J Virol. (2020) 94:e00127–20. doi: 10.1128/JVI.00127-20
5. Zhou P, Yang X-L, Wang X-G, Hu B, Zhang L, Zhang W, et al. A pneumonia outbreak associated with a new coronavirus of probable bat origin. Nature. (2020) 579:270–3. doi: 10.1038/s41586-020-2012-7
6. Devaux CA, Rolain J-M, Colson P, Raoult D. New insights on the antiviral effects of chloroquine against coronavirus: what to expect for COVID-19? Int J Antimicrob Agents. (2020) 55:105938. doi: 10.1016/j.ijantimicag.2020.105938
7. Golden EB, Cho HY, Hofman FM, Louie SG, Schönthal AH, Chen TC. Quinoline-based antimalarial drugs: a novel class of autophagy inhibitors. Neurosurg Focus. (2015) 38:E12. doi: 10.3171/2014.12.FOCUS14748
8. Gbinigie K, Frie K. Should chloroquine and hydroxychloroquine be used to treat COVID-19? A rapid review. BJGP Open. (2020) 4:20X101069. doi: 10.3399/bjgpopen20X101069
9. Yao X, Ye F, Zhang M, Cui C, Huang B, Niu P, et al. In vitro antiviral activity and projection of optimized dosing design of hydroxychloroquine for the treatment of severe acute respiratory syndrome coronavirus 2 (SARS-CoV-2). Clin Infect Dis. (2020). doi: 10.1093/cid/ciaa237. [Epub ahead of print].
10. Sahraei Z, Shabani M, Shokouhi S, Saffaei A. Aminoquinolines against coronavirus disease 2019 (COVID-19): chloroquine or hydroxychloroquine. Int J Antimicrob Agents. (2020) 55:105945. doi: 10.1016/j.ijantimicag.2020.105945
11. Juurlink DN. Safety considerations with chloroquine, hydroxychloroquine and azithromycin in the management of SARS-CoV-2 infection. CMAJ. (2020) 192:E450–3. doi: 10.1503/cmaj.200528
12. Beaumont P, Ratcliffe R. Chloroquine: Trump's misleading claims spark hoarding and overdoses. The Guardian (2020 March 25). Available online at: https://www.theguardian.com/science/2020/mar/25/can-chloroquine-reallyhelp-treat-coronavirus-patients (accessed June 7, 2020).
13. Gautret P, Lagier JC, Parola P, Hoang VT, Meddeb L, Mailhe M, et al. Hydroxychloroquine and azithromycin as a treatment of COVID-19: results of an open-label non-randomized clinical trial. Int J Antimicrob Agents. (2020). doi: 10.1016/j.ijantimicag.2020.105949. [Epub ahead of print].
14. Chen J, Liu D, Liu L, Liu P, Xu Q, Xia L, et al. A pilot study of hydroxychloroquine in treatment of patients with moderate COVID-19. Zhejiang Da Xue Xue Bao Yi Xue Ban. (2020) 49:215–9. doi: 10.3785/j.issn.1008-9292.2020.03.03
15. Tang W, Cao Z, Han M, Wang Z, Chen J, Sun W, et al. Hydroxychloroquine in patients with mainly mild to moderate coronavirus disease 2019: open label, randomised controlled trial. BMJ. (2020) 369:m1849. doi: 10.1136/bmj.m1849
16. Huang M, Tang T, Pang P, Li M, Ma R, Lu J, et al. Treating COVID-19 with Chloroquine. J Mol Cell Biol. (2020) 12:322–5. doi: 10.1093/jmcb/mjaa014
17. Chen Z, Hu J, Zhang Z, Jiang S, Han S, Yan D, et al. Efficacy of hydroxychloroquine in patients with COVID-19: results of a randomized clinical trial. medRxiv. (2020). doi: 10.1101/2020.03.22.20040758. [Epub ahead of print].
18. Borba M, de Almeida Val F, Sampaio VS, Alexandre MAA, Melo GC, Brito M, et al. Effect of high vs low doses of chloroquine diphosphate as adjunctive therapy for patients hospitalized with severe acute respiratory syndrome coronavirus 2 (SARS-CoV-2) infection: a randomized clinical trial. JAMA Netw Open. (2020) 3:e208857. doi: 10.1001/jamanetworkopen.2020.8857
19. Mahévas M, Tran VT, Roumier M, Chabrol A, Paule R, Guillaud C, et al. Clinical efficacy of hydroxychloroquine in patients with covid-19 pneumonia who require oxygen: observational comparative study using routine care data. BMJ. (2020) 369:m1844. doi: 10.1136/bmj.m1844
20. Geleris J, Sun Y, Platt J, Zucker J, Baldwin M, Hripcsak G, et al. Observational study of hydroxychloroquine in hospitalized patients with Covid-19. N Engl J Med. (2020) 382:2411–8. doi: 10.1056/NEJMoa2012410
21. Rosenberg ES, Dufort EM, Udo T, Wilberschied LA, Kumar J, Tesoriero J, et al. Association of treatment with hydroxychloroquine or azithromycin with in-hospital mortality in patients with COVID-19 in New York State. JAMA. (2020) 323:e208630. doi: 10.1001/jama.2020.8630
22. Saleh M, Gabriels J, Chang D, Kim BS, Mansoor A, Mahmood E, et al. The effect of chloroquine, hydroxychloroquine and azithromycin on the corrected QT interval in patients with SARS-CoV-2 infection. Circ Arrhythm Electrophysiol. (2020) 13:CIRCEP.120.008662. doi: 10.1161/CIRCEP.120.008662
23. Yu B, Li C, Chen P, Zhou N, Wang L, Li J, et al. Low dose of hydroxychloroquine reduces fatality of critically ill patients with COVID-19. Sci China Life Sci. (2020). doi: 10.1007/s11427-020-1751-3. [Epub ahead of print].
24. Huang M, Li M, Xiao F, Pang P, Liang J, Tang T, et al. Preliminary evidence from a multicenter prospective observational study of the safety and efficacy of chloroquine for the treatment of COVID-19. Natl Sci Rev. (2020). doi: 10.1101/2020.04.26.20081059. [Epub ahead of print].
25. Magagnoli J, Narendran S, Pereira F, Cummings TH, Hardin JW, Sutton SS, et al. Outcomes of hydroxychloroquine usage in United States veterans hospitalized with COVID-19. Med. (2020). doi: 10.1016/j.medj.2020.06.001. [Epub ahead of print].
26. Ayerbe L, Risco C, Ayis S. The association between treatment with heparin and survival in patients with Covid-19. J Thromb Thrombolysis. (2020) 50:1–4. doi: 10.1101/2020.05.27.20114694
27. Kuderer NM, Choueiri TK, Shah DP, Shyr Y, Rubinstein SM, Rivera DR, et al. Clinical impact of COVID-19 on patients with cancer (CCC19): a cohort study. Lancet. (2020) 395:1907–18. doi: 10.1016/S0140-6736(20)31187-9
28. Mallat J, Hamed F, Balkis M, Mohamed MA, Mooty M, Malik A, et al. Hydroxychloroquine is associated with slower viral clearance in clinical COVID-19 patients with mild to moderate disease: a retrospective study. medRxiv [Preprint]. (2020). doi: 10.1101/2020.04.27.20082180
29. Membrillo FJ, Ramírez-Olivencia G, Estébanez M, de Dios B, Herrero MD, Mata T, et al. Early hydroxychloroquine is associated with an increase of survival in COVID-19 patients: an observational study. Preprints. (2020). doi: 10.20944/preprints202005.0057.v2. [Epub ahead of print].
30. Chen X, Zhang Y, Zhu B, Zeng J, Hong W, He X, et al. Associations of clinical characteristics and antiviral drugs with viral RNA clearance in patients with COVID-19 in Guangzhou, China: a retrospective cohort study. medRxiv [Preprint]. (2020). doi: 10.1101/2020.04.09.20058941
31. Feng Z, Li J, Yao S, Yu Q, Zhou W, Mao X, et al. The use of adjuvant therapy in preventing progression to severe pneumonia in patients with coronavirus disease 2019: a multicenter data analysis. medRxiv [Preprint]. (2020). doi: 10.1101/2020.04.08.20057539
32. Shabrawishi MH, Naser AY, Alwafi H, Aldobyany AM, Touman AA. Negative nasopharyngeal SARS-CoV-2 PCR conversion in response to different therapeutic interventions. medRxiv [Preprint] (2020). doi: 10.1101/2020.05.08.20095679
33. Chowdhury MS, Rathod J, Gernsheimer J. A rapid systematic review of clinical trials utilizing chloroquine and hydroxychloroquine as a treatment for COVID-19. Acad Emerg Med. (2020) 27:493–504. doi: 10.1111/acem.14005
34. Sarma P, Kaur H, Kumar H, Mahendru D, Avti P, Bhattacharyya A, et al. Virological and clinical cure in COVID-19 patients treated with hydroxychloroquine: a systematic review and meta-analysis. J Med Virol. (2020) 92:776–85. doi: 10.1002/jmv.25898
35. NIH Halts Clinical Trial of Hydroxychloroquine. Available online at: https://www.nih.gov/news-events/news-releases/nih-halts-clinical-trial-hydroxychloroquine (accessed July 5, 2020).
36. Peng F, Tu L, Yang Y, Hu P, Wang R, Hu Q, et al. Management and treatment of COVID-19: the Chinese experience. Can J Cardiol. (2020) 36:915–30. doi: 10.1016/j.cjca.2020.04.010
37. Management of Persons With COVID-19: National Institute of Health (NIH) Covid-19 Treatment Guidelines. Available online at: https://www.covid19treatmentguidelines.nih.gov/overview/management-of-covid-19/ (accessed June 12, 2020).
38. Higgins JPT, Altman DG, Gøtzsche PC, Juni P, Moher D, Oxman AD, et al. The Cochrane Collaboration's tool for assessing risk of bias in randomised trials. BMJ. (2011) 343:d5928. doi: 10.1136/bmj.d5928
39. Wells G, Shea B, O'Connell J, Peterson J. The Newcastle Ottawa Scale (NOS) for Assessing the Quality of Nonrandomised Studies in Meta-Analysis. Available online at: http://www.ohri.ca/programs/clinical_epidemiology/oxford.asp (accessed June 12, 2020).
40. Sterne JA, Hernán MA, Reeves BC, Savovic J, Berkman ND, Viswanathan M, et al. ROBINS-I: a tool for assessing risk of bias in non-randomised studies of interventions. BMJ. (2016) 355:i4919. doi: 10.1136/bmj.i4919
41. Review Manager (RevMan) [Computer program]. Version 5.3. Copenhagen; London, UK: The Nordic Cochrane Center; The Cochrane Collaboration (2014).
42. Luo D, Wan X, Liu J, Tong T. Optimally estimating the sample mean from the sample size, median, mid-range, and/or mid-quartile range. Stat Methods Med Res. (2018) 27:1785–805. doi: 10.1177/0962280216669183
43. Schmidt FL, Oh IS, Hayes TL. Fixed- versus random-effects models in meta-analysis: model properties and an empirical comparison of differences in results. Br J Math Stat Psychol. (2009) 62:97–128. doi: 10.1348/000711007X255327
44. Higgins JPT, Green S. Cochrane Handbook for Systematic Reviews of Interventions Version 5.0.0. The Cochrane Collaboration. (2008). Available online at: www.cochrane-handbook.org (accessed June 12, 2020).
45. Sterne JA, Egger M, Smith GD. Systematic reviews in health care: investigating and dealing with publication and other biases in meta-analysis. BMJ. (2001) 323:101–5. doi: 10.1136/bmj.323.7304.101
46. GRADEpro GDT: GRADEpro Guideline Development Tool [Software]. McMaster University (developed by Evidence Prime, Inc.) (2015). Available online at: gradepro.org (accessed June 12, 2020).
47. Schünemann H, Brozek J, Guyatt G, Oxman A editors. GRADE handbook for grading quality of evidence and strength of recommendations. The GRADE Working Group (2013). Available online at: guidelinedevelopment.org/handbook (accessed June 12, 2020).
48. Harper SA, Bradley JS, Englund JA, File TM, Gravenstein S, Hayden FG, et al. Expert Panel of the Infectious Diseases Society of America. Seasonal influenza in adults and children–diagnosis, treatment, chemoprophylaxis, and institutional outbreak management: clinical practice guidelines of the Infectious Diseases Society of America. Clin Infect Dis. (2009) 48:1003–32. doi: 10.1086/598513
49. Touret F, de Lamballerie X. Of chloroquine and COVID-19. Antiviral Res. (2020) 177:104762. doi: 10.1016/j.antiviral.2020.104762
50. Barnard DL, Day CW, Bailey K, Heiner M, Montgomery R, Lauridsen L, et al. Evaluation of immunomodulators, interferons and known in vitro SARS-coV inhibitors for inhibition of SARS-coV replication in BALB/c mice. Antivir Chem Chemother. (2006) 17:275–84. doi: 10.1177/095632020601700505
51. Guan WJ, Ni ZY, Hu Y, Liang W-H, Ou C-Q, He J-X, et al. Clinical characteristics of coronavirus disease 2019 in China. N Engl J Med. (2020) 382:1708–20. doi: 10.1056/NEJMoa2002032
52. Chen T, Wu D, Chen H, Yan W, Yang D, Chen G, et al. Clinical characteristics of 113 deceased patients with coronavirus disease 2019: retrospective study. BMJ. (2020) 368:m1091. doi: 10.1136/bmj.m1091
Keywords: aminoquinoline, azithromycin, SARS-CoV-2, evidence-based medicine, COVID-19, mortality, Chloroquine, Hydroxychloroquine
Citation: Das RR, Jaiswal N, Dev N, Jaiswal N, Naik SS and Sankar J (2020) Efficacy and Safety of Anti-malarial Drugs (Chloroquine and Hydroxy-Chloroquine) in Treatment of COVID-19 Infection: A Systematic Review and Meta-Analysis. Front. Med. 7:482. doi: 10.3389/fmed.2020.00482
Received: 30 April 2020; Accepted: 16 July 2020;
Published: 29 July 2020.
Edited by:
Zisis Kozlakidis, International Agency for Research on Cancer (IARC), FranceReviewed by:
Ajay Kumar Sharma, Institute of Nuclear Medicine & Allied Sciences (DRDO), IndiaRiccardo Pinciroli, Massachusetts General Hospital and Harvard Medical School, United States
Copyright © 2020 Das, Jaiswal, Dev, Jaiswal, Naik and Sankar. This is an open-access article distributed under the terms of the Creative Commons Attribution License (CC BY). The use, distribution or reproduction in other forums is permitted, provided the original author(s) and the copyright owner(s) are credited and that the original publication in this journal is cited, in accordance with accepted academic practice. No use, distribution or reproduction is permitted which does not comply with these terms.
*Correspondence: Rashmi Ranjan Das, cnJkYXMwNSYjeDAwMDQwO2dtYWlsLmNvbQ==; Jhuma Sankar, amh1bWFqaSYjeDAwMDQwO2dtYWlsLmNvbQ==