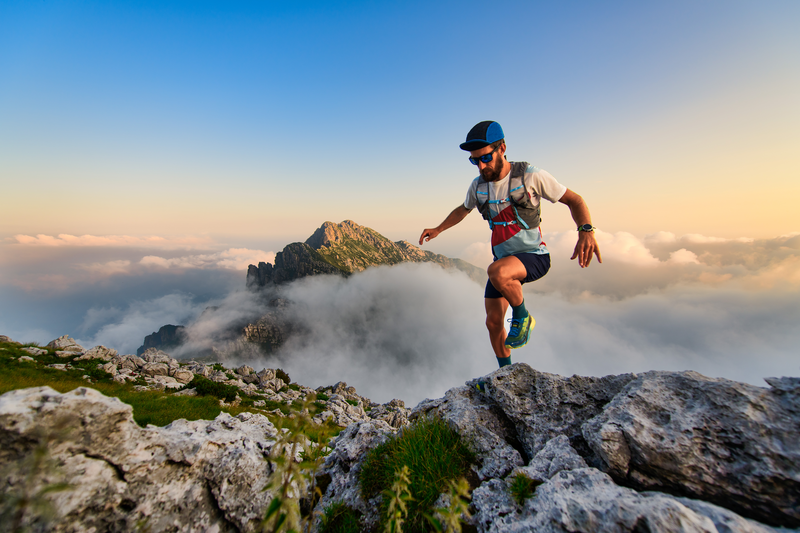
94% of researchers rate our articles as excellent or good
Learn more about the work of our research integrity team to safeguard the quality of each article we publish.
Find out more
MINI REVIEW article
Front. Med. , 15 May 2020
Sec. Infectious Diseases – Surveillance, Prevention and Treatment
Volume 7 - 2020 | https://doi.org/10.3389/fmed.2020.00240
This article is part of the Research Topic Coronavirus Disease (COVID-19): Pathophysiology, Epidemiology, Clinical Management and Public Health Response View all 400 articles
The coronavirus infection is constantly diffusing worldwide and the incidence of death is dramatically increasing, representing one of the greatest disasters in human history. Nowadays, no effective therapeutic approaches have been licensed, despite the rising interest of the scientific research in this specific field, and the daily growing number of publications, while the need to find novel strategies is urgent. Evidence in the literature reported the antiviral activity of polyphenols, the largest class of bioactive compounds in nature. Interestingly, a limited number of studies investigated the efficacy of polyphenols from different raw materials, directly against coronaviruses. The present manuscript aimed to report this evidence and provide a viewpoint on the possibility to use it as a start point for the development of novel natural approaches against this viral infection, eventually designing further appropriate researches.
The 2019 coronavirus (SARS-CoV2) pandemic, reported for the first time in Wuhan (China), in December 2019 (1), is rapidly growing with marked morbidity and mortality, resulting in a dramatic socio-economic impact. The diagnosis of SARS-CoV2 infection is based on qualitative Real-time reverse transcriptase-polymerase chain reaction (rRT-PCR) analysis on a nasopharyngeal swab. However, the presence of this virus has been also demonstrated in further tissues, including sputum, feces, bronchoalveolar fluids, and blood, with different viral kinetics (1–3).
Nowadays, no pharmacological treatments have been licensed as effective in terms of both viral titer reduction and/or lowering the virus spread. In several countries, subjects tested positive are receiving off-label and compassionate therapies, including chloroquine, hydroxychloroquine, azithromycin, lopinavir-ritonavir, favipiravir, remdesivir, ribavirin, interferon, convalescent plasma, steroids, and anti–IL-6 inhibitors (4–8). However, a number of adverse effects, including QT prolongation, torsade de pointes, hepatitis, acute pancreatitis, neutropenia, and anaphylaxis have been reported, particularly in patients treated with chloroquine/hydroxychloroquine, azithromycin, and lopinavir -ritonavir. The need to find a strategy that is both effective and safe to face this emergency is urgent.
Polyphenols are the largest class of bioactive compounds present in plants, where are produced as secondary metabolites with protective functions against ultraviolet radiations, pathogen aggression, and oxidative stress protection. Structurally, the term polyphenol refers to the presence of one or more phenolic rings with hydroxyl groups. On that bases, polyphenols can be classified into flavonoid (including anthocyanins, flavones, flavanones, flavonols, isoflavones, and flavan-3-ols), phenolic acids, polyphenolic amides, and other polyphenol compounds (including stilbenes or lignans) (9).
Besides the well-known antioxidant and anti-inflammatory activities of polyphenols, evidence highlighted the antiviral potential exerted by this class of bioactive compounds. In particular, a large number of studies demonstrated the efficacy of polyphenols against several pathogens, including Epstein-Barr virus (10, 11), enterovirus 71 (12), herpes simplex virus (HSV) (13, 14), influenza virus (15), and other virus causing respiratory tract-related infections (16–18). In this context, a great interest has been focused on resveratrol (RSV), whose antiviral mechanisms of actions are mainly attributable to its ability to inhibit the viral replication via (i) inhibition of immediate-early virus protein expression (i.e., ICP-4 and−27), (ii) inhibition of the NFκB signaling pathway, and (iii) activation of the AMPK/Sirt1 axis in the host cell (14).
The present mini-review aimed to report the few promising evidence regarding the potential anti-coronavirus activity of polyphenols, which may serve to drive the research toward the development of novel strategies to counteract the SARS-CoV2 pandemic.
Besides the general mechanisms of action described against various viruses, a limited number of studies investigated the effects of polyphenols directly against coronaviruses. These are in vitro studies conducted on different experimental models of infection, using microorganisms belonging to the coronavirus family (Table 1).
In 2017, Lin et al., performed an interesting study aimed to evaluate the anti-coronavirus activity of RSV (3,5,4′-trihydroxystilbene), a 14-carbon skeleton stilbene widely presents in plants, including Vitis vinifera and Polygonum cuspidatum. RSV exhibits three hydroxyl groups in position 3, 5, and 4′ joined to the two aromatic rings by a double styrene bond that determines the existence of cis- and trans-RSV isomers (Figure 1) (14). The antiviral activity of RSV was evaluated on Vero E6 cells infected with Middle East Respiratory Syndrome coronavirus (MERS-CoV) and treated with RSV at concentrations ranging from 250 to 7.8125 μM. It was demonstrated that RSV (i) reduced the cell death caused by MERS-CoV at concentrations ranging from 250 to 125 μM, (ii) inhibited the viral RNA replication at concentrations ranging from 250 to 31.25 μM, (iii) reduced the viral titer at concentrations ranging from 250 to 125 μM, (iv) inhibited dose-dependently the expression of nucleocapsid proteins at concentrations ranging from 250 to 125 μM, and (v) inhibited the apoptosis, as evidenced by a dose-dependent reduction of the Caspase-3 expression. Thus, this study evidenced the ability of RSV to counteract MERS-CoV infection acting on the main putative mechanisms of action. In particular, according to authors, it was speculated that RSV might be able to (i) activate the ERK1/2 and SIR1 signaling pathways, related to both cell survival and DNA protection, (ii) inhibit the MERS-CoV-induced apoptosis via down-regulation of the FGF-2 signaling pathway, and (iii) reduce the infection interfering with the NFκB-regulated signaling pathway (21). In addition to MERS-CoV, further studies investigated the antiviral potential of polyphenols against infectious bronchitis virus (IBV), another microorganism belonging to the coronavirus family. In particular, the anti-IBV activity of Forsythoside A (FTA) (Figure 2), a phenylethanoid glycoside with chemical formula C29H36O15 isolated from Forsythia suspense, was evaluated on chicken embryo kidney (CEK) cells. Cells (both prior to and after virus infection) were treated with FTA 0.16, 0.32, and 0.64mM. It was observed that FTA (i) induced a dose-dependent decrease in viral load, (ii) reduced the gene expression of IBV nucleocapsid proteins, and (iii) dose-dependently inhibited the IBV infection, but had no effect on infected cells (19), suggesting the potential of this bioactive compound as an antiviral agent against IBV. Similarly, the same virus was used to infect Vero cells and the anti-IBV activity of the polyphenols of Sambucus nigra was tested. In particular, a crude polyphenolic extract (0.004 g/ml) was used to treat cells 24 h prior to being infected. The pre-treatment with the S. nigra polyphenolic extract resulted in the (i) inhibition of the viral replication, (ii) dose-dependent reduction of the virus titers by four to six orders of magnitude at 1.0 and 0.1 multiplicity of infection (MOI), respectively, (iii) inhibition of the infection process at an early stage, and (iv) alteration of both virus structures and membrane vesicles (23). Although the results regarding the anti-IBV potential of S. nigra polyphenols are promising, the authors did not characterize the crude extract, thus, the main actors responsible for the antiviral activity cannot be identified. However, previous studies described the phytochemicals contained in S. nigra extract, reporting the presence of cyanidin, kaempferol, myricetin, dihydromyricetin, and quercetin derivatives 3-, 4-, and 5-caffeoylquinic acid; kaempferol 3-rutin; rutin; pelargonidin 3-glucoside; isorhamnetin 3-rutin, isorhamnetin 3-glucoside (24–26) and flavonols (5,7,3′,4′-tetra-O-methylquercetin and 5,7-dihydroxy-4-oxo-2-(3,4,5- trihydroxyphenyl)chroman-3-yl-3,4,5-trihydroxycyclohexanecarboxylate) (27). Interestingly, it was also reported that some of these S. nigra-derived polyphenols exhibited antivirus activities (27, 28). In this sense, it can be speculated that the antiviral activity is exerted by the phytocomplex including a large number of polyphenolic compounds that, in turn, are eventually responsible for a synergistic effect.
Besides the investigations on the aforementioned cell lines, two mechanistic studies have been performed to elucidate the specific targets of polyphenols in their anti-coronavirus activity. Particularly, it was tested the anti-MERS- and SARS-CoV activity of ten different polyphenols isolated from Brussonetia papyrifera, whose chemical structures are reported in Figure 3. In particular, all the isolated compounds were individually tested at concentrations ranging from 0 to 200 μM, demonstrating their dose-dependent inhibitory activities against MERS/SARS-CoV proteases and finding the half-maximum inhibitory concentration (IC50) at concentrations ranging from 30.2 to 233.3 μM (22). On the other hand, a large number of polyphenolic compounds were tested in a quantum dots-conjugated oligonucleotide system for the inhibitor screening of SARS-CoV nucleocapsid proteins. More specifically, the following compounds were studied: quercetin, acacetin, apigenin, baicalein, hesperidin, morin, rutin, naringin, naringenin, (–)-catechin, (–)-catechin gallate, (–)-gallocatechin gallate, diosmin, daidzein, genistein, glycitein, kaempferol, luteolin, myricetin, silibinin, silymarin, orientin, oroxylin A. Among these, (–)-catechin gallate and (–)-gallocatechin gallate (Figure 4) exhibited a marked anti-SARS-CoV nucleocapsid protein activity. In particular, (i) a dose-dependent ability to attenuate the binding activity was observed at concentrations ≥0.005 μg/ml, (ii) at 0.05 μg/ml both the compounds exerted more than 40% inhibition activity, and (iii) at the same concentration was found the IC50 (20). These results appear interesting since they clarify specific mechanisms of action and/or putative targets for the antiviral activity of polyphenols. Moreover, they allow to limit the large variety of polyphenolic compounds, leading to identification of such polyphenols for the development of novel natural approaches against coronavirus infection.
Figure 3. Polyphenols isolated from Brussonetia papyrifera. (A) Broussochalcone B (C20H20O4); (B) broussochalcone A (C20H20O5); (C) kazinol A (C25H30O4); (D) 3'-(3-methylbut-2-enyl)-3',4,7-trihydroxyflavone (C20H22O4); (E) papyriflavonol A (C25H26O7); (F) 4-hydroxyisolonchocarpin (C20H20O4); (G) kazinol B (C25H28O4); (H) broussoflavan A (C25H30O6); (I) kazinol F (C25H32O4); (J) kazinol J (C26H34O4).
Although these studies are appealing and well-conducted, and provide promising results, their careful analysis leads to individuate the following limitations. Firstly, evidence from the anti-coronavirus activity of polyphenols is only provided by in vitro studies, although animal-based studies reported the efficacy of polyphenols on other kinds of viruses. No clinical evidence, nor, at least, animal-based studies, are available. However, this lack might be due to the difficulty of designing appropriate studies on animals infected with this kind of virus, due to its dangerousness. Similarly, the need to eventually test natural compounds in humans have not emerged until now, since no many cases were registered. Another limitation is due to the fact that polyphenols are a very large class of bioactive compounds, in which further subclasses can be identified. Although promising, these studies do not provide evidence to establish which subclass of polyphenols deserves to be further investigated. Moreover, taking into account the in vitro nature of these studies, no information is provided by authors concerning a possible dose in humans, necessary to design clinical trials.
Overall, this evidence suggests that polyphenols may exert a marked and well-demonstrated activity against coronaviruses, at least in vitro, in addition to the previously demonstrated antiviral activity in vivo. Studies available in the literature agree in establishing that the reduction of virus titer and the inhibition of nucleocapsid protein expression are their main general mechanisms of action at the base of this promising effect of polyphenols. These elucidated mechanisms are of great interest, since nowadays no effective treatments have been licensed, and the development of novel synthetic drugs against specific coronavirus molecular targets are still far from being achieved.
Despite the aforementioned limitations, these studies should be taken into consideration to design clinical trials. In this sense, the main strength concerning the use of polyphenols in this global emergency is related to the well-established absence of both side effects and drug interactions of such polyphenols with concomitant pharmacological treatments. Indeed, it is well-known that coronavirus-infected subjects are highly prone to develop such respiratory diseases, sometimes complicated by the co-existence of previous cardio-metabolic or chronic diseases. This articulated pathological scenario drastically limits the use of such therapeutic schemes. As an example, the French non-randomized clinical trial showed encouraging results on the efficacy of the combination hydroxychloroquine and azithromycin against COVID19 (6), although both drugs are potentially associated with QT-prolongation (29, 30).
Another relevant point that should be taken into account, is the proper formulation of polyphenol-based nutraceuticals that may be efficient for this scope. Undoubtedly, according to the available studies, potential anti-COVID-19 nutraceutical approaches should contain polyphenols whose effects against coronaviruses have been demonstrated. However, the evaluation of potential synergistic effects between different polyphenols is intriguing. Despite the different structure, indeed, polyphenols share the same chemical features, including the presence of phenolic rings with hydroxyl groups (9). Thus, it could be hypothesized that, although not directly investigated, different classes of polyphenols might exert, at least in part, similar antiviral activities, but eventually with different mechanisms of action. In this sense, according to the studies of Rho (20) and Lin et al. (21), a possible association between RSV and catechins might be speculated for a potential synergy, resulting in hampering the antiviral effect. With this rationale, it should be stressed the importance to investigate the effect of natural polyphenolic extract, rather than the single purified molecules. Interestingly, various plant- or food-derived extracts have been found to be polyphenol-rich matrices for formulation of nutraceutical supplements. Among these, grape pomace extract (GPE) has been reported as an excellent source of bioactive compounds, mainly polyphenols, including RSV, cathechins, and proanthocyanidins (31–33). Notably, evidence indicated the antiviral activity of GPE against various microorganisms, including human immunodeficiency virus type 1 (34), human enteric virus, human norovirus surrogates [feline calicivirus (FCV) F9 and murine norovirus (MNV-19)] (35), hepatitis A virus (36), and hepatitis C virus (HCV) (37). Different mechanisms of actions have been demonstrated, including down-regulation of the HIV-1 entry co-receptor expression (for the activity against HIV) (34), suppression of virus replication via reduction of COX2 expression and regulation of NFκB and MAPK signaling pathways and reduction of virus-induced inflammation (for the anti-HCV activity) (37). Interestingly, two in vitro studies investigated the effects of GPE against respiratory syncytial virus, using an airway epithelial A549 cell model (38, 39). In particular, it was demonstrated that GPE interfered with nucleoprotein and fusion protein expression, reducing virus replication. In addition to direct antiviral activity, GPE was reported to be effective in alleviating the pathological complications of the viral infection at respiratory level, reducing the expression of (i) mucins, whose levels increased during the airway mucosa inflammation (38) and (ii) pro-inflammatory interleukins, including IL-1β,−6, and−8 (39). In this sense, the anti-inflammatory potential of polyphenols, mainly exerted via reduction of the interleukin levels, appears noteworthy, and investigating this effect in the context of a virus-induced inflammatory status is intriguing. Overall, this evidence may support the use of polyphenolic extracts, including GPE, for the formulation of potential nutraceutical supplements aimed to counteract the COVID-19 infection. This potential activity might be considered in addition to the well-established antioxidant and anti-inflammatory effects of polyphenols, which may contribute to the general management of respiratory complications of coronavirus infection.
Considering this background, the ideal way to test the antiviral polyphenol effect in humans would be a controlled randomized clinical trial with measurable, reproducible, and clinically relevant outcomes. Most of the current trials are set on the compassionate use of the studied treatment or based on single-arm intervention. Thus, definitive conclusion related to efficacy or safety is hardly deducible. On balance, controlled randomized clinical trials with meaningful clinical outcomes are mandatory to best assess the therapeutic effects and the clinical impact of polyphenol treatment on COVID-19 (40).
All the authors contributed to conceptualization, evaluation of the literature and draft-writing.
The authors declare that the research was conducted in the absence of any commercial or financial relationships that could be construed as a potential conflict of interest.
1. Xu Y, Li X, Zhu B, Liang H, Fang C, Gong Y, et al. Characteristics of pediatric SARS-CoV-2 infection and potential evidence for persistent fecal viral shedding. Nat Med. (2020) 26:502–5. doi: 10.1038/s41591-020-0817-4
2. Pan Y, Zhang D, Yang P, Poon LLM, Wang Q. Viral load of SARS-CoV-2 in clinical samples. Lancet Infect Dis. (2020) 20:411–2. doi: 10.1016/S1473-3099(20)30113-4
3. Zou L, Ruan F, Huang M, Liang L, Huang H, Hong Z, et al. SARS-CoV-2 viral load in upper respiratory specimens of infected patients. N Engl J Med. (2020) 382:1177–9. doi: 10.1056/NEJMc2001737
4. Cao B, Wang Y, Wen D, Liu W, Wang J, Fan G, et al. A trial of lopinavir-ritonavir in adults hospitalized with severe Covid-19. N Engl J Med. (2020) 382:1787–99. doi: 10.1056/NEJMoa2001282
5. Clinicaltrials.Gov (2020). Adaptive COVID-19 Treatment Trial. Available online at: https://clinicaltrials.gov/ct2/show/NCT04280705?term=remdesivir&cond=covid-19&draw=2&rank=5 (accessed March 27, 2020).
6. Gautret P, Lagier JC, Parola P, Hoang VT, Meddeb L, Mailhe M, et al. Hydroxychloroquine and azithromycin as a treatment of COVID-19: results of an open-label non-randomized clinical trial. Int J Antimicrob Agents. (2020) 105949. doi: 10.1016/j.ijantimicag.2020.105949. [Epub ahead of print].
7. Shen C, Wang Z, Zhao F, Yang Y, Li J, Yuan J, et al. Treatment of 5 critically Ill patients with COVID-19 with convalescent plasma. JAMA. (2020) 323:1582–9. doi: 10.1001/jama.2020.4783
8. Xu X, Han M, Li T, Sun W, Wang D, Fu B, et al. Effective treatment of severe COVID-19 patients with Tocilizumab. ChinaXiV. (2020) 202003:v1. doi: 10.1073/pnas.2005615117
9. Annunziata G, Jimenez-Garcia M, Capo X, Moranta D, Arnone A, Tenore GC, et al. Microencapsulation as a tool to counteract the typical low bioavailability of polyphenols in the management of diabetes. Food Chem Toxicol. (2020) 139:111248. doi: 10.1016/j.fct.2020.111248
10. Yiu CY, Chen SY, Chang LK, Chiu YF, Lin TP. Inhibitory effects of resveratrol on the Epstein-Barr virus lytic cycle. Molecules. (2010) 15:7115–24. doi: 10.3390/molecules15107115
11. De Leo A, Arena G, Lacanna E, Oliviero G, Colavita F, Mattia E. Resveratrol inhibits epstein barr virus lytic cycle in Burkitt's lymphoma cells by affecting multiple molecular targets. Antiviral Res. (2012) 96:196–202. doi: 10.1016/j.antiviral.2012.09.003
12. Zhang L, Li Y, Gu Z, Wang Y, Shi M, Ji Y, et al. Resveratrol inhibits enterovirus 71 replication and pro-inflammatory cytokine secretion in rhabdosarcoma cells through blocking IKKs/NF- κB signaling pathway. PLoS ONE. (2015) 10:e0116879. doi: 10.1371/journal.pone.0116879
13. Faith SA, Sweet TJ, Bailey E, Booth T, Docherty JJ. Resveratrol suppresses nuclear factor-kappaB in herpes simplex virus infected cells. Antiviral Res. (2006) 72:242–51. doi: 10.1016/j.antiviral.2006.06.011
14. Annunziata G, Maisto M, Schisano C, Ciampaglia R, Narciso V, Tenore GC, et al. Resveratrol as a novel anti-herpes simplex virus nutraceutical agent: an overview. Viruses. (2018) 10:473 doi: 10.3390/v10090473
15. Lin CJ, Lin HJ, Chen TH, Hsu YA, Liu CS, Hwang GY, et al. Polygonum cuspidatum and its active components inhibit replication of the influenza virus through toll-like receptor 9-induced interferon beta expression. PLoS ONE. (2015) 10:e0117602. doi: 10.1371/journal.pone.0117602
16. Zang N, Xie X, Deng Y, Wu S, Wang L, Peng C, et al. Resveratrol-mediated gamma interferon reduction prevents airway inflammation and airway hyperresponsiveness in respiratory syncytial virus-infected immunocompromised mice. J Virol. (2011) 85:13061–8. doi: 10.1128/JVI.05869-11
17. Liu T, Zang N, Zhou N, Li W, Xie X, Deng Y, et al. Resveratrol inhibits the TRIF-dependent pathway by upregulating sterile alpha and armadillo motif protein, contributing to anti-inflammatory effects after respiratory syncytial virus infection. J Virol. (2014) 88:4229–36. doi: 10.1128/JVI.03637-13
18. Mastromarino P, Capobianco D, Cannata F, Nardis C, Mattia E, De Leo A, et al. Resveratrol inhibits rhinovirus replication and expression of inflammatory mediators in nasal epithelia. Antiviral Res. (2015) 123:15–21. doi: 10.1016/j.antiviral.2015.08.010
19. Li H, Wu J, Zhang Z, Ma Y, Liao F, Zhang Y, et al. Forsythoside a inhibits the avian infectious bronchitis virus in cell culture. Phytother Res. (2011) 25:338–42. doi: 10.1002/ptr.3260
20. Roh C. A facile inhibitor screening of SARS coronavirus N protein using nanoparticle-based RNA oligonucleotide. Int J Nanomed. (2012) 7:2173–9. doi: 10.2147/IJN.S31379
21. Lin SC, Ho CT, Chuo WH, Li S, Wang TT, Lin CC. Effective inhibition of MERS-CoV infection by resveratrol. BMC Infect Dis. (2017) 17:144. doi: 10.1186/s12879-017-2253-8
22. Park JY, Yuk HJ, Ryu HW, Lim SH, Kim KS, Park KH, et al. Evaluation of polyphenols from Broussonetia papyrifera as coronavirus protease inhibitors. J Enzyme Inhib Med Chem. (2017) 32:504–15. doi: 10.1080/14756366.2016.1265519
23. Chen C, Zuckerman DM, Brantley S, Sharpe M, Childress K, Hoiczyk E, et al. Sambucus nigra extracts inhibit infectious bronchitis virus at an early point during replication. BMC Vet Res. (2014) 10:24. doi: 10.1186/1746-6148-10-24
24. Veberic R, Jakopic J, Stampar F, Schmitzer V. European elderberry (Sambucus nigra L.) rich in sugars, organic acids, anthocyanins and selected polyphenols. Food Chem. (2009) 114:511–5. doi: 10.1016/j.foodchem.2008.09.080
25. Lee J, Finn CE. Anthocyanins and other polyphenolics in American elderberry (Sambucus canadensis) and European elderberry (S. nigra) cultivars. J Sci Food Agric. (2007) 87, 2665–75. doi: 10.1002/jsfa.3029
26. Schmitzer V, Veberic R, Slatnar A, Stampar F. Elderberry (Sambucus nigra L.) wine: a product rich in health promoting compounds. J Agric Food Chem. (2010) 58:10143–6. doi: 10.1021/jf102083s
27. Roschek B Jr, Fink RC, McMichael MD, Li D, Alberte RS. Elderberry flavonoids bind to and prevent H1N1 infection in vitro. Phytochemistry. (2009)70:1255–61. doi: 10.1016/j.phytochem.2009.06.003
28. Naithani R, Huma LC, Holland LE, Shukla D, McCormick DL, Mehta RG, et al. Antiviral activity of phytochemicals: a comprehensive review. Mini Rev Med Chem. (2008) 8:1106–33. doi: 10.2174/138955708785909943
29. Chen CY, Wang FL, Lin CC. Chronic hydroxychloroquine use associated with QT prolongation and refractory ventricular arrhythmia. Clin Toxicol. (2006) 44:173–5. doi: 10.1080/15563650500514558
30. Morgan ND, Patel SV, Dvorkina O. Suspected hydroxychloroquine-associated QT-interval prolongation in a patient with systemic lupus erythematosus. J Clin Rheumatol. (2013) 19:286–8. doi: 10.1097/RHU.0b013e31829d5e50
31. Fontana AR, Antoniolli A, Bottini R. Grape pomace as a sustainable source of bioactive compounds: extraction, characterization, and biotechnological applications of phenolics. J Agric Food Chem. (2013) 61:8987–9003. doi: 10.1021/jf402586f
32. Beres C, Costa GNS, Cabezudo I, da Silva-James NK, Teles ASC, Cruz APG, et al. Towards integral utilization of grape pomace from winemaking process: a review. Waste Manag. (2017) 68:581–94. doi: 10.1016/j.wasman.2017.07.017
33. Muhlack RA, Potumarthi R, Jeffery DW. Sustainable wineries through waste valorisation: a review of grape marc utilisation for value-added products. Waste Manag. (2018) 72:99–118. doi: 10.1016/j.wasman.2017.11.011
34. Nair MP, Kandaswami C, Mahajan S, Nair HN, Chawda R, Shanahan T, et al. Grape seed extract proanthocyanidins downregulate HIV-1 entry coreceptors, CCR2b, CCR3 and CCR5 gene expression by normal peripheral blood mononuclear cells. Biol Res. (2002) 35:421–31. doi: 10.4067/S0716-97602002000300016
35. Su X, D'Souza DH. Grape seed extract for control of human enteric viruses. Appl Environ Micriobiol. (2011) 77:3982–7. doi: 10.1128/AEM.00193-11
36. Joshi SS, Su X, D'Souza DH. Antiviral effects of grape seed extract against feline calicivirus, murine norovirus, and hepatitis A virus in model food systems and under gastric conditions. Food Microbiol. (2015) 52:1–10. doi: 10.1016/j.fm.2015.05.011
37. Chen WC, Tseng CK, Chen BH, Lin CK, Lee JC. Grape seed extract attenuates hepatitis C virus replication and virus-induced inflammation. Front Pharmacol. (2016) 7:490. doi: 10.3389/fphar.2016.00490
38. Lee JW, Kim YI, Im CN, Kim SW, Kim SJ, Min S, et al. Grape seed proanthocyanidin inhibits mucin synthesis and viral replication by suppression of AP-1 and NF-κB via p38 MAPKs/JNK signaling pathways in respiratory syncytial virus-infected A549 Cells. J Agric Food Chem. (2017) 65:4472–83. doi: 10.1021/acs.jafc.7b00923
39. Kim SJ, Lee JW, Eun YG, Lee KH, Yeo SG, Kim SW. Pretreatment with a grape seed proanthocyanidin extract downregulates proinflammatory cytokine expression in airway epithelial cells infected with respiratory syncytial virus. Mol Med Rep. (2019) 19:3330–6. doi: 10.3892/mmr.2019.9967
Keywords: SARS-CoV2, COVID-19 pandemic, polyphenols, antiviral, nutraceutical
Citation: Annunziata G, Sanduzzi Zamparelli M, Santoro C, Ciampaglia R, Stornaiuolo M, Tenore GC, Sanduzzi A and Novellino E (2020) May Polyphenols Have a Role Against Coronavirus Infection? An Overview of in vitro Evidence. Front. Med. 7:240. doi: 10.3389/fmed.2020.00240
Received: 29 March 2020; Accepted: 06 May 2020;
Published: 15 May 2020.
Edited by:
Ata Murat Kaynar, University of Pittsburgh, United StatesReviewed by:
Xiaojiong Jia, University of Pennsylvania, United StatesCopyright © 2020 Annunziata, Sanduzzi Zamparelli, Santoro, Ciampaglia, Stornaiuolo, Tenore, Sanduzzi and Novellino. This is an open-access article distributed under the terms of the Creative Commons Attribution License (CC BY). The use, distribution or reproduction in other forums is permitted, provided the original author(s) and the copyright owner(s) are credited and that the original publication in this journal is cited, in accordance with accepted academic practice. No use, distribution or reproduction is permitted which does not comply with these terms.
*Correspondence: Gian Carlo Tenore, Z2lhbmNhcmxvLnRlbm9yZUB1bmluYS5pdA==
†These authors have contributed equally to this work and share first authorship
Disclaimer: All claims expressed in this article are solely those of the authors and do not necessarily represent those of their affiliated organizations, or those of the publisher, the editors and the reviewers. Any product that may be evaluated in this article or claim that may be made by its manufacturer is not guaranteed or endorsed by the publisher.
Research integrity at Frontiers
Learn more about the work of our research integrity team to safeguard the quality of each article we publish.