- 1Laboratory of Veterinary Biochemistry, School of Veterinary Medicine, Rakuno Gakuen University, Ebetsu, Japan
- 2ELMS Animal Medical Center, Suginami City, Japan
- 3Vet Derm Tokyo CO., Ltd., Fujisawa, Japan
The spread of antibiotic-resistant bacteria (ARB) in human and veterinary medicine is of global concern. Notably, the emergence of methicillin-resistant Staphylococcus pseudintermedius has become a serious problem. In this context, bacteriophages and their lytic enzymes, endolysins, have received considerable attention as therapeutics for infectious diseases in place of antibiotics. The aim of the present study was to investigate the antibiotic-resistance patterns of staphylococcal species isolated from canine skin at a primary care animal hospital in Tokyo, Japan and evaluate the lytic activity of the staphylococcal bacteriophage phiSA012 and its endolysin Lys-phiSA012 against isolated antibiotic-resistant staphylococcal strains. Forty clinical staphylococcal samples were isolated from infection sites of dogs (20 from skin and 20 from the external ear canal). Susceptibility to antimicrobial agents was determined by a disk diffusion method. The host range of phiSA012 was determined by using a spot test against staphylococcal isolates. Against staphylococcal isolates that showed resistance toward five classes or more of antimicrobials, the lytic activity of phiSA012 and Lys-phiSA012 was evaluated using a turbidity reduction assay. Twenty-three S. pseudintermedius, 16 Staphylococcus schleiferi, and 1 Staphylococcus intermedius were detected from canine skin and ear infections, and results revealed 43.5% methicillin resistance in S. pseudintermedius and 31.3% in S. schleiferi. In addition, the prevalence multidrug resistance (MDR) S. pseudintermedius was 65.2%. PhiSA012 could infect all staphylococcal isolates by spot testing, but showed little lytic activity by turbidity reduction assay against MDR S. pseudintermedius isolates. On the other hand, Lys-phiSA012 showed lytic activity and reduced significantly the number of staphylococcal colony-forming units. These results demonstrated that ARB issues underlying in small animal hospital and proposed substitutes for antibiotics. Lys-phiSA012 has broader lytic activity than phiSA012 against staphylococcal isolates; therefore, Lys-phiSA012 is a more potential candidate therapeutic agent for several staphylococcal infections including that of canine skin.
Introduction
Administration of systemic and/or topical antimicrobial agents is the most common therapeutic treatment for bacterial infectious diseases. Besides, antibiotics are often contained in feed for growth promotion of livestock (1). It has been previously reported that antibiotics are used in livestock more so than in humans (2–4). In this context, the overuse and misuse of antibiotics in humans and animals have resulted in the global wide-spread emergence of antibiotic-resistant bacteria (ARB) (5). A previous study by the UK government estimated that ARB would cause tens of millions of deaths per year and pose a greater human health risk than cancer by 2050 (6, 7).
Staphylococcus aureus is a well-known member of the human skin microbiota (8–10). However, methicillin-resistant S. aureus (MRSA) is one of the most frequently isolated ARB and can cause nosocomial infections (11). Meanwhile, Staphylococcus pseudintermedius is most frequently isolated as part of the normal canine skin flora and also as a pathogen of pyoderma, bacterial otitis, wounds, and abscess. As with MRSA in humans, methicillin-resistant S. pseudintermedius (MRSP) is frequently isolated from canine skin infections. It has been suggested that MRSP has acquired multi-drug resistance (MDR) to antimicrobials (12). Therefore, it is imperative that alternative therapeutic agents against staphylococci including MRSP are identified in the field of companion animal medicine.
At present, the use of bacteriophages as therapy for infectious diseases is receiving significant attention (13, 14). Bacteriophages are viruses that specifically infect bacteria and are lethal (15, 16). Previously, we reported the isolation of a bacteriophage against S. aureus, phiSA012, which showed efficient lytic activity toward various S. aureus strains in vitro (17) and demonstrated the therapeutic effect of phiSA012 on a mouse mastitis model caused by S. aureus (18).
Endolysins are bacteriophage-encoded and are translated at the end of the phage life cycle to lyse host bacteria, by hydrolyzing cell wall peptidoglycan from within or without, leading to the release of phage offspring (19–21). Most of the endolysins targeting Gram-positive bacteria harbor two functional domains: an enzymatically active domain (EAD), which hydrolyzes specific peptidoglycan bonds, and a cell wall–binding domain (CBD), which recognizes and binds specific peptidoglycan ligands or secondary cell wall polymers such as teichoic acids and determines the spectrum of endolysins within particular bacterial species or strains (22, 23). Staphylococcus aureus bacteriophage endolysins such as Lys-phiK, Lys-GH15, and Lys-phiSA012 harbor a multidomain composed of a cysteine, histidine-dependent amidohydrolase/peptidase domain and an amidase (AMID) domain as EADs, and an SH3b as a CBD at the C-terminus (21, 24, 25). It has been suggested that bacteria cannot easily acquire endolysin resistance (26, 27). In fact, it was reported that several bacteria could not develop resistance against endolysins after repeated exposure, as endolysins may cleave peptidoglycan sites that are essential for bacterial survival (23, 28, 29). At present, thus, endolysins are garnering abundant attention as a substitute for antibiotics in the treatment of bacterial infections (30–32). Previously, we reported that an endolysin, Lys-phiSA012, which was derived from phiSA012 and is categorized as a Lys-phiK like endolysin, exhibited high lytic activity against S. aureus including MRSA, a S. pseudintermedius strain, and a Staphylococcus haemolyticus strain (25).
The objective of the present study was to investigate the antibiotic-resistance patterns of staphylococcal species, isolated from canine skin at a primary care animal hospital, to confirm the spread of MRSP and MDR S. pseudintermedius in companion animals. In addition, we examined the utility of polyvalent phiSA012 and its endolysin Lys-phiSA012 as a treatment against clinical staphylococcal isolates, thereby expanding the available treatment options against ARB.
Materials and Methods
Sample Collection and Bacterial Identification
All samples were isolated from dogs visiting the ELMS Animal Medical Center, Tokyo, Japan. A total of 40 clinical isolates were collected aseptically from infected sites of dogs (20 from skin and 20 from the external ear canal) from April 2017 to August 2018. The animal study was reviewed and approved by Animal Care and Use Committee of Rakuno Gakuen University (Approval No. VH19B22). Each lesion site was sampled using a Culture Swab Plus (Nippon Becton Dickinson Company, Ltd., Tokyo, Japan) and sent to Vet Derm Tokyo, Ltd. for bacterial identification and determination of antibiotic resistance profile.
Clinical samples were cultivated on 5% sheep blood agar and incubated at 37°C for 24 h. Staphylococci were identified by BBLCRYSTAL (Nippon Becton Dickinson Company, Ltd.), and nuc-targeted multiplex polymerase chain reaction amplification was conducted for genotypic confirmation of S. aureus, S. pseudintermedius, and Staphylococcus schleiferi identification (33). Staphylococcus pseudintermedius used in the turbidity reduction assay was classified using the random amplification polymorphic DNA (RAPD) method to detect polymorphisms in S. pseudintermedius isolates (33).
Antimicrobial Susceptibility Testing
Susceptibility to a panel of 21 antimicrobial agents was determined by a disk diffusion method according to the guidelines of the Clinical Laboratory Standards Institute (34, 35). The examined antibiotics were oxacillin (1 μg), amoxicillin/clavulanic acid (20/10 μg), cephalexin (30 μg), cefpodoxime (10 μg), cefovecin (30 μg), faropenem (5 μg), enrofloxacin (5 μg), orbifloxacin (10 μg), marbofloxacin (5 μg), gentamicin (10 μg), fradiomycin (30 μg), sulfamethoxazole/trimethoprim (23.75/1.25 μg), clindamycin (2 μg), lincomycin (2 μg), erythromycin (15 μg), doxycycline (30 μg), minocycline (30 μg), chloramphenicol (30 μg), florfenicol (30 μg), fosfomycin (50 μg), and rifampicin (5 μg).
Bacteriophage and Endolysin Preparation
The S. aureus virulent phage phiSA012 (accession number NC_023573.1) was isolated from sewage in Tokyo, Japan in a previous study (17). PhiSA012 was propagated by the plate lysate method (36). In brief, 100 μL of phage lysate was mixed with 100 μL of an overnight culture of S. aureus strain SA003 (17) in 3 mL of 0.5% top agar, then plated on Luria-Bertani (LB) agar, and incubated at 37°C overnight. After 3 mL of salt magnesium buffer [100 mM NaCl, 8 mM MgSO4, 50 mM Tris-HCl (pH 7.5), 0.01% gelatin] was added to the plate, the overlayer was scraped off to extract the phage, and the supernatant was collected by centrifugation (10,000 × g for 5 min at 4°C). The supernatant was purified by CsCl density gradient centrifugation (36). Purified phiSA012 was titrated and stored at 4°C until use.
Lys-phiSA012 was expressed and purified as described in our previous report (25). In brief, Escherichia coli BL21(DE3) strain, which possesses the Lys-phiSA012 encoding plasmid, was cultured in LB medium containing 100 μg/mL of ampicillin, and protein expression was induced by the addition of isopropyl β-thiogalactopyranoside to a final concentration of 0.1 mM at the logarithmic phase (corresponding to 0.4–0.6 OD600) and then incubated overnight at 25°C with shaking. Cells were harvested by centrifugation (2,300 × g for 5 min at 4°C) and the pellets were lysed via sonication. The lysate was centrifuged at 16,000 × g for 30 min at 4°C, and then the supernatant containing soluble GST-tagged protein was collected using a chromatographic column packed with Glutathione Sepharose 4B. Pure Lys-phiSA012 protein was obtained by loading the PreScission Protease mix [80 μL (160 units)] of PreScission Protease and 920 μL of cleavage buffer (50 mM Tris-HCl, 150 mM NaCl, 1 mM dithiothreitol) onto the column and then stored at −30°C until use.
Spot Testing
Four microliters of phiSA012 suspension at a titer of 109 plaque-forming unit (PFU)/mL was dropped onto a double-layer LB agar plate containing the staphylococcal isolates and S. aureus SA003 as a positive control. After overnight incubation at 37°C, the infected area was characterized as one of four categories: clear plaque (C), turbid plaque (T), faint plaque (F), or no plaque (N).
Turbidity Reduction Assay
All staphylococcal isolates that showed resistance against five classes or more of antimicrobials (SS3014, SP3018, SP3401, SP3567, SP4531, SP5158, SP5405, SP5432, SP5515, SP7369, SP7454, SP7542, and SP7971) and SA003 were grown in LB medium. At a concentration of approximately 108 colony-forming units (CFU/mL), each bacterium was mixed with phiSA012 suspension at a final titer of 108 PFU/ml (Multiplicity of infection ≒ 1.0), and then the OD595 value was monitored using a plate reader (Sunrise Rainbow Thermos RC; TECAN Austria GmbH, Salzburg, Austria) with incubation.
The lytic activity of Lys-phiSA012 was assessed using a turbidity reduction assay as described previously (25). In brief, the same as above staphylococcal isolates were grown in LB medium at 37°C to an OD600 of 1.0. Each culture was centrifuged at 2,300 × g for 5 min at 4°C, and the cells were resuspended in 2 × LB medium, and then stored on ice until use. The turbidity reduction assay was initiated by adding the same amount of purified Lys-phiSA012 (100 μg/mL), and then the OD595 value was monitored using a plate reader with incubation. The decrease in viable cells corresponding to the loss of turbidity was tested by plating the aliquots from the reaction solution of turbidity assay at the end of monitoring (2 h) for counting of CFUs.
Statistical Analysis
Statistical analysis was performed using two-tailed Student t-tests or Welch t-tests according to the result of F-tests, and Dunnett test from three independent experiments. p < 0.05 was considered to be statistically significant.
Results
Strain Identification and Antimicrobial Susceptibility Testing
The prevalence of MRSP in veterinary teaching hospitals has been confirmed in several reports, but that in primary care animal hospitals remains unclear. Therefore, we investigated the antibiotic-resistance patterns of staphylococcal isolates at a primary care animal hospital in Tokyo, Japan. The most frequently isolated Staphylococcus species was S. pseudintermedius (23/40), followed by S. schleiferi (16/40), and Staphylococcus intermedius (1/40). The antibiotic-resistance pattern is summarized in Table 1 and Figure 1. Methicillin resistance as determined by oxacillin disk susceptibility was 43.5% in S. pseudintermedius (10/23) and 31.3% in S. schleiferi (5/16). Surprisingly, both S. pseudintermedius and S. schleiferi exhibited obvious resistance to fluoroquinolones (enrofloxacin, orbifloxacin, and marbofloxacin). Next, we attempted to determine whether these isolates demonstrated MDR based on the definition proposed by the European Center for Disease Prevention and Control and the Centers for Disease Control and Prevention (37). The prevalence of MDR was 65.2% (15/23) for S. pseudintermedius and 12.5% (2/16) for S. schleiferi (Supplementary Table S1). On the other hand, no resistance was observed toward faropenem, florfenicol, and rifampicin for all staphylococcal isolates.
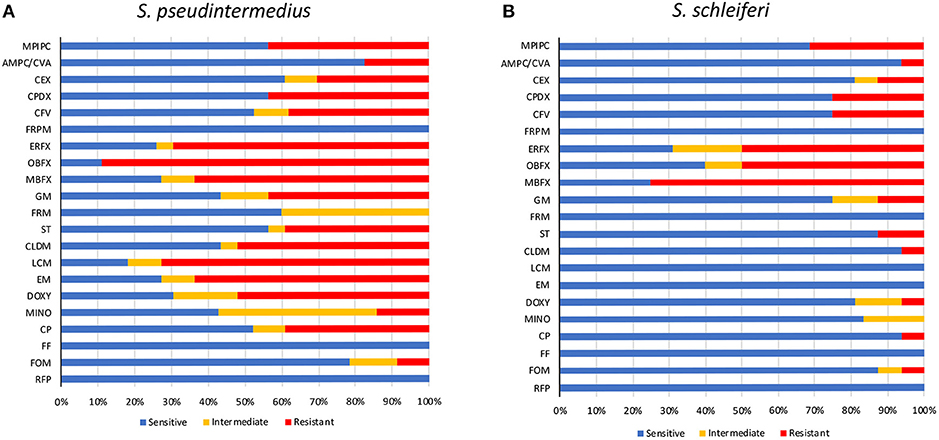
Figure 1. Antimicrobial susceptibility of staphylococcal isolates. Antimicrobial susceptibility of S. pseudintermedius (A) and S. schleiferi (B). MPIPC, oxacillin; AMPC/CVA, amoxycillin/clavulanic acid; CEX, cephalexin; CPDX, cefpodoxime; CFV, cefovecin; FRPM, faropenem; ERFX, enrofloxacin; OBFX, orbifloxacin; MBFX, marbofloxacin; GM, gentamicin; FRM, fradiomycin; ST, sulfamethoxazole/trimethoprim; CLDM, clindamycin; LCM, lincomycin; EM, erythromycin; DOXY, doxycycline; MINO, minocycline; CP, chloramphenicol; FF, florfenicol; FOM, fosfomycin; RFP, rifampicin.
Host Range and Lytic Activity of the Bacteriophage phiSA012 Against Staphylococcal Isolates
PhiSA012 has been reported as a broad-range lytic phage against S. aureus strains (17, 18). In this study, we evaluated the host range of phiSA012 against all staphylococcal isolates except two S. pseudintermedius strains (SP3399 and SP6931), which could not be re-cultured. PhiSA012 could infect all staphylococcal isolates (S. pseudintermedius, S. schleiferi, and S. intermedius) including methicillin-resistant and MDR staphylococci (Figure 2A). Against S. pseudintermedius, clear plaques, turbid plaques and faint plaques accounted for 9.5% (2/21), 71.4% (15/21), and 19.0% (4/21), respectively.
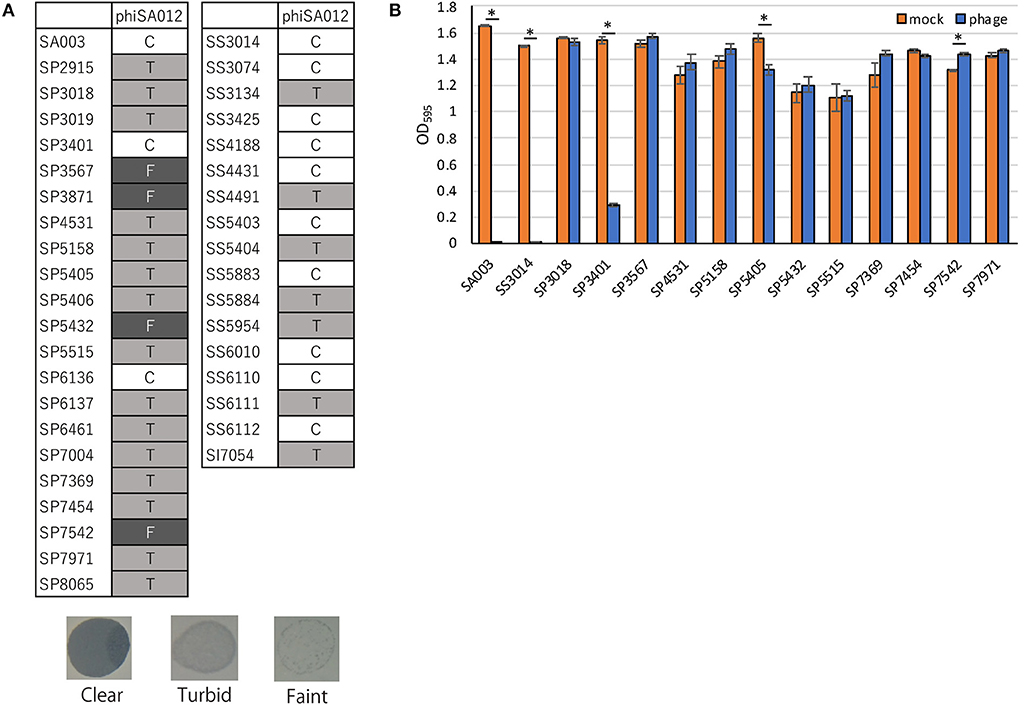
Figure 2. Host range and lytic activity of phiSA012 against staphylococcal isolates from canine skin infection sites. Host range and lytic activity of phiSA012 were evaluated by spot testing (A) and turbidity reduction assay (B). (A) Clear plaques “C” indicates the highest lysis activity, followed by turbid plaques “T” and faint plaques “F” in spot testing. Representative images of formed spots are showed below. (B) The graph shows OD595 value of mock treated or phage treated bacterial cultures at the end of monitoring (24 h). The columns indicate the mean and error bars indicate standard error (SE). Statistical significance (*p < 0.01) is indicated with an asterisk by Student's t-test.
In addition to spot-testing evaluation, turbidity reduction assays were performed to observe sequential and quantitative lytic activity of phiSA012 (Supplementary Figure S1). As target bacteria, 12 MDR S. pseudintermedius strains and one MDR S. schleiferi that showed resistance against five classes or more of antimicrobials were selected, and SA003 was used as a positive control. Twelve S. pseudintermedius strains exhibited different polymorphisms in the RAPD method (Supplementary Figures S2A,B). PhiSA012 significantly inhibited bacterial growth of SA003, SS3014, SP3401, and SP5405 compared with mock (Figure 2B). On the other hand, phiSA012 showed slightly bacterial growth inhibition in a few hours after phage infection against others isolates, but there was little difference in OD595 values compared with mock at the 24 h point (Figure 2B, Supplementary Figure S1). These results demonstrated that phiSA012 could infect all staphylococcal isolates, but showed little lytic activity against most of the S. pseudintermedius isolates.
Lytic Activity of the Endolysin Lys-phiSA012 Against MDR S. pseudintermedius
Previously, we reported that Lys-phiSA012 showed lytic activity against S. pseudintermedius (25). In this study, we examined whether Lys-phiSA012 showed lytic activity against 13 MDR staphylococcal clinical isolates. Except SP3401, Lys-phiSA012 showed rapid antimicrobial activity in turbidity reduction assay against all MDR S. pseudintermedius and S. schleiferi within 2 h (Figure 3A, Supplementary Figure S3). Next, we confirmed the decrease in viable cells corresponding to the loss of turbidity by counting CFUs at the end of turbidity monitoring (2 h). Lys-phiSA012 significantly yielded 2–5.5 log reduction of CFUs compared to buffer controls, except SP3401 (Figure 3B).
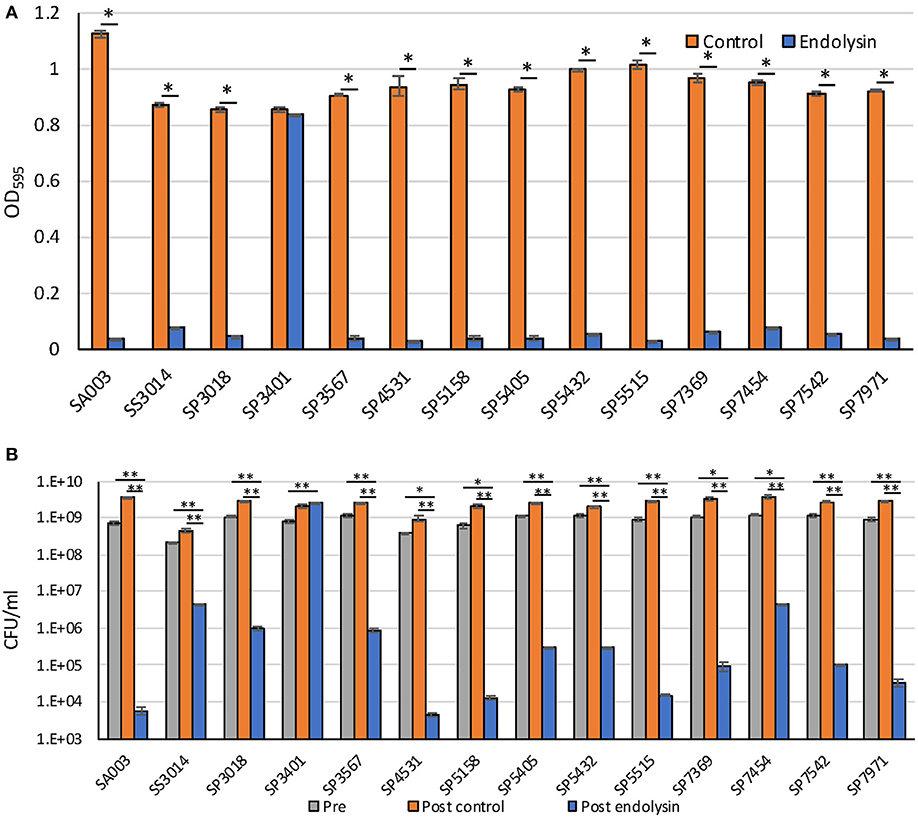
Figure 3. Lytic activity of Lys-phiSA012 against MDR staphylococcal isolates. Lytic activity of Lys-phiSA012 was evaluated by turbidity reduction assay (A) and following CFUs counting (B). The columns indicate the mean and error bars indicate standard error (SE). (A) The graph shows OD595 value of buffer control treated or endolysin treated bacterial cultures at the end of monitoring (2 h). Statistical significance (*p < 0.01) is indicated with an asterisk by Student's t-test or Welch's t-test. (B) The graph shows CFUs of pretreated, post–buffer control treated or post–endolysin-treated bacterial cultures at the end of monitoring (2 h). Statistical significance (*p < 0.05 and **p < 0.01) is indicated with an asterisk by Dunnett's test.
Discussion
At present, the emergence of ARB is one of the most pressing global health issues. Particularly, ESKAPE pathogens (Enterococcus faecium, S. aureus, Klebsiella pneumoniae, Acinetobacter baumannii, Pseudomonas aeruginosa, and Enterobacter species) have the potential to develop several drug resistance mechanisms and cause lethal nosocomial infections (38). We have demonstrated the therapeutic potential of bacteriophages and endolysins against P. aeruginosa and S. aureus as alternatives to antimicrobials (17, 18, 25, 36, 39). In the present study, we focused on antibiotic-resistant staphylococci isolated from canine skin infectious diseases and evaluated the lytic activity of the bacteriophage phiSA012 and its endolysin Lys-phiSA012.
The most frequently isolated strains in this study were S. pseudintermedius, and the second were S. schleiferi. Staphylococcus pseudintermedius is well-known as the main cause of canine pyoderma and external otitis, and S. schleiferi is also a causative agent of these infections. As with MRSA in human medicine, the emergence of MRSP is of concern in veterinary medicine. According to several reports, the MRSP isolation frequencies were 10.4% in Spain, 17.6% in Korea, 27.3–38.2% in the United States, 47.9% in South China, and 57% in Japan (12, 40–43). In this study, the frequency of MRSP was 43.5%, which was lower than that in teaching hospitals in Japan (57%). In university or teaching hospitals, many cases are treated with antibiotics prior to referral, and this may result in a high prevalence of MRSP compared with primary care hospitals. Several reports described that S. schleiferi has a high rate of methicillin resistance compared with S. pseudintermedius (44, 45); however, in this investigation, the rate of MRSP was higher than methicillin-resistant S. schleiferi (Figure 1). In addition, high rates of fluoroquinolone-resistant S. pseudintermedius and S. schleiferi were detected in this investigation. Moreover, and most remarkably, the rate of MDR S. pseudintermedius was 65.2%, which was greater than the rate of MRSP. According to the present study, the emergence of antibiotic-resistant staphylococci is becoming a serious problem in primary care animal hospitals, necessitating confirmation of the need for antibiotics in the treatment of individual cases as well as ongoing surveillance of ARB prevalence.
Adsorption of a bacteriophage to the host cell is the first step of infection and is one of the important processes that determines the host range (46). Staphylococci have various glycoepitopes (GlcNAc, GalNAc, or Glc) and two types of wall teichoic acid (WTA) backbone [ribitol–phosphate (RboP) and glycerol–phosphate (GroP)] (47, 48). The infectivity of staphylococcal Siphoviridae and Podoviridae phages depends on the WTA type of the host cell; for example Siphoviridae phage Φ11 only recognizes GlcNAc of RboP WTA (49). On the other hand, phiSA012, which is classified as a Myoviridae phage, utilizes the back bone of WTA as a receptor and can infect several staphylococci regardless of the WTA type (46, 50). We and another research group reported that phiSA012 has a broad host range against various S. aureus strains including MRSA (17, 18). In addition to the previous reports, we found that phiSA012 could infect MDR S. pseudintermedius, S. schleiferi, and S. intermedius by spot testing. To apply bacteriophages as therapeutics for canine pyoderma or otitis externa, it is important determine not only the host range toward staphylococci but also the strength of the lytic activity, especially against S. pseudintermedius and S. schleiferi. However, the lytic activity of phiSA012 against S. pseudintermedius might be somewhat weak, as the rate of making clear plaques during spot testing was fewer than against S. aureus in the present study and previous reports (18, 50). In addition, turbidity reduction assay confirmed that phiSA012 has little activity against most of the staphylococcal isolates which were showed turbid or faint plaques by spot testing (Figure 2, Supplementary Figure S1). On the other hand, S. pseudintermedius phages ΦSP0120, ΦSP0197, and ΦSP276 could infect only S. pseudintermedius of the staphylococci; however, they showed clear plaques toward almost all S. pseudintermedius strains (50). Therefore, it might be preferable to find and use bacteriophages that show high lytic activity toward S. pseudintermedius to treat canine pyoderma or externa otitis.
We previously demonstrated that Lys-phiSA012 had lytic activity against S. aureus, S. pseudintermedius, and S. haemolyticus (25). In this study, we examined whether Lys-phiSA012 has lytic activity against several MDR staphylococcal clinical strains isolated from canine skin. We observed that Lys-phiSA012 showed lytic activity and reduced significantly the number of CFUs compared to buffer control against almost all MDR staphylococcal isolates (Figure 3, Supplementary Figure S3). In addition, phiSA012 showed little lytic activity against almost of the S. pseudintermedius isolates, whereas Lys-phiSA012 showed clearly lytic activity toward staphylococcal isolates including these strains. Lys-phiSA012 harbors the SH3b domain as a CBD, and this domain has been shown to recognize and bind the pentaglycine bridge of peptidoglycan, a characteristic structure of most staphylococci (51). That is to say, Lys-phiSA012 probably shows lytic activity against almost all staphylococci that have the pentaglycine bridge in peptidoglycan and a broader host range than phiSA012. On the other hands, it has been previously reported that WTAs conformations affect binding of endolysins to the cells and prevents lysis from without by endolysins (52, 53). In this study, only SP3401 could not be lysed by Lys-phiSA012 but lysed by phiSA012, suggesting that WTAs on SP3401 play a role in phiSA012 infection as a receptor while might inhibit the lysis from without mediated by the Lys-phiSA012. Further investigations are required to elucidate the mechanisms underlying lysis from without, which would contribute to appropriate use of phiSA012 and Lys-phiSA012.
Conclusion
Our investigation indicated that the emergence of MDR S. pseudintermedius is becoming a serious problem in primary care animal hospitals as observed for MRSP. In the context of ARB emergence, we propose that phage therapy has therapeutic potential as an alternative to antibiotics. We confirmed that phiSA012 has a broad range toward staphylococci; however, further studies are warranted to verify whether its lytic activity is sufficient to treat canine skin staphylococcal infections. Moreover, Lys-phiSA012 showed lytic activity against most of the MDR S. pseudintermedius and S. schleiferi strains. This finding supports the use of Lys-phiSA012 as a candidate therapeutic agent for canine skin staphylococcal infections.
Data Availability Statement
All datasets generated for this study are included in the article/Supplementary Material.
Author Contributions
TN, JF, and HI designed experiments. TN and MT collected skin swab samples. KI and KS performed bacterial identification and antimicrobial susceptibility testing. TN and JK performed experiments and analyzed the data. TN and HI wrote the paper.
Funding
This study was supported by Grants-in-Aid for Scientific Research (A) program (JP17H01506) from Japan Society for the Promotion of Science (JSPS) KAKENHI.
Conflict of Interest
Authors KI and KS were employed by company Vet Derm Tokyo, Ltd.
The remaining authors declare that the research was conducted in the absence of any commercial or financial relationships that could be construed as a potential conflict of interest.
Supplementary Material
The Supplementary Material for this article can be found online at: https://www.frontiersin.org/articles/10.3389/fmed.2020.00234/full#supplementary-material
References
1. Silbergeld EK, Graham J, Price LB. Industrial food animal production, antimicrobial resistance, and human health. Annu Rev Public Health. (2008) 29:151–69. doi: 10.1146/annurev.publhealth.29.020907.090904
2. Smith TC, Gebreyes WA, Abley MJ, Harper AL, Forshey BM, Male MJ, et al. Methicillin-resistant Staphylococcus aureus in Pigs and Farm Workers on Conventional and Antibiotic-Free Swine Farms in the USA. PLoS ONE. (2013) 8:e63704. doi: 10.1371/journal.pone.0063704
3. Neyra RC, Frisancho JA, Rinsky JL, Resnick C, Carroll KC, Rule AM, et al. Multidrug-resistant and Methicillin-Resistant Staphylococcus aureus (MRSA) in hog slaughter and processing plant workers and their community in North Carolina (USA). Environ Health Perspect. (2014) 122:471–477. doi: 10.1289/ehp.1306741
4. Khanna T, Friendship R, Dewey C, Weese JS. Methicillin resistant Staphylococcus aureus colonization in pigs and pig farmers. Vet Microbiol. (2008) 128:298–303. doi: 10.1016/j.vetmic.2007.10.006
5. Jansen KU, Knirsch C, Anderson AS. The role of vaccines in preventing bacterial antimicrobial resistance. Nat Med. (2018) 24:10–19. doi: 10.1038/nm.4465
6. de Kraker MEA, Stewardson AJ, Harbarth S. Will 10 million people die a year due to antimicrobial resistance by 2050? PLOS Med. (2016) 13:e1002184. doi: 10.1371/journal.pmed.1002184
7. O'Neill J. Antimicrobial Resistance: Tackling a Crisis for the Health and Wealth of Nations. London: Review on Antimicrobial Resistance (2014). Available online: https://amr-review.org/sites/default/files/AMR%20Review%20Paper%20-%20Tackling%20a%20crisis%20for%20the%20health%20and%20wealth%20of%20nations_1.pdf (accessed May 29, 2020).
8. Bannoehr J, Ben Zakour NL, Waller AS, Guardabassi L, Thoday KL, van den Broek AHM, et al. Population genetic structure of the Staphylococcus intermedius group: insights into agr diversification and the emergence of methicillin-resistant strains. J Bacteriol. (2007) 189:8685–92. doi: 10.1128/JB.01150-07
9. May ER, Kinyon JM, Noxon JO. Nasal carriage of Staphylococcus schleiferi from healthy dogs and dogs with otitis, pyoderma or both. Vet Microbiol. (2012) 160:443–8. doi: 10.1016/j.vetmic.2012.06.020
10. Kluytmans J, van Belkum A, Verbrugh H. Nasal carriage of Staphylococcus aureus: epidemiology, underlying mechanisms, and associated risks. Clin Microbiol Rev. (1997) 10:505–20. doi: 10.1128/CMR.10.3.505
11. Klevens RM, Morrison MA, Nadle J, Petit S, Gershman K, Ray S, et al. Invasive methicillin-resistant Staphylococcus aureus infections in the United States. J Am Med Assoc. (2007) 298:1763–71. doi: 10.1001/jama.298.15.1763
12. Huerta B, Maldonado A, Ginel PJ, Tarradas C, Gómez-Gascón L, Astorga RJ, et al. Risk factors associated with the antimicrobial resistance of staphylococci in canine pyoderma. Vet Microbiol. (2011) 150:302–8. doi: 10.1016/j.vetmic.2011.02.002
13. Domingo-Calap P, Delgado-Martínez J. Bacteriophages: protagonists of a post-antibiotic era. Antibiotics. (2018) 7:66. doi: 10.3390/antibiotics7030066
14. Golkar Z, Bagasra O, Pace DG. Bacteriophage therapy: a potential solution for the antibiotic resistance crisis. J Infect Dev Ctries. (2014) 8:129–36. doi: 10.3855/jidc.3573
15. Cisek AA, Dabrowska I, Gregorczyk KP, Wyzewski Z. Phage therapy in bacterial infections treatment: one hundred years after the discovery of bacteriophages. Curr Microbiol. (2017) 74:277–83. doi: 10.1007/s00284-016-1166-x
16. El-Shibiny A, El-Sahhar S. Bacteriophages: the possible solution to treat infections caused by pathogenic bacteria. Can J Microbiol. (2017) 63:865–79. doi: 10.1139/cjm-2017-0030
17. Synnott AJ, Kuang Y, Kurimoto M, Yamamichi K, Iwano H, Tanji Y. Isolation from sewage influent and characterization of novel Staphylococcus aureus bacteriophages with wide host ranges and potent lytic capabilities. Appl Environ Microbiol. (2009) 75:4483–90. doi: 10.1128/AEM.02641-08
18. Iwano H, Inoue Y, Takasago T, Kobayashi H, Furusawa T, Taniguchi K, et al. Bacteriophage ΦSA012 has a broad host range against Staphylococcus aureus and effective lytic capacity in a mouse mastitis model. Biology. (2018) 7:8. doi: 10.3390/biology7010008
19. Obeso JM, Martínez B, Rodríguez A, García P. Lytic activity of the recombinant staphylococcal bacteriophage ΦH5 endolysin active against Staphylococcus aureus in milk. Int J Food Microbiol. (2008) 128:212–8. doi: 10.1016/j.ijfoodmicro.2008.08.010
20. Schmelcher M, Shen Y, Nelson DC, Eugster MR, Eichenseher F, Hanke DC, et al. Evolutionarily distinct bacteriophage endolysins featuring conserved peptidoglycan cleavage sites protect mice from MRSA infection. J Antimicrob Chemother. (2015) 70:1453–65. doi: 10.1093/jac/dku552
21. Gu J, Xu W, Lei L, Huang J, Feng X, Sun C, et al. LysGH15, a novel bacteriophage lysin, protects a murine bacteremia model efficiently against lethal methicillin-resistant Staphylococcus aureus infection. J Clin Microbiol. (2011) 49:111–7. doi: 10.1128/JCM.01144-10
22. Loessner MJ. Bacteriophage endolysins — current state of research and applications. Curr Opin Microbiol. (2005) 8:480–7. doi: 10.1016/j.mib.2005.06.002
23. Schuch R, Nelson D, Fischetti VA. A bacteriolytic agent that detects and kills Bacillus anthracis. Nature. (2002) 418:884–9. doi: 10.1038/nature01026
24. O'Flaherty S, Coffey A, Meaney W, Fitzgerald GF, Ross RP. The recombinant phage lysin lysk has a broad spectrum of lytic activity against clinically relevant staphylococci, including methicillin-resistant Staphylococcus aureus. J Bacteriol. (2005) 187:7161–4. doi: 10.1128/JB.187.20.7161-7164.2005
25. Fujiki J, Nakamura T, Furusawa T, Ohno H, Takahashi H, Kitana J, et al. Characterization of the lytic capability of a LysK-like endolysin, Lys-phiSA012, derived from a polyvalent Staphylococcus aureus bacteriophage. Pharmaceuticals. (2018) 11:25. doi: 10.3390/ph11010025
26. Fischetti VA. Bacteriophage lytic enzymes: novel anti-infectives. Trends Microbiol. (2005) 13:491–6. doi: 10.1016/j.tim.2005.08.007
27. Loeffler JM. Rapid killing of Streptococcus pneumoniae with a bacteriophage cell wall hydrolase. Science. (2001) 294:2170–2. doi: 10.1126/science.1066869
28. Rodríguez-Rubio L, Martínez B, Rodríguez A, Donovan DM, Götz F, García P. The phage lytic proteins from the Staphylococcus aureus bacteriophage vB_SauS-phiIPLA88 display multiple active catalytic domains and do not trigger staphylococcal resistance. PLoS ONE. (2013) 8:e64671. doi: 10.1371/journal.pone.0064671
29. Pastagia M, Euler C, Chahales P, Fuentes-Duculan J, Krueger JG, Fischetti VA. A novel chimeric lysin shows superiority to mupirocin for skin decolonization of methicillin-resistant and -sensitive Staphylococcus aureus strains. Antimicrob Agents Chemother. (2011) 55:738–744. doi: 10.1128/AAC.00890-10
30. Haddad Kashani H, Schmelcher M, Sabzalipoor H, Seyed Hosseini E, Moniri R. Recombinant endolysins as potential therapeutics against antibiotic-resistant Staphylococcus aureus : current status of research and novel delivery strategies. Clin Microbiol Rev. (2017) 31:e00071–17. doi: 10.1128/CMR.00071-17
31. São-José C. Engineering of phage-derived lytic enzymes: improving their potential as antimicrobials. Antibiotics. (2018) 7:29. doi: 10.3390/antibiotics7020029
32. Schmelcher M, Loessner MJ. Bacteriophage endolysins: applications for food safety. Curr Opin Biotechnol. (2016) 37:76–87. doi: 10.1016/j.copbio.2015.10.005
33. Wang D, Zhang L, Zhou X, He Y, Yong C, Shen M, et al. Antimicrobial susceptibility, virulence genes, and randomly amplified polymorphic DNA analysis of Staphylococcus aureus recovered from bovine mastitis in Ningxia, China. J Dairy Sci. (2016) 99:9560–9. doi: 10.3168/jds.2016-11625
34. Clinical and Laboratory Standards Institute. Performance Standards for Antimicrobial Disk and Dilution Susceptibility Tests for Bacteria Isolated from Animals. 4th ed. CLSI Suppl VET08. Wayne, PA: Clinical and Laboratory Standards Institute (2018).
35. Clinical and Laboratory Standards Institute. Performance Standards for Antimicrobial Susceptibility Testing. 28th ed. CLSI Suppl M100. Wayne, PA: Clinical and Laboratory Standards Institute (2018).
36. Furusawa T, Iwano H, Hiyashimizu Y, Matsubara K, Higuchi H, Nagahata H, et al. Phage therapy is effective in a mouse model of bacterial equine keratitis. Appl Environ Microbiol. (2016) 82:5332–9. doi: 10.1128/AEM.01166-16
37. Magiorakos A-P, Srinivasan A, Carey RB, Carmeli Y, Falagas ME, Giske CG, et al. Multidrug-resistant, extensively drug-resistant and pandrug-resistant bacteria: an international expert proposal for interim standard definitions for acquired resistance. Clin Microbiol Infect. (2012) 18:268–281. doi: 10.1111/j.1469-0691.2011.03570.x
38. Rice LB. Progress and challenges in implementing the research on ESKAPE pathogens. Infect Control Hosp Epidemiol. (2010) 31:S7–10. doi: 10.1086/655995
39. Furusawa T, Iwano H, Higuchi H, Yokota H, Usui M, Iwasaki T, et al. Bacteriophage can lyse antibiotic-resistant Pseudomonas aeruginosa isolated from canine diseases. J Vet Med Sci. (2016) 78:1035–8. doi: 10.1292/jvms.15-0310
40. Feng Y, Tian W, Lin D, Luo Q, Zhou Y, Yang T, et al. Prevalence and characterization of methicillin-resistant Staphylococcus pseudintermedius in pets from South China. Vet Microbiol. (2012) 160:517–24. doi: 10.1016/j.vetmic.2012.06.015
41. Yoon JW, Lee KJ, Lee SY, Chae MJ, Park JK, Yoo JH, et al. Antibiotic resistance profiles of Staphylococcus pseudintermedius isolates from canine patients in Korea. J Microbiol Biotechnol. (2010) 20:1764–8. doi: 10.4014/jmb.1001.01011
42. Eckholm NG, Outerbridge CA, White SD, Sykes JE. Prevalence of and risk factors for isolation of meticillin-resistant Staphylococcus spp. from dogs with pyoderma in northern California, USA. Vet Dermatol. (2013) 24:154–e34. doi: 10.1111/j.1365-3164.2012.01051.x
43. Murayama N, Nagata M, Terada Y, Okuaki M, Takemura N, Nakaminami H, et al. In vitro antiseptic susceptibilities for Staphylococcus pseudintermedius isolated from canine superficial pyoderma in Japan. Vet Dermatol. (2013) 24:126–9e29. doi: 10.1111/j.1365-3164.2012.01103.x
44. Jones RD, Kania SA, Rohrbach BW, Frank LA, Bemis DA. Prevalence of oxacillin- and multidrug-resistant staphylococci in clinical samples from dogs: 1,772 samples (2001–2005). J Am Vet Med Assoc. (2007) 230:221–7. doi: 10.2460/javma.230.2.221
45. Cain CL, Morris DO, O'Shea K, Rankin SC. Genotypic relatedness and phenotypic characterization of Staphylococcus schleiferi subspecies in clinical samples from dogs. Am J Vet Res. (2011) 72:96–102. doi: 10.2460/ajvr.72.1.96
46. Takeuchi I, Osada K, Azam AH, Asakawa H, Miyanaga K, Tanji Y. The presence of two receptor-binding proteins contributes to the wide host range of staphylococcal twort-like phages. Appl Environ Microbiol. (2016) 82:5763–74. doi: 10.1128/AEM.01385-16
47. Endl J, Seidl HP, Fiedler F, Schleider KH. Chemical composition and structure of cell wall teichoic acids of staphylococci. Arch Microbiol. (1983) 135:215–23. doi: 10.1007/BF00414483
48. Brown S, Santa Maria JP, Walker S. Wall teichoic acids of gram-positive bacteria. Annu Rev Microbiol. (2013) 67:313–36. doi: 10.1146/annurev-micro-092412-155620
49. Winstel V, Sanchez-Carballo P, Holst O, Xia G, Peschel A. Biosynthesis of the unique wall teichoic acid of Staphylococcus aureus lineage ST395. MBio. (2014) 5:e00869-14. doi: 10.1128/mBio.00869-14
50. Azam AH, Kadoi K, Miyanaga K, Usui M, Tamura Y, Cui L, et al. Analysis host-recognition mechanism of staphylococcal kayvirus ϕSA039 reveals a novel strategy that protects Staphylococcus aureus against infection by Staphylococcus pseudintermedius Siphoviridae phages. Appl Microbiol Biotechnol. (2019) 103:6809–23. doi: 10.1007/s00253-019-09940-7
51. Schleifer KH, Kandler O. Peptidoglycan types of bacterial cell walls and their taxonomic implications. Bacteriol Rev. (1972) 36:407–77.
52. Eugster MR, Loessner MJ. Wall teichoic acids restrict access of bacteriophage endolysin Ply118, Ply511, and PlyP40 cell wall binding domains to the Listeria monocytogenes peptidoglycan. J Bacteriol. (2012) 194:6498–506. doi: 10.1128/JB.00808-12
Keywords: antibiotic-resistant bacteria, bacteriophage, endolysin, canine skin infection, Staphylococcus pseudintermedius
Citation: Nakamura T, Kitana J, Fujiki J, Takase M, Iyori K, Simoike K and Iwano H (2020) Lytic Activity of Polyvalent Staphylococcal Bacteriophage PhiSA012 and Its Endolysin Lys-PhiSA012 Against Antibiotic-Resistant Staphylococcal Clinical Isolates From Canine Skin Infection Sites. Front. Med. 7:234. doi: 10.3389/fmed.2020.00234
Received: 08 January 2020; Accepted: 05 May 2020;
Published: 10 June 2020.
Edited by:
Fintan Thomas Moriarty, AO Research Institute, SwitzerlandReviewed by:
Nitya Singh, University of Florida, United StatesEric Thomas Sumrall, AO Research Institute, Switzerland
Copyright © 2020 Nakamura, Kitana, Fujiki, Takase, Iyori, Simoike and Iwano. This is an open-access article distributed under the terms of the Creative Commons Attribution License (CC BY). The use, distribution or reproduction in other forums is permitted, provided the original author(s) and the copyright owner(s) are credited and that the original publication in this journal is cited, in accordance with accepted academic practice. No use, distribution or reproduction is permitted which does not comply with these terms.
*Correspondence: Hidetomo Iwano, aC1pd2FubyYjeDAwMDQwO3Jha3Vuby5hYy5qcA==