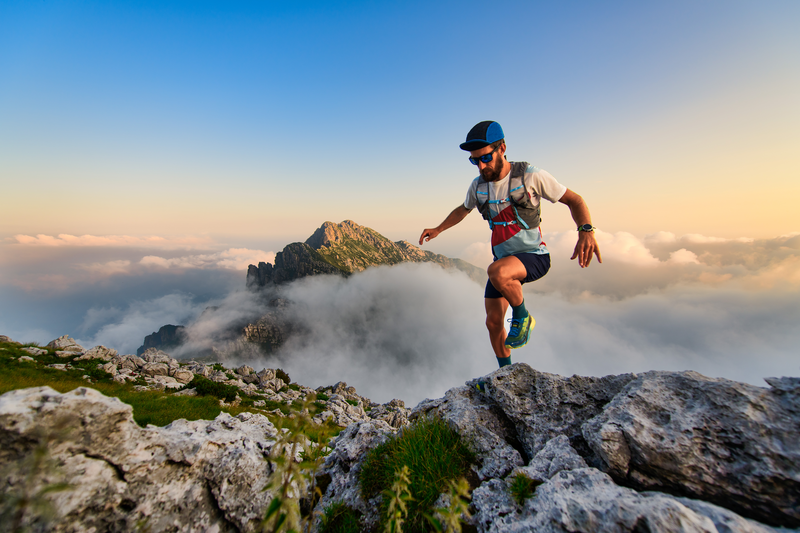
95% of researchers rate our articles as excellent or good
Learn more about the work of our research integrity team to safeguard the quality of each article we publish.
Find out more
SYSTEMATIC REVIEW article
Front. Med. , 02 April 2020
Sec. Regulatory Science
Volume 7 - 2020 | https://doi.org/10.3389/fmed.2020.00091
Regulatory T cells (Tregs) have a prominent role in the control of immune homeostasis. Pharmacological impact on their activity or balance with effector T cells could contribute to (impaired) clinical responses or adverse events. Monitoring treatment-related effects on T cell subsets may therefore be part of (pre-)clinical studies for medicinal products. However, the extent of immune monitoring performed in studies for marketing authorisation and the degree of correspondence with data available in the public domain is not known. We evaluated the presence of T cell immunomonitoring in 46 registration dossiers of monoclonal antibodies indicated for immune-related disorders and published scientific papers. We found that the depth of Treg analysis in registration dossiers was rather small. Nevertheless, data on treatment-related Treg effects are available in public academia-driven studies (post-registration) and suggest that Tregs may act as a biomarker for clinical responses. However, public data are fragmented and obtained with heterogeneity of experimental approaches from a diversity of species and tissues. To reveal the potential added value of T cell (and particular Treg) evaluation in (pre-)clinical studies, more cell-specific data should be acquired, at least for medicinal products with an immunomodulatory mechanism. Therefore, extensive analysis of T cell subset contribution to clinical responses and the relevance of treatment-induced changes in their levels is needed. Preferably, industry and academia should work together to obtain these data in a standardised manner and to enrich our knowledge about T cell activity in disease pathogenesis and therapies. This will ultimately elucidate the necessity of T cell subset monitoring in the therapeutic benefit-risk assessment.
The mammalian immune system is indispensable for the protection against a broad range of pathogens. For this, immune cells should be able to differentiate between (pathogenic) non-self and self. In addition, immune responses should be fine-tuned to demarcate the localisation and extent of an inflammatory reaction. Preservation of self-tolerance and immune homeostasis is mediated by various immunosuppressive mechanisms, including regulatory T cells (Tregs) (1). These suppressor cells appear to be specifically equipped to control the activation of other immune cells (2).
Human Tregs can be classified in different subtypes. The major subtype consists of the classical CD4+ Tregs that are either differentiated in the thymus (also known as natural Tregs) or peripherally induced from conventional (effector) CD4+ T cells (3, 4). Classical Tregs highly express CD25 [i.e., interleukin (IL)-2α receptor] and cytotoxic T lymphocyte-associated antigen-4 (CTLA-4) (5–7). These surface markers, together with the transcription factor forkhead box protein 3 (FoxP3), have essential roles in Treg-mediated suppressive functionality (8–12). Non-classical Tregs include FoxP3− Tr1 and T helper (Th)3 cells. These types are depending on IL-10 and tumour growth factor-β production for their suppressive activity (13, 14). Also γδ T cell and CD8+ T cell populations contain suppressive subsets, but their specific roles in regulating the immune system have yet to be identified (15–19).
The negative regulation of an immune response as mediated by Tregs is essential to prevent auto-immune and allergic disorders. On the other hand, this suppressive activity may prevent pathogen clearance during infections and hinder effective immune responses against (mutated) self-antigens in cancer (20). Therefore, in diseases where the balance between immune activation and suppression is skewed, Tregs could be attractive pharmacological targets (21, 22). For Th1- and Th17-dominated auto-immune disorders and Th2-dominated allergies, a therapy increasing Treg suppressive activity is sought (21, 23, 24). In contrast, for malignant diseases reversing an immunosuppressive tumour micro-environment by reducing Treg functionality would be the goal of treatment (21, 24–26). However, targeting Tregs in vivo is challenging, because a single (surface) marker with high specificity and selectivity for Tregs is still lacking (25). In addition, interfering with Treg numbers and/or functionality may also increase the risk for (auto-)immune-related adverse events (8). Examples are auto-immune enterocolitis and myocarditis following treatment with immune checkpoint inhibitors such as anti-CTLA-4 and anti-programmed cell death-1 (PD-1) (27–33). But also therapies against auto-immune disorders, for example tumour necrosis factor (TNF) inhibitors, have been reported to result in paradoxical immune-related inflammation (34).
Given the role of Tregs in (maintenance of) the immune balance, inclusion of these cells in the investigation of treatment effects on T cell subsets would be expected to be part of the (clinical) development program of medicinal products, at least for therapies targeting the immune system. Comprehensive overviews of immunomodulatory therapy-related effects on the balance between effector and regulatory T cells are available, for example for arthritis and solid organ transplantation (21, 35, 36). They show that general immunosuppressive drugs (such as corticosteroids), which target intracellular signalling pathways, do not only affect conventional T cell activation, but may also affect Treg activity. However, the sensitivity to the pathway-suppressive effects of these products differs between effector and regulatory T cells, and this difference determines whether immunomodulatory products will inhibit or stimulate immune cell activity. Differences in inhibition sensitivity of shared intracellular pathways are also apparent for more selective immunomodulating drug products. For example, blocking TNF has an effect on both TNF receptor-expressing effector T cells and Tregs, although it appears that positive clinical responses in several auto-immune disorders are the result of a greater inhibition of the effector than the regulatory cells (37).
Medicinal products may also disturb the balance between effector and regulatory T cells or the total T cell population more indirectly or even unintendedly (i.e., off-target effects). For example, monoclonal antibody (mAb)-mediated apoptosis results in the tumour tissue infiltration of immune cells, including Tregs. These Tregs can negatively influence the cytotoxic potential of effector cells, which could result in reduced efficacy. Therefore, immunomonitoring in (pre-)clinical studies is a useful tool to elucidate unintended treatment effects (and potential underlying mechanisms) caused by disturbance of the immune balance. In addition, immunomonitoring can provide more insight in the role of specific immune cells in the disease pathophysiology and thereby contribute to the identification of biomarkers predictive for the clinical response (38).
Given the potential clinical impact of Treg modulation, appropriate monitoring of treatment-induced effects on Treg frequency, phenotype and function would be required. We questioned whether Tregs have been investigated in (pre-)clinical studies to support a marketing authorisation application (MAA). Therefore, we surveyed if and when T cells, and Tregs in particular, were evaluated in these studies and whether the data in the registration dossiers corresponded to the available data in the public domain. There are multiple immunomodulatory therapies registered in the EU. We have chosen to restrict the sample size of registration dossiers to MAAs for approved mAb products based on the assumption that for mAbs immunomonitoring studies most frequently have been performed. After all, the majority is indicated for immune system-related disorders. In addition, we assessed T cell monitoring for a few tyrosine kinases inhibitors known to specifically target cytokine signalling pathways in T cells. We conclude this review with our perspective on the value of Treg monitoring and recommendations for their evaluation in (pre-)clinical studies.
We have evaluated the presence of data on T cell immunomonitoring (with the focus on Tregs) reported in published literature and in registration dossiers for MAA. We included all mAb products used as anti-neoplastic agents (anatomical therapeutic chemical classification code L01XC) or as selective immune inhibitor in the context of auto-immunity (L04AA, AB and AC) or asthma (R03DX), which have been EU-registered between 2006 and the first half year of 2019. Products that have been authorised and subsequently withdrawn in this time frame (for commercial, insufficient supply or unfavourable benefit-risk reasons) have been included. Biosimilars were excluded from our evaluation, because it was not expected that information on Tregs would be included in these dossiers (39). In total, 46 monoclonal antibodies were considered eligible.
As far as applicable, this study followed the recommendations of PRISMA in conducting and reporting a systemic review. Registration dossier search is summarised in Figure 1A, literature search is summarised in Figure 1B.
Figure 1. Flow chart of registration dossiers and literature reports selection process. (A) Registration dossier search and (B) literature search.
We searched in both registration dossiers [common technical documents, CTDs, required to apply for regulatory approval of a new medicinal product (40)] and PubMed literature for Treg-related keywords (including: regulatory T cell, suppressor T cell, Treg, FoxP3, CD25, mAb generic, and trade names). Because the Treg field is relatively new and the extent of T cell monitoring in dossiers of mAb products was not known, we decided to search more generally for lymphocyte and T cell populations in the CTDs, but with a focus on Tregs. Therefore, we also included keywords related to the whole T cell population (such as: lymphocyte, T cell, CD4, and CD8). When lymphocytes or T cells were mentioned in an individual study report, we also searched with the Treg-related keywords. Our main focus was on CD4+ FoxP3+ T cells, because these are the major Tregs in the immune system. However, other (non-classical) suppressor T cells—when mentioned in reports—were also taken along.
To explore lymphocyte and T cell immunomonitoring in registration dossiers, we searched the pre-clinical and clinical sections of the CTD (pre-clinical module 4 and clinical module 5, respectively) for each individual monoclonal antibody. Most study reports containing immunomonitoring results were found in the sections about pre-clinical pharmacology (module 4.2.1), toxicology (module 4.2.3), clinical pharmacokinetics (module 5.3.3), efficacy and safety (module 5.3.5).
We investigated whether potential mAb treatment-related effects on Tregs reported in published studies are also reported as individual studies presented in registration dossiers. Table 1 describes the immunomonitoring parameters used to determine potential effects of mAb products on lymphocytes, T cell subsets or specifically Tregs in registration dossier reports. In this table, no individual mAb products have been indicated for confidentiality reasons. The effects of the individual mAb products on Treg frequency, phenotype, and function as found in literature are described in Tables 2–4. Table 5 represents all mAb products for which no scientific literature was publicly available. Product-related effects were measured either pre-clinically (in vitro or in vivo in animal models) or clinically (human healthy donors and patients), the latter further subdivided in systemic (in peripheral blood) and local (at the tumour site or in inflamed tissue) effects. Potential (absence of) associations between clinical results and the presence or activity of Tregs prior to or during/after treatment are included.
To gain better insight in the type of products for which T cell monitoring was available, we divided the mAb products in three groups, based on the relevance of the product's pharmacological target for T cell (subset) function and survival:
1. mAb target is highly relevant for Tregs, i.e., the target is constitutively expressed on most Tregs (e.g., CTLA-4);
2. mAb target is -expected to be- relevant for the T cell population, i.e., the target is expressed on specific T cell and Treg subsets (e.g., α4 integrins) or the mAb product has a more indirect effect on Treg activity, when the target is involved in the balance between T cell subsets (e.g., IL-6 and IL-17A pathways);
3. mAb target is not directly relevant for T cells, i.e., the target is not involved in T cell functionality (e.g., CD20, which is expressed on B cells).
For the majority of registration dossiers of mAbs targeting Tregs or other T cell subsets, only absolute and relative counts of lymphocytes, lymphocyte subsets (i.e., T and B cells, in some cases also natural killer cells) or T cell subsets (CD4+ and CD8+) were determined clinically and pre-clinically (Table 1). T cell functionality testing (i.e., proliferative or cytotoxic capacity) was limited to pre-clinical studies, whereas further differentiation of T cell subsets (such as naïve/memory state) and determination of the CD4+ to CD8+ ratio was primarily found in clinical reports. Most data were derived from samples evaluated via clinical haematology, flow cytometry or immunohistochemistry. In most cases, however, no summarising data or concluding remarks (such as clinical significance) concerning the treatment effects on T cell frequency and functionality were provided.
Seven mAb products were classified as affecting targets (here: cell surface receptors) essential for Treg function or survival. In literature, treatment-related effects on frequency and phenotype (other than identity markers such as CD25 and FoxP3) were studied for all these mAb products, whereas effects on suppressive function were evaluated for four mAbs (Table 2). Nevertheless, high variability between mAb products existed in the number of available studies (most on products targeting CTLA-4 and PD-1) and the level of Treg analysis per study. For the majority of the publications, identification of Tregs within the T cell population was based on several markers (mainly a combination of CD25, CD127, and/or FoxP3) to exclude activated effector T cells as much as possible. In four of the seven mAb registration dossiers, effects on the frequency of Tregs (defined as CD4+/CD8+ CD25+ FoxP3+ in most studies) were taken into account (Table 1), although actual results were not always reported. Next to frequency, Treg functionality is an important determinant of the degree of immune suppression and thus requires evaluation. But in none of the dossiers Treg functionality was determined.
In both publications and registration dossier studies, mAb-related effects on Tregs were found. However, comparing these sources elucidated a clear discrepancy. For most mAb products, the public domain contained more studies and within these studies, Tregs were analysed more extensively than in registration dossiers. For example, activation markers (such as Ki67 and CD69), phenotypic and functionality-related markers (e.g., Helios, CD39, PD-1, CTLA-4, cytokines such as IL-10 and the combination of CD45RA− with FoxP3++) or actual suppressive activity were monitored for all mAb products in published studies, except cemiplimab. In contrast, in only one registration dossier, two of such markers (i.e., CTLA-4 and CCR4, thus directly related to the mAb target) were taken into account. Analysis of surface markers next to standard Treg identity indicators could be a useful surrogate for Treg activity when actual functionality assays cannot be performed [see section Recommendations for (Pre-)clinical Studies].
In several public studies, Treg (subset) frequency could be used to differentiate responders from non-responders or to predict the clinical response prior to treatment. A decrease in local or systemic Treg frequency was associated with a better (anti-tumour) treatment response (52, 55–59). A high Treg frequency at baseline was associated with either better or worse clinical outcome, depending on the evaluated Treg phenotype (60, 61, 87–89). These studies indicate that Tregs may be assigned as potential biomarker for disease activity or clinical outcome [see section Value of Treg Monitoring]. Because Treg data would be available much quicker than, for example, long-term clinical responses such as progression-free survival, it could be worthwhile to investigate applicability of such biomarker in product-specific studies (as surrogate clinical end point) (144). Nevertheless, this probably requires more (pre-)clinical experience than available at the time of MAA.
Most evaluated mAb products [i.e., (28)] were designed to target cell surface receptors or cytokines that have a role in the physiology of T cells. All targets (or their receptors) were known to be expressed on Treg subsets or were earlier defined as involved in maintaining the delicate balance between effector and regulatory T cells.
In general, the number of published studies (Table 3) was related to the level of Treg analysis in the registration dossiers (Table 1), except for Treg functionality. However, where Treg identification in literature was based on a combination of several phenotypic markers (e.g., CD25 and CD127 next to CD4), most dossier reports defined Tregs solely on one marker (if defined at all). Therefore, the latter may have measured therapeutic effects on a mix of Tregs and activated effector T cells, which interferes with correct interpretation of the data [see section Recommendations for (Pre-)clinical Studies]. It was also noted that changes in Treg phenotype (e.g., activation) were analysed more in-depth in public literature compared to registration dossiers.
Eleven mAb products were not expected to directly impact T cell function or survival and targets were therefore considered “non-relevant”. Published studies for mAb products targeting non-relevant molecules did indeed not report any Treg monitoring, except for belimumab and trastuzumab (Table 4). Belimumab targets the B lymphocyte stimulator (BLyS) protein, thereby blocking the activation of cells bearing the BLyS receptor. Target cells are primarily B cells, but also T follicular helper cells, which produce IL-21. Belimumab appears to reduce IL-21 production and subsequently restores Treg development at the expense of Th17 expansion (134).
Trastuzumab is indicated for Her2+ breast cancer and does not directly target the immune system. Nevertheless, Treg frequency and phenotype and their association with clinical outcome were evaluated both in human patients and in mice. One reason for assessing Tregs in breast cancer patients and the effect of trastuzumab on these cells may be that disease progression appears to be related to tumour-associated immunosuppression and FoxP3+ cell infiltration (135, 145–147). Indirect effects of the mAb on the balance between pro- and anti-inflammatory immune cells could therefore contribute to a more effective anti-tumour response.
For mAbs with a target outside the T cell population, still the number of the total T population or CD4+ and CD8+ cells were monitored, although no Treg monitoring was performed (Table 1), which is in line with the published reports on these products.
Taken together, we found that the depth of Treg (and T cell subset) immunomonitoring differs between products, depending on the likeliness that the mAb affects T cell functionality or survival. In addition, the extent of Treg evaluation varies between registration dossiers and published studies for individual mAbs. This is most probably because the majority of literature studies were academia-driven and were published only after marketing authorisation. Nevertheless, the involvement of the company in approximately half of these studies reveals that collaboration between industry and academia contributes to increased insight in treatment-related effects on the immune system.
We took a pragmatic approach by evaluating mAb products EU-registered in a time period of 13 years without selecting for products that were actually meant to modulate the immune system. Only seven (of the 46 evaluated) products targeted molecules with high relevance for Treg function and survival. To determine whether other drug products with a Treg-relevant target took these suppressor cells into account in (pre-)clinical studies, we investigating two recently EU-registered Janus kinase (JAK) inhibitors indicated for rheumatoid arthritis. The JAK/STAT (i.e., signal transducer and activator of transcription) pathway is known to play an important role in the activation and survival of immune cells (148). Especially STAT5, a downstream target of the IL-2 receptor, is crucial for FoxP3 induction and Treg differentiation in the thymus (149, 150).
In both registration dossiers, effects of JAK inhibitors on lymphocyte and T cell subsets (cell count, phenotypic markers, cytokine production, and STAT phosphorylation) were determined, both clinically and pre-clinically. Clinically, also effects on Tregs (i.e., frequency) were investigated and a potential association between Tregs and the clinical response was explored. However, the amount of data presented in published studies (i.e., on local and systemic Treg frequency and functionality in mice and men, but also in vitro) was much more extensive than present in the dossier reports, although for one of the JAK inhibitors only one literature study was available (151–158).
Some limitations in our study need mentioning. First, we specifically selected EU-authorised mAb products (although not restricted to their registered indications). Therapies that did not reach the market or were still under review were not evaluated, although some of these products may target Tregs and thus dossiers could contain valuable information [e.g., isatuximab (159)]. In addition, we acknowledge that several mAb products with direct immune-related or even T cell-related targets are in the late-stage pipeline of several companies (160). Future MAAs containing data on Treg frequency or even functionality may thus be expected.
Second, mAb products authorised before 2006 were excluded, because the main increase in attention for and knowledge about Tregs occurred in the last decade. For several of these older immunomodulatory mAb products with a direct impact on the T cell population (e.g., infliximab, adalimumab, alemtuzumab, daclizumab), recently published immunomonitoring studies involved Treg frequency and functionality, because of their importance in the disease pathophysiology and to (further) elucidate the pharmacological mechanisms of action in lack-of-response issues or for biomarker definition (161, 162). Tregs are also monitored for old mAbs targeting non-T cell receptors, such as CD20 (rituximab) (163, 164). Above-mentioned studies investigating effects of recently authorised products or relatively old products on the immune system could contribute to our knowledge of T cell subsets.
Third, mAb products indicated for infections (e.g., caused by Clostridium difficile or HIV) or immune-related diseases with a distinct pathophysiology (such as paroxysmal nocturnal haemoglobinuria) were also excluded. Only a few mAb products are registered for these indications and it is expected that data concerning Tregs in existing dossiers will be limited.
Fourth, for literature reports, we limited our search to the same products as described for MAA dossiers and thus excluding studies with non-registered human or “mousenised” mAbs against the same target. We acknowledge that these excluded studies would be helpful when more insight in efficacy or safety-related effects of mAb products on specific immune cells would be required. Nevertheless, to establish the relevance of experience with such non-registered products, interpretation of the published data would be needed, which was not the aim of our study.
Tregs have a crucial function in regulating immune responses to dampen inflammation, limit tissue damage and prevent auto-reactivity. Pharmacological impact on their number and/or (local) activity, either directly or indirectly, is likely to contribute to (or impair) clinical responses or to adverse events. Therefore, monitoring effects of immunomodulatory products on T cells -including Tregs- should be part of (pre-)clinical studies.
In addition, Tregs or specific Treg subsets may turn out to be predictive biomarkers for specific diseases or patient populations. We noted that in the majority of mAb product dossiers, no clinical relevance was estimated for treatment-induced changes in Treg frequency or phenotype. For only two products, an association was determined between Treg frequency and the clinical response. Published reports, on the other hand, frequently mentioned associations between the amount of (local) Tregs and clinical outcome. Thereby, Tregs could act as biomarker for the clinical response (144). Associations with the baseline Treg level prior to treatment may be used as prognostic biomarker, for example to select patients eligible for mAb (anti-tumour) therapy. Changes in Treg level following treatment may act as predictive marker of the mAb-mediated clinical response, in auto-immune as well as in neoplastic indications. Nonetheless, interpretation of clinical associations and treatment-related Treg effects is still rather difficult. For example, differentiation between effects that are a direct consequence of the medicinal product activity or a result of disease remission is usually not accomplished. In addition, the clinical significance of fluctuations in Tregs during or after therapy remains to be established.
In general, immunomonitoring has a substantial value to assess the effectiveness and safety of therapeutic interventions and to select patients eligible to these treatments (2, 165). On the level of T cell subsets, scientific knowledge regarding immune responses is growing exponentially (also for older immunomodulatory treatments) and this knowledge should be taken into account when selecting for specific immunomonitoring parameters. But we consider that the general added value of measuring Tregs in (pre-)clinical studies is not yet sufficiently clear and their contribution to the clinical response requires more extensive analysis. This would include gathering information regarding the Treg role in disease pathophysiology and therapy-related adverse events. Regulatory authorities need this information to estimate the value of Tregs and the necessity to take treatment-related Treg changes along in the benefit-risk assessment. Tregs should therefore be taken into account as exploratory parameter in (pre-)clinical studies, either prior to MAA or post-registration in collaboration with academia.
Despite growing knowledge regarding treatment effects on Tregs, we observed a high variability in data between the different studies, probably due to heterogeneity of the experimental approach. Studies differed in markers used to identify Tregs, in methods to measure their functionality and in assay read-out techniques. Also tissues and species used to monitor Tregs, the time between treatment and analysis points varied between studies. Moreover, the treatment protocol (e.g., administration route, number and quantity of doses and dose intervals, concomitant therapies), therapeutic indication and the number of patients also added to study heterogeneity. D'Arena et al. ran into the same problem of heterogeneity when evaluating the relevance of Tregs as biomarker in the context of hematologic malignancies. Their study also exemplified “the need for more standardised approaches in the study of Tregs” (166). Thus, harmonisation of Treg identification and monitoring is required before these cells can become actual endpoints in clinical investigations or can be used as prognostic or predictive biomarker (25, 144).
Apart from this lack of harmonisation, the scientific knowledge is too limited to demand or guide Treg monitoring in registration dossiers. Nevertheless, we hereby stimulate companies (and academia) to take these cells into account in their investigations or to collaborate with academia to perform T cell subset-specific studies (post-registration).
We will end this review with some specific points-to-consider for Treg (and other T cell subset) monitoring in pre-clinical and clinical studies.
Treg monitoring (both clinically and pre-clinically) could be restricted to products with a target known to play a vital role in T cell development, differentiation, functionality, or survival. Especially when the target is related to regulation of the immune system and loss-of-function would considerably increase the risk of auto-immunity [e.g., CTLA-4 expression on Tregs (27)], monitoring the frequency and functionality of immune cells closely related to this target may significantly add to the identification of potential safety concerns early in product development. Obviously, for products containing (ex vivo expanded) Tregs or for therapies typically aiming to enhance Treg activity (e.g., tolerogenic dendritic cells), analysis of Treg frequency and/or function will be imperative (24). For immunomodulatory treatments with a target in the non-T cell compartment, a risk-based approach could identify whether monitoring of T cell subset responses would be required to substantiate clinical data.
We suggest to add several Treg-related markers to an existing immune monitoring panel (see below in subsection Identifying Tregs). When this would not be feasible, one could retain clinical samples to be able to retrospectively measure effects on specific T cell or Treg subsets when required [as recently performed for unexpected events with nivolumab (167–169)]. More “standard” Treg monitoring could then be restricted to pre-clinical investigation.
What samples would be most appropriate? In general, in humans peripheral blood is the most accessible compartment for multiple analyses over time. Nevertheless, changes in circulating T cell subsets may not accurately reflect the local environment. Furthermore, it has been reported that the ratio and phenotype of Treg subsets at tumour sites differ substantially from peripheral blood (8, 9, 25, 132, 170). Therefore, when feasible, treatment effects on local T cell subsets may be taken into account as well (26).
We noted that pre-clinical in vitro pharmacologic studies are frequently performed with cells from healthy donors. This can be acceptable, but using cells from patients may have added value when the disease has impaired the intrinsic function of the cells. For example, patients with giant cell arteritis can have a defect in their FoxP3 protein, which affects the suppressive capacity of the Tregs, but could be pharmacologically corrected (114).
In general, treatment-related effects on the immune system are dose-dependent and difficult to predict. For example, lymphocyte-depleting approaches (such as anti-thymocyte globulin) do not simply deplete all T cells, but also act as immunosuppressant by, for example, converting effector into regulatory T cells and by preserving or even expanding already existing Tregs. An increased Treg to conventional T cell ratio may therefore be an unexpected effect of T cell-depleting antibodies (21, 36, 171, 172). This indicates that monitoring drug-mediated effects on the whole T cell population may not correctly predict effects on T cell subsets. Therefore, these analyses should preferably discriminate between effector and regulatory T cells, at least for products indicated to specifically target T cells.
Accurately defining Tregs is, however, a challenge. Although there are several useful reviews available that highlight different markers and cytokines that may help identifying Tregs, there is no unique Treg marker (7, 22, 24, 173–175). Expression of FoxP3, the master regulator of classical CD4+ Tregs, is not limited to human regulatory cells: effector T cells transiently upregulate FoxP3 expression after activation and also other immune cells and even tumour cells may express this transcription factor (176–181). In addition, not all regulatory T cell subtypes express FoxP3. Moreover, FoxP3 cannot be used to isolate Tregs alive for ex vivo functionality testing. Combined use of several (surface) markers will therefore be needed to identify and purify Tregs. In contrast to murine CD4+ CD25+ regulatory T cells, only CD4+ cells with a high level of CD25 expression have a suppressive capacity in humans. The other CD4+ CD25+ T cells are activated effector T cells. According to the vision of several experts in the field, CD3, CD4, CD25, CD127, and FoxP3 are the minimally required markers to define human Treg cells in flow cytometric samples and addition of Ki67 and CD45RA/RO could provide information on the activation status of Tregs (173) or improve selection of pure Treg fractions (182). Such Treg panel would also allow for monitoring of different effector T cell subsets (naïve/memory state of both CD4+ and CD8+ T cells). The experts also emphasised that a proper flow cytometric gating strategy will improve the reliability and purity of the defined Treg population, and in the meantime diminish inter-assay variability (173, 183). Recently, Pitoiset et al. provided a standardised protocol to monitor Tregs in multicentre clinical trials, using above-mentioned markers (184).
One should, however, keep in mind that there are phenotypic differences between circulating Tregs and Tregs at sites of inflammation (173). A more precise discrimination of Treg subtypes may thus be needed, especially in the peripherally-induced (heterogeneous) population (25, 185). And differentiating between naïve and activated Treg subsets may be needed to find treatment-related effects or clinical associations (186, 187). Nevertheless, distinguishing between Treg subsets (especially thymus- vs. periphery-derived cells) is rather challenging (3–5, 188–190). Measuring the amount of demethylation of the FoxP3 gene may provide insight in the stability of FoxP3 expression and thereby distinguish thymus-derived Tregs from peripherally-induced Tregs and activated conventional T cells (191, 192). Nevertheless, this demethylation status analysis requires a highly pure lymphocyte sample.
There are various methods to analyse the suppressive capacity of Tregs. Most of these assays aim to measure inhibition of effector T cell proliferation or cytokine production, although cytotoxicity inhibition may also be used as read-out (193, 194). The requirement of a rather large amount of autologous cells for such co-cultures would make these types of assays less suitable for patient samples (195). Indeed, we found in several registration dossiers that such testing was considered clinically, but impossible to perform. In addition, the in vivo functionality may be impacted by the tissue environment, which is difficult to mimic in vitro (26). Moreover, impaired in vivo suppressive function is not always reflected by results from an in vitro assay (20).
Treg functionality was only (pre-clinically) analysed in one of the 46 mAb dossiers evaluated. Lack of functionality testing is probably the result of difficulties with assay design. Nevertheless, there are literature examples where Treg functionality testing appeared possible (62). We therefore would like to draw attention to different approaches that may enhance the possibility of Treg function analysis. Instead of using autologous cells, a mixed lymphocyte reaction may be considered (196, 197). In addition, to prevent long co-culture periods, a surrogate read-out (i.e., inhibition of activation marker expression instead of proliferation) could be used (198–200). Identification of functional Tregs via marker gene analysis (e.g., FoxP3, CTLA-4, and IL-10) may also be a simple and quick method, although the level of mRNA expression does not necessarily reflect protein expression and this read-out is also considered surrogate for Treg functionality (201). Simply distinguishing between resting and activated Tregs and effector T cells can also provide information about the presence or absence of suppressive T cells in a sample (182, 187). More considerations and technical challenges for Treg functionality assays can be found in the public domain (25, 115, 183, 194, 202–204).
We are now starting to understand the role of different T cell subsets in disease pathogenesis and immunotherapeutic mechanisms of action. This provides the opportunity to selectively target specific subpopulations rather than a whole T cell population to improve the effectiveness and safety of immunomodulatory therapies. In addition, monitoring the activation status, function and amount of specific T cell subsets could assist in identifying the patients that would most likely benefit from therapy (2). A risk-based approach is considered helpful to select products that would require T cell subset monitoring to more reliably assess the product's benefits and risks.
Immunomonitoring, as proposed in this review, will also help to enrich our knowledge about Tregs and their association with the clinical response. This will, however, require accurate phenotypic identification of regulatory subsets and further investigation of the clinical relevance of treatment-induced changes in their levels. To obtain and report such information in a systematic way, a collaboration between industry and academia will be required (205).
We believe that there are still many issues to address before Tregs can be used as biomarkers for targeted therapies, but gathering knowledge about Treg subpopulations in health and disease will eventually shed more light on the (pre-)clinical value of these regulatory cells. This will ultimately result in more concrete regulatory guidance for T cell (and particularly Treg) monitoring in studies used for marketing authorisation.
The datasets generated for this study will not be made publicly available because they contain confidential information from registration dossiers. Requests to access the datasets should be directed tobWguaG9lZm5hZ2VsQGNiZy1tZWIubmw=.
AW collected the data and wrote the first draft of the manuscript. AW and MH were involved in the interpretation of the data. All authors contributed to the conception and design of the work, manuscript revision, read, and approved the final version.
The contents of the article represent the authors' personal opinion and do not necessarily reflect any position of the Dutch Medicines Evaluation Board.
The authors declare that the research was conducted in the absence of any commercial or financial relationships that could be construed as a potential conflict of interest.
We thank Tineke van den Hoorn, Jan-Willem van der Laan, and Liesbeth Rook for their helpful comments on the article. Simone van den Bosch, Job van Bragt, and Mitra Dwarkasing, performing their research internship, are acknowledged for their substantial contribution to the dossier search work.
1. Sakaguchi S, Sakaguchi N, Asano M, Itoh M, Toda M. Immunologic self-tolerance maintained by activated T cells expressing IL-2 receptor a-chains (CD25): breakdown of a single mechanism of self-tolerance causes various autoimmune diseases. J Immunol. (1995) 155:1151–64.
2. Whiteside TL, Gulley JL, Clay TM, Tsang KY. Immunologic monitoring of cellular immune responses in cancer vaccine therapy. J Biomed Biotechnol. (2011) 2011:370374. doi: 10.1155/2011/370374
3. Lee HM, Bautista JL, Hsieh CS. Thymic and peripheral differentiation of regulatory T Cells. Adv Immunol. (2011) 112:25–71. doi: 10.1016/B978-0-12-387827-4.00002-4
4. Bluestone JA, Abbas AK. Natural versus adaptive regulatory T cells. Nat Rev Immunol. (2003) 3:253–7. doi: 10.1038/nri1032
5. Schmitt EG, Williams CB. Generation and function of induced regulatory T cells. Front Immunol. (2013) 4:152. doi: 10.3389/fimmu.2013.00152
6. Ohkura N, Kitagawa Y, Sakaguchi S. Development and maintenance of regulatory T cells. Immunity. (2013) 38:414–23. doi: 10.1016/j.immuni.2013.03.002
7. Chen X, Oppenheim JJ. Resolving the identity myth: key markers of functional CD4+FoxP3+regulatory T cells. Int Immunopharmacol. (2011) 11:1489–96. doi: 10.1016/j.intimp.2011.05.018
8. Tanaka A, Sakaguchi S. Regulatory T cells in cancer immunotherapy. Cell Res. (2017) 27:109–18. doi: 10.1038/cr.2016.151
9. Takeuchi Y, Nishikawa H. Roles of regulatory T cells in cancer immunity. Int Immunol. (2016) 28:401–9. doi: 10.1093/intimm/dxw025
10. Zheng Y, Rudensky AY. Foxp3 in control of the regulatory T cell lineage. Nat Immunol. (2007) 8:457–62. doi: 10.1038/ni1455
11. Hori S, Nomura T, Sakaguchi S. Control of regulatory T cell development by the transcription factor Foxp3. Science. (2003) 299:1057–61. doi: 10.1126/science.1079490
12. Fontenot JD, Gavin MA, Rudensky AY. Foxp3 programs the development and function of CD4+CD25+regulatory T cells. Nat Immunol. (2003) 4:330–6. doi: 10.1038/ni904
13. Roncarolo MG, Gregori S, Battaglia M, Bacchetta R, Fleischhauer K, Levings MK. Interleukin-10-secreting type 1 regulatory T cells in rodents and humans. Immunol Rev. (2006) 212:28–50. doi: 10.1111/j.0105-2896.2006.00420.x
14. Weiner HL. Induction and mechanism of action of transforming growth factor-ß-secreting Th3 regulatory cells. Immunol Rev. (2001) 182:207–14. doi: 10.1034/j.1600-065X.2001.1820117.x
15. Peters C, Kabelitz D, Wesch D. Regulatory functions of γδ T cells. Cell Mol Life Sci. (2018) 75:2125–35. doi: 10.1007/s00018-018-2788-x
16. Siegmund K, Rückert B, Ouaked N, Bürgler S, Speiser A, Akdis CA, et al. Unique phenotype of human tonsillar and in vitro-induced FOXP3 +CD8+ T cells. J Immunol. (2009) 182:2124–30. doi: 10.4049/jimmunol.0802271
17. Najafian N, Chitnis T, Salama AD, Zhu B, Benou C, Yuan X, et al. Regulatory functions of CD8+CD28-T cells in an autoimmune disease model. J Clin Invest. (2003) 112:1037–48. doi: 10.1172/JCI17935
18. Hayday A, Tigelaar R. Immunoregulation in the tissues by γδ T cells. Nat Rev Immunol. (2003) 3:233–42. doi: 10.1038/nri1030
19. Seo N, Tokura Y, Takigawa M, Egawa K. Depletion of IL-10- and TGF-β-producing regulatory γδ T cells by administering a daunomycin-conjugated specific monoclonal antibody in early tumor lesions augments the activity of CTLs and NK cells. J Immunol. (1999) 163:242–9.
20. Plitas G, Rudensky AY. Regulatory T cells: differentiation and function. Cancer Immunol Res. (2016) 4:721–5. doi: 10.1158/2326-6066.CIR-16-0193
21. Furukawa A, Wisel SA, Tang Q. Impact of immune-modulatory drugs on regulatory T cell. Transplantation. (2016) 100:2288–300. doi: 10.1097/TP.0000000000001379
22. Rakebrandt N, Littringer K, Joller N. Regulatory T cells: balancing protection versus pathology. Swiss Med Wkly. (2016) 146:w14343. doi: 10.4414/smw.2016.14343
23. Palomares O, Akdis M, Martín-Fontecha M, Akdis CA. Mechanisms of immune regulation in allergic diseases: the role of regulatory T and B cells. Immunol Rev. (2017) 278:219–36. doi: 10.1111/imr.12555
24. Perdigoto AL, Chatenoud L, Bluestone JA, Herold KC. Inducing and administering tregs to treat human disease. Front Immunol. (2016) 6:654. doi: 10.3389/fimmu.2015.00654
25. Whiteside TL. The role of regulatory T cells in cancer immunology. Immunotargets Ther. (2015) 4:159–71. doi: 10.2147/ITT.S55415
26. Jacobs JFM, Nierkens S, Figdor CG, de Vries IJM, Adema GJ. Regulatory T cells in melanoma: The final hurdle towards effective immunotherapy? Lancet Oncol. (2012) 13:e32–42. doi: 10.1016/S1470-2045(11)70155-3
27. Carbonnel F, Soularue E, Coutzac C, Chaput N, Mateus C, Lepage P, et al. Inflammatory bowel disease and cancer response due to anti-CTLA-4: is it in the flora? Semin Immunopathol. (2017) 39:327–31. doi: 10.1007/s00281-016-0613-x
28. Läubli H, Balmelli C, Bossard M, Pfister O, Glatz K, Zippelius A. Acute heart failure due to autoimmune myocarditis under pembrolizumab treatment for metastatic melanoma. J Immunother Cancer. (2015) 3:11. doi: 10.1186/s40425-015-0057-1
29. Kong YC, Flynn JC. Opportunistic autoimmune disorders potentiated by immune-checkpoint inhibitors anti-CTLA-4 and anti-PD-1. Front Immunol. (2014) 5:206. doi: 10.3389/fimmu.2014.00206
30. Nancey S, Boschetti G, Cotte E, Ruel K, Almeras T, Chauvenet M, et al. Blockade of cytotoxic T-lymphocyte antigen-4 by ipilimumab is associated with a profound long-lasting depletion of Foxp3+regulatory T cells: a mechanistic explanation for ipilimumab-induced severe enterocolitis? Inflamm Bowel Dis. (2012) 18:E1598–600. doi: 10.1002/ibd.21927
31. Wolchok JD, Neyns B, Linette G, Negrier S, Lutzky J, Thomas L, et al. Ipilimumab monotherapy in patients with pretreated advanced melanoma: a randomised, double-blind, multicentre, phase 2, dose-ranging study. Lancet Oncol. (2010) 11:155–64. doi: 10.1016/S1470-2045(09)70334-1
32. Attia P, Phan GQ, Maker AV, Robinson MR, Quezado MM, Yang JC, et al. Autoimmunity correlates with tumor regression in patients with metastatic melanoma treated with anti-cytotoxic T-lymphocyte antigen-4. J Clin Oncol. (2005) 23:6043–53. doi: 10.1200/JCO.2005.06.205
33. Phan GQ, Yang JC, Sherry RM, Hwu P, Topalian SL, Schwartzentruber DJ, et al. Cancer regression and autoimmunity induced by cytotoxic T lymphocyte-associated antigen 4 blockade in patients with metastatic melanoma. Proc Natl Acad Sci USA. (2003) 100:8372–7. doi: 10.1073/pnas.1533209100
34. Fiorino G, Danese S, Pariente B, Allez M. Paradoxical immune-mediated inflammation in inflammatory bowel disease patients receiving anti-TNF-a agents. Autoimmun Rev. (2014) 13:15–9. doi: 10.1016/j.autrev.2013.06.005
35. Mijnheer G, Prakken BJ, Van Wijk F. The effect of autoimmune arthritis treatment strategies on regulatory T-cell dynamics. Curr Opin Rheumatol. (2013) 25:260–7. doi: 10.1097/BOR.0b013e32835d0ee4
36. Demirkiran A, Hendrikx TK, Baan CC, Van Der Laan LJW. Impact of immunosuppressive drugs on CD4+CD25+FOXP3+ regulatory T cells: does in vitro evidence translate to the clinical setting? Transplantation. (2008) 85:783–9. doi: 10.1097/TP.0b013e318166910b
37. Chen X, Oppenheim JJ. The phenotypic and functional consequences of tumour necrosis factor receptor type 2 expression on CD4+FoxP3+ regulatory T cells. Immunology. (2011) 133:426–33. doi: 10.1111/j.1365-2567.2011.03460.x
38. Teniente-Serra A, Ramo-Tello C, Martinez-Caceres EM. Immunomonitoring lymphocyte subpopulations in multiple sclerosis patients. In: Zagon IS, McLaughlin PJ, editors. Multiple Sclerosis: Perspectives in Treatment and Pathogenesis. Brisbane, QLD: (AU) Codon Publications (2017). p. 139–54. doi: 10.15586/codon.multiplesclerosis.2017.ch9
39. EMA/CHMP/BMWP/403543/2010. Guideline on Similar Biological Medicinal Products Containing Monoclonal Antibodies – Non-Clinical and Clinical Issues. (2012). Available online at: https://www.ema.europa.eu/en/documents/scientific-guideline/guideline-similar-biological-medicinal-products-containing-monoclonal-antibodies-non-clinical_en.pdf (assessed November 1, 2019).
40. Jordan D. An overview of the Common Technical Document (CTD) regulatory dossier. Med Writing. (2014) 23:101–5. doi: 10.1179/2047480614Z.000000000207
41. Doi T, Muro K, Ishii H, Kato T, Tsushima T, Takenoyama M, et al. A Phase I study of the Anti-CC chemokine receptor 4 antibody, mogamulizumab, in combination with nivolumab in patients with advanced or metastatic solid tumors. Clin Cancer Res. (2019) 25:6614–22. doi: 10.1158/1078-0432.CCR-19-1090
42. Sato T, Coler-Reilly ALG, Yagishita N, Araya N, Inoue E, Furuta R, et al. Mogamulizumab (Anti-CCR4) in HTLV-1-associated myelopathy. N Engl J Med. (2018) 378:529–38. doi: 10.1056/NEJMoa1704827
43. Ureshino H, Shindo T, Nishikawa H, Watanabe N, Watanabe E, Satoh N, et al. Effector regulatory T cells reflect the equilibrium between antitumor immunity and autoimmunity in adult T-cell leukemia. Cancer Immunol Res. (2016) 4:644–9. doi: 10.1158/2326-6066.CIR-15-0303
44. Sugata K, Yasunaga J, Miura M, Akari H, Utsunomiya A, Nosaka K, et al. Enhancement of anti-STLV-1/HTLV-1 immune responses through multimodal effects of anti-CCR4 antibody. Sci Rep. (2016) 6:27150. doi: 10.1038/srep27150
45. Tatsuno K, Sano T, Fukuchi K, Kuriyama S, Aoshima M, Kasuya A, et al. Emergence of photosensitivity with decreased treg cells in a patient with mycosis fungoides treated with anti-cc chemokine receptor 4 antibody mogamulizumab. Acta Derm Venereol. (2016) 96:420–1. doi: 10.2340/00015555-2257
46. Ni X, Jorgensen JL, Goswami M, Challagundla P, Decker WK, Kim YH, et al. Reduction of regulatory T cells by mogamulizumab, a defucosylated Anti-CC chemokine receptor 4 antibody, in patients with aggressive/refractory mycosis fungoides and sézary syndrome. Clin Cancer Res. (2015) 21:274–85. doi: 10.1158/1078-0432.CCR-14-0830
47. Kurose K, Ohue Y, Wada H, Iida S, Ishida T, Kojima T, et al. Phase Ia study of FoxP3+ CD4 Treg depletion by infusion of a humanized anti-CCR4 antibody, KW-0761, in cancer patients. Clin Cancer Res. (2015) 21:4327–36. doi: 10.1158/1078-0432.CCR-15-0357
48. Hashimoto A, Iyama S, Sato T, Fujimi A, Ono K, Horiguchi H, et al. A case of associated refractory acute graft-versus-host disease following umbilical cord blood transplantation in an adult T-cell leukemia/lymphoma patient pretreated with mogamulizumab. J Hematop Cell Transpl. (2015) 4:52–6. doi: 10.7889/hct.4.52
49. Ogura M, Ishida T, Hatake K, Taniwaki M, Ando K, Tobinai K, et al. Multicenter phase II study of mogamulizumab (KW-0761), a defucosylated anti-cc chemokine receptor 4 antibody, in patients with relapsed peripheral T-cell lymphoma and cutaneous T-cell lymphoma. J Clin Oncol. (2014) 32:1157–63. doi: 10.1200/JCO.2013.52.0924
50. Sugiyama D, Nishikawa H, Maeda Y, Nishioka M, Tanemura A, Katayama I, et al. Anti-CCR4 mAb selectively depletes effector-Type FoxP3+CD4+ regulatory T cells, evoking antitumor immune responses in humans. Proc Natl Acad Sci USA. (2013) 110:17945–50. doi: 10.1073/pnas.1316796110
51. Ishida T, Ito A, Sato F, Kusumoto S, Iida S, Inagaki H, et al. Stevens-Johnson syndrome associated with mogamulizumab treatment of adult T-cell leukemia/lymphoma. Cancer Sci. (2013) 104:647–50. doi: 10.1111/cas.12116
52. Duell J, Dittrich M, Bedke T, Mueller T, Eisele F, Rosenwald A, et al. Frequency of regulatory T cells determines the outcome of the T-cell-engaging antibody blinatumomab in patients with B-precursor all. Leukemia. (2017) 31:2181–90. doi: 10.1038/leu.2017.41
53. Atanackovic D, Reinhard H, Meyer S, Spöck S, Grob T, Luetkens T, et al. The trifunctional antibody catumaxomab amplifies and shapes tumor-specific immunity when applied to gastric cancer patients in the adjuvant setting. Hum Vaccines Immunother. (2013) 9:2533–42. doi: 10.4161/hv.26065
54. Goéré D, Flament C, Rusakiewicz S, Poirier-Colame V, Kepp O, Martins I, et al. Potent immunomodulatory effects of the trifunctional antibody catumaxomab. Cancer Res. (2013) 73:4663–73. doi: 10.1158/0008-5472.CAN-12-4460
55. Davids MS, Kim HT, Bachireddy P, Costello C, Liguori R, Savell A, et al. Ipilimumab for patients with relapse after allogeneic transplantation. New Engl J Med. (2016) 375:143–53. doi: 10.1056/NEJMoa1601202
56. Mozzillo N, Simeone E, Benedetto L, Curvietto M, Giannarelli D, Gentilcore G, et al. Assessing a novel immuno-oncology-based combination therapy: ipilimumab plus electrochemotherapy. OncoImmunology. (2015) 4:e1008842. doi: 10.1080/2162402X.2015.1008842
57. Romano E, Kusio-Kobialka M, Foukas PG, Baumgaertner P, Meyer C, Ballabeni P, et al. Ipilimumab-dependent cell-mediated cytotoxicity of regulatory T cells ex vivo by nonclassical monocytes in melanoma patients. Proc Natl Acad Sci USA. (2015) 112:6140–5. doi: 10.1073/pnas.1417320112
58. Simeone E, Gentilcore G, Giannarelli D, Grimaldi AM, Caracò C, Curvietto M, et al. Immunological and biological changes during ipilimumab treatment and their potential correlation with clinical response and survival in patients with advanced melanoma. Cancer Immunol Immunother. (2014) 63:675–83. doi: 10.1007/s00262-014-1545-8
59. Hodi FS, Butler M, Oble DA, Seiden MV, Haluska FG, Kruse A, et al. Immunologic and clinical effects of antibody blockade of cytotoxic T lymphocyte-associated antigen 4 in previously vaccinated cancer patients. Proc Natl Acad Sci USA. (2008) 105:3005–10. doi: 10.1073/pnas.0712237105
60. Jochems C, Tucker JA, Tsang KY, Madan RA, Dahut WL, Liewehr DJ, et al. A combination trial of vaccine plus ipilimumab in metastatic castration-resistant prostate cancer patients: immune correlates. Cancer Immunol Immunother. (2014) 63:407–18. doi: 10.1007/s00262-014-1524-0
61. Tarhini AA, Edington H, Butterfield LH, Lin Y, Shuai Y, Tawbi H, et al. Immune monitoring of the circulation and the tumor microenvironment in patients with regionally advanced melanoma receiving neoadjuvant ipilimumab. PLoS ONE. (2014) 9:e87705. doi: 10.1371/journal.pone.0087705
62. Retseck J, VanderWeele R, Lin HM, Lin Y, Butterfield LH, Tarhini AA. Phenotypic and functional testing of circulating regulatory T cells in advanced melanoma patients treated with neoadjuvant ipilimumab. J Immunother Cancer. (2016) 4:38. doi: 10.1186/s40425-016-0141-1
63. Retseck J, Nasr A, Lin Y, Lin H, Mendiratta P, Butterfield LH, et al. Long term impact of CTLA4 blockade immunotherapy on regulatory and effector immune responses in patients with melanoma. J Transl Med. (2018) 16:184. doi: 10.1186/s12967-018-1563-y
64. Ariyan CE, Brady MS, Siegelbaum RH, Hu J, Bello DM, Rand J, et al. Robust antitumor responses result from local chemotherapy and CTLA-4 Blockade. Cancer Immunol Res. (2018) 6:189–200. doi: 10.1158/2326-6066.CIR-17-0356
65. Balatoni T, Mohos A, Papp E, Sebestyén T, Liszkay G, Oláh J, et al. Tumor-infiltrating immune cells as potential biomarkers predicting response to treatment and survival in patients with metastatic melanoma receiving ipilimumab therapy. Cancer Immunol Immunother. (2018) 67:141–51. doi: 10.1007/s00262-017-2072-1
66. Yi JS, Ready N, Healy P, Dumbauld C, Osborne R, Berry M, et al. Immune activation in early-stage non-small cell lung cancer patients receiving neoadjuvant chemotherapy plus ipilimumab. Clin Cancer Res. (2017) 23:7474–82. doi: 10.1158/1078-0432.CCR-17-2005
67. Oh DY, Cham J, Zhang L, Fong G, Kwek SS, Klinger M, et al. Immune toxicities elicted by CTLA-4 blockade in cancer patients are associated with early diversification of the T-cell repertoire. Cancer Res. (2017) 77:1322–30. doi: 10.1158/0008-5472.CAN-16-2324
68. Haymaker CL, Kim D, Uemura M, Vence LM, Phillip A, McQuail N, et al. Metastatic melanoma patient had a complete response with clonal expansion after whole brain radiation and PD-1 blockade. Cancer Immunol Res. (2017) 5:100–5. doi: 10.1158/2326-6066.CIR-16-0223
69. McArthur HL, Diab A, Page DB, Yuan J, Solomon SB, Sacchini V, et al. A pilot study of preoperative single-dose ipilimumab and/or cryoablation in women with early-stage breast cancer with comprehensive immune profiling. Clin Cancer Res. (2016) 22:5729–37. doi: 10.1158/1078-0432.CCR-16-0190
70. Martens A, Wistuba-Hamprecht K, Yuan J, Postow MA, Wong P, Capone M, et al. Increases in absolute lymphocytes and circulating CD4+ and CD8+ T Cells are associated with positive clinical outcome of melanoma patients treated with ipilimumab. Clin Cancer Res. (2016) 22:4848–58. doi: 10.1158/1078-0432.CCR-16-0249
71. Merchant MS, Wright M, Baird K, Wexler LH, Rodriguez-Galindo C, Bernstein D, et al. Phase I clinical trial of ipilimumab in pediatric patients with advanced solid tumors. Clin Cancer Res. (2016) 22:1364–70. doi: 10.1158/1078-0432.CCR-15-0491
72. Bjoern J, Juul Nitschke N, Zeeberg Iversen T, Schmidt H, Fode K, Svane IM. Immunological correlates of treatment and response in stage IV malignant melanoma patients treated with Ipilimumab. OncoImmunology. (2016) 5:e1100788. doi: 10.1080/2162402X.2015.1100788
73. Kwek SS, Lewis J, Zhang L, Weinberg V, Greaney SK, Harzstark AL, et al. Preexisting levels of CD4 T cells expressing PD-1 are related to overall survival in prostate cancer patients treated with ipilimumab. Cancer Immunol Res. (2015) 3:1008–16. doi: 10.1158/2326-6066.CIR-14-0227
74. Jie HB, Schuler PJ, Lee SC, Srivastava RM, Argiris A, Ferrone S, et al. CTLA-4+ regulatory t cells increased in cetuximab-treated head and neck cancer patients suppress nk cell cytotoxicity and correlate with poor prognosis. Cancer Res. (2015) 75:2200–10. doi: 10.1158/0008-5472.CAN-14-2788
75. Vudattu NK, Waldron-Lynch F, Truman LA, Deng S, Preston-Hurlburt P, Torres R, et al. Humanized mice as a model for aberrant responses in human T cell immunotherapy. J Immunol. (2014) 193:587–96. doi: 10.4049/jimmunol.1302455
76. Pico de Coaña Y, Poschke I, Gentilcore G, Mao Y, Nyström M, Hansson J, et al. Ipilimumab treatment results in an early decrease in the frequency of circulating granulocytic myeloid-derived suppressor cells as well as their Arginase1 production. Cancer Immunol Res. (2013) 1:158–62. doi: 10.1158/2326-6066.CIR-13-0016
77. Gyorki DE, Yuan J, Mu Z, Zaidi B, Pulitzer M, Busam K, et al. Immunological insights from patients undergoing surgery on ipilimumab for metastatic melanoma. Ann Surg Oncol. (2013) 20:3106–11. doi: 10.1245/s10434-013-2999-1
78. Weber JS, Hamid O, Chasalow SD, Wu DY, Parker SM, Galbraith S, et al. Ipilimumab increases activated T cells and enhances humoral immunity in patients with advanced melanoma. J Immunother. (2012) 35:89–97. doi: 10.1097/CJI.0b013e31823aa41c
79. Hamid O, Schmidt H, Nissan A, Ridolfi L, Aamdal S, Hansson J, et al. A prospective phase II trial exploring the association between tumor microenvironment biomarkers and clinical activity of ipilimumab in advanced melanoma. J Transl Med. (2011) 9:204. doi: 10.1186/1479-5876-9-204
80. Zhou J, Bashey A, Zhong R, Corringham S, Messer K, Pu M, et al. CTLA-4 blockade following relapse of malignancy after allogeneic stem cell transplantation is associated with T cell activation but not with increased levels of T regulatory cells. Biol Blood Marrow Transplant. (2011) 17:682–92. doi: 10.1016/j.bbmt.2010.08.005
81. Sarnaik AA, Yu B, Yu D, Morelli D, Hall M, Bogle D, et al. Extended dose ipilimumab with a peptide vaccine: Immune correlates associated with clinical benefit in patients with resected high-risk stage IIIc/IV melanoma. Clin Cancer Res. (2011) 17:896–906. doi: 10.1158/1078-0432.CCR-10-2463
82. Carthon BC, Wolchok JD, Yuan J, Kamat A, Ng Tang DS, Sun J, et al. Preoperative CTLA-4 blockade: tolerability and immune monitoring in the setting of a presurgical clinical trial. Clin Cancer Res. (2010) 16:2861–71. doi: 10.1158/1078-0432.CCR-10-0569
83. Liakou CI, Kamat A, Tang DN, Chen H, Sun J, Troncoso P, et al. CTLA-4 blockade increases IFNgamma-producing CD4+ICOShi cells to shift the ratio of effector to regulatory T cells in cancer patients. Proc Natl Acad Sci USA. (2008) 105:14987–92. doi: 10.1073/pnas.0806075105
84. Kavanagh B, O'Brien S, Lee D, Hou Y, Weinberg V, Rini B, et al. CTLA4 blockade expands FoxP3+ regulatory and activated effector CD4+ T cells in a dose-dependent fashion. Blood. (2008) 112:1175–83. doi: 10.1182/blood-2007-11-125435
85. O'Mahony D, Morris JC, Quinn C, Gao W, Wilson WH, Gause B, et al. A pilot study of CTLA-4 blockade after cancer vaccine failure in patients with advanced malignancy. Clin Cancer Res. (2007) 13:958–64. doi: 10.1158/1078-0432.CCR-06-1974
86. Maker AV, Attia P, Rosenberg SA. Analysis of the cellular mechanism of antitumor responses and autoimmunity in patients treated with CTLA-4 blockade. J Immunol. (2005) 175:7746–54. doi: 10.4049/jimmunol.175.11.7746
87. Wu SP, Liao RQ, Tu HY, Wang WJ, Dong ZY, Huang SM, et al. Stromal PD-L1–Positive regulatory T cells and PD-1–Positive CD8-Positive T cells define the response of different subsets of non–small cell lung cancer to PD-1/PD-L1 Blockade Immunotherapy. J Thorac Oncol. (2018) 13:521–32. doi: 10.1016/j.jtho.2017.11.132
88. Ferris RL, Blumenschein G, Harrington K, Fayette J, Guigay J, Colevas AD, et al. Tumor-associated immune cell PD-L1 expression and peripheral immune profiling: analyses from checkMate 141 [abstract]. In: Proceedings of the AACR Annual Meeting; 2017, Apr 1-5; Washington DC, USA. Cancer Res. (2017) 77(Suppl. 13): Abstract nr CT021. doi: 10.1158/1538-7445.AM2017-CT021
89. Gibney GT, Kudchadkar RR, DeConti RC, Thebeau MS, Czupryn MP, Tetteh L, et al. Safety, correlative markers, and clinical results of adjuvant nivolumab in combination with vaccine in resected high-risk metastatic melanoma. Clin Cancer Res. (2015) 21:712–20. doi: 10.1158/1078-0432.CCR-14-2468
90. DiDomenico J, Lamano JB, Oyon D, Li Y, Veliceasa D, Kaur G, et al. The immune checkpoint protein PD-L1 induces and maintains regulatory T cells in glioblastoma. Oncoimmunology. (2018) 7:e1448329. doi: 10.1080/2162402X.2018.1448329
91. Herrera AF, Moskowitz AJ, Bartlett NL, Vose JM, Ramchandren R, Feldman TA, et al. Interim results of brentuximab vedotin in combination with nivolumab in patients with relapsed or refractory Hodgkin lymphoma. Blood. (2018) 131:1183–94. doi: 10.1182/blood-2017-10-811224
92. Nonomura Y, Otsuka A, Nakashima C, Seidel JA, Kitoh A, Dainichi T, et al. Peripheral blood Th9 cells are a possible pharmacodynamic biomarker of nivolumab treatment efficacy in metastatic melanoma patients. OncoImmunology. (2016) 5:e1248327. doi: 10.1080/2162402X.2016.1248327
93. Sanmamed MF, Rodriguez I, Schalper KA, Oñate C, Azpilikueta A, Rodriguez-Ruiz ME, et al. Nivolumab and urelumab enhance antitumor activity of human T lymphocytes engrafted in Rag2-/-IL2Rγnullimmunodeficient mice. Cancer Res. (2015) 75:3466–78. doi: 10.1158/0008-5472.CAN-14-3510
94. Wang C, Thudium KB, Han M, Wang XT, Huang H, Feingersh D, et al. In vitro characterization of the anti-PD-1 antibody nivolumab, BMS-936558, and in vivo toxicology in non-human primates. Cancer Immunol Res. (2014) 2:846–56. doi: 10.1158/2326-6066.CIR-14-0040
95. Toor SM, Sasidharan Nair V, Pfister G, Elkord E. Effect of pembrolizumab on CD4(+) CD25(+), CD4(+) LAP(+) and CD4(+) TIM-3(+) T cell subsets. Clin Exp Immunol. (2019) 196:345–52. doi: 10.1111/cei.13264
96. Toor SM, Syed Khaja AS, Alkurd I, Elkord E. In-vitro effect of pembrolizumab on different T regulatory cell subsets. Clin Exp Immunol. (2018) 191:189–97. doi: 10.1111/cei.13060
97. Ribas A, Shin DS, Zaretsky J, Frederiksen J, Cornish A, Avramis E, et al. PD-1 blockade expands intratumoral memory T cells. Cancer Immunol Res. (2016) 4:194–203. doi: 10.1158/2326-6066.CIR-15-0210
98. Balasa RI, Simu M, Voidazan S, Barcutean LI, Bajko Z, Hutanu A, et al. Natalizumab changes the peripheral profile of the Th17 panel in MS patients: new mechanisms of action. CNS Neurol Disord Drug Targets. (2017) 16:1018–26. doi: 10.2174/1871527316666170807130632
99. Rodi M, Dimisianos N, De Lastic AL, Sakellaraki P, Deraos G, Matsoukas J, et al. Regulatory cell populations in relapsing-remitting multiple sclerosis (RRMS) patients: effect of disease activity and treatment regimens. Int J Mol Sci. (2016) 17:E1398. doi: 10.3390/ijms17091398
100. Kimura K, Nakamura M, Sato W, Okamoto T, Araki M, Lin Y, et al. Disrupted balance of T cells under natalizumab treatment in multiple sclerosis. Neurol Neuroimmunol Neuroinflamm. (2016) 3:e210. doi: 10.1212/NXI.0000000000000210
101. Kurmaeva E, Lord JD, Zhang S, Bao JR, Kevil CG, Grisham MB, et al. T cell-associated alpha4beta7 but not alpha4beta1 integrin is required for the induction and perpetuation of chronic colitis. Mucosal Immunol. (2014) 7:1354–65. doi: 10.1038/mi.2014.22
102. Wyant T, Yang L, Fedyk E. In vitro assessment of the effects of vedolizumab binding on peripheral blood lymphocytes. MAbs. (2013) 5:842–50. doi: 10.4161/mabs.26392
103. Burman J, Fransson M, Tötterman TH, Fagius J, Mangsbo SM, Loskog ASI. T-cell responses after haematopoietic stem cell transplantation for aggressive relapsing-remitting multiple sclerosis. Immunology. (2013) 140:211–9. doi: 10.1111/imm.12129
104. Ramos-Cejudo J, Oreja-Guevara C, Aroeira LS, De Antonio LR, Chamorro B, Diez-Tejedor E. Treatment with natalizumab in relapsing-remitting multiple sclerosis patients induces changes in inflammatory mechanism. J Clin Immunol. (2011) 31:623–31. doi: 10.1007/s10875-011-9522-x
105. Frisullo G, Iorio R, Plantone D, Marti A, Nociti V, Patanella AK, et al. CD4+T-bet+, CD4+pSTAT3+ and CD8+T-bet+ T cells accumulate in peripheral blood during NZB treatment. Mult Scler J. (2011) 17:556–66. doi: 10.1177/1352458510392263
106. Mouzaki A, Koutsokera M, Dervilli Z, Rodi M, Kalavrizioti D, Dimisianos N, et al. Remitting-relapsing multiple sclerosis patient refractory to conventional treatments and bone marrow transplantation who responded to natalizumab. Int J Gen Med. (2010) 3:313–20. doi: 10.2147/IJGM.S13648
107. Putzki N, Baranwal MK, Tettenborn B, Limmroth V, Kreuzfelder E. Effects of natalizumab on circulating B cells, T regulatory cells and natural killer cells. Eur Neurol. (2010) 63:311–7. doi: 10.1159/000302687
108. Stenner MP, Waschbisch A, Buck D, Doerck S, Einsele H, Toyka KV, et al. Effects of natalizumab treatment on Foxp3+ T regulatory cells. PLoS ONE. (2008) 3:e3319. doi: 10.1371/journal.pone.0003319
109. Fischer A, Zundler S, Atreya R, Rath T, Voskens C, Hirschmann S, et al. Differential effects of a4ß7 and GPR15 on homing of effector and regulatory T cells from patients with UC to the inflamed gut in vivo. Gut. (2016) 65:1642–64. doi: 10.1136/gutjnl-2015-310022
110. Alfinito F, Ruggiero G, Sica M, Udhayachandran A, Rubino V, Pepa RD, et al. Eculizumab treatment modifies the immune profile of PNH patients. Immunobiology. (2012) 217:698–703. doi: 10.1016/j.imbio.2011.11.009
111. Chen YB, Perales MA, Li S, Kempner M, Reynolds C, Brown J, et al. Phase 1 multicenter trial of brentuximab vedotin for steroid-refractory acute graft-versus-host disease. Blood. (2017) 129:3256–61. doi: 10.1182/blood-2017-03-772210
112. Kitadate A, Kobayashi H, Abe Y, Narita K, Miura D, Takeuchi M, et al. Pretreatment CD38-positive regulatory T-cells affect the durable response to daratumumab in relapsed/refractory multiple myeloma patients. Haematologica. (2020) 105:e37–40. doi: 10.3324/haematol.2019.219683
113. Krejcik J, Casneuf T, Nijhof IS, Verbist B, Bald J, Plesner T, et al. Daratumumab depletes CD38+immune regulatory cells, promotes T-cell expansion, and skews T-cell repertoire in multiple myeloma. Blood. (2016) 128:384–94. doi: 10.1182/blood-2015-12-687749
114. Miyabe C, Miyabe Y, Strle K, Kim ND, Stone JH, Luster AD, et al. An expanded population of pathogenic regulatory T cells in giant cell arteritis is abrogated by IL-6 blockade therapy. Ann Rheum Dis. (2017) 76:898–905. doi: 10.1136/annrheumdis-2016-210070
115. Pedersen AE, Holmstrøm K, Jørgensen F, Jensen SS, Gad M. Development of assay platforms for in vitro screening of Treg modulating potential of pharmacological compounds. Immunopharmacol Immunotoxicol. (2015) 37:63–71. doi: 10.3109/08923973.2014.977449
116. Khoury T, Molho-Pessach V, Ramot Y, Ayman AR, Elpeleg O, Berkman N, et al. Tocilizumab promotes regulatory T-cell alleviation in STAT3 gain-of-function-associated multi-organ autoimmune syndrome. Clin Ther. (2017) 39:444–9. doi: 10.1016/j.clinthera.2017.01.004
117. Tada Y, Ono N, Suematsu R, Tashiro S, Sadanaga Y, Tokuda Y, et al. The balance between Foxp3 and Ror-gammat expression in peripheral blood is altered by tocilizumab and abatacept in patients with rheumatoid arthritis. BMC Musculoskelet Disord. (2016) 17:290. doi: 10.1186/s12891-016-1137-1
118. Li S, Wu Z, Li L, Liu X. Interleukin-6 (IL-6) receptor antagonist protects against rheumatoid arthritis. Med Sci Monit. (2016) 22:2113–8. doi: 10.12659/MSM.896355
119. Trinschek B, Luessi F, Gross CC, Wiendl H, Jonuleit H. Interferon-beta therapy of multiple sclerosis patients improves the responsiveness of t cells for immune suppression by regulatory T cells. Int J Mol Sci. (2015) 16:16330–46. doi: 10.3390/ijms160716330
120. Ortiz MA, Diaz-Torné C, Hernández MV, Reina D, de la Fuente D, Castellví I, et al. IL-6 blockade reverses the abnormal STAT activation of peripheral blood leukocytes from rheumatoid arthritis patients. Clin Immunol. (2015) 158:174–82. doi: 10.1016/j.clim.2015.03.025
121. Takatori H, Kawashima H, Matsuki A, Meguro K, Tanaka S, Iwamoto T, et al. Helios enhances treg cell function in cooperation with FoxP3. Arthritis Rheum. (2015) 67:1491–502. doi: 10.1002/art.39091
122. Daïen CI, Gailhac S, Audo R, Mura T, Hahne M, Combe B, et al. High levels of natural killer cells are associated with response to tocilizumab in patients with severe rheumatoid arthritis. Rheumatology. (2014) 54:601–8. doi: 10.1093/rheumatology/keu363
123. Kikuchi J, Hashizume M, Kaneko Y, Yoshimoto K, Nishina N, Takeuchi T. Peripheral blood CD4+CD25+CD127low regulatory T cells are significantly increased by tocilizumab treatment in patients with rheumatoid arthritis: Increase in regulatory T cells correlates with clinical response. Arthritis Res Ther. (2015) 17:10. doi: 10.1186/s13075-015-0526-4
124. Sarantopoulos A, Tselios K, Gkougkourelas I, Pantoura M, Georgiadou AM, Boura P. Tocilizumab treatment leads to a rapid and sustained increase in Treg cell levels in rheumatoid arthritis patients: comment on the article by Thiolat et al. Arthritis Rheum. (2014) 66:2638. doi: 10.1002/art.38714
125. Thiolat A, Semerano L, Pers YM, Biton J, Lemeiter D, Portales P, et al. Interleukin-6 receptor blockade enhances CD39+ regulatory T cell development in rheumatoid arthritis and in experimental arthritis. Arthritis Rheum. (2014) 66:273–83. doi: 10.1002/art.38246
126. Guggino G, Giardina AR, Raimondo S, Giardina G, Sireci G, Dieli F, et al. Targeting IL-6 signalling in early rheumatoid arthritis is followed by Th1 and Th17 suppression and Th2 expansion. Clin Exp Rheumatol. (2014) 32:77–81.
127. Pesce B, Soto L, Sabugo F, Wurmann P, Cuchacovich M, López MN, et al. Effect of interleukin-6 receptor blockade on the balance between regulatory T cells and T helper type 17 cells in rheumatoid arthritis patients. Clin Exp Immunol. (2013) 171:237–42. doi: 10.1111/cei.12017
128. Samson M, Audia S, Janikashvili N, Ciudad M, Trad M, Fraszczak J, et al. Brief report: inhibition of interleukin-6 function corrects Th17/Treg cell imbalance in patients with rheumatoid arthritis. Arthritis Rheum. (2012) 64:2499–503. doi: 10.1002/art.34477
129. Betts BC, St Angelo ET, Kennedy M, Young JW. Anti-IL6-receptor-alpha (tocilizumab) does not inhibit human monocyte-derived dendritic cell maturation or alloreactive T-cell responses. Blood. (2011) 118:5340–3. doi: 10.1182/blood-2011-06-363390
130. Pidala J, Beato F, Kim J, Betts B, Jim H, Sagatys E, et al. In Vivo IL-12/IL-23p40 neutralization blocks Th1/Th17 response after allogeneic hematopoietic cell transplantation. Haematologica. (2018) 103:531–9. doi: 10.3324/haematol.2017.171199
131. Samson M, Ghesquière T, Berthier S, Bonnotte B. Ustekinumab inhibits Th1 and Th17 polarisation in a patient with giant cell arteritis. Ann Rheum Dis. (2018) 77:e6. doi: 10.1136/annrheumdis-2017-211622
132. Tada Y, Togashi Y, Kotani D, Kuwata T, Sato E, Kawazoe A, et al. Targeting VEGFR2 with ramucirumab strongly impacts effector/ activated regulatory T cells and CD8+ T cells in the tumor microenvironment. J Immunother Cancer. (2018) 6:106. doi: 10.1186/s40425-018-0403-1
133. Romano A, Parrinello NL, Chiarenza A, Motta G, Tibullo D, Giallongo C, et al. Immune off-target effects of brentuximab vedotin in relapsed/refractory Hodgkin lymphoma. Br J Haematol. (2019) 185:468–79. doi: 10.1111/bjh.15801
134. Prete M, Leone P, Frassanito MA, Desantis V, Marasco C, Cicco S, et al. Belimumab restores Treg/Th17 balance in patients with refractory systemic lupus erythematosus. Lupus. (2018) 27:1926–35. doi: 10.1177/0961203318797425
135. Perez SA, Karamouzis MV, Skarlos DV, Ardavanis A, Sotiriadou NN, Iliopoulou EG, et al. CD4+CD25+ regulatory T-cell frequency in HER-2/neu (HER)-positive and HER-negative advanced-stage breast cancer patients. Clin Cancer Res. (2007) 13:2714–21. doi: 10.1158/1078-0432.CCR-06-2347
136. Tono Y, Ishihara M, Miyahara Y, Tamaru S, Oda H, Yamashita Y, et al. Pertuzumab, trastuzumab and eribulin mesylate therapy for previously treated advanced HER2-positive breast cancer: a feasibility study with analysis of biomarkers. Oncotarget. (2018) 9:14909–21. doi: 10.18632/oncotarget.24504
137. Ishikawa T, Okayama T, Sakamoto N, Ideno M, Oka K, Enoki T, et al. Phase I clinical trial of adoptive transfer of expanded natural killer cells in combination with IgG1 antibody in patients with gastric or colorectal cancer. Int J Cancer. (2018) 142:2599–609. doi: 10.1002/ijc.31285
138. Muller P, Kreuzaler M, Khan T, Thommen DS, Martin K, Glatz K, et al. Trastuzumab emtansine (T-DM1) renders HER2+ breast cancer highly susceptible to CTLA-4/PD-1 blockade. Sci Transl Med. (2015) 7:315ra188. doi: 10.1126/scitranslmed.aac4925
139. García-Martínez E, Gil GL, Benito AC, González-Billalabeitia E, Conesa MAV, García TG, et al. Tumor-infiltrating immune cell profiles and their change after neoadjuvant chemotherapy predict response and prognosis of breast cancer. Breast Cancer Res. (2014) 16:488. doi: 10.1186/s13058-014-0488-5
140. Petricevic B, Laengle J, Singer J, Sachet M, Fazekas J, Steger G, et al. Trastuzumab mediates antibody-dependent cell-mediated cytotoxicity and phagocytosis to the same extent in both adjuvant and metastatic HER2/neu breast cancer patients. J Transl Med. (2013) 11:307. doi: 10.1186/1479-5876-11-307
141. Horlock C, Stott B, Dyson PJ, Morishita M, Coombes RC, Savage P, et al. The effects of trastuzumab on the CD4CD25FoxP3 and CD4IL17A T-cell axis in patients with breast cancer. Br J Cancer. (2009) 100:1061–7. doi: 10.1038/sj.bjc.6604963
142. Ladoire S, Arnould L, Apetoh L, Coudert B, Martin F, Chauffert B, et al. Pathologic complete response to neoadjuvant chemotherapy of breast carcinoma is associated with the disappearance of tumor-infiltrating Foxp3+regulatory T cells. Clin Cancer Res. (2008) 14:2413–20. doi: 10.1158/1078-0432.CCR-07-4491
143. Force J, Howie LJ, Abbott SE, Bentley R, Marcom PK, Kimmick G, et al. Early stage HER2-positive breast cancers not achieving a pCR from neoadjuvant trastuzumab- or pertuzumab-based regimens have an immunosuppressive phenotype. Clin Breast Cancer. (2018) 18:410–7. doi: 10.1016/j.clbc.2018.02.010
144. Roep BO, Peakman M. Surrogate end points in the design of immunotherapy trials: emerging lessons from type 1 diabetes. Nat Rev Immunol. (2010) 10:145–52. doi: 10.1038/nri2705
145. Shou J, Zhang Z, Lai Y, Chen Z, Huang J. Worse outcome in breast cancer with higher tumor-infiltrating FOXP3+ Tregs : a systematic review and meta-analysis. BMC Cancer. (2016) 16:687. doi: 10.1186/s12885-016-2732-0
146. Bates GJ, Fox SB, Han C, Leek RD, Garcia JF, Harris AL, et al. Quantification of regulatory T cells enables the identification of high-risk breast cancer patients and those at risk of late relapse. J Clin Oncol. (2006) 24:5373–80. doi: 10.1200/JCO.2006.05.9584
147. Liyanage UK, Moore TT, Joo HG, Tanaka Y, Herrmann V, Doherty G, et al. Prevalence of regulatory T cells is increased in peripheral blood and tumor microenvironment of patients with pancreas or breast adenocarcinoma. J Immunol. (2002) 169:2756–61. doi: 10.4049/jimmunol.169.5.2756
148. Winthrop KL. The emerging safety profile of JAK inhibitors in rheumatic disease. Nat Rev Rheumatol. (2017) 13:234–43. doi: 10.1038/nrrheum.2017.23
149. Yao Z, Kanno Y, Kerenyi M, Stephens G, Durant L, Watford WT, et al. Nonredundant roles for Stat5a/b in directly regulating foxp. Blood. (2007) 109:4368–75. doi: 10.1182/blood-2006-11-055756
150. Burchill MA, Yang J, Vogtenhuber C, Blazar BR, Farrar MA. IL-2 receptor ß-dependent STAT5 activation is required for the development of Foxp3+ regulatory T cells. J Immunol. (2007) 178:280–90. doi: 10.4049/jimmunol.178.1.280
151. Choi J, Cooper ML, Staser K, Ashami K, Vij KR, Wang B, et al. Baricitinib-induced blockade of interferon gamma receptor and interleukin-6 receptor for the prevention and treatment of graft-versus-host disease. Leukemia. (2018) 32:2483–94. doi: 10.1038/s41375-018-0123-z
152. Aguilar-Pimentel A, Graessel A, Alessandrini F, Fuchs H, Gailus-Durner V, Hrabe De Angelis M, et al. Improved efficacy of allergen-specific immunotherapy by JAK inhibition in a murine model of allergic asthma. PLoS ONE. (2017) 12:e0178563. doi: 10.1371/journal.pone.0178563
153. Zhou Y, Leng X, Luo S, Su Z, Luo X, Guo H, et al. Tolerogenic dendritic cells generated with tofacitinib ameliorate experimental autoimmune encephalomyelitis through modulation of Th17/Treg balance. J Immunol Res. (2016) 2016:5021537. doi: 10.1155/2016/5021537
154. Yokoyama S, Perera PY, Terawaki S, Watanabe N, Kaminuma O, Waldmann TA, et al. Janus kinase inhibitor tofacitinib shows potent efficacy in a mouse model of Autoimmune Lymphoproliferative Syndrome (ALPS). J Clin Immunol. (2015) 35:661–7. doi: 10.1007/s10875-015-0203-z
155. Kubo S, Yamaoka K, Kondo M, Yamagata K, Zhao J, Iwata S, et al. The JAK inhibitor, tofacitinib, reduces the T cell stimulatory capacity of human monocyte-derived dendritic cells. Ann Rheum Dis. (2014) 73:2192–8. doi: 10.1136/annrheumdis-2013-203756
156. Stevenson W, Sadrai Z, Hua J, Kodati S, Huang JF, Chauhan SK, et al. Effects of topical Janus Kinase inhibition on ocular surface inflammation and immunity. Cornea. (2014) 33:177–83. doi: 10.1097/ICO.0000000000000019
157. Sewgobind VDKD, Quaedackers ME, Van Der Laan LJW, Kraaijeveld R, Korevaar SS, Chan G, et al. The JAK inhibitor CP-690,550 preserves the function of CD4+CD25brightFoxP3+regulatory T cells and inhibits effector T cells. Am J Transplant. (2010) 10:1785–95. doi: 10.1111/j.1600-6143.2010.03200.x
158. Van Gurp EAFJ, Schoordijk-Verschoor W, Klepper M, Korevaar SS, Chan G, Weimar W, et al. The effect of the JAK inhibitor CP-690,550 on peripheral immune parameters in stable kidney allograft patients. Transplantation. (2009) 87:79–86. doi: 10.1097/TP.0b013e31818bbea7
159. Feng X, Zhang L, Acharya C, An G, Wen K, Qiu L, et al. Targeting CD38 suppresses induction and function of T regulatory cells to mitigate immunosuppression in multiple myeloma. Clin Cancer Res. (2017) 23:4290–300. doi: 10.1158/1078-0432.CCR-16-3192
160. Kaplon H, Reichert JM. Antibodies to watch in 2019. MAbs. (2019) 11:219–38. doi: 10.1080/19420862.2018.1556465
161. Amat F, Tallon P, Foray AP, Michaud B, Lambert N, Saint-Pierre P, et al. Control of asthma by omalizumab: the role of CD4+Foxp3+regulatory T cells. Clin Exp Allergy. (2016) 46:1614–6. doi: 10.1111/cea.12839
162. Talotta R, Berzi A, Atzeni F, Batticciotto A, Clerici M, Sarzi-Puttini P, et al. Paradoxical expansion of Th1 and Th17 Lymphocytes in rheumatoid arthritis following infliximab treatment: a possible explanation for a lack of clinical response. J Clin Immunol. (2015) 35:550–7. doi: 10.1007/s10875-015-0182-0
163. Bhattacharjee R, De D, Handa S, Minz RW, Saikia B, Joshi N. Assessment of the effects of rituximab monotherapy on different subsets of circulating t-regulatory cells and clinical disease severity in severe pemphigus vulgaris. Dermatology. (2017) 232:572–7. doi: 10.1159/000448031
164. Roccatello D, Sciascia S, Di Simone D, Solfietti L, Naretto C, Fenoglio R, et al. New insights into immune mechanisms underlying response to rituximab in patients with membranous nephropathy: a prospective study and a review of the literature. Autoimmun Rev. (2016) 15:529–38. doi: 10.1016/j.autrev.2016.02.014
165. Butterfield LH, Palucka AK, Britten CM, Dhodapkar MV, Håkansson L, Janetzki S, et al. Recommendations from the iSBTc-SITC/FDA/NCI workshop on immunotherapy biomarkers. Clin Cancer Res. (2011) 17:3064–76. doi: 10.1158/1078-0432.CCR-10-2234
166. D'Arena G, Vitale C, Coscia M, Festa A, Di Minno NMD, De Feo V, et al. Regulatory T cells and their prognostic relevance in hematologic malignancies. J Immunol Res. (2017) 2017:1832968. doi: 10.1155/2017/1832968
167. Okamura K, Fukuda Y, Soda H, Ogawara D, Iwasaki K, Fuchi S, et al. Pulmonary pleomorphic carcinoma with few PD-1-positive immune cells and regulatory T cells that showed a complete response to nivolumab. Thorac Cancer. (2018) 9:193–6. doi: 10.1111/1759-7714.12557
168. Ogawara D, Soda H, Iwasaki K, Suyama T, Taniguchi H, Fukuda Y, et al. Remarkable response of nivolumab-refractory lung cancer to salvage chemotherapy. Thorac Cancer. (2018) 9:175–80. doi: 10.1111/1759-7714.12543
169. Uchida A, Watanabe M, Nawata A, Ikari Y, Sasaki M, Shigemoto K, et al. Tubulointerstitial nephritis as adverse effect of programmed cell death 1 inhibitor, nivolumab, showed distinct histological findings. CEN Case Rep. (2017) 6:169–74. doi: 10.1007/s13730-017-0269-y
170. Lin YC, Mahalingam J, Chiang JM, Su PJ, Chu YY, Lai HY, et al. Activated but not resting regulatory T cells accumulated in tumor microenvironment and correlated with tumor progression in patients with colorectal cancer. Int J Cancer. (2013) 132:1341–50. doi: 10.1002/ijc.27784
171. Mohty M. Mechanisms of action of antithymocyte globulin: T-cell depletion and beyond. Leukemia. (2007) 21:1387–94. doi: 10.1038/sj.leu.2404683
172. Lopez M, Clarkson MR, Albin M, Sayegh MH, Najafian N. A novel mechanism of action for anti-thymocyte globulin: induction of CD4+CD25+Foxp3+ regulatory T cells. J Am Soc Nephrol. (2006) 17:2844–53. doi: 10.1681/ASN.2006050422
173. Santegoets SJAM, Dijkgraaf EM, Battaglia A, Beckhove P, Britten CM, Gallimore A, et al. Monitoring regulatory T cells in clinical samples: consensus on an essential marker set and gating strategy for regulatory T cell analysis by flow cytometry. Cancer Immunol Immunother. (2015) 64:1271–86. doi: 10.1007/s00262-015-1729-x
174. Vignali DAA, Collison LW, Workman CJ. How regulatory T cells work. Nat Rev Immunol. (2008) 8:523–32. doi: 10.1038/nri2343
175. Mills KHG. Regulatory T cells: friend or foe in immunity to infection? Nat Rev Immunol. (2004) 4:841–55. doi: 10.1038/nri1485
176. Vadasz Z, Toubi E. FoxP3 expression in macrophages, cancer, and B Cells - Is it real? Clin Rev Allergy Immunol. (2017) 52:364–72. doi: 10.1007/s12016-016-8572-5
177. Ladoire S, Arnould L, Mignot G, Coudert B, Rébé C, Chalmin F, et al. Presence of Foxp3 expression in tumor cells predicts better survival in HER2-overexpressing breast cancer patients treated with neoadjuvant chemotherapy. Breast Cancer Res Treat. (2011) 125:65–72. doi: 10.1007/s10549-010-0831-1
178. Karanikas V, Speletas M, Zamanakou M, Kalala F, Loules G, Kerenidi T, et al. Foxp3 expression in human cancer cells. J Transl Med. (2008) 6:19. doi: 10.1186/1479-5876-6-19
179. Ebert LM, Bee ST, Browning J, Svobodova S, Russell SE, Kirkpatrick N, et al. The regulatory T cell-associated transcription factor FoxP3 is expressed by tumor cells. Cancer Res. (2008) 68:3001–9. doi: 10.1158/0008-5472.CAN-07-5664
180. Wang J, Ioan-Facsinay A, van der Voort EIH, Huizinga TWJ, Toes REM. Transient expression of FOXP3 in human activated nonregulatory CD4+ T cells. Eur J Immunol. (2007) 37:129–38. doi: 10.1002/eji.200636435
181. Morgan ME, Van Bilsen JHM, Bakker AM, Heemskerk B, Schilham MW, Hartgers FC, et al. Expression of FOXP3 mRNA is not confined to CD4 +CD25 + T regulatory cells in humans. Hum Immunol. (2005) 66:13–20. doi: 10.1016/j.humimm.2004.05.016
182. Petsiou A, Paschou SA, Vartholomatos G, Chatzigianni K, Kolaitis N, Giotaki E, et al. A modified flow cytometry method for objective estimation of human CD4(+) regulatory T cells (CD4(+) Tregs) in peripheral blood, via CD4/CD25/CD45RO/FoxP3 labeling. Cytometry B Clin Cytom. (2019). doi: 10.1002/cyto.b.21841. [Epub ahead of print].
183. Camisaschi C, Tazzari M, Rivoltini L, Castelli C. Monitoring the frequency and function of regulatory T cells and summary of the approaches currently used to inhibit regulatory T cells in cancer patients. Methods Mol Biol. (2014) 1139:201–21. doi: 10.1007/978-1-4939-0345-0_18
184. Pitoiset F, Barbie M, Monneret G, Braudeau C, Pochard P, Pellegrin I, et al. A standardized flow cytometry procedure for the monitoring of regulatory T cells in clinical trials. Cytometry B Clin Cytom. (2018) 94:621–6. doi: 10.1002/cyto.b.21622.
185. Miyara M, Sakaguchi S. Human FoxP3+ CD4+ regulatory T cells: their knowns and unknowns. Immunol Cell Biol. (2011) 89:346–51. doi: 10.1038/icb.2010.137
186. Ihara F, Sakurai D, Horinaka A, Makita Y, Fujikawa A, Sakurai T, et al. CD45RA(−)Foxp3(high) regulatory T cells have a negative impact on the clinical outcome of head and neck squamous cell carcinoma. Cancer Immunol Immunother. (2017) 66:1275–85. doi: 10.1007/s00262-017-2021-z
187. Miyara M, Yoshioka Y, Kitoh A, Shima T, Wing K, Niwa A, et al. Functional delineation and differentiation dynamics of human CD4+ T cells expressing the FoxP3 transcription factor. Immunity. (2009) 30:899–911. doi: 10.1016/j.immuni.2009.03.019
188. Shevach EM, Thornton AM. tTregs, pTregs, and iTregs: similarities and differences. Immunol Rev. (2014) 259:88–102. doi: 10.1111/imr.12160
189. Lin X, Chen M, Liu Y, Guo Z, He X Dr., Brand D, et al. Advances in distinguishing natural from induced Foxp3+regulatory T cells. Int J Clin Exp Pathol. (2013) 6:116–23.
190. Ito T, Hanabuchi S, Wang YH, Park WR, Arima K, Bover L, et al. Two functional subsets of FOXP3+ regulatory T cells in human thymus and periphery. Immunity. (2008) 28:870–80. doi: 10.1016/j.immuni.2008.03.018
191. Huehn J, Polansky JK, Hamann A. Epigenetic control of FOXP3 expression: the key to a stable regulatory T-cell lineage? Nat Rev Immunol. (2009) 9:83–9. doi: 10.1038/nri2474
192. Baron U, Floess S, Wieczorek G, Baumann K, Grützkau A, Dong J, et al. DNA demethylation in the human FOXP3 locus discriminates regulatory T cells from activated FOXP3+conventional T cells. Eur J Immunol. (2007) 37:2378–89. doi: 10.1002/eji.200737594
193. Zhang L, Manirarora JN, Wei CH. Evaluation of immunosuppressive function of regulatory T cells using a novel in vitro cytotoxicity assay. Cell Biosci. (2014) 4:51. doi: 10.1186/2045-3701-4-51
194. Mcmurchy AN, Levings MK. Suppression assays with human T regulatory cells: a technical guide. Eur J Immunol. (2012) 42:27–34. doi: 10.1002/eji.201141651
195. Whiteside TL. What are regulatory T cells (Treg) regulating in cancer and why? Semin Cancer Biol. (2012) 22:327–34. doi: 10.1016/j.semcancer.2012.03.004
196. Levitsky J, Miller J, Leventhal J, Huang X, Flaa C, Wang E, et al. The human “treg MLR”: immune monitoring for FOXP3+ T regulatory cell generation. Transplantation. (2009) 88:1303–11. doi: 10.1097/TP.0b013e3181bbee98
197. Porter SB, Liu B, Rogosheske J, Levine BL, June CH, Kohl VK, et al. Suppressor function of umbilical cord blood-derived CD4+CD25+T-regulatory cells exposed to graft-versus-host disease drugs. Transplantation. (2006) 82:23–9. doi: 10.1097/01.tp.0000225824.48931.af
198. Hill D, Eastaff-Leung N, Bresatz-Atkins S, Warner N, Ruitenberg J, Krumbiegel D, et al. Inhibition of activation induced CD154 on CD4 CD25 cells: a valid surrogate for human Treg suppressor function. Immunol Cell Biol. (2012) 90:812–21. doi: 10.1038/icb.2012.18
199. Canavan JB, Afzali B, Scottà C, Fazekasova H, Edozie FC, Macdonald TT, et al. A rapid diagnostic test for human regulatory T-cell function to enable regulatory T-cell therapy. Blood. (2012) 119:e57–66. doi: 10.1182/blood-2011-09-380048
200. Ruitenberg JJ, Boyce C, Hingorani R, Putnam AA, Ghanekar S. Rapid assessment of in vitro expanded human regulatory T cell function. J Immunol Methods. (2011) 372:95–106. doi: 10.1016/j.jim.2011.07.001
201. Chu ST, Chien KH, Lin HH, Wu WH, Jian JY, Tzeng WF, et al. Using marker gene analysis instead of mixed lymphocyte reaction assay for identification of functional CD4+FOXP3+ regulatory T cells. Biotechnol Lett. (2018) 40:535–42. doi: 10.1007/s10529-017-2498-8
202. Azimi M, Aslani S, Mortezagholi S, Salek A, Javan MR, Rezaiemanesh A, et al. Identification, isolation, and functional assay of regulatory T Cells. Immunol Invest. (2016) 45:584–602. doi: 10.1080/08820139.2016.1193869
203. Whiteside TL. Regulatory T Cell (Treg) assays: repertoire, functions, and clinical importance of human treg. In: Detrick B. Schmitz J, Hamilton R, editors. Manual of Molecular and Clinical Laboratory Immunology. American Society for Microbiology (2016). p. 296–9. doi: 10.1128/9781555818722.ch31
204. Brusko TM, Hulme MA, Myhr CB, Haller MJ, Atkinson MA. Assessing the in vitro suppressive capacity of regulatory T cells. Immunol Invest. (2007) 36:607–28. doi: 10.1080/08820130701790368
Keywords: regulatory T cells, immunomonitoring, monoclonal antibodies, JAK inhibitors, registration dossiers, biomarkers, (pre-)clinical study recommendations
Citation: de Wolf ACMT, Herberts CA and Hoefnagel MHN (2020) Dawn of Monitoring Regulatory T Cells in (Pre-)clinical Studies: Their Relevance Is Slowly Recognised. Front. Med. 7:91. doi: 10.3389/fmed.2020.00091
Received: 19 November 2019; Accepted: 03 March 2020;
Published: 02 April 2020.
Edited by:
Sandor Kerpel-Fronius, Semmelweis University, HungaryReviewed by:
Frits Lekkerkerker, Consultant, Amsterdam, NetherlandsCopyright © 2020 de Wolf, Herberts and Hoefnagel. This is an open-access article distributed under the terms of the Creative Commons Attribution License (CC BY). The use, distribution or reproduction in other forums is permitted, provided the original author(s) and the copyright owner(s) are credited and that the original publication in this journal is cited, in accordance with accepted academic practice. No use, distribution or reproduction is permitted which does not comply with these terms.
*Correspondence: Marcel H. N. Hoefnagel, bWguaG9lZm5hZ2VsQGNiZy1tZWIubmw=
Disclaimer: All claims expressed in this article are solely those of the authors and do not necessarily represent those of their affiliated organizations, or those of the publisher, the editors and the reviewers. Any product that may be evaluated in this article or claim that may be made by its manufacturer is not guaranteed or endorsed by the publisher.
Research integrity at Frontiers
Learn more about the work of our research integrity team to safeguard the quality of each article we publish.