- 1Department of Molecular Microbiology and Immunology, Bloomberg School of Public Health, Johns Hopkins University, Baltimore, MD, United States
- 2FOCUS Health Group, Naturopathic, Novato, CA, United States
- 3California Center for Functional Medicine, Kensington, CA, United States
Lyme disease is the most common vector-borne disease in the US and Europe. Although the current recommended Lyme antibiotic treatment is effective for the majority of Lyme disease patients, about 10–20% of patients continue to suffer from persisting symptoms. There have been various anecdotal reports on the use of herbal extracts for treating patients with persisting symptoms with varying degree of improvements. However, it is unclear whether the effect of the herb products is due to their direct antimicrobial activity or their effect on host immune system. In the present study, we investigated the antimicrobial effects of 12 commonly used botanical medicines and three other natural antimicrobial agents for potential anti-Borrelia burgdorferi activity in vitro. Among them, 7 natural product extracts at 1% were found to have good activity against the stationary phase B. burgdorferi culture compared to the control antibiotics doxycycline and cefuroxime. These active botanicals include Cryptolepis sanguinolenta, Juglans nigra (Black walnut), Polygonum cuspidatum (Japanese knotweed), Artemisia annua (Sweet wormwood), Uncaria tomentosa (Cat's claw), Cistus incanus, and Scutellaria baicalensis (Chinese skullcap). In contrast, Stevia rebaudiana, Andrographis paniculata, Grapefruit seed extract, colloidal silver, monolaurin, and antimicrobial peptide LL37 had little or no activity against stationary phase B. burgdorferi. The minimum inhibitory concentration (MIC) values of Artemisia annua, Juglans nigra, and Uncaria tomentosa were quite high for growing B. burgdorferi, despite their strong activity against the non-growing stationary phase B. burgdorferi. On the other hand, the top two active herbs, Cryptolepis sanguinolenta and Polygonum cuspidatum, showed strong activity against both growing B. burgdorferi (MIC = 0.03–0.06% and 0.25–0.5%, respectively) and non-growing stationary phase B. burgdorferi. In subculture studies, only 1% Cryptolepis sanguinolenta extract caused complete eradication, while doxycycline and cefuroxime and other active herbs could not eradicate B. burgdorferi stationary phase cells as many spirochetes were visible after 21-day subculture. Further studies are needed to identify the active constituents of the effective botanicals and evaluate their combinations for more effective eradication of B. burgdorferi in vitro and in vivo. The implications of these findings for improving treatment of persistent Lyme disease are discussed.
Introduction
Lyme disease, caused by Borrelia burgdorferi, and multiple closely related Borrelia species, is the most common vector-borne human disease in the Northern Hemisphere (1, 2). About 300,000 new cases are diagnosed in the United States annually (3, 4). Tick-borne infections are on the rise in the USA and Europe due to a host of different factors including climate change (5, 6) and disruption of predator density in suburban areas (7). Recent studies on tick prevalence and pathogen load have identified new geographical areas where vector ticks are present (8), as well as novel tick-borne pathogens present in areas where they had not previously been identified (such as B. miyamotoi in Northern California) (9).
Lyme disease can affect many different body systems and organs (10). While many patients recover fully with early antibiotic therapy, at least 10–20% of patients experience persistent symptoms following the conventionally recommended course of 2–4 weeks of antibiotics (11, 12), and a recent retrospective analysis documented 63% of patients experienced persistent symptoms after receiving antibiotic treatment for Lyme disease (13). Patients who experience persistent symptoms can have significant and ongoing disability (11, 14) and increased health care costs and utilization (13). B. burgdorferi can evade the immune system response (15, 16) and multiple studies have shown that the bacteria is capable of persisting in diverse tissues across a variety of animal models despite aggressive and prolonged antibiotic therapy (17–19).
In addition to the mammalian studies noted above, B. burgdorferi persistence following antibiotic treatment has been demonstrated in human studies and case reports (20–23). Persistent Lyme borreliosis symptoms significantly affect quality of life (24, 25), therefore some physicians treat these patients with extended courses of antibiotics. However, this approach is controversial with one medical society guideline (26) advocating against retreating patients with persistent (>6 months) symptoms and another medical society guideline (27) recommending individualized risk-benefit assessments and potential retreatment or longer duration treatment of patients with persistent symptoms. While antibiotic retreatment has been associated with improved clinical outcomes (27, 28), antibiotic therapy appears to be more effective against the actively dividing spirochete form. In addition, it has been shown that B. burgdorferi can change morphology and form biofilm-like microcolonies consisting of stationary phase persister bacteria (29–31). Traditional antibiotics have poor activity against the atypical persister forms (round bodies, microcolonies, and biofilm) and we have previously worked to identify novel drugs and drug combinations that are effective against these atypical forms (29, 30, 32). While Daptomycin and Dapsone have been identified as having significant effects against borrelia persister cells in vitro (29, 33) and in vivo in a murine model (31), their use in clinical practice can be limited by side effects (both), cost (daptomycin), parenteral administration (daptomycin), and poor CNS penetration (daptomycin) (34). Given the limitations of current Lyme treatment it is of vital importance that novel, safe, and effective therapies be identified for clinical use.
Importantly, botanical medicines have been shown to have in vitro antimicrobial activity against various morphologic forms of B. burgdorferi. Because there are a limited number of studies evaluating the effects of botanical medicine on B. burgdorferi, it is helpful to draw on clinical studies that have shown benefit using botanical medicines for other spirochetal infections and infections like mycobacterium that are known to form antibiotic tolerant persister cells (35). For example, Andrographis has been shown to effectively treat leptospirosis in Chinese clinical trials (36) and improve clinical outcomes when combined with standard treatment for tuberculosis (37).
Botanical medicine has a long history of use, beginning almost 5,000 years ago in Mesopotamia and has over 3,000 years of documented usage in China (38). The safety of botanical medicines has been documented in various traditional systems of medicine such as Ayurvedic Medicine and Traditional Chinese Medicine over centuries. Recent retrospective and systematic reviews in the European Union and South America have concluded severe adverse events associated with Botanical Medicine usage were rare (39, 40).
This study builds on previous studies that used our in vitro stationary phase persister model and SYBR Green I/propidium iodide (PI) assay to screen potential antimicrobial candidates. Having previously identified novel drugs and drug combinations from an FDA drug library (32), as well as selected botanicals in essential oil form that have anti-B. burgdorferi activity (41, 42), in the present study (Feng et al. https://www.biorxiv.org/content/10.1101/652057v1.full), we investigated the effect of 12 botanical medicines and 3 other natural antimicrobial agents for potential anti-B. burgdorferi activity in vitro.
Materials and Methods
Strain, Media, and Culture Techniques
B. burgdorferi strain B31 was cultured in BSK-H medium (HiMedia Laboratories Pvt. Ltd.) with 6% rabbit serum (Sigma-Aldrich, St. Louis, MO, USA). All culture medium was filter-sterilized by 0.2 μm filter. Cultures were incubated in sterile 50 ml conical tubes (BD Biosciences, CA, USA) in microaerophilic incubator (33°C, 5% CO2) without antibiotics.
Botanical and Natural Medicines
A panel of natural product extracts: Polygonum cuspidatum, Cryptolepis sanguinolenta, Artemisia annua, Juglans nigra, Uncaria tomentosa, Scutellaria baicalensis, Stevia rebaudiana, Cistus incanus, Andrographis paniculata, Ashwagandha somnifera, Dipsacus fullonum rad, grapefruit seed extract, LL37, monolaurin, colloidal silver, and relevant solvent controls (see Table 1) were identified. The botanical medicines or natural products were chosen based on anecdotal clinical usage and preclinical data from the literature. Primary criteria for selecting compounds for the present study included agents that had shown significant anti-borrelial effects in previous studies, have favorable safety profiles and can be absorbed systemically. Additional criteria for selecting compounds included anecdotal reports from patients and/or providers, anti-biofilm effects and ability to cross the blood brain barrier.
Botanical medicines were sourced from KW Botanicals (San Anselmo, California) and Heron Botanicals (Kingston, Washington). Botanicals were identified via macroscopic and organoleptic methods and voucher specimens are on file with the respective production facilities. Most botanical medicines were provided as alcohol extracts at 30, 60, and 90% alcohol, and the alcohol used was also tested separately as a control in different dilutions. Monolaurin (Lauricidin™ brand) (dissolved in 100% DMSO), and colloidal silver (Argentyn™ brand) were purchased commercially. LL37 and a control was obtained from Taylor Made Pharmacy in Nicholasville, KY. Citrosept™ (Cintamani, Poland) and Nutribiotic™ grapefruit seed extract products and a control were purchased commercially. See Table 1 for additional details on sourcing, testing, and validation of botanical and natural medicines used.
Doxycycline (Dox) and cefuroxime (CefU) (Sigma-Aldrich, USA) were dissolved in suitable solvents (43) to form 5 mg/ml stock solutions. The antibiotic stocks were filter-sterilized by 0.2 μm filter and stored at −20°C.
Microscopy
B. burgdorferi spirochetes and aggregated microcolonies treated with natural products or control drugs were stained with SYBR Green I and PI (propidium iodide) and checked with BZ-X710 All-in-One fluorescence microscope (KEYENCE, Itasca, IL, USA). The bacterial viability was performed by calculating the ratio of green/red fluorescence to determine the ratio of live and dead cells, as described previously (29). The residual cell viability reading was obtained by analyzing three representative images of the same bacterial cell suspension taken by fluorescence microscopy. To quantitatively determine the bacterial viability from microscope images, Image Pro-Plus software was employed to evaluate fluorescence intensity as described previously (30).
Evaluation of Natural Products for Their Activity Against B. burgdorferi Stationary Phase Cultures
B. burgdorferi B31 was cultured for 7 days in microaerophilic incubator (33°C, 5% CO2) as stationary phase cultures (~107−8 spirochetes/mL). To evaluate potential anti-persister activity of the natural products, their stocks and their control solvents were added to 100 μL of the B. burgdorferi stationary phase culture in 96-well plates to obtain the desired concentrations. The botanical medicines and natural product extracts were tested with the concentration of 1, 0.5, and 0.25% (v/v); antibiotics of daptomycin, doxycycline, and cefuroxime were used as controls at a final concentration of 5 μg/ml. All the tests mentioned above were run in triplicate. The microtiter plates were sealed and incubated at 33°C without shaking for 7 days with 5% CO2.
Subculture Studies to Confirm the Activity of the Top Natural Product Hits
For the subculture study, 1 mL B. burgdorferi stationary phase culture was treated by natural products or control drugs in 1.5 ml Eppendorf tubes for 7 days at 33°C without shaking. Next, cells were centrifuged, and cell pellets were washed with fresh BSK-H medium (1 mL) followed by resuspension in fresh BSK-H medium without antibiotics. Then 50 μl of cell suspension was inoculated into 1 ml of fresh BSK-H medium for subculture at 33°C, 5% CO2. Cell growth was monitored using SYBR Green I/PI assay and fluorescence microscopy after 7–20 days.
Results
Evaluation of Activity of Natural Product Extracts Against Stationary Phase B. burgdorferi
We tested a panel of botanical medicines and natural product extracts and their corresponding controls against a 7-day old B. burgdorferi stationary phase culture in 96-well plates incubated for 7 days. Table 2 summarizes the activity of these natural product extracts against the stationary phase B. burgdorferi culture at 1, 0.5, and 0.25%. Among them, 7 natural product extracts at 1% were found to have strong activity against the stationary phase B. burgdorferi culture compared to the control antibiotics doxycycline and cefuroxime (Table 2). To eliminate auto-fluorescence background, we checked the ratio of residual live cells and dead cells by examining microscope images as described previously (30). Using fluorescence microscopy, we confirmed that 1% Cryptolepis sanguinolenta, Juglans nigra, and Polygonum cuspidatum could eradicate almost all live cells with only dead and aggregated cells left as shown in Figure 1. At 0.5% concentration, 11 natural product extracts (Polygonum cuspidatum 60% EE, Cryptolepis sanguinolenta 60% EE, Artemisia annua 90% EE, Juglans nigra 30–60% EE, Uncaria tomentosa WE, Artemisia annua 60% EE, Polygonum cuspidatum 90% EE, Scutellaria baicalensis) still exhibited stronger activity than the current clinically used doxycycline and cefuroxime (Table 2 and Figure 1). Among them, the most active natural product extracts were Cryptolepis sanguinolenta 60% EE, Polygonum cuspidatum 60% EE, Artemisia annua 90% EE, Juglans nigra 60% EE, Uncaria tomentosa WE, Artemisia annua 60% EE, because of their outstanding activity even at 0.25%, as shown by better activity than control drugs (Table 2 and Figure 1). In particular, 0.25% Cryptolepis sanguinolenta could eradicate or dissolve all the B. burgdorferi cells including aggregated forms as we found rare live and even dead cells with SYBR Green I/PI microscope observation (Figure 1). Although Juglans nigra could eradicate almost all stationary phase B. burgdorferi cells at 0.5% (Figure 1), it could not kill the aggregated microcolony form at 0.25% as shown by many live (green) microcolonies by SYBR Green I/PI microscopy. Although the plate reader data showed Polygonum cuspidatum 60% ethanol extract had the strongest activity at 0.25%, the microscope result did not confirm it due to higher residual viability than that of Cryptolepis sanguinolenta and Juglans nigra (Figure 1).
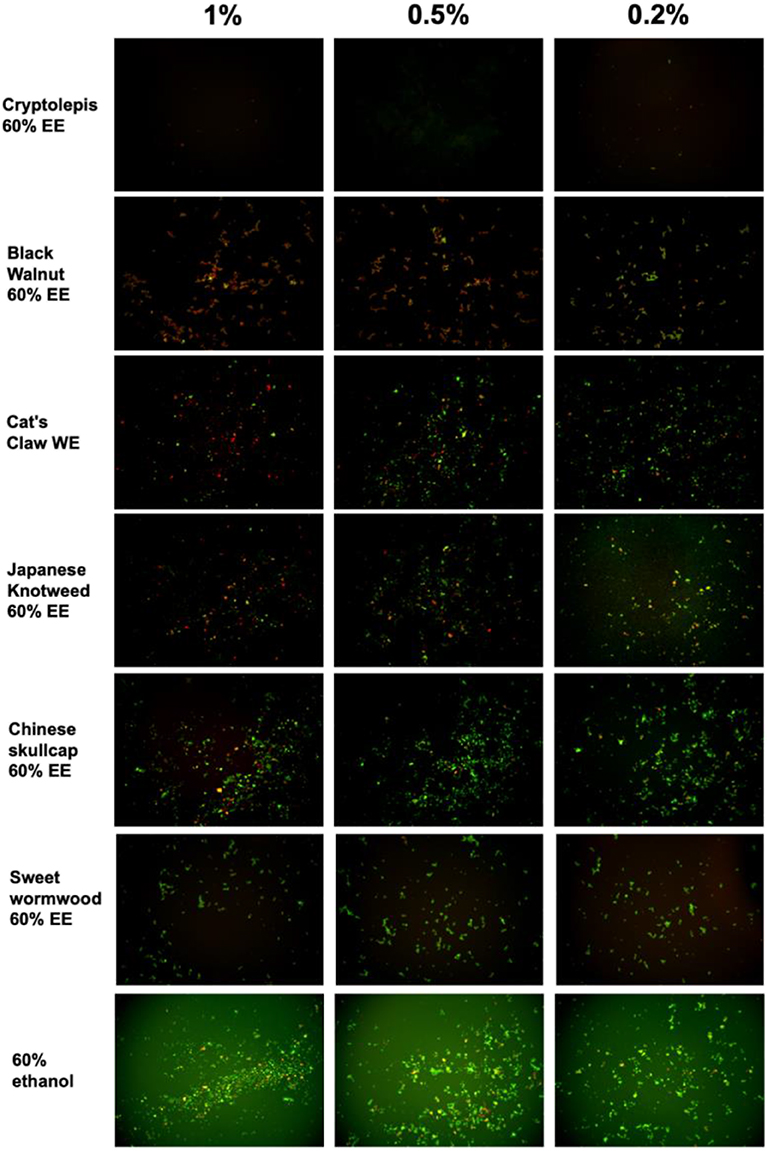
Figure 1. Effect of natural product extracts on the viability of stationary phase B. burgdorferi. A 7-day old B. burgdorferi stationary phase culture was treated with the natural product extracts at 1, 0.5, and 0.2% for 7 days followed by staining with SYBR Green I/PI viability assay and fluorescence microscopy.
We also tested several other herbs and substances that are used by Lyme patients including Stevia rebaudiana, Andrographis paniculata, Grapefruit seed extract, Ashwagandha somnifera, Colloidal silver, Lauricidin, and antimicrobial peptide LL-37, but found they had little or no activity against stationary phase B. burgdorferi cells.
MIC Values of the Active Natural Product Extracts
Because the activity of antibiotics against non-growing B. burgdorferi is not always correlated with their activity against growing bacteria (30), we therefore determined the MICs of these natural product extracts against the replicating B. burgdorferi as described previously (32). The MIC values of some natural product extracts such as Artemisia annua, Juglans nigra, Uncaria tomentosa were quite high for growing B. burgdorferi, despite their strong activity against the non-growing stationary phase B. burgdorferi cells (Table 2). On the other hand, the top two active natural product extracts Cryptolepis sanguinolenta and Polygonum cuspidatum showed strong activity against the growing B. burgdorferi with a low MIC (0.03–0.06% and 0.25–0.5% respectively) and also non-growing stationary phase B. burgdorferi (Table 2).
Subculture Studies to Evaluate the Activity of Natural Product Extracts Against Stationary Phase B. burgdorferi
To confirm the activity of the natural product extracts in eradicating the stationary phase B. burgdorferi cells, we performed subculture studies as previously described (30). We further tested the top active natural product extracts (Cryptolepis sanguinolenta, Polygonum cuspidatum, Artemisia annua, Juglans nigra, and Scutellaria baicalensis) to ascertain if they could eradicate stationary phase B. burgdorferi cells at 1 or 0.5% by subculture after the treatment (Table 2). Treatment with 1% Cryptolepis sanguinolenta extract caused no regrowth in the subculture study (Table 2 and Figure 2). However, the other natural product extracts including Polygonum cuspidatum, Artemisia annua, Juglans nigra, and Uncaria tomentosa could not eradicate B. burgdorferi stationary phase cells as many spirochetes were still visible after 21-day subculture (Table 2 and Figure 2). At 0.5%, all the natural product extracts treated samples grew back after 21-day subculture (Table 2 and Figure 2), however, only one of the three Cryptolepis sanguinolenta extract treated samples grew back. This indicates that 0.5% Cryptolepis sanguinolenta extract still has strong activity and could almost eradicate the stationary phase B. burgdorferi cells. By contrast, the clinically used antibiotics doxycycline and cefuroxime at clinically relevant concentration (5 μg/ml) could not sterilize the B. burgdorferi stationary phase culture, since spirochetes were visible after 21-day subculture (Table 2).
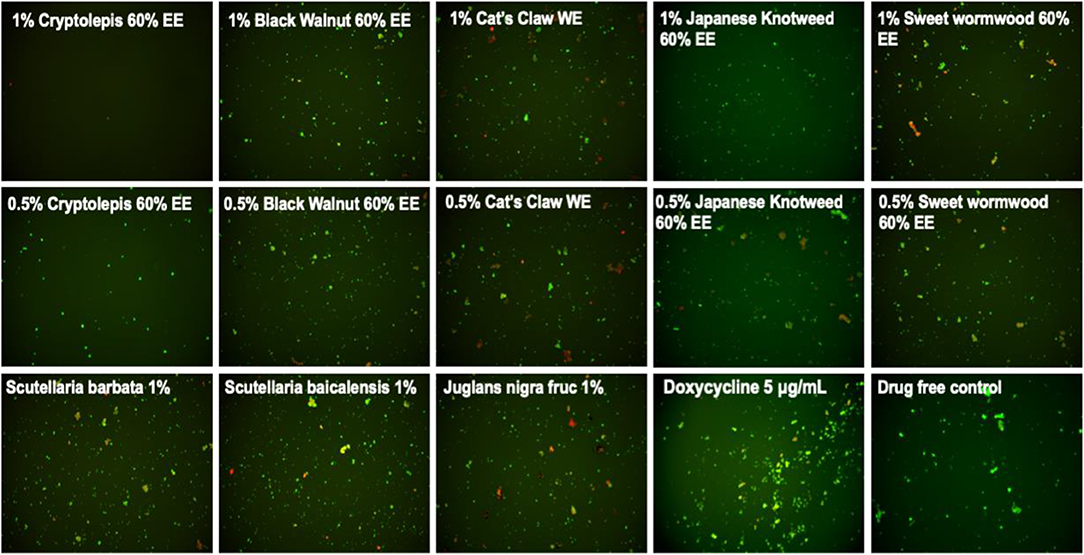
Figure 2. Subculture of Borrelia burgdorferi after treatment with natural product extracts. A 7-day stationary phase B. burgdorferi culture was treated with the indicated natural product extracts for 7 days followed by washing and resuspension in fresh BSK-H medium and subculture for 21 days. The viability of the subculture was examined by SYBR Green I/PI stain and fluorescence microscopy.
Discussion
In this study, we evaluated a panel of botanical medicines and natural products commonly used by some patients to manage their persisting symptoms of Lyme disease and found that indeed some of them have strong activity against B. burgdorferi. These include Cryptolepis sanguinolenta, Polygonum cuspidatum, Juglans nigra, Artemisia annua, Uncaria tomentosa, Cistus incanus, and Scutellaria baicalensis. The antimicrobial activities of these 7 active herbs are presented in Supplementary Table 1. These findings may provide a basis for the clinical improvement of patients who take these medicines and also indirectly suggest their persisting symptoms may be due to persistent bacteria that are not killed by conventional Lyme antibiotic treatment. Surprisingly, Andrographis paniculata, Stevia rebaudiana (44),Colloidal silver (Argentyn 23™), Monolaurin (Lauricidin™), Dipsacus spp., and Withania somnifera, which are assumed or previously reported to have anti-borrelia activity, did not show significant activity against either stationary phase or growing B. burgdorferi in this study.
Cryptolepis sanguinolenta is a plant indigenous to Africa where it has been used in traditional medicine to treat malaria, tuberculosis, hepatitis, and septicemia (45). Cryptolepis sanguinolenta has been shown in preclinical studies to have anti-inflammatory (46, 47) antibacterial (48–50), anti-fungal (51), anti-amoebic (52), and anti-malarial (53, 54) properties. Two preliminary clinical studies have documented significant efficacy in treating uncomplicated malaria without signs of overt toxicity (55). While multiple secondary metabolites with antimicrobial activity have been identified, an alkaloid called cryptolepine has been the most well-studied to date. Cryptolepine's antimicrobial activity is thought to be secondary to multiple mechanisms of action including both bactericidal and bacteriostatic effects (48). More specifically, cryptolepine has been shown to cause morphologic changes and cellular breakdown (51), as well as DNA intercalating and topoisomerase II inhibiting effects (56, 57). It should be noted that, in addition to cryptolepine, other constituents in Cryptolepis sanguinolenta have also been shown to have antimicrobial activity (58).
Cryptolepis sanguinolenta is generally well-tolerated and few side effects have been documented in humans during its relatively long-term use in parts of China and India. Rat studies indicate that doses of the extract up to 500 mg/kg are relatively safe (59). Importantly, a novel finding of this current study is the fact that Cryptolepis sanguinolenta has strong activity against growing B. burgdorferi with low MIC and also non-growing stationary phase B. burgdorferi (Table 2 and Figures 1, 2). Given its traditional use against malaria, in the Lyme treatment community Cryptolepis sanguinolenta has been used for treatment of Babesia spp. (60) which can be a co-infecting malaria like organism. To our knowledge, the anti-Borrelial effect of Cryptolepis sanguinolenta has not previously been documented and further in vitro and in vivo studies are warranted to investigate the potential role Cryptolepis sanguinolenta may serve in the treatment of Lyme disease.
Juglans nigra and its constituents have been shown to have antioxidant, antibacterial, antitumor and chemoprotective effects (61, 62). Previous in vitro testing has documented that Juglans nigra exhibited bacteriostatic activity against log phase spirochetes of B. burgdorferi and B. garinii and bactericidal activity against Borrelia round bodies (63). Two different commercially available botanical formulations which contain Juglans nigra were also recently shown to have activity against log phase spirochetes of B. burgdorferi strain GCB726, round bodies and biofilm formation in in vitro testing (64). Juglans nigra has also been shown to have multiple constituents (65) with antimicrobial properties including juglone (5-hydroxy-1,4-naphthalenedione), phenolic acids, flavonoids, and catechins (including epigallocatechin gallate) (66, 67). Further studies are needed to elucidate which constituents have anti-borrelial activity. Juglans nigra is well-tolerated and side effects are uncommon. In some individuals, it can cause gastrointestinal disturbance (68) and induce changes in skin pigmentation (69, 70). There can be some allergic cross reactivity in those allergic to tree nuts or walnuts, as well as cases of dermatitis reported in humans (71). The active compound juglone was found to have an oral LD50 in rats of 112 mg/kg (72).
Polygonum cuspidatum has documented anti-tumor, antimicrobial, anti-inflammatory, neuroprotective, and cardioprotective effects (73, 74), with the polyphenol resveratrol being one of the main active constituents. Previous in vitro testing has documented that resveratrol exhibited activity against log phase spirochetes of Borrelia burgdorferi and Borrelia garinii, minimal activity against borrelia round bodies, and no significant activity against borrelia associated biofilms (63). Another active constituent, Emodin (6-methyl-1,3,8-trihydroxyanthraquinone), has documented activity against stationary phase B. burgdorferi cells (75). Additionally, preclinical research has documented additional antibacterial and anti-biofilm effects (76, 77). The antibacterial activity of P. cuspidatum has been attributed to its stilbenes (including resveratrol) and hydroxyanthraquinone content (78). Polygonum cuspidatum has been found to have minimal toxicity in animal and human studies. Gastrointestinal upset and diarrhea can occur but resolves with decreasing or stopping the intake (79). While few studies have been performed in humans, a 2010 review found that it is well-absorbed, and rapidly metabolized.
Artemisia annua (Sweet wormwood also called Chinese wormwood and Qing Hao) is a medicinal plant that has been used for medicinal purposes for over 2,000 years (80) and the isolation of an active constituent called artemisinin was awarded the Nobel Prize in 2015 for its role in treating malaria (81). Artemisinin also has prior documented activity against stationary phase B. burgdorferi persisters in in vitro models (32, 82). Furthermore, a small pilot study demonstrated that a synthetic analog to artemisinin, called artesunate, showed a significant reduction in short term memory impairment in patients with Lyme disease when combined with intravenous ceftriaxone (83). Artemisinin's antimicrobial mechanism of action is not completely understood (84), but is thought to be related to its ability to generate free radicals that damage proteins (85, 86). The artemisinin content of the Artemisia annua sample used in the present study was confirmed to be 0.11% by high-performance liquid chromatography/UV-visual spectroscopy at the Institute for Food Safety and Defense (Centralia, WA). High quality Artemisia annua should generally contain >0.3% artemisinin. Despite potential suboptimal levels of artemisinin present in the Artemisia annua used for the present study, both 60 and 90% alcohol extracts of Artemisia annua exhibited better activity against stationary phase B. burgdorferi compared to the control antibiotics cefuroxime and doxycycline. One explanation for these results could be that constituents other than artemisinin are important in providing antimicrobial effects, a finding supported by prior studies (59, 87). Artemisia annua is generally considered safe provided that the product administered has minimal or no thujone and other terpene derivatives that are potentially neurotoxic (88). Rat studies found that the NOAEL (no-observed-adverse-effect-level) of Artemisia annua extract was estimated to be equivalent to 1.27 g/kg/day in males and 2.06 g/kg/day in females) or more (89). In humans, Artemisia annua has been used safely in doses up to 2,250 mg daily for up to 10 weeks (88), and 1,800 mg daily have also been used safely for up to 6 months (88). Some gastrointestinal upset including mild nausea, vomiting (more rare), and abdominal pain can occur at higher doses (60). The use of whole plant extracts instead of single constituents offers potential advantages including providing multiple mechanisms of action and synergistic effects that can reduce the risk of developing microbial resistance. An emerging example of this can be seen in malaria treatment where significant resistance has been reported with artemisinin-based combination therapy (ACT) (90, 91), whereas preliminary studies show improved efficacy and reduced side-effects when treatment with the whole Artemisia plant is used (87, 92).
Scutellaria baicalensis and its constituents have been shown to have neuroprotective, antioxidant, anti-apoptotic, anti-inflammatory, and anti-excitotoxicity activity (93–96). One of the active constituents found in Scutellaria baicalensis, baicalein, was found to exhibit in vitro activity against various morphologic forms of B. burgdorferi and B. garinii, including log phase spirochetes, latent round bodies, and biofilm formations (97). Additional research has further documented antimicrobial activity (98), synergistic effects with antibiotics (99–101), and reduced biofilm formation (102). Scutellaria baicalensis has documented clinical safety (103, 104). There are reports of sedation and it has been shown to be active on the GABA receptor sites (105, 106). A medical food combination of purified Scutellaria baicalensis and the bark of Acacia catechu containing concentrated baicalin and catechin (Limbrel™, Move Free Advanced™) caused reversible liver damage in at least 35 cases, with a calculated estimated incidence of approximately 1 in 10,000 (107). Despite the case reports of hepatotoxicity, a dose of 1,000 mg/kg daily was identified as the no-observed-adverse-effect level (NOAEL) for this commercial product (108). Hepatotoxicity is generally not seen from the whole plant extract and in a recent study no hepatotoxicity was found in patients taking 1,335 mg per day for an average of 444 days (109).
Uncaria tomentosa has documented neuroprotective effects in preclinical studies (110), and preliminary human studies have shown improved quality of life in individuals with cancer (111), enhanced DNA repair (112), and symptom improvement in individuals with rheumatoid arthritis (113) and osteoarthritis (114). The potential antimicrobial effects of Uncaria tomentosa have not been widely evaluated. In a non-peer reviewed publication, Uncaria tomentosa was reported to have anti-borrelial effects in an in vitro model (115). Uncaria tomentosa has also been shown in peer reviewed research to have antimicrobial effects against human oral pathogens (116). Uncaria tomentosa has been found to be safe and to have minimal side effects in a variety of animal and human studies (112). Human studies ranging from 4 weeks (114) to 52 weeks (113) demonstrated side effects comparable to placebo. While gastrointestinal complaints such as nausea, diarrhea, abdominal pain, and anemia, were reported, it was thought that study patients had experienced health issues from their solid tumor disease progression and not necessarily from the Uncaria (111). The acute median lethal dose in mice was >16 g/kg body weight (117).
It has been proposed that Cistus incanus and Cistus creticus are synonymous (www.theplantlist.org) while other sources have suggested that Cistus creticus is a subspecies of Cistus incanus (118). Preliminary clinical studies have shown significant improvement in upper respiratory infection and inflammatory markers in patients taking Cistus incanus (119), a volatile oil extract of Cistus creticus has been shown to have anti-borrelial effects in an in vitro model (120). Additional in vitro studies have documented the antimicrobial effects of Cistus creticus against several bacteria (118, 121). Cistus creticus also demonstrated significant inhibition of Streptococcus mutans biofilm formation (121) and reduction in bacterial adherence to enamel (122). Cistus creticus has been shown to contain several active constituents (123), including carvacrol (120). Given that our lab previously documented carvacrol to have a significant activity against log and stationary phase B. burgdorferi cells (41), it is possible that the carvacrol content in the Cistus incanus sample tested in the present study contributed to the significant reduction in log and stationary phase B. burgdorferi cells in the present study. Cistus incanus plant extracts have been used for centuries in traditional medicine without reports of side effects or allergic reactions (124). In a randomized placebo-controlled study of 160 patients, 220 mg per day Cistus incanus was well-tolerated with less adverse effects than in the placebo group (119). While pharmacokinetic safety data is sparse, a cell culture study showed that Cistus incanus did not cause any adverse changes on cell proliferation, survival, or cellular receptor function (124).
Grapefruit seed extract (GSE) was previously reported to have in vitro activity against motile and cystic morphologic forms of borrelia bacteria in an in vitro model (125). In contrast, the current study did not demonstrate meaningful activity against B. burgdorferi. There are several potential reasons to explain the difference in results between the current study and previous study including differences in GSE formulations and/or different borrelia species used in culture. In the current study we used B. burgdorferi strain B31 whereas the 2007 study states that “B. afzelii ACA-1” was used. While both studies used Citrosept™ brand GSE, the formulation has been modified and currently holds an “organic” designation. Because previous studies have documented several contaminants in commercial GSE formulations, including Benzalkonium chloride, triclosan, and methylparaben (126, 127), we screened the GSE products for contaminants prior to inclusion in our present study. The Citrosept™ sample was found to have no detectable levels of contaminants and therefore was used as the GSE source in the current study. In contrast, a second commercially available brand of GSE (Nutribiotic™) did test positive for elevated levels of Benzalkonium chloride, which is a known antimicrobial compound (128) and has been implicated in drug-herb interactions causing potential safety concerns for patients taking GSE (129). The 2007 study did not note testing for contaminants, so it is possible that the previous formulation of Citrosept™ contained a contaminant that exerted anti-borrelial activity.
Stevia rebaudiana was recently reported to have strong anti-borrelia activity (44). However, in our testing, Stevia rebaudiana failed to show any activity against B. burgdorferi. One possibility to explain this discrepancy is that the study that reported Stevia rebaudiana having activity against B. burgdorferi did not have appropriate alcohol control. Hypothetically, the previously documented anti-borrelial effect seen may have been due to a non-specific alcohol effect on the Borrelia bacteria and not due to Stevia rebaudiana itself, or due to differences in plant species, growing conditions, or how the herb is processed. Since we obtained our Stevia rebaudiana preparation from an experienced herbalist who extracted it using a known concentration of alcohol, we worked with a preparation with known alcohol concentration. When we used proper alcohol controls we did not find Stevia rebaudiana to have any activity against B. burgdorferi (Table 2).
Andrographis paniculata has been used to treat the spirochetal infection leptospirosis (36) and is anecdotally used by patients with Lyme Disease (60). However, we found Andrographis failed to show any activity against B. burgdorferi in our testing. It is possible that Andrographis indirectly acts on the host immune system to kill B. burgdorferi or induces a non-specific host response. Further studies are needed to test the possible effect of Andrographis on the host immune cells.
While this current study has identified novel new botanical and natural medicines with in vitro anti-Borrelia activity, it is also notable that many herbs or compounds tested did not show direct anti-Borrelia activity despite the fact that they are widely used, with anecdotal reports of clinical effectiveness, by patients and practitioners in the community setting (https://www.lymedisease.org/mylymedata-alternative-lyme-disease-treatment/) (60). It is important to consider the potential limitations of the in vitro model given that it exists outside of the biological organism. The in vitro model can provide information on direct antimicrobial activity, and while this can be part of the function of botanical and natural medicines, they can also function via additional diverse pathways. For example, they can exert effects via anti-inflammatory/anti-cytokine activity, immune system regulation/augmentation, adaptogenic stimulation of cellular, and organismal defense systems, and biofilm disruption to name a few. In these activities, the mechanisms of the medicines rely on complex interplay and interaction between different body systems, which can only occur within the living organism. Because the in vitro model is unable to provide information with regards to alternative pathways through which natural botanical medicines act, it is important that future in vivo studies be performed to investigate the activity and efficacy of these and other botanical and natural medicines against Borrelia and other tick-borne diseases. These types of studies will be of vital importance given the multiple factors at play with the current epidemic of tick-borne diseases in our society and globally. While research is beginning to provide information on novel antibiotic combinations that might be effective against the multiple forms of the Borrelia bacteria (31), there is ongoing concern regarding extended antibiotic use and care is required regarding issues of responsible stewardship of antibiotic use and antibiotic resistance. It is also important to recognize that, while being cognizant of specific side effects and interactions, botanical and natural medicines generally have a favorable safety profile compared to prescription antibiotics and have a broader spectrum of action with multiple synergistic compounds present within a single plant. Furthermore, using multiple botanical medicines in combination can further increase synergy and efficacy and lower the risk of pathogen resistance development.
Conclusion
In conclusion, we tested a panel of botanical and natural products that are commonly used by Lyme disease patients and found several to be highly active in vitro against stationary phase B. burgdorferi including Cryptolepsis sanguinolenta, Juglans nigra, Polygonum cuspidatum, Uncaria tomentosa, Artemisia annua, Cistus creticus, and Scutellaria baicalensis. In contrast, we found that Stevia rebaudiana, Andrographis paniculata, Grapefruit seed extract, colloidal silver, monolaurin, and antimicrobial peptide LL37 had little or no activity against B. burgdorferi in our in vitro model.
Since traditional antibiotic approaches fail to resolve all symptoms in a subset of patients treated for Lyme disease, there is a need for developing novel treatment strategies including identifying antimicrobial agents that are effective against persister microcolonies of B. burgdorferi. Future studies are needed to further evaluate the seven active botanical medicines identified in the present study as having better activity than doxycycline and cefuroxime against stationary phase B. burgdorferi. Specifically, studies should be directed at identifying the active constituents of each botanical, evaluating synergistic combinations, and confirming safety and efficacy in animal models and subsequent clinical studies.
Data Availability Statement
All data sets generated for this study are included in the article/Supplementary Material.
Author Contributions
YZ, JF, JL, and SS conceived the experiments, analyzed the data, and wrote the paper. JF performed the experiments.
Conflict of Interest
JL is owner of two naturopathic medical practices, FOCUS Health Group and Door One Concierge, which provides treatment to patients with tick-borne diseases. JL does receive profits from medical services and botanical preparations he exclusively makes available to patients in these two practices and does not currently sell botanical products commercially.
The remaining authors declare that the research was conducted in the absence of any commercial or financial relationships that could be construed as a potential conflict of interest.
Acknowledgments
We thank herbalists Eric Yarnell ND and Brian Kie Weissbuch for providing botanical extracts for evaluation in this study and for helpful discussions. We would like to thank Mischa Grieder ND for lending his expertise and helpful discussions. We also gratefully acknowledge the support of this work by the Bay Area Lyme Foundation and the Steven & Alexandra Cohen Foundation.
Supplementary Material
The Supplementary Material for this article can be found online at: https://www.frontiersin.org/articles/10.3389/fmed.2020.00006/full#supplementary-material
References
1. Stanek G, Reiter M. The expanding Lyme Borrelia complex–clinical significance of genomic species? Clin Microbiol Infect. (2011) 17:487–93. doi: 10.1111/j.1469-0691.2011.03492.x
2. Long KC, Cohn KA. Lyme arthritis: an update for clinical practice. Pediatr Emerg Care. (2018) 34:588–91. doi: 10.1097/PEC.0000000000001576
3. Hinckley AF, Connally NP, Meek JI, Johnson BJ, Kemperman MM, Feldman KA, et al. Lyme disease testing by large commercial laboratories in the United States. Clin Infect Dis. (2014) 59:676–81. doi: 10.1093/cid/ciu397
4. CDC. Lyme Disease. (2017). Available online at: http://www.cdc.gov/lyme/ (accessed June 13, 2018).
5. Stone BL, Tourand Y, Brissette CA. Brave new worlds: the expanding universe of lyme disease. Vector Borne Zoonotic Dis. (2017) 17:619–29. doi: 10.1089/vbz.2017.2127
6. Lieske DJ, Lloyd VK. Combining public participatory surveillance and occupancy modelling to predict the distributional response of Ixodes scapularis to climate change. Ticks Tick Borne Dis. (2018) 9:695–706. doi: 10.1016/j.ttbdis.2018.01.018
7. Ostfeld RS, Levi T, Keesing F, Oggenfuss K, Canham CD. Tick-borne disease risk in a forest food web. Ecology. (2018) 99:1562–73. doi: 10.1002/ecy.2386
8. Nieto NC, Porter WT, Wachara JC, Lowrey TJ, Martin L, Motyka PJ, et al. Using citizen science to describe the prevalence and distribution of tick bite and exposure to tick-borne diseases in the United States. PLoS ONE. (2018) 13:e0199644. doi: 10.1371/journal.pone.0199644
9. Salkeld DJ, Nieto NC, Carbajales-Dale P, Carbajales-Dale M, Cinkovich SS, Lambin EF. Disease risk & landscape attributes of tick-borne borrelia pathogens in the San Francisco Bay Area, California. PLoS ONE. (2015) 10:e0134812. doi: 10.1371/journal.pone.0134812
10. Steere AC, Strle F, Wormser GP, Hu LT, Branda JA, Hovius JW, et al. Lyme borreliosis. Nat Rev Dis Primers. (2016) 2:16090. doi: 10.1038/nrdp.2016.90
11. Aucott JN. Posttreatment Lyme disease syndrome. Infect Dis Clin North Am. (2015) 29:309–23. doi: 10.1016/j.idc.2015.02.012
12. CDC. Post-Treatment Lyme Disease Syndrome. (2017). Available online at: http://www.cdc.gov/lyme/postLDS/index.html (accessed July 3, 2018).
13. Adrion ER, Aucott J, Lemke KW, Weiner JP. Health care costs, utilization and patterns of care following Lyme disease. PLoS ONE. (2015) 10:e0116767. doi: 10.1371/journal.pone.0116767
14. Johnson L, Aylward A, Stricker RB. Healthcare access and burden of care for patients with Lyme disease: a large United States survey. Health Policy. (2011) 102:64–71. doi: 10.1016/j.healthpol.2011.05.007
15. Tracy KE, Baumgarth N. Borrelia burgdorferi manipulates innate and adaptive immunity to establish persistence in rodent reservoir hosts. Front Immunol. (2017) 8:116. doi: 10.3389/fimmu.2017.00116
16. Bernard Q, Smith AA, Yang X, Koci J, Foor SD, Cramer SD, et al. Plasticity in early immune evasion strategies of a bacterial pathogen. Proc Natl Acad Sci USA. (2018) 115:E3788–97. doi: 10.1073/pnas.1718595115
17. Straubinger RK, Summers BA, Chang YF, Appel MJ. Persistence of Borrelia burgdorferi in experimentally infected dogs after antibiotic treatment. J Clin Microbiol. (1997) 35:111–6. doi: 10.1128/JCM.35.1.111-116.1997
18. Hodzic E, Imai D, Feng S, Barthold SW. Resurgence of persisting non-cultivable Borrelia burgdorferi following antibiotic treatment in mice. PLoS ONE. (2014) 9:e86907. doi: 10.1371/journal.pone.0086907
19. Embers ME, Hasenkampf NR, Jacobs MB, Tardo AC, Doyle-Meyers LA, Philipp MT, et al. Variable manifestations, diverse seroreactivity and post-treatment persistence in non-human primates exposed to Borrelia burgdorferi by tick feeding. PLoS ONE. (2017) 12:e0189071. doi: 10.1371/journal.pone.0189071
20. Hudson BJ, Stewart M, Lennox VA, Fukunaga M, Yabuki M, Macorison H, et al. Culture-positive Lyme borreliosis. Med J Aust. (1998) 168:500–2. doi: 10.5694/j.1326-5377.1998.tb141415.x
21. Oksi J, Marjamaki M, Nikoskelainen J, Viljanen MK. Borrelia burgdorferi detected by culture and PCR in clinical relapse of disseminated Lyme borreliosis. Ann Med. (1999) 31:225–32. doi: 10.3109/07853899909115982
22. Marques A, Telford SR 3rd, Turk SP, Chung E, Williams C, Dardick K, et al. Xenodiagnosis to Detect Borrelia burgdorferi infection: a first-in-human study. Clin Infect Dis. (2014) 58:937–45. doi: 10.1093/cid/cit939
23. Middelveen MJ, Sapi E, Burke J, Filush KR, Franco A, Fesler MC, et al. Persistent Borrelia infection in patients with ongoing symptoms of Lyme disease. Healthcare. (2018) 6:E33. doi: 10.3390/healthcare6020033
24. Johnson L, Wilcox S, Mankoff J, Stricker RB. Severity of chronic Lyme disease compared to other chronic conditions: a quality of life survey. PeerJ. (2014) 2:e322. doi: 10.7717/peerj.322
25. Rebman AW, Bechtold KT, Yang T, Mihm EA, Soloski MJ, Novak CB, et al. The clinical, symptom, and quality-of-life characterization of a well-defined group of patients with posttreatment Lyme disease syndrome. Front Med. (2017) 4:224. doi: 10.3389/fmed.2017.00224
26. Wormser GP, Dattwyler RJ, Shapiro ED, Halperin JJ, Steere AC, Klempner MS, et al. The clinical assessment, treatment, and prevention of Lyme disease, human granulocytic anaplasmosis, and babesiosis: clinical practice guidelines by the infectious diseases Society of America. Clin Infect Dis. (2006) 43:1089–134. doi: 10.1086/508667
27. Cameron DJ, Johnson LB, Maloney EL. Evidence assessments and guideline recommendations in Lyme disease: the clinical management of known tick bites, erythema migrans rashes and persistent disease. Expert Rev Anti Infect Ther. (2014) 12:1103–35. doi: 10.1586/14787210.2014.940900
28. Delong AK, Blossom B, Maloney E, Phillips SE. Potential benefits of retreatment highlight the need for additional Lyme disease research. Am J Med. (2014) 127:e9–10. doi: 10.1016/j.amjmed.2013.08.028
29. Feng J, Wang T, Shi W, Zhang S, Sullivan D, Auwaerter PG, et al. (2014). Identification of novel activity against Borrelia burgdorferi persisters using an FDA approved drug library. Emerg Microb Infect. (2014) 3:e49. doi: 10.1038/emi.2014.53
30. Feng J, Auwaerter PG, Zhang Y. Drug combinations against Borrelia burgdorferi persisters in vitro: eradication achieved by using daptomycin, cefoperazone and doxycycline. PLoS ONE. (2015) 10:e0117207. doi: 10.1371/journal.pone.0117207
31. Feng J, Li T, Yee R, Yuan Y, Bai C, Cai M, et al. Stationary phase persister/biofilm microcolony of Borrelia burgdorferi causes more severe disease in a mouse model of Lyme arthritis: implications for understanding persistence, Post-treatment Lyme Disease Syndrome (PTLDS), and treatment failure. Discov Med. (2019) 27:125–38.
32. Feng J, Shi W, Zhang S, Sullivan D, Auwaerter PG, Zhang Y. A drug combination screen identifies drugs active against amoxicillin-induced round bodies of in vitro Borrelia burgdorferi persisters from an FDA drug library. Front Microbiol. (2016) 7:743. doi: 10.3389/fmicb.2016.00743
33. Feng J, Zhang S, Shi W, Zhang Y. Activity of sulfa drugs and their combinations against stationary phase B. burgdorferi in vitro. Antibiotics. (2017) 6:E10. doi: 10.1101/112607
34. Steenbergen JN, Alder J, Thorne GM, Tally FP. Daptomycin: a lipopeptide antibiotic for the treatment of serious Gram-positive infections. J Antimicrob Chemother. (2005) 55:283–8. doi: 10.1093/jac/dkh546
35. Zhang Y, Yew WW, Barer MR. Targeting persisters for tuberculosis control. Antimicrob Agents Chemother. (2012) 56:2223–30. doi: 10.1128/AAC.06288-11
36. Deng S. Preliminary study on the prevention and treatment of leptospirosis with traditional Chinese medicine. Liaoning J Tradition Chin Med. (1985) 9:15–7.
37. Widhawati R, Hanani E, Zaini J. Andrographis Paniculata (Burm. F) nees induces clinical and sputum conversion in pulmonary tuberculosis patients medicine science. Int Med J. (2015) 4:1. doi: 10.5455/medscience.2014.03.8184
38. Borchardt JK. The beginnings of drug therapy: ancient mesopotamian medicine. Drug News Perspect. (2002) 15:187–92. doi: 10.1358/dnp.2002.15.3.840015
39. Di Lorenzo C, Ceschi A, Kupferschmidt H, Lude S, De Souza Nascimento E, Dos Santos A, et al. Adverse effects of plant food supplements and botanical preparations: a systematic review with critical evaluation of causality. Br J Clin Pharmacol. (2015) 79:578–92. doi: 10.1111/bcp.12519
40. Lude S, Vecchio S, Sinno-Tellier S, Dopter A, Mustonen H, Vucinic S, et al. Adverse effects of plant food supplements and plants consumed as food: results from the poisons centres-based PlantLIBRA study. Phytother Res. (2016) 30:988–96. doi: 10.1002/ptr.5604
41. Feng J, Zhang S, Shi W, Zubcevik N, Miklossy J, Zhang Y. Selective essential oils from spice or culinary herbs have high activity against stationary phase and biofilm Borrelia burgdorferi. Front Med. (2017) 4:169. doi: 10.3389/fmed.2017.00169
42. Feng J, Shi W, Miklossy J, Tauxe GM, Mcmeniman CJ, Zhang Y. Identification of essential oils with strong activity against stationary phase Borrelia burgdorferi. Antibiotics. (2018) 7:E89. doi: 10.3390/antibiotics7040089
43. The United States Pharmacopeial Convention. The United States Pharmacopeia. Philadelphia, PA (2000).
44. Theophilus PA, Victoria MJ, Socarras KM, Filush KR, Gupta K, Luecke DF, et al. Effectiveness of stevia rebaudiana whole leaf extract against the various morphological forms of Borrelia Burgdorferi in vitro. Eur J Microbiol Immunol. (2015) 5:268–80. doi: 10.1556/1886.2015.00031
45. Osafo N, Mensah KB, Yeboah OK. Phytochemical and pharmacological review of Cryptolepis sanguinolenta (Lindl.) Schlechter. Adv Pharmacol Sci. (2017). 2017:3026370. doi: 10.1155/2017/3026370
46. Olajide OA, Bhatia HS, De Oliveira AC, Wright CW, Fiebich BL. Anti-neuroinflammatory properties of synthetic cryptolepine in human neuroblastoma cells: possible involvement of NF-kappaB and p38 MAPK inhibition. Eur J Med Chem. (2013) 63:333–9. doi: 10.1016/j.ejmech.2013.02.004
47. Hanprasertpong N, Teekachunhatean S, Chaiwongsa R, Ongchai S, Kunanusorn P, Sangdee C, et al. Analgesic, anti-inflammatory, and chondroprotective activities of Cryptolepis buchanani extract: in vitro and in vivo studies. Biomed Res Int. (2014) 2014:978582. doi: 10.1155/2014/978582
48. Boakye-Yiadom K, Heman-Ackah SM. Cryptolepine hydrochloride effect on Staphylococcus aureus. J Pharm Sci. (1979) 68:1510–4. doi: 10.1002/jps.2600681212
49. Cimanga K, De Bruyne T, Lasure A, Van Poel B, Pieters L, Claeys M, et al. In vitro biological activities of alkaloids from Cryptolepis sanguinolenta. Planta Med. (1996) 62:22–7. doi: 10.1055/s-2006-957789
50. Mills-Robertson FC, Tay SC, Duker-Eshun G, Walana W, Badu K. In vitro antimicrobial activity of ethanolic fractions of Cryptolepis sanguinolenta. Ann Clin Microbiol Antimicrob. (2012) 11:16. doi: 10.1186/1476-0711-11-16
51. Sawer IK, Berry MI, Brown MW, Ford JL. The effect of cryptolepine on the morphology and survival of Escherichia coli, Candida albicans and Saccharomyces cerevisiae. J Appl Bacteriol. (1995) 79:314–21. doi: 10.1111/j.1365-2672.1995.tb03143.x
52. Tona L, Kambu K, Ngimbi N, Cimanga K, Vlietinck AJ. Antiamoebic and phytochemical screening of some Congolese medicinal plants. J Ethnopharmacol. (1998) 61:57–65. doi: 10.1016/S0378-8741(98)00015-4
53. Cimanga K, De Bruyne T, Pieters L, Vlietinck AJ, Turger CA. In vitro and in vivo antiplasmodial activity of cryptolepine and related alkaloids from Cryptolepis sanguinolenta. J Nat Prod. (1997) 60:688–91. doi: 10.1021/np9605246
54. Forkuo AD, Ansah C, Mensah KB, Annan K, Gyan B, Theron A, et al. In vitro anti-malarial interaction and gametocytocidal activity of cryptolepine. Malar J. (2017) 16:496. doi: 10.1186/s12936-017-2142-z
55. Bugyei KA, Boye GL, Addy ME. Clinical efficacy of a tea-bag formulation of Cryptolepis sanguinolenta root in the treatment of acute uncomplicated falciparum malaria. Ghana Med J. (2010) 44:3–9. doi: 10.4314/gmj.v44i1.68849
56. Ansah C, Gooderham NJ. The popular herbal antimalarial, extract of Cryptolepis sanguinolenta, is potently cytotoxic. Toxicol Sci. (2002) 70:245–51. doi: 10.1093/toxsci/70.2.245
57. Guittat L, Alberti P, Rosu F, Van Miert S, Thetiot E, Pieters L, et al. Interactions of cryptolepine and neocryptolepine with unusual DNA structures. Biochimie. (2003) 85:535–47. doi: 10.1016/S0300-9084(03)00035-X
58. Paulo A, Duarte A, Gomes ET. In vitro antibacterial screening of Cryptolepis sanguinolenta alkaloids. J Ethnopharmacol. (1994) 44:127–30. doi: 10.1016/0378-8741(94)90079-5
59. Ansah C, Otsyina H, Duwiejua M, Woode E, Aboagye F, Aning K. Toxicological assessment of Cryptolepis sanguinolenta for possible use in veterinary medicine. J Vet Med Animal Health. (2009) 1:11–6.
60. Buhner SH. Healing Lyme: Natural Healing of Lyme Borreliosis and the Coinfections Chlamydia and Spotted Fever Rickettsiosis. 2nd ed. Silver City, NM: Raven Press (2015).
61. Bi D, Zhao Y, Jiang R, Wang Y, Tian Y, Chen X, et al. Phytochemistry, bioactivity and potential impact on health of juglans: the original plant of walnut. Nat Prod Commun. (2016) 11:869–80. doi: 10.1177/1934578X1601100643
62. Ahmad T, Suzuki YJ. Juglone in oxidative stress and cell signaling. Antioxidants. (2019) 8:E91. doi: 10.3390/antiox8040091
63. Goc A, Rath M. The anti-borreliae efficacy of phytochemicals and micronutrients: an update. Ther Adv Infect Dis. (2016) 3:75–82. doi: 10.1177/2049936116655502
64. Karvonen K, Gilbert L. Effective killing of Borrelia burgdorferi in vitro with novel herbal compounds. General Medicine Open. (2018) 2:1–4. doi: 10.15761/GMO.1000153
65. Vu DC, Vo PH, Coggeshall MV, Lin CH. Identification and characterization of phenolic compounds in Black Walnut Kernels. J Agric Food Chem. (2018) 66:4503–11. doi: 10.1021/acs.jafc.8b01181
66. Nakayama M, Shimatani K, Ozawa T, Shigemune N, Tomiyama D, Yui K, et al. Mechanism for the antibacterial action of epigallocatechin gallate (EGCg) on Bacillus subtilis. Biosci Biotechnol Biochem. (2015) 79:845–54. doi: 10.1080/09168451.2014.993356
67. Ho K-V, Lei Z, Sumner L, Coggeshall M, Hsieh H-Y, Stewart G, et al. Identifying Antibacterial Compounds in Black Walnuts (Juglans nigra) Using a Metabolomics Approach. Metabolites. (2018) 8:58. doi: 10.3390/metabo8040058
68. Therapeutic Research Center. Natural Medicines Monograph: Black Walnut. (2019). Available online at: https://naturalmedicines.therapeuticresearch.com/ (accessed March 4, 2019).
69. Bonamonte D, Foti C, Angelini G. Hyperpigmentation and contact dermatitis due to Juglans regia. Contact Derm. (2001) 44:102–3. doi: 10.1034/j.1600-0536.2001.4402095.x
70. Neri I, Bianchi F, Giacomini F, Patrizi A. Acute irritant contact dermatitis due to Juglans regia. Contact Derm. (2006) 55:62–3. doi: 10.1111/j.0105-1873.2006.0847h.x
71. Siegel JM. Dermatitis due to black walnut juice. AMA Arch Dermatol Syphilol. (1954) 70:511–3. doi: 10.1001/archderm.1954.01540220113015
72. Aithal BK, Sunil Kumar MR, Rao BN, Upadhya R, Prabhu V, Shavi G, et al. Evaluation of pharmacokinetic, biodistribution, pharmacodynamic, and toxicity profile of free juglone and its sterically stabilized liposomes. J Pharm Sci. (2011) 100:3517–28. doi: 10.1002/jps.22573
73. Wu X, Li Q, Feng Y, Ji Q. Antitumor research of the active ingredients from traditional Chinese medical plant Polygonum cuspidatum. Evid Based Complement Alternat Med. (2018) 2018:2313021. doi: 10.1155/2018/2313021
74. Breuss JM, Atanasov AG, Uhrin P. Resveratrol and its effects on the vascular system. Int J Mol Sci. (2019) 20:E1523. doi: 10.3390/ijms20071523
75. Feng J, Shi W, Zhang S, Zhang Y. Identification of new compounds with high activity against stationary phase Borrelia burgdorferi from the NCI compound collection. Emerg Microbes Infect. (2015) 4:e31. doi: 10.1038/emi.2015.31
76. Kim JR, Oh DR, Cha MH, Pyo BS, Rhee JH, Choy HE, et al. Protective effect of polygoni cuspidati radix and emodin on Vibrio vulnificus cytotoxicity and infection. J Microbiol. (2008) 46:737–43. doi: 10.1007/s12275-008-0232-x
77. Pandit S, Kim HJ, Park SH, Jeon JG. Enhancement of fluoride activity against Streptococcus mutans biofilms by a substance separated from Polygonum cuspidatum. Biofouling. (2012) 28:279–87. doi: 10.1080/08927014.2012.672646
78. Shan B, Cai Y-Z, Brooks JD, Corke H. Antibacterial properties of Polygonum cuspidatum roots and their major bioactive constituents. Food Chem. (2008) 109:530–7. doi: 10.1016/j.foodchem.2007.12.064
79. La Porte C, Voduc N, Zhang G, Seguin I, Tardiff D, Singhal N, et al. Steady-state pharmacokinetics and tolerability of trans-resveratrol 2000mg twice daily with food, Quercetin and Alcohol (Ethanol) in healthy human subjects. Clin Pharmacokinet. (2010) 49:449–54. doi: 10.2165/11531820-000000000-00000
80. WHO. WHO Monograph on Good Agricultural and Collection Practices (GACP) for Artemisia annua L (2006).
81. Liu W, Liu Y. Youyou Tu: significance of winning the 2015 Nobel Prize in Physiology or Medicine. Cardiovasc Diagn Ther. (2016) 6:1–2. doi: 10.3978/j.issn.2223-3652.2015.12.11
82. Feng J, Weitner M, Shi W, Zhang S, Sullivan D, Zhang Y. Identification of additional anti-persister activity against Borrelia burgdorferi from an FDA drug library. Antibiotics. (2015) 4:397–410. doi: 10.3390/antibiotics4030397
83. Puri BK, Hakkarainen-Smith JS, Monro JA. The effect of artesunate on short-term memory in Lyme borreliosis. Med Hypotheses. (2017) 105:4–5. doi: 10.1016/j.mehy.2017.06.015
84. O'neill PM, Barton VE, Ward SA. The molecular mechanism of action of artemisinin—the debate continues. Molecules. (2010) 15:1705–21. doi: 10.3390/molecules15031705
85. Eckstein-Ludwig U, Webb RJ, Van Goethem ID, East JM, Lee AG, Kimura M, et al. Artemisinins target the SERCA of Plasmodium falciparum. Nature. (2003) 424:957–61. doi: 10.1038/nature01813
86. Wang J, Zhang CJ, Chia WN, Loh CC, Li Z, Lee YM, et al. Haem-activated promiscuous targeting of artemisinin in Plasmodium falciparum. Nat Commun. (2015) 6:10111. doi: 10.1038/ncomms10111
87. Munyangi J, Cornet-Vernet L, Idumbo M, Lu C, Lutgen P, Perronne C, et al. Artemisia annua and Artemisia afra tea infusions vs. artesunate-amodiaquine (ASAQ) in treating Plasmodium falciparum malaria in a large scale, double blind, randomized clinical trial. Phytomedicine. (2019) 57:49–56. doi: 10.1016/j.phymed.2018.12.002
88. Krebs S, Omer TN, Omer B. Wormwood (Artemisia absinthium) suppresses tumour necrosis factor alpha and accelerates healing in patients with Crohn's disease - a controlled clinical trial. Phytomedicine. (2010) 17:305–9. doi: 10.1016/j.phymed.2009.10.013
89. Muto T, Watanabe T, Okamura M, Moto M, Kashida Y, Mitsumori K. Thirteen-week repeated dose toxicity study of wormwood (Artemisia absinthium) extract in rats. J Toxicol Sci. (2003) 28:471–8. doi: 10.2131/jts.28.471
90. Haldar K, Bhattacharjee S, Safeukui I. Drug resistance in Plasmodium. Nat Rev Microbiol. (2018) 16:156. doi: 10.1038/nrmicro.2017.161
92. Daddy NB, Kalisya LM, Bagire PG, Watt RL, Towler MJ, Weathers PJ. Artemisia annua dried leaf tablets treated malaria resistant to ACT and i.v. artesunate: case reports. Phytomedicine. (2017) 32:37–40. doi: 10.1016/j.phymed.2017.04.006
93. Dinda B, Dinda S, Dassharma S, Banik R, Chakraborty A, Dinda M. Therapeutic potentials of baicalin and its aglycone, baicalein against inflammatory disorders. Eur J Med Chem. (2017) 131:68–80. doi: 10.1016/j.ejmech.2017.03.004
94. Liang W, Huang X, Chen W. The effects of baicalin and baicalein on cerebral ischemia: a review. Aging Dis. (2017) 8:850–67. doi: 10.14336/AD.2017.0829
95. Cheng CS, Chen J, Tan HY, Wang N, Chen Z, Feng Y. Scutellaria baicalensis and cancer treatment: recent progress and perspectives in biomedical and clinical studies. Am J Chin Med. (2018) 46:25–54. doi: 10.1142/S0192415X18500027
96. Sowndhararajan K, Deepa P, Kim M, Park SJ, Kim S. Neuroprotective and cognitive enhancement potentials of baicalin: a review. Brain Sci. (2018) 8:E104. doi: 10.3390/brainsci8060104
97. Goc A, Niedzwiecki A, Rath M. In vitro evaluation of antibacterial activity of phytochemicals and micronutrients against Borrelia burgdorferi and Borrelia garinii. J Appl Microbiol. (2015) 119:1561–72. doi: 10.1111/jam.12970
98. Ming J, Zhuoneng L, Guangxun Z. Protective role of flavonoid baicalin from Scutellaria baicalensis in periodontal disease pathogenesis: a literature review. Complement Ther Med. (2018) 38:11–8. doi: 10.1016/j.ctim.2018.03.010
99. Fujita M, Shiota S, Kuroda T, Hatano T, Yoshida T, Mizushima T, et al. Remarkable synergies between baicalein and tetracycline, and baicalein and β-lactams against methicillin-resistant Staphylococcus aureus. Microbiol Immunol. (2005) 49:391–6. doi: 10.1111/j.1348-0421.2005.tb03732.x
100. Cai W, Fu Y, Zhang W, Chen X, Zhao J, Song W, et al. Synergistic effects of baicalein with cefotaxime against Klebsiella pneumoniae through inhibiting CTX-M-1 gene expression. BMC Microbiol. (2016) 16:181. doi: 10.1186/s12866-016-0797-1
101. Wang J, Qiao M, Zhou Y, Du H, Bai J, Yuan W, et al. In vitro synergistic effect of baicalin with azithromycin against Staphylococcus saprophyticus isolated from francolins with ophthalmia. Poult Sci. (2019) 98:373–80. doi: 10.3382/ps/pey356
102. Luo J, Dong B, Wang K, Cai S, Liu T, Cheng X, et al. Baicalin inhibits biofilm formation, attenuates the quorum sensing-controlled virulence and enhances Pseudomonas aeruginosa clearance in a mouse peritoneal implant infection model. PLoS ONE. (2017) 12:e0176883. doi: 10.1371/journal.pone.0176883
103. Gol'dberg V, Ryzhakov V, Matiash M, Stepovaia E, Boldyshev D, Litvinenko V, et al. Dry extract of Scutellaria baicalensis as a hemostimulant in antineoplastic chemotherapy in patents with lung cancer. Eksp Klin Farmakol. (1997) 60:28–30.
104. Smol'ianinov ES, Gol'dberg VE, Matiash MG, Ryzhakov VM, Boldyshev DA, Litvinenko VI, et al. Effect of Scutellaria baicalensis extract on the immunologic status of patients with lung cancer receiving antineoplastic chemotherapy. Eksp Klin Farmakol. (1997) 60:49–51.
105. Hui KM, Wang XH, Xue H. Interaction of flavones from the roots of Scutellaria baicalensis with the benzodiazepine site. Planta Med. (2000) 66:91–3. doi: 10.1055/s-0029-1243121
106. Chang HH, Yi PL, Cheng CH, Lu CY, Hsiao YT, Tsai YF, et al. Biphasic effects of baicalin, an active constituent of Scutellaria baicalensis Georgi, in the spontaneous sleep-wake regulation. J Ethnopharmacol. (2011) 135:359–68. doi: 10.1016/j.jep.2011.03.023
107. Chalasani N, Vuppalanchi R, Navarro V, Fontana R, Bonkovsky H, Barnhart H, et al. Acute liver injury due to flavocoxid (Limbrel), a medical food for osteoarthritis: a case series. Ann Intern Med. (2012) 156:857–60; W297–300. doi: 10.7326/0003-4819-156-12-201206190-00006
108. Yimam M, Zhao Y, Ma W, Jia Q, Do SG, Shin JH. 90-day oral toxicity study of UP446, a combination of defined extracts of Scutellaria baicalensis and Acacia catechu, in rats. Food Chem Toxicol. (2010) 48:1202–9. doi: 10.1016/j.fct.2010.02.011
109. Puri BK, White N, Monro JA. The effect of supplementation with Scutellaria baicalensis on hepatic function. Med Hypotheses. (2019) 133:109402. doi: 10.1016/j.mehy.2019.109402
110. Snow AD, Castillo GM, Nguyen BP, Choi PY, Cummings JA, Cam J, et al. The Amazon rain forest plant Uncaria tomentosa (cat's claw) and its specific proanthocyanidin constituents are potent inhibitors and reducers of both brain plaques and tangles. Sci Rep. (2019) 9:561. doi: 10.1038/s41598-019-38645-0
111. De Paula LC, Fonseca F, Perazzo F, Cruz FM, Cubero D, Trufelli DC, et al. Uncaria tomentosa (cat's claw) improves quality of life in patients with advanced solid tumors. J Altern Complement Med. (2015) 21:22–30. doi: 10.1089/acm.2014.0127
112. Sheng Y, Li L, Holmgren K, Pero RW. DNA repair enhancement of aqueous extracts of Uncaria tomentosa in a human volunteer study. Phytomedicine. (2001) 8:275–82. doi: 10.1078/0944-7113-00045
113. Mur E, Hartig F, Eibl G, Schirmer M. Randomized double blind trial of an extract from the pentacyclic alkaloid-chemotype of Uncaria tomentosa for the treatment of rheumatoid arthritis. J Rheumatol. (2002) 29:678–81.
114. Piscoya J, Rodriguez Z, Bustamante SA, Okuhama NN, Miller MJ, Sandoval M. Efficacy and safety of freeze-dried cat's claw in osteoarthritis of the knee: mechanisms of action of the species Uncaria guianensis. Inflamm Res. (2001) 50:442–8. doi: 10.1007/PL00000268
115. Datar AN, Kaur N, Patel S, Luecke DF, Sapi E. In Vitro Effectiveness of Samento and Banderol Herbal Extracts on Different Morphological Forms of Borrelia Burgdorferi. University of New Haven (2010).
116. Herrera DR, Durand-Ramirez JE, Falcao A, Silva EJ, Santos EB, Gomes BP. Antimicrobial activity and substantivity of Uncaria tomentosa in infected root canal dentin. Braz Oral Res. (2016) 30:e61. doi: 10.1590/1807-3107BOR-2016.vol30.0061
117. Keplinger K, Laus G, Wurm M, Dierich MP, Teppner H. Uncaria tomentosa (Willd.) DC.—Ethnomedicinal use and new pharmacological, toxicological and botanical results. J Ethnopharmacol. (1998) 64:23–34. doi: 10.1016/S0378-8741(98)00096-8
118. Chinou I, Demetzos C, Harvala C, Roussakis C, Verbist JF. Cytotoxic and antibacterial labdane-type diterpenes from the aerial parts of Cistus incanus subsp. creticus Planta Med. (1994) 60:34–6. doi: 10.1055/s-2006-959403
119. Kalus U, Kiesewetter H, Radtke H. Effect of CYSTUS052 and green tea on subjective symptoms in patients with infection of the upper respiratory tract. Phytother Res. (2010) 24:96–100. doi: 10.1002/ptr.2876
120. Hutschenreuther A, Birkemeyer C, Grotzinger K, Straubinger RK, Rauwald HW. Growth inhibiting activity of volatile oil from Cistus creticus L. against Borrelia burgdorferi ss in vitro. Pharmazie. (2010) 65:290–5. doi: 10.1691/ph.2010.9762
121. Hickl J, Argyropoulou A, Sakavitsi ME, Halabalaki M, Al-Ahmad A, Hellwig E, et al. Mediterranean herb extracts inhibit microbial growth of representative oral microorganisms and biofilm formation of Streptococcus mutans. PLoS ONE. (2018) 13:e0207574. doi: 10.1371/journal.pone.0207574
122. Hannig C, Sorg J, Spitzmuller B, Hannig M, Al-Ahmad A. Polyphenolic beverages reduce initial bacterial adherence to enamel in situ. J Dent. (2009) 37:560–6. doi: 10.1016/j.jdent.2009.03.017
123. Papaefthimiou D, Papanikolaou A, Falara V, Givanoudi S, Kostas S, Kanellis AK. Genus Cistus: a model for exploring labdane-type diterpenes' biosynthesis and a natural source of high value products with biological, aromatic, and pharmacological properties. Front Chem. (2014) 2:35. doi: 10.3389/fchem.2014.00035
124. Ehrhardt C, Hrincius ER, Korte V, Mazur I, Droebner K, Poetter A, et al. A polyphenol rich plant extract, CYSTUS052, exerts anti influenza virus activity in cell culture without toxic side effects or the tendency to induce viral resistance. Antiviral Res. (2007) 76:38–47. doi: 10.1016/j.antiviral.2007.05.002
125. Brorson O, Brorson SH. Grapefruit seed extract is a powerful in vitro agent against motile and cystic forms of Borrelia burgdorferi sensu lato. Infection. (2007) 35:206–8. doi: 10.1007/s15010-007-6105-0
126. Von Woedtke T, Schluter B, Pflegel P, Lindequist U, Julich WD. Aspects of the antimicrobial efficacy of grapefruit seed extract and its relation to preservative substances contained. Pharmazie. (1999) 54:452–6.
127. Avula B, Dentali S, Khan IA. Simultaneous identification and quantification by liquid chromatography of benzethonium chloride, methyl paraben and triclosan in commercial products labeled as grapefruit seed extract. Pharmazie. (2007) 62:593–6. doi: 10.1691/ph.2007.8.6261
128. Takeoka G, Dao L, Wong RY, Lundin R, Mahoney N. Identification of benzethonium chloride in commercial grapefruit seed extracts. J Agric Food Chem. (2001) 49:3316–20. doi: 10.1021/jf010222w
Keywords: Borrelia burgdorferi, Lyme disease, persisters, botanical medicines, herbs, natural medicines, antimicrobial activity, biofilm
Citation: Feng J, Leone J, Schweig S and Zhang Y (2020) Evaluation of Natural and Botanical Medicines for Activity Against Growing and Non-growing Forms of B. burgdorferi. Front. Med. 7:6. doi: 10.3389/fmed.2020.00006
Received: 22 August 2019; Accepted: 09 January 2020;
Published: 21 February 2020.
Edited by:
Marc Jean Struelens, European Centre for Disease Prevention and Control (ECDC), SwedenReviewed by:
SriSowmya Sanisetty, Independent Researcher, Cambridge, United StatesAjay Kumar Sharma, Institute of Nuclear Medicine & Allied Sciences, India
Copyright © 2020 Feng, Leone, Schweig and Zhang. This is an open-access article distributed under the terms of the Creative Commons Attribution License (CC BY). The use, distribution or reproduction in other forums is permitted, provided the original author(s) and the copyright owner(s) are credited and that the original publication in this journal is cited, in accordance with accepted academic practice. No use, distribution or reproduction is permitted which does not comply with these terms.
*Correspondence: Sunjya Schweig, c3VuanlhQGNjZm1lZC5jb20=; Ying Zhang, eXpoYW5nQGpoc3BoLmVkdQ==
†Present address: Jie Feng, Institute of Pathogen Biology, School of Basic Medical Sciences, Lanzhou University, Lanzhou, China